Now, let’s cover the TEM. The best way to get to know the TEM is to understand where and why you’d use one. When would you ever need to see something so small that light can never penetrate it? It’s like staring at an ant—but in space. This is not a microparticle microscope you’d find in a chemistry lab. This is a microscope used with industrial strength, on levels millions of times smaller than normal sight—2 nanometer resolution. That’s nearly atomic! But when did this technology become a reality? In the 1920s, it all started to come together. Louis de Broglie theorized that electrons were particles and waves. Like a vibrating guitar string held in place by a finger pluck resonates in energy while simultaneously playing one note. Thus, switching to electron waves, as opposed to photons, to observe something made even more sense and was even easier to achieve.
Two years later, in 1931, the electron microscope was created. The invisible lenses were magnetic fields, and Ruska and Knoll directed those beams of electrons into a focus as one would beams of photons. It was like using a laser instead of a lightbulb. Ruska would subsequently release a prototype with Siemens, and by 1933, there existed a transmission electron microscope (TEM).
Why does this matter? What’s the difference between reading the fine print of a contract with a jeweler’s loupe and having access to a state-of-the-art text scanner? A transmission electron microscope goes beyond magnification—a transmission electron microscope enables researchers to view material structures at the atomic level, granting information about material structures, compositions, and, at times, arrangements of atoms.
Although this process seems like a futile exercise for researchers who want to know for the sake of knowing, it grants materials scientists the power to develop new, stronger materials and doctors a better understanding of how disease manifests at the cellular level. Because when you can see distinctions that are 0.2 micrometers apart, it’s more than micro—it’s investigative. Ultimately, TEMs are integral to modern scientific research. They have become the aperture through which we explore an entire universe of sub-atomic and nanoscale possibilities—and discoveries—one infinitesimal detail at a time.
What is transmission electron microscope?
The Transmission Electron Microscope (TEM) is a type of newer generation, high powered electron microscope. Essentially, the TEM “photos an object using a focused electron beam, resulting in high magnification and high detector imaging (over 2 million times that of a regular light microscope).”
Therefore, the imaging capabilities allow for greater study of materials’ morphology, composition, and crystallization on a nanoscale. Furthermore, the imaging occurs with particles as small as 2 nm with a working resolution limit of 0.2 microns, meaning this is the strongest microscope used in a lab setting. The story of the Transmission Electron Microscope (TEM) started in the twentieth century. Cathode rays were determined as electrons by Louis de Broglie.
Subsequently, de Broglie’s research in the 1920s concerning the wave nature of electrons led to the formation of the electron microscope. By this time, cathode rays were the electrons and magnetic fields were used as lenses to focus and aim the beams of electrons. The first electron microscope was constructed in 1931—not by two men simultaneously, Ernst Ruska and Max Knoll. Two years later, Ruska worked with the Siemens corporation to construct the first TEM.
Advantages of using a transmission electron microscope include that it’s relatively easy to use and provides relatively high magnification and resolution in comparison to a light microscope. This scientific instrument is important because it allows for the microanalysis of a variety of samples at very low levels.
Parts of A Transmission Electron Microscope
Three Basic Components of the Scanning Electron Microscope:
- Electron Gun- This is the source of the electron beam. A tungsten or lanthanum hexaboride filament emits electrons when heated. The resulting beam travels at such high energies (80 to 300 kiloelektron volts (keV)) that it penetrates the sample.
- Vacuum System – This is the component that establishes and maintains high vacuum conditions within the microscope so that air does not scatter the electrons, producing inaccurate images.
- Condenser Lens System– This is the series of electromagnetic lenses that focus the electron beam on the specimen and allow the experimenter to control the intensity and convergence of the beam.
- Specimen Holder- A slide containing the ultrathin specimen which allows for positioning and angling to be precise within the electron beam trajectory.
- Condenser Lens- The primary imaging lens which intensifies the electrons that pass through the specimen to generate an image.
- Projection Lens- The lens which magnifies the image developed by the objective lens and projects it onto a monitor.
- Condenser Apertures- Vertical openings located within the center of the column which decrease aberrations and enhance focus.
- Detectors and Viewing System – Watch and assess the final magnified image that was created on fluorescent screens, digital cameras, and other detectors.
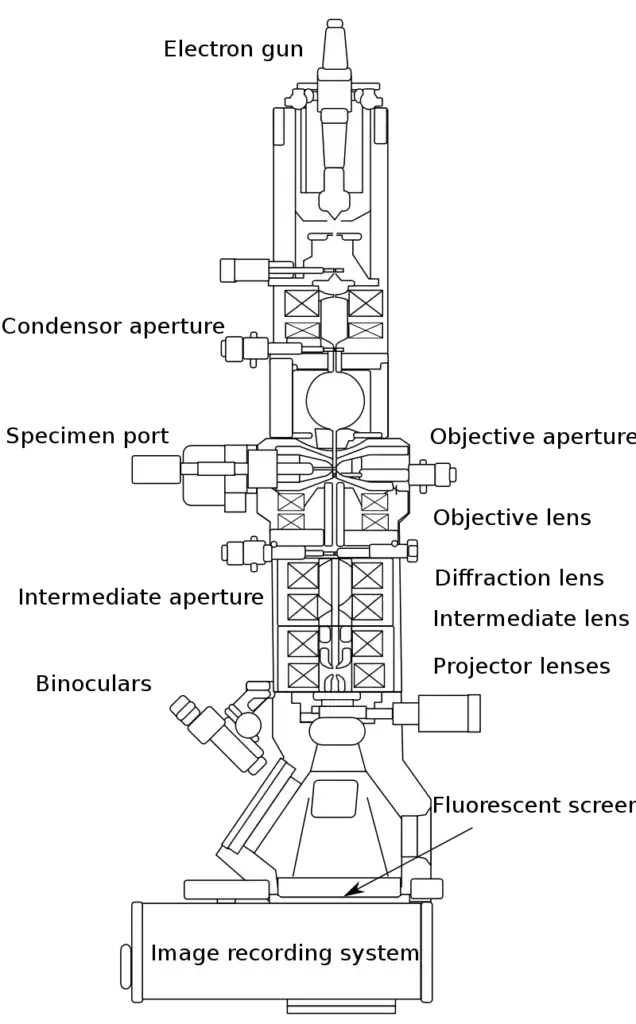
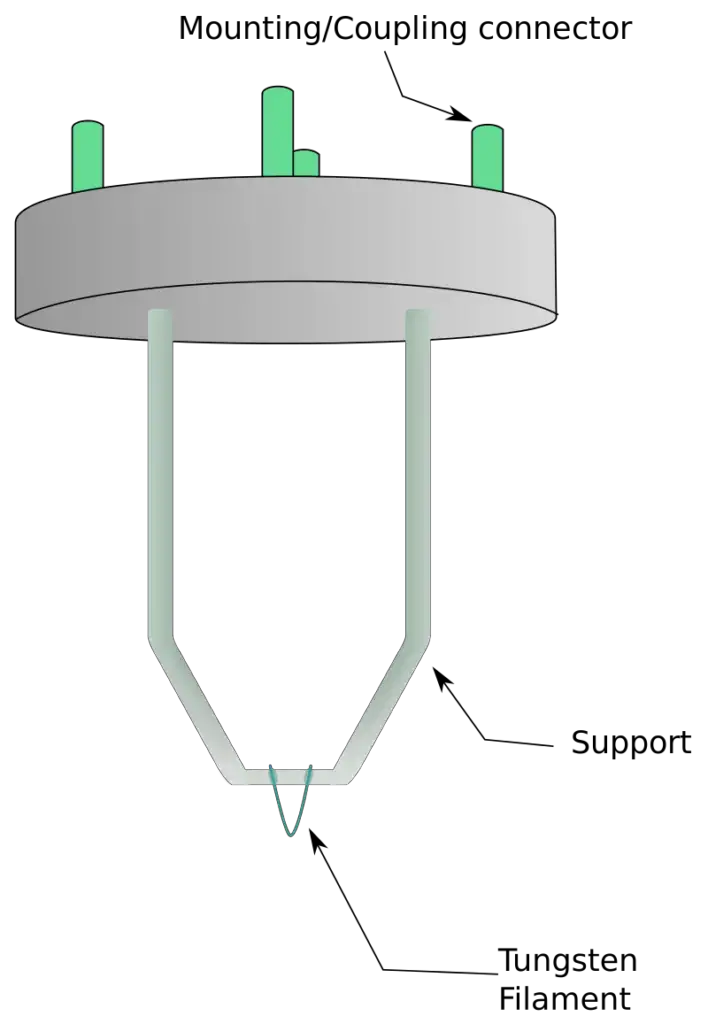
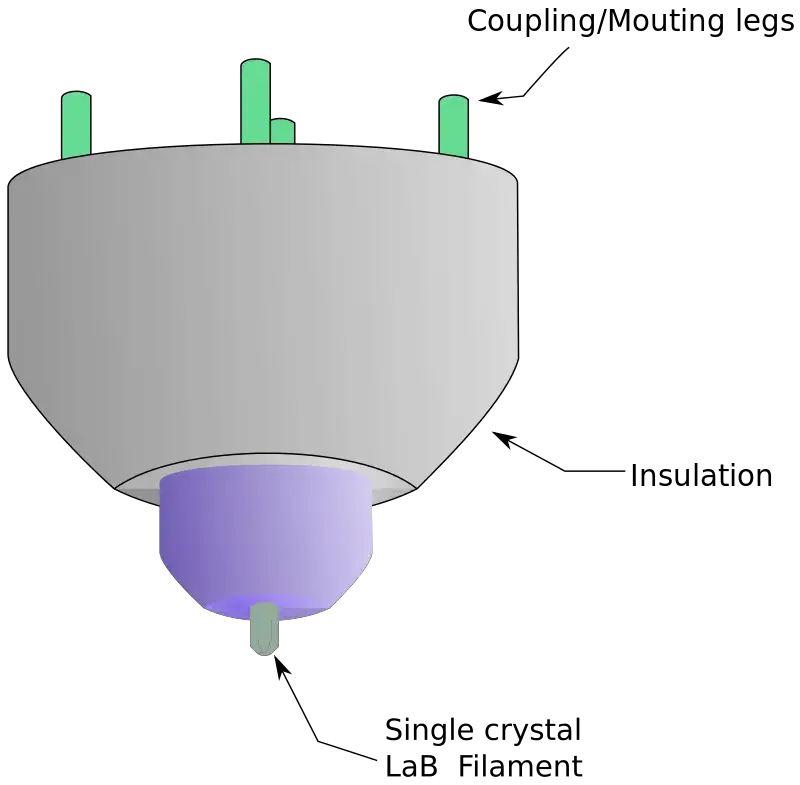
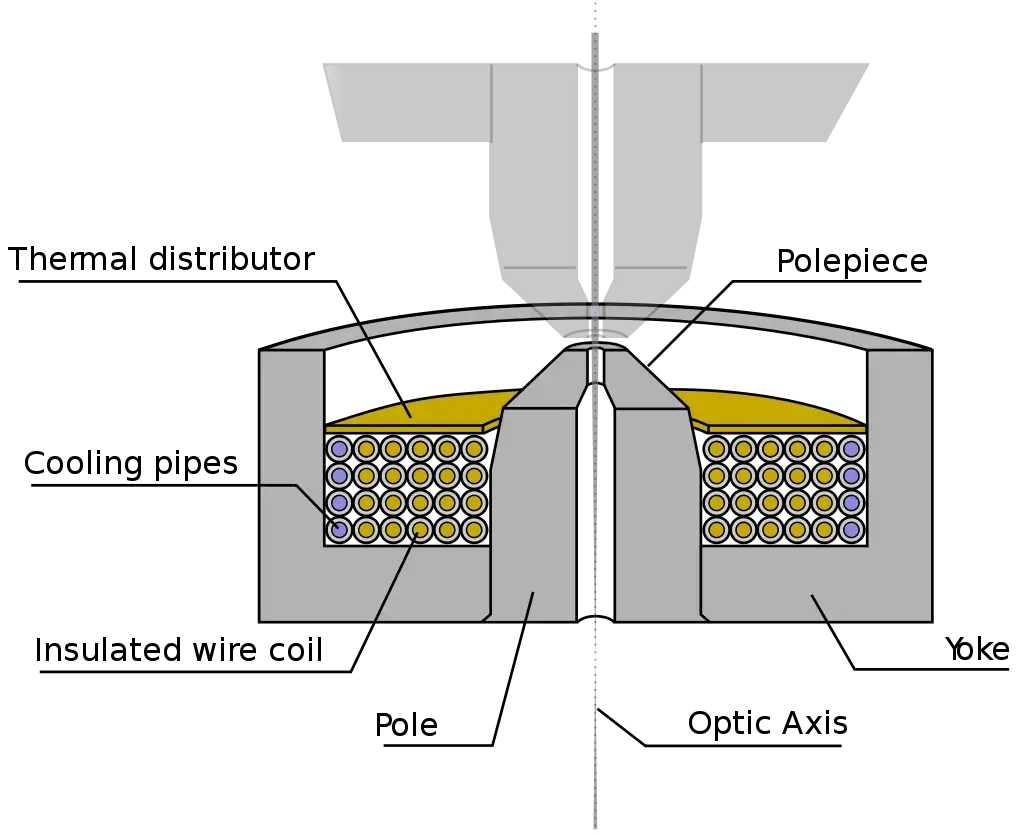
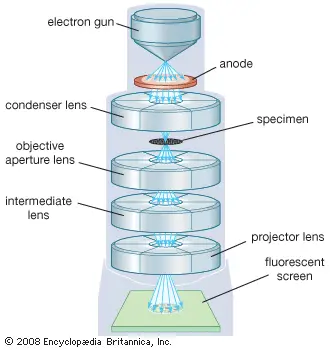
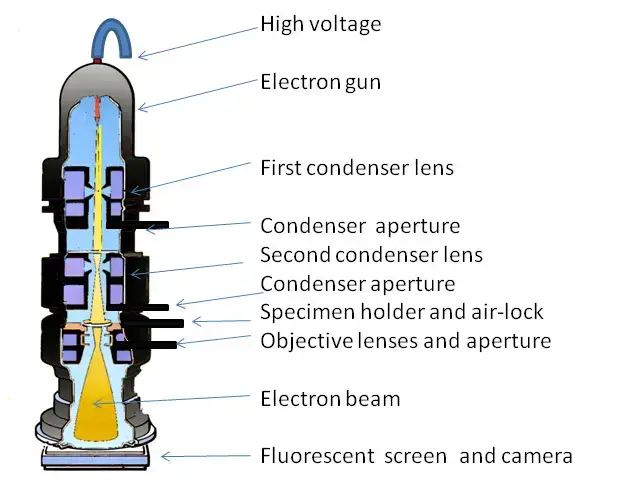
Working principle of Transmission Electron Microscope
The way TEM works is that a high-energy beam of electrons is sent through a sample to visualize what is occurring within. However, unlike a light microscope which uses light to visualize, a TEM uses electrons as a beam because the wavelength of electrons is smaller than that of the visible light spectrum, and thus, the resolution is that much higher. First, a beam of electrons is created using an electron gun and focused using two condenser lenses. Second, the electron beam is sent through the sample. Third, based on the structure of the sample, the electrons are either transmitted or scattered based on electron density and composition. The transmitted scanners are then focused through an objective lens and projector lens to assess the image on a digital detector or a fluorescent screen. Because this must all happen in a vacuum—where any air molecules can scatter and deflect the image—scientists can use the information compiled to their advantage. Thus, TEM is used across various technologies within nanotechnology, biology, and material sciences because it allows for an image at an atomic level.
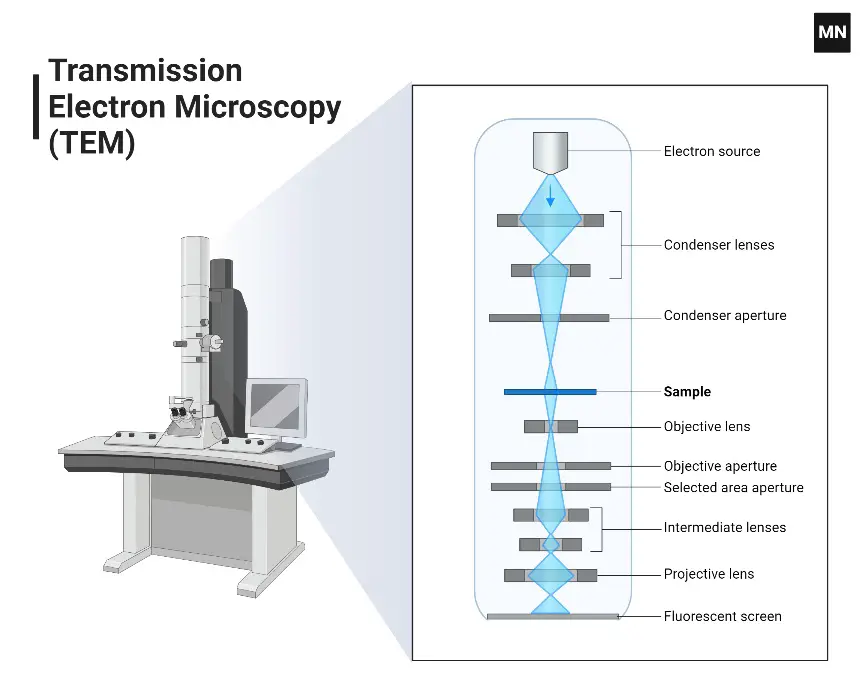
How does the transmission electron microscope work?
- First, a tungsten filament is heated—also called an electron gun. This heated tungsten filament/electron gun will begin to create beams of electrons.
- An electromagnetic coil and high voltage (up to several million volts) are applied to these beams of electrons to give them velocity (extraordinarily high velocity).
- An aperture-type condenser lens cuts out all the high-angle electrons and collimates all the beams of electrons into a tight, focused beam.
- Now the beams of electrons, at high speed, are directed through the specimen.
- The beams of electrons that are transmitted are focused into an image by an objective lens. The
- TEM’s vacuums chamber guarantees that the electron beams will never interact with the gaseous particles. The gaseous particles interact with the fluorescent screen, which either reveals the silhouette of the specimen or a micrograph image.
- The CCD camera, which captures all micrographs, is located just below the screen.
Sample Preparation for TEM
TEM sample preparation is intricate and depends on the variety of sample, whether biological or materials, and which properties are studied. TEM sample preparation involves the following:
- Fixation- Biological specimens require fixation to avoid deterioration and maintain a biological state for future study. This is achieved through the use of chemical fixatives such as glutaraldehyde, formaldehyde, and osmium tetroxide. The first two encourage cross-linking of proteins, while the last one bonds to lipids.
- Dehydration- Dehydration comes next, as water must be avoided in the TEM, which runs under a vacuum. This is achieved through graded ethanol or acetone in increasing percentages of each solvent.
- Embedding- After dehydration, the resin (epoxy, acrylic) used to harden and preserve the structure after cutting infiltrates the tissue. The liquid resin solvent has a transition solvent—propylene oxide—that acclimates the tissue for infiltration and polymerization once infiltrated to solidify it into a block.
- Sectioning- An ultramicrotome with a glass or diamond knife cuts the resin block into a super thin section. These sections are about 50 to 100 nanometers thick and are placed onto metal grids for observation.
- Staining- In order to enhance contrast, sections are stained with electron-dense heavy metals. The two most common are uranyl acetate and lead citrate, which bind to certain cellular components and provide the contrast required for TEM visualization. Stained sections are then viewed with the light microscope to see the sections better and decide what areas are of interest. That material is either excised/included on MEM grids and then, depending upon whether the state change during the process requires additional contrast, additional stains are added before placing in the TEM for imaging.
As for samples where biological material is not involved, say metal or semiconductor materials, the processing would be:
- Mechanical Sawing and Lapping: The materials are sawed into smaller pieces and then thinned via lapping and polishing to reach a TEM thin level.
- Ion milling: The specimen can be ion milled as well to obtain electron transparency. Ion milling is an ion-based process to thin the specimen further whereby ions enter the specimen at a certain depth level to essentially chip away material.
These are just a few of the steps involved, all crucial and connected to the particular specimen and the requirements for imaging for TEM.
Image Formation in TEM
- Once the electrons leave the electron gun, the condenser lens focuses the beam onto the material of interest.
- The condenser aperture strips high-angle electrons from the beam.
- Next, a small portion of the beam is allowed to interact with the material and pass through. An objective lens focuses this transmitted beam either onto a phosphorescent screen or CCD camera, rendering the image.
- A second objective aperture strips high-angle diffracted electrons from passing through as well, enhancing image resolution.
- Finally, the beam passes through the column and projector lens for further optical magnification. It’s this confluence of moments that create what the image renders to the viewer.
- The dark areas are where fewer electrons were emitted to the sample from the micrograph. The bright areas are where more electrons were emitted to the sample from the micrograph.
What is Diffraction Point?
Once the electrons have passed through the specimen, it is found that they scatter because of the electrostatic potential created by the superimposed atoms that make up the specimen. Thus, the electrons that are transmitted pass through an electromagnetic objective lens which, in turn, focuses all of the scattered electrons coming from one point of interest in the specimen to one point of interest in the image plane. Additionally, there is a dotted line in the subsequent image where all of the scattered electrons—those scattered by the specimen in the uniform direction—converge to one point as well. This is called the back focal plane of the objective lens. This is where the diffraction point exists.
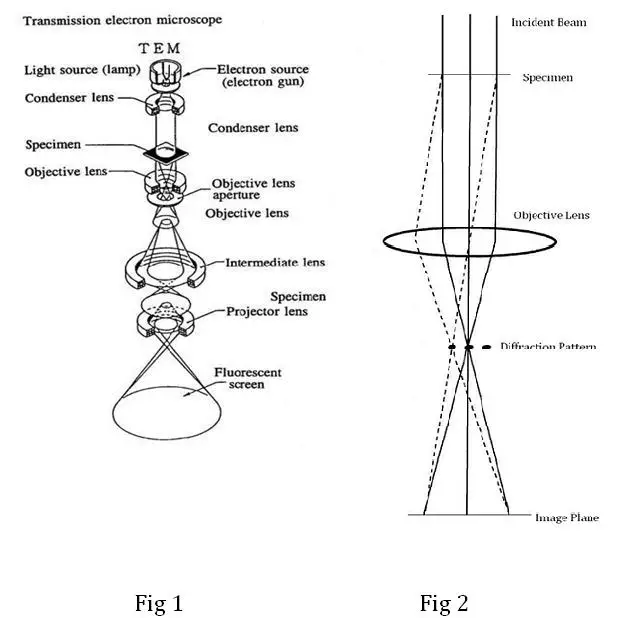
Application of Transmission Electron Microscope
- It is used to examine the microstructure of materials in materials science.
- It characterizes nanoparticles and nanostructures in nanotechnology.
- It studies viruses, bacteria, and cellular structures in life sciences.
- It analyzes microstructures for advanced devices in the semiconductor industry.
- It helps study energy materials like batteries and fuel cells.
- It is used for analyzing pollutants and environmental samples.
- It examines microscopic evidence in forensic investigations.
- It reveals atomic structures in material science research.
- It characterizes nanoparticles for nanotechnology and advanced materials.
- It studies cellular structures and viruses in life sciences.
- It helps identify defects in semiconductor manufacturing.
- It aids in developing efficient energy storage systems.
- It analyzes pollutants and particulates for environmental monitoring.
- It examines microscopic evidence for criminal investigations.
- It observes crystal structures in metals and alloys.
- It provides detailed imaging of thin samples at high resolution.
- It helps in studying corrosion and material degradation.
Advantages of Transmission Electron Microscope (TEM)
- It offers high-resolution imaging at atomic levels.
- It provides detailed structural information of samples.
- It allows for imaging of thin specimen sections.It enables visualization of internal structures in materials.
- It offers versatile imaging modes, including dark and bright field.
- It allows for electron diffraction analysis to study crystallography.
- It provides elemental analysis through energy-dispersive X-ray spectroscopy.
- It allows for in-situ observation of dynamic processes.
- It offers high magnification, exceeding one million times.
- It provides detailed imaging of nanoscale materials.
Limitations of Transmission Electron Microscope (TEM)
- It requires samples to be extremely thin for electron transmission.
- Sample preparation can introduce artifacts and damage.
- It requires a high-vacuum environment, unsuitable for living samples.
- It is a time-consuming process with low sample throughput.
- It requires expensive equipment and maintenance.
- It can damage sensitive samples due to electron beam exposure.
- It requires extensive sample preparation, which can alter structures.It has a limited field of view, potentially missing sample heterogeneity.
- It requires specialized training to operate and interpret results.
- It is unsuitable for imaging thick or bulk samples.
Differences between a SEM and a TEM
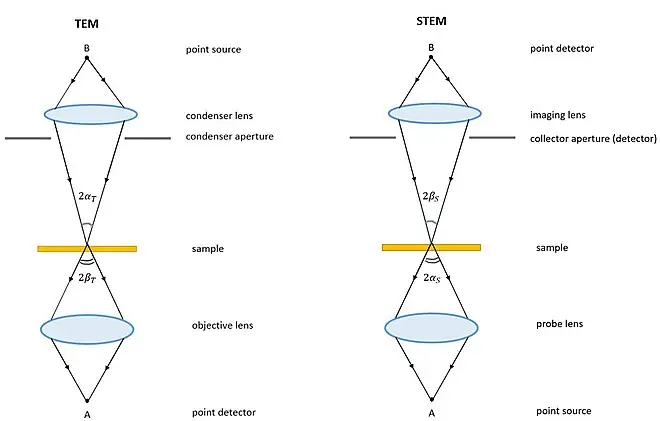
SEM | TEM | |
Type of electrons | Scattered, scanning electrons | Transmitted electrons |
High tension | ~1–30 kV | ~60–300 kV |
Specimen thickness | Any | Typically <150 nm |
Type of info | 3D image of surface | 2D projection image of inner structure |
Max. magnification | Up to ~1–2 million times | More than 50 million times |
Max. FOV | Large | Limited |
Optimal spatial resolution | ~0.5 nm | <50 pm |
Image formation | Electrons are captured and counted by detectors, image on PC screen | Direct imaging on fluorescent screen or PC screen with CCD |
Operation | Little or no sample preparation, easy to use | Laborious sample preparation, trained users required |
Transmission Electron Microscope Definition, Parts, Working Principle, Applications, Advantages – Video
Transmission electron microscope images
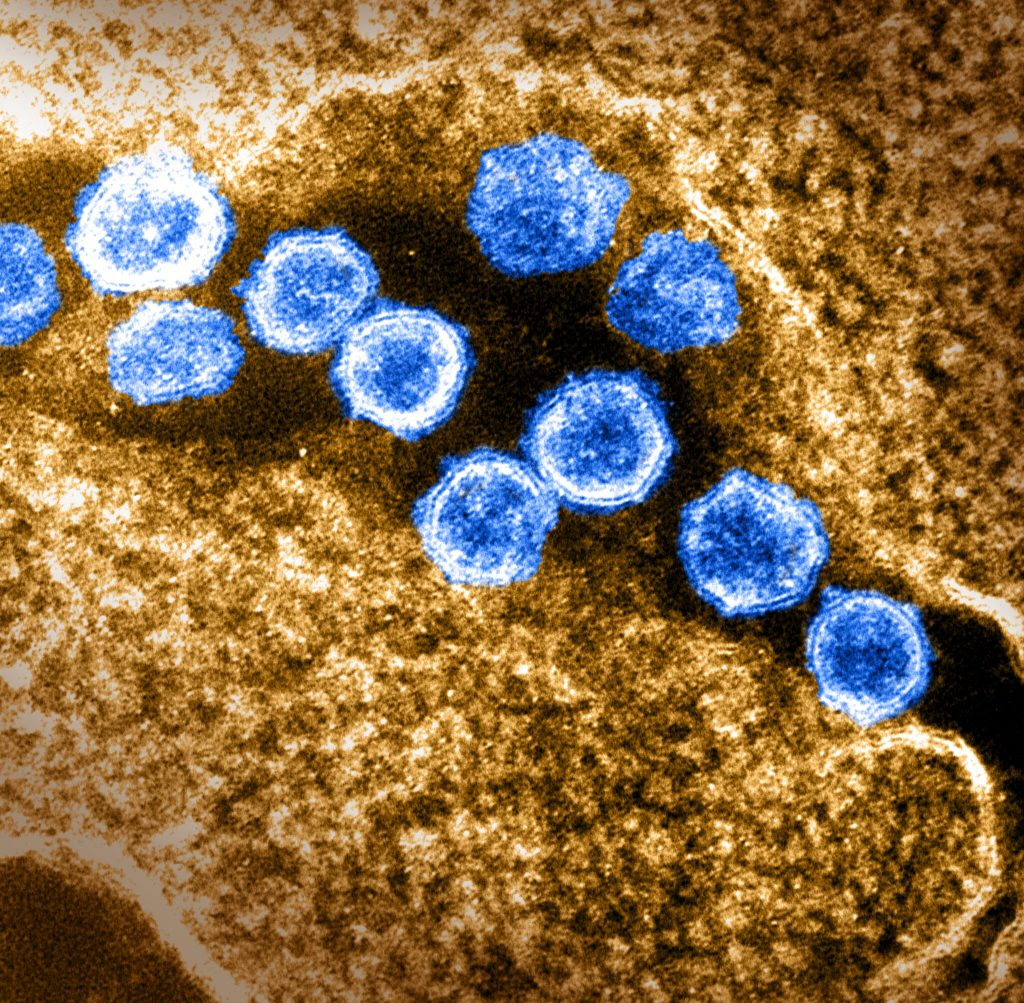
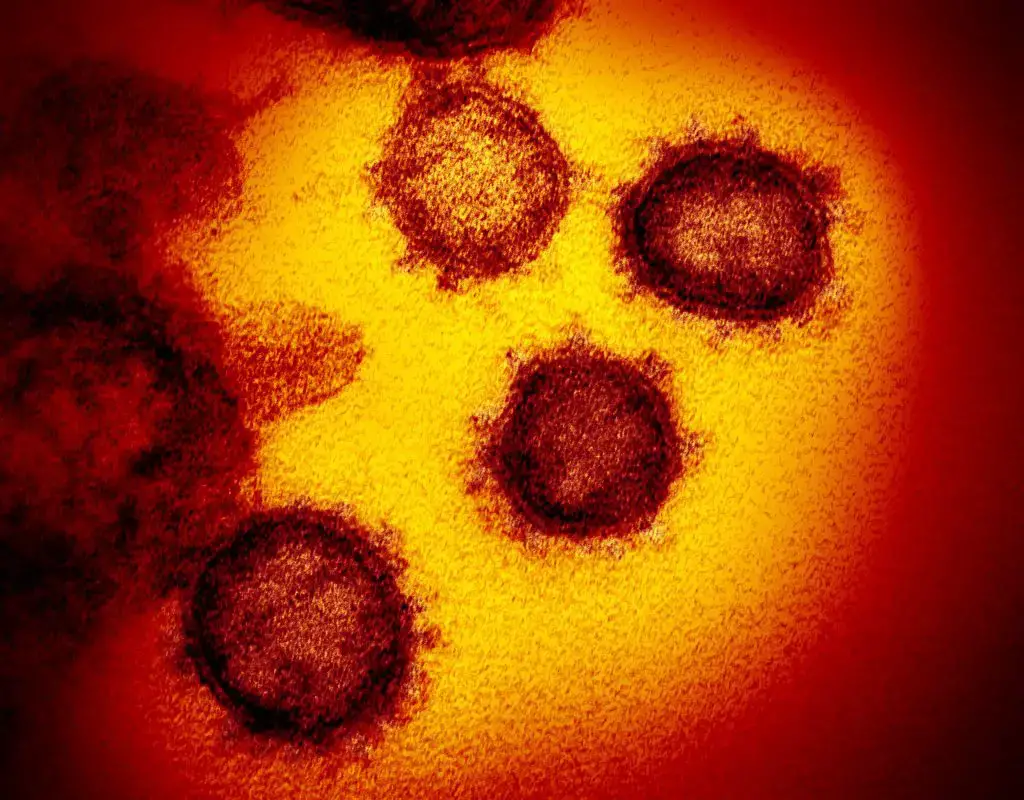
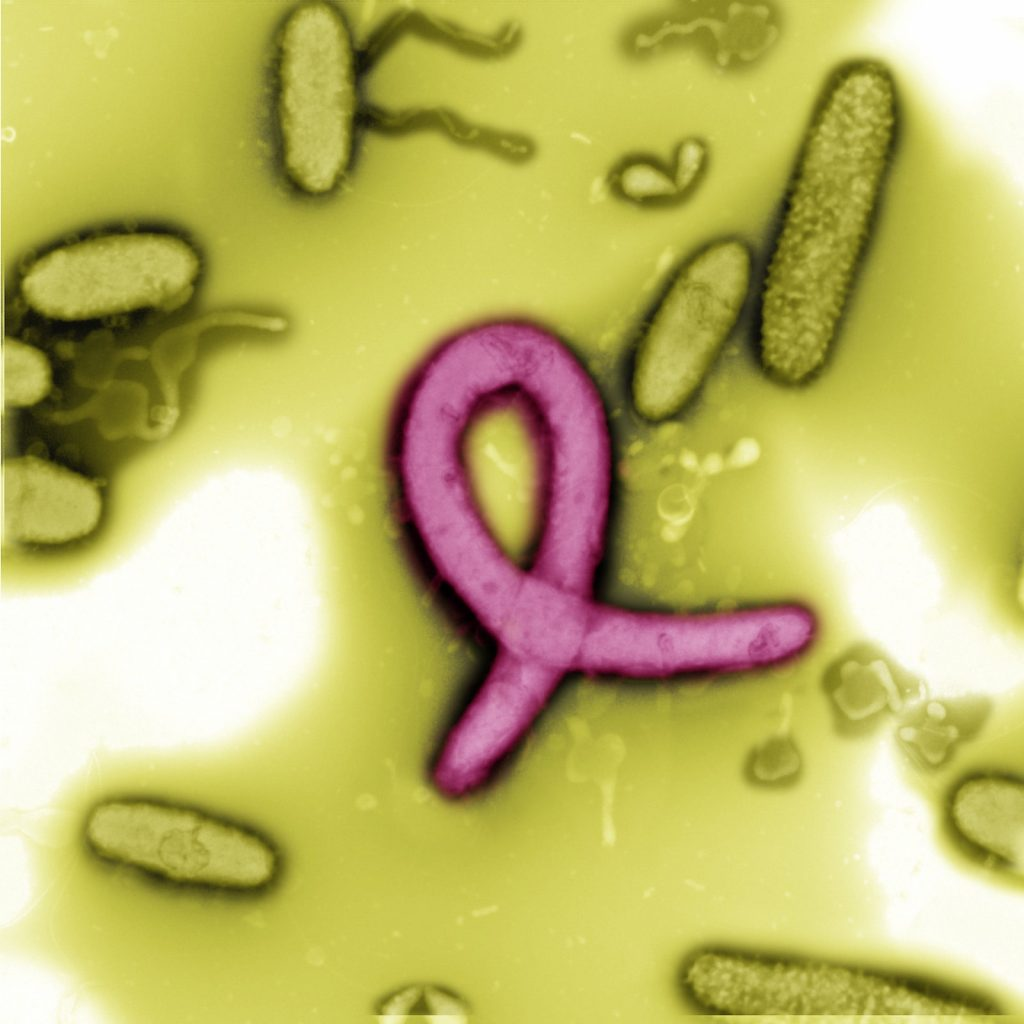
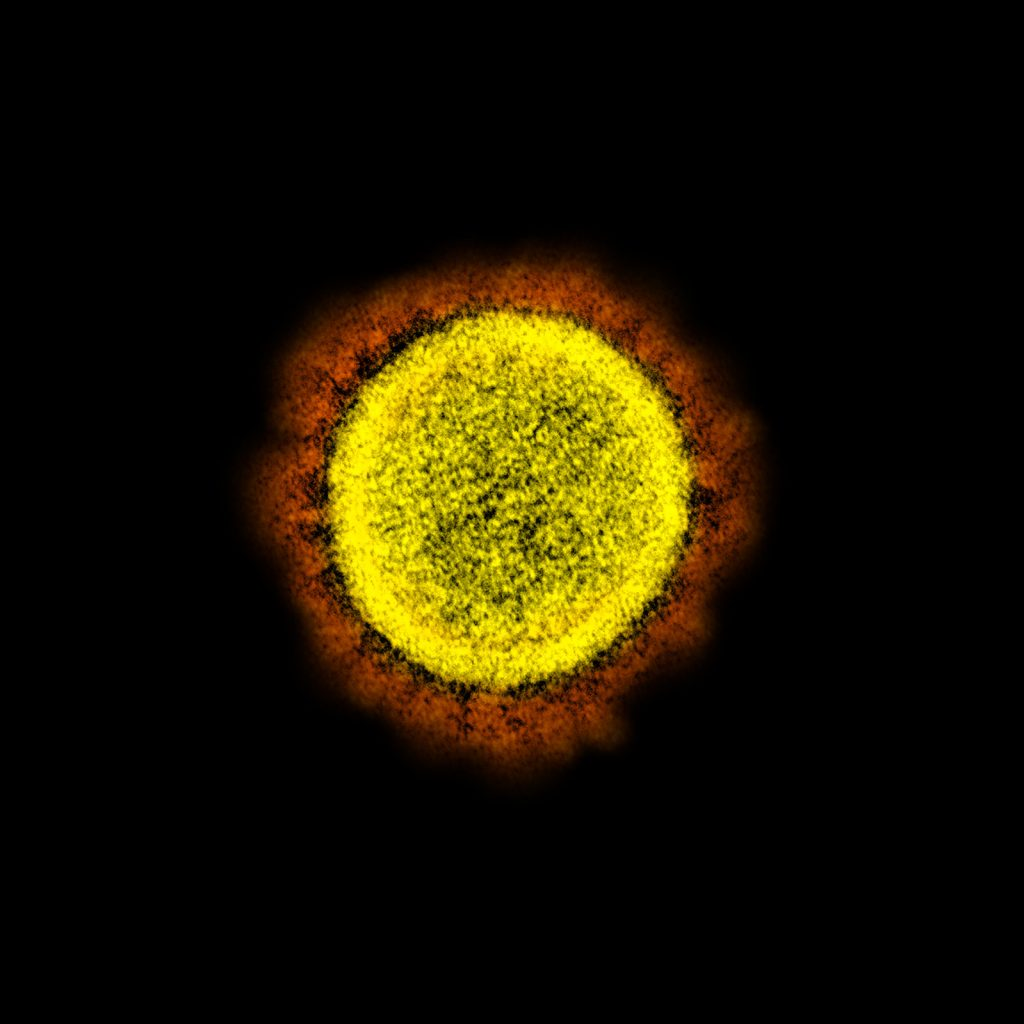
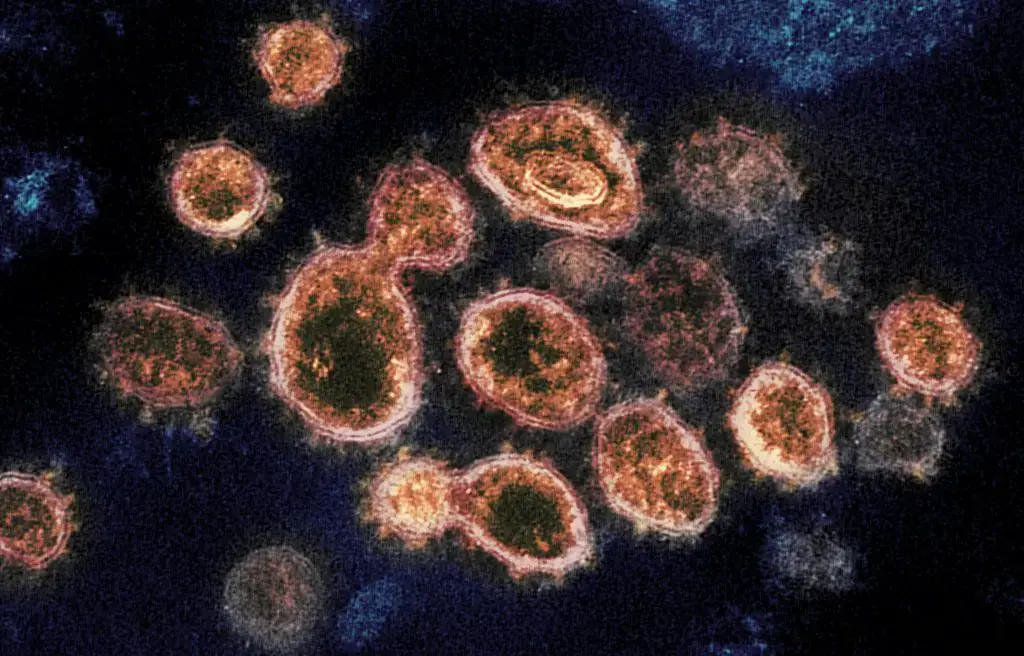
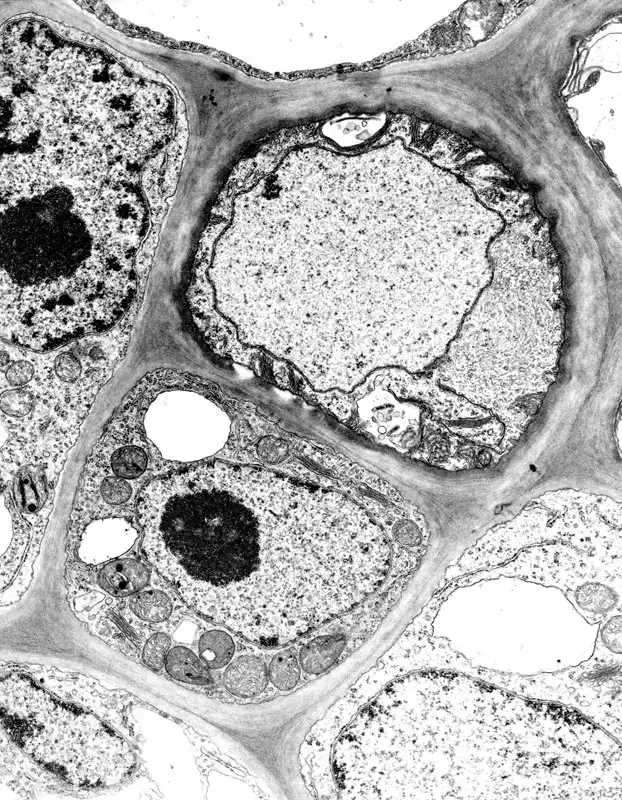
- https://www.news-medical.net/life-sciences/Sample-Preparation-in-TEM.aspx
- https://warwick.ac.uk/fac/sci/physics/current/postgraduate/regs/mpagswarwick/
- https://www.jeol.co.jp/en/science/em.html
- https://www.britannica.com/technology/electron-microscope
- https://www.ccber.ucsb.edu/collections-botanical-collections-plant-anatomy/transmission-electron-microscope
- https://www.news-medical.net/life-sciences/What-is-Transmission-Electron-Microscopy.aspx
- https://www.microscopemaster.com/transmission-electron-microscope.html
- https://en.wikipedia.org/wiki/Transmission_electron_microscopy
- https://www.slideshare.net/TakeenKhurshid/transmission-electron
- https://cfamm.ucr.edu/media/161/download?attachment=
- https://www.nanoscience.com/techniques/transmission-electron-microscopy/
- https://www.news-medical.net/life-sciences/Limitations-of-TEM.aspx
- https://pmc.ncbi.nlm.nih.gov/articles/PMC3907272/
- https://pmc.ncbi.nlm.nih.gov/articles/PMC4578338/
- https://www.labx.com/resources/what-is-a-limitation-of-using-electron-microscopes-to-view-specimens/40
- https://www.technologynetworks.com/analysis/articles/electron-microscopy-techniques-strengths-limitations-and-applications-353076