The regulation of heart rate is a critical component of cardiovascular physiology, influencing overall heart function and health. Heart rate (HR) is defined as the number of times the heart beats within a minute. Together with stroke volume (SV), which is the volume of blood ejected from each ventricle during a single contraction, heart rate plays a vital role in determining cardiac output (CO). Cardiac output, measured in liters per minute, represents the total volume of blood the heart pumps and can be calculated using the equation:
CO=SV×HR
Under typical resting conditions, an average heart rate hovers around 72 beats per minute, with a stroke volume of approximately 70 mL per beat. Therefore, the resting cardiac output can be quantified as:
CO=70 mL/beat×72 bpm≈5 L/min
This baseline can vary significantly among individuals, particularly between athletes and nonathletes. Athletes generally exhibit a lower resting heart rate and higher stroke volume due to physiological adaptations such as increased heart size and enhanced myocardial efficiency resulting from regular training. In contrast, nonathletes tend to present a higher resting heart rate and a correspondingly lower stroke volume.
During physical exertion, heart rate can escalate dramatically. For nonathletes, the heart rate may surge to approximately 190 beats per minute, accompanied by a stroke volume that can rise to about 115 mL per beat. The resulting cardiac output in such scenarios can reach approximately:
CO=115 mL/beat×190 bpm≈22 L/min
This output is substantially higher than at rest and highlights the remarkable capacity of the cardiovascular system to adapt to increased physiological demands. Athletes often achieve even greater increases in cardiac output, demonstrating their enhanced cardiovascular capabilities.
The mechanisms controlling heart rate and stroke volume are classified into two categories: intrinsic and extrinsic. Intrinsic mechanisms involve the heart’s natural pacemaker, the sinoatrial (SA) node, which initiates electrical impulses that dictate heart rate. In contrast, extrinsic mechanisms include hormonal influences and neural regulation. For example, the autonomic nervous system plays a crucial role in adjusting heart rate through sympathetic and parasympathetic pathways. The sympathetic nervous system typically increases heart rate and myocardial contractility during stress or exercise, while the parasympathetic system promotes a reduction in heart rate during rest.
Understanding the regulation of heart rate is essential for both medical professionals and students of physiology, as it underpins many aspects of cardiovascular health and disease management. Through careful study of these mechanisms, one can appreciate how various factors—both intrinsic and extrinsic—contribute to the heart’s performance, ultimately supporting the body’s metabolic needs under varying conditions.
Intrinsic Regulation of the Heart
Intrinsic regulation of the heart encompasses mechanisms that are inherent to the cardiac tissue itself. This regulation primarily involves the relationship between cardiac muscle contraction and the stretching of muscle fibers due to blood volume changes within the heart chambers. The following points elaborate on the key aspects of intrinsic regulation and its significance in cardiac output:
- Force of Contraction and Stretch: The contractile force generated by cardiac muscle is directly linked to the extent of stretch experienced by the muscle fibers. This stretch is determined by the volume of blood present in the ventricles at the end of diastole, known as end-diastolic volume.
- Preload Definition: The term preload refers to the degree to which the ventricular walls are stretched due to the volume of blood filling the heart. It plays a crucial role in regulating stroke volume.
- Venous Return: Venous return is the volume of blood returning to the heart from the systemic circulation. An increase in venous return elevates the end-diastolic volume, thereby enhancing the stretch of the cardiac muscle fibers.
- Response to Increased Preload: As venous return increases, the heart accommodates the additional volume, leading to greater stretching of the cardiac muscle fibers. In response to this increased preload, the heart contracts with greater force, resulting in an elevated stroke volume—the amount of blood ejected from the heart with each contraction.
- Relationship Between Preload and Cardiac Output: This interplay between preload and stroke volume is articulated by Starling’s law of the heart, which states that the stroke volume of the heart increases in response to an increase in the volume of blood filling the heart (preload). Consequently, when venous return rises, cardiac output also increases, facilitating the enhanced delivery of oxygen to tissues during increased physical activity.
- Influence of Exercise on Venous Return: During muscular activity, such as exercise, venous return is augmented due to factors such as skeletal muscle contractions, which assist in pushing blood back to the heart. This increase in venous return elevates preload, subsequently boosting stroke volume and cardiac output, thereby meeting the increased oxygen demands of exercising muscles.
- Afterload Explanation: In contrast to preload, afterload refers to the resistance the ventricles must overcome to eject blood during contraction. It is primarily influenced by arterial pressure; higher aortic pressures (as seen in hypertension) result in increased afterload.
- Implications of Increased Afterload: When afterload is elevated, as in the case of individuals with hypertension, the heart must exert greater effort to pump blood from the left ventricle into the aorta. This increased workload can lead to detrimental effects on heart function over time, potentially culminating in heart failure.
- Reduced Afterload Benefits: Conversely, a reduced afterload diminishes the workload required by the heart to eject blood. Individuals with lower blood pressure experience decreased afterload, thereby lessening their risk of developing heart failure compared to those with hypertension.
- Comparison of Preload and Afterload: It is essential to note that while both preload and afterload affect cardiac output, preload has a more pronounced influence. Significant increases in afterload are necessary before any marked decrease in the volume of blood pumped by a healthy heart occurs.
Extrinsic Regulation of the Heart
Extrinsic regulation refers to the mechanisms that influence heart function from outside the cardiac muscle itself. This includes both nervous and chemical regulation, which play critical roles in modulating heart rate and stroke volume to maintain homeostasis in response to various physiological changes.
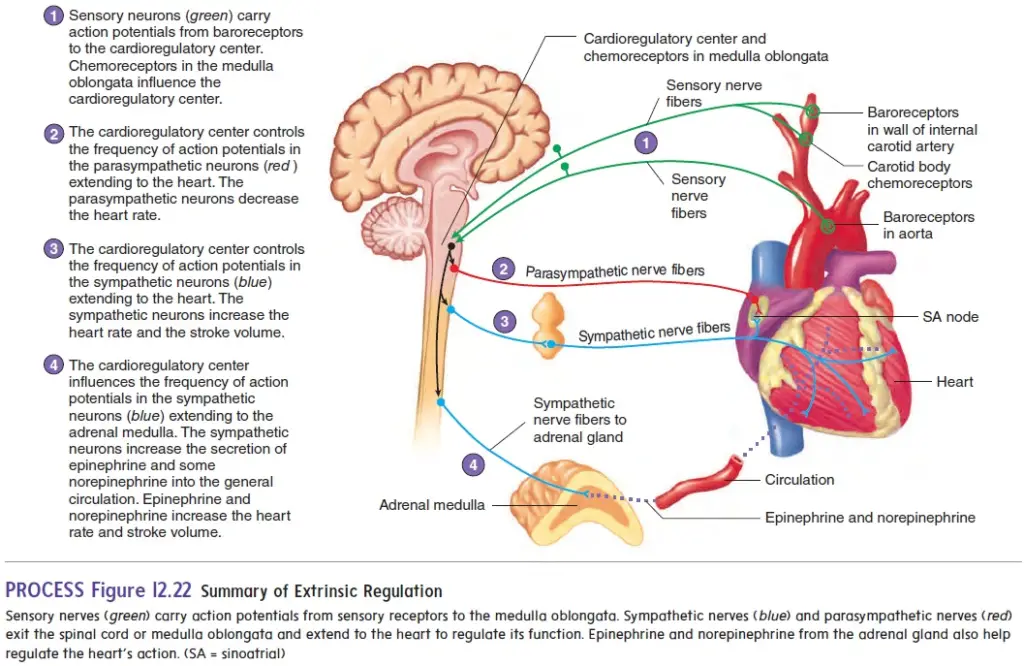
- Nervous Regulation: Baroreceptor Reflex
- The autonomic nervous system is responsible for the nervous influences on heart activity, consisting of sympathetic and parasympathetic nerve fibers that innervate the heart.
- Sympathetic Activation:
- Stimulation of sympathetic nerve fibers leads to an increase in both heart rate and stroke volume.
- Parasympathetic Activation:
- Stimulation of parasympathetic nerve fibers results in a decrease in heart rate.
- Baroreceptor Mechanism:
- Baroreceptors are specialized stretch receptors located in the aorta and the internal carotid arteries, monitoring blood pressure.
- Changes in blood pressure alter the stretch of these vessels, which in turn changes the frequency of action potentials generated by the baroreceptors.
- These action potentials are conveyed along nerve fibers to the medulla oblongata, where they are processed.
- Cardioregulatory Center:
- Within the medulla oblongata lies the cardioregulatory center, which receives and integrates signals from baroreceptors.
- This center modulates the frequency of action potentials in the sympathetic and parasympathetic fibers that innervate the heart.
- It also influences sympathetic stimulation of the adrenal gland, resulting in the release of epinephrine and norepinephrine, which further enhance heart rate and stroke volume.
- Response to Blood Pressure Changes:
- Increased Blood Pressure:
- Stimulation of baroreceptors leads to an increased frequency of action potentials to the medulla oblongata.
- This results in heightened parasympathetic and decreased sympathetic stimulation, leading to a reduction in heart rate and stroke volume, thus lowering blood pressure.
- Decreased Blood Pressure:
- Reduced stimulation of baroreceptors results in a lower frequency of action potentials sent to the medulla.
- This prompts increased sympathetic stimulation and decreased parasympathetic stimulation, causing an increase in heart rate and stroke volume, subsequently raising blood pressure.
- If the decrease is significant, sympathetic stimulation of the adrenal medulla increases, releasing more epinephrine and norepinephrine to further elevate heart rate and stroke volume.
- Increased Blood Pressure:
- Chemical Regulation: Chemoreceptor Reflex
- Hormonal Influence:
- Epinephrine and norepinephrine, released by the adrenal medulla during exercise or stress, bind to cardiac muscle receptors, enhancing heart rate and stroke volume.
- Emotional states such as excitement can lead to increased sympathetic stimulation, while depression may elevate parasympathetic activity, reducing cardiac output.
- Chemoreceptors:
- The medulla oblongata also contains chemoreceptors sensitive to changes in pH and CO2 levels.
- A decrease in pH, often due to elevated CO2 levels, triggers sympathetic stimulation of the heart.
- Ionic Concentration Effects:
- Variations in extracellular concentrations of potassium (K+), calcium (Ca2+), and sodium (Na+) can significantly influence cardiac function:
- An excess of K+ decreases heart rate and stroke volume, potentially leading to blockage of action potential conduction if concentrations rise excessively.
- Increased extracellular Ca2+ results in arrhythmic contractions, whereas reduced levels cause both heart rate and stroke volume to decline.
- Variations in extracellular concentrations of potassium (K+), calcium (Ca2+), and sodium (Na+) can significantly influence cardiac function:
- Hormonal Influence:
- Influence of Body Temperature:
- Body temperature can impact cardiac metabolism similar to other tissues.
- Elevated body temperature generally increases heart rate, which is commonly observed during fever.
- Conversely, reduced body temperature can slow heart rate, a technique sometimes used during heart surgery to lower metabolic rates and heart function temporarily.
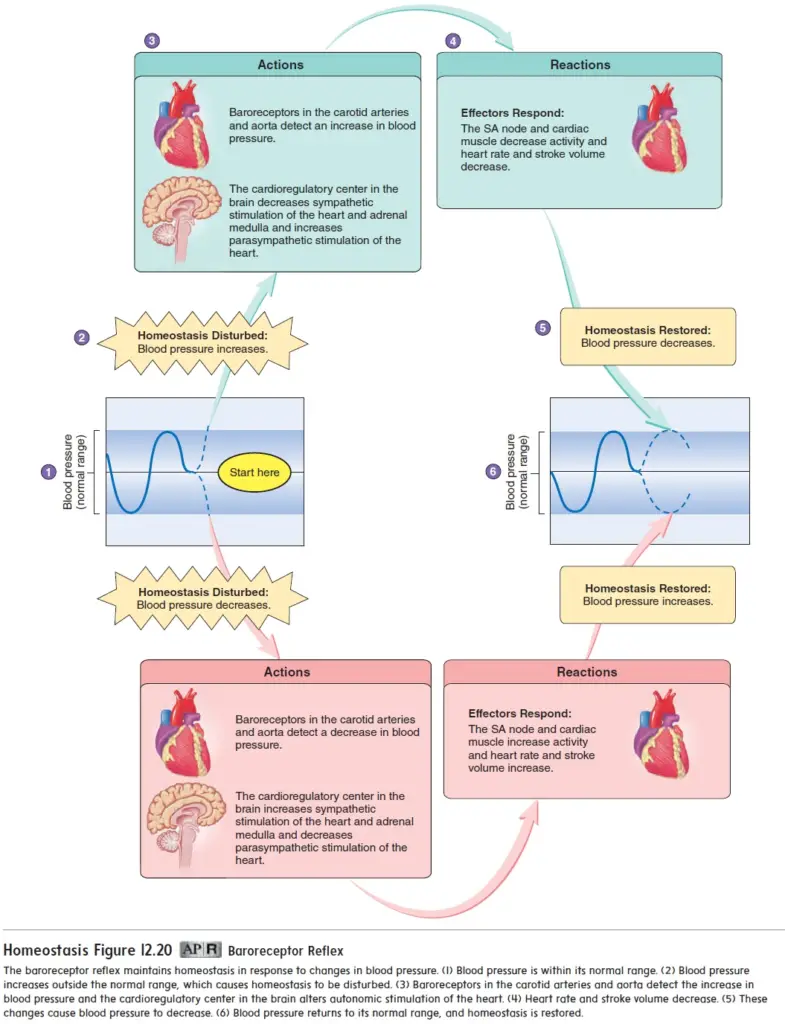
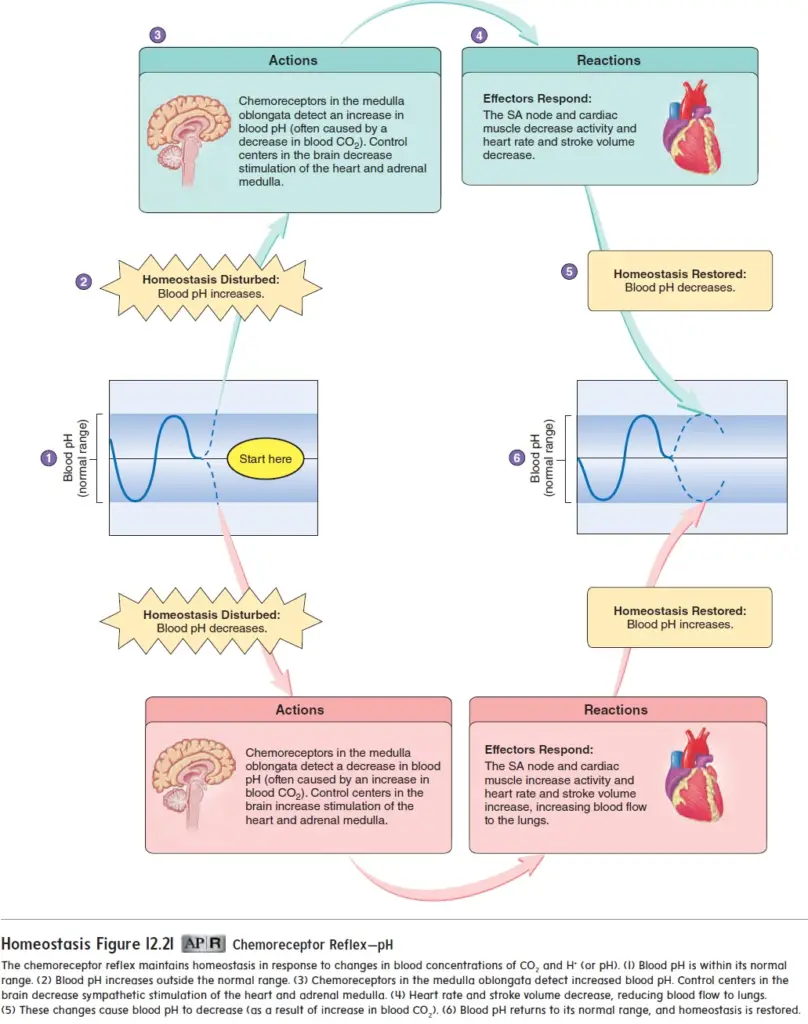
- https://www.brainkart.com/article/Regulation-of-Heart-Function_21884/
- https://www.hansrajcollege.ac.in/hCPanel/uploads/elearning/elearning_document/Nervous_and_Chemical_regulation_of_heart_rate.pdf
- https://www.embibe.com/exams/regulation-of-cardiac-activity/
- https://www.slideshare.net/slideshow/regulation-of-heart-rate/238329424