Sources of industrially significant microorganisms and methods for isolating, preserving, and maintaining them, as well as strain improvement, A production strain is the most crucial factor for the success of any fermentation enterprise. Utilizing a producing strain with the following four traits is extremely desirable:
- It must be a high-yielding kind.
- It must possess stable biochemical properties.
- It should not yield harmful byproducts.
- It must be simple to cultivate on a wide scale.
1. Screening Techniques
Screening refers to the detection and isolation of high-yielding species from natural source material having a heterogeneous microbial community, such as soil. There are numerous screening methods. Typically, screening regimens consist of both primary and secondary screening.
A. Primary Screening
- This includes the elementary assays required to detect and isolate novel microbial species exhibiting the desired characteristic.
- Primary screening programmes for antibiotic producers serve to eliminate worthless microorganisms based on relatively simple, fundamental criteria.
- Important selection criteria include the in vitro and possibly in vivo activity of antibiotics against a small number of the most important test organisms.
- In the case of other beneficial microorganisms, first screening is also necessary (e.g. micro-organisms capable of producing organic acids, amines, vitamins, etc.).
- Evaluation of the primary screening in industrial research programmes can be accomplished by mentioning the following specific examples of screening procedures:
a. The crowded plate technique
- The crowded plate technique is the most straightforward screening method for identifying and isolating antibiotic producers.
- It involves generating a series of dilutions of the soil or other source material for the microorganisms that produce antibiotics, followed by spreading the dilutions on nutrient agar plates.
- Considered are agar plates with 300 to 400 or more colonies per plate, as they are useful for locating colonies with antibiotic action.
- The presence of a zone of growth inhibition surrounding a colony indicates its ability to produce antibiotic action.
- Before creating stock cultures, such a colony is subcultured to a comparable medium and purified by streaking.
- It is required to do additional tests to confirm the antibiotic activity of a microorganism, as the zone of inhibition surrounding the colony may occasionally be attributable to other factors.
- The application of the crowded plate approach is limited, as it only provides information regarding the inhibitory activity of a colony against any undesired bacteria that may be present on the plate by coincidence.
- Therefore, the technique has been enhanced by the incorporation of a “test organism.” In this modified procedure, well-isolated colonies on agar plates (about 100 to 300 colonies per plate) are flooded with a suspension of the test organism.
- The plates are then treated to additional incubation to allow the test organism to develop.
- Antibiotic action is indicated by the establishment of inhibitory zones around certain colonies.
- To acquire an approximate approximation of the relative amounts of antibiotic(s) produced by various colonies, the diameter of the inhibition zones is measured in millimetres.
- Before further testing, the antibiotic-producing colonies must be identified and purified.
b. Auxanography
This technique is commonly used to detect microorganisms capable of producing extracellular growth factors (such as amino acids and vitamins). The two primary steps of the method are as follows:
i. Preparation of First Plate
- A 1.5 x 12-centimeter strip of filter paper is placed over the bottom of a petri dish such that both ends hang over the edge of the dish.
- A filter paper disc the size of a petri dish is placed over the paper strip at the dish’s base.
- On the paper disc in the plate, 45°C nutritional agar is poured and allowed to harden.
- Soil or other microbial source material is diluted to the extent that aliquots on plating will produce well-isolated colonies.
- Aliquots of a sufficiently diluted soil sample are plated.
ii. Preparation of Second Plate
- The test organism is introduced into a minimal medium devoid of the growth factor in question.
- The medium with seeds is poured onto the surface of a new Petri dish.
- The dish is left to set.
The agar in the first plate, as prepared in step (A), is carefully and aseptically removed with tweezers and a spatula and placed on the surface of the second plate, as prepared in steps (B) and (C), without inverting.
The growth factor(s) produced by colonies on the top layer of agar can diffuse into the layer containing the test organism. The zones of stimulated growth surrounding the colonies of the test organism indicate that they produce growth factors extracellularly. Productive colonies are propagated and tested further.
c. Enrichment culture technique
- Beijerinck, a soil microbiologist, devised this technique to separate the necessary microorganisms from the soil’s varied microbial population.
- Adjustments are made to either the medium or the incubation conditions to promote the development of the desired microorganisms.
- On the other hand, undesired microorganisms are killed or develop poorly since the newly formed environment does not support their growth.
- Today, this approach has become a key instrument in numerous screening programmes designed to isolate industrially significant strains.
Procedure of Enrichment culture technique
Typically, it includes the following steps:
- Incubated nutrient broth containing an unusual substrate (e.g. Cellulose powder) is inoculated with microbial source material (e.g. soil).
- A small amount of the inoculum from step one is plated on a solid medium with the same composition. After incubation, colonies are well isolated.
- Suspect colonies from the second step plate are sub-cultured on fresh media and subjected to additional testing.
It is possible to provide an example of screening enzyme-producing microorganisms. It is possible to discover alkaline protease-producing microorganisms in the soil as follows:
- The soil undergoes serial dilution.
- All soil dilutions are heated for 10 minutes at 80°C. This treatment eliminates vegetative cells but has no effect on spores.
- Heat-treated samples are plated by spreading diluted samples (typically 0.1 ml) onto the surface of nutrient agar containing casein at pH 10-12.
- The sub-cultured colonies are surrounded by a clean zone.
d. Use of an indicator dye
- The pH indicating dyes may be used in various screening procedures for discovering bacteria capable of creating organic acids or amines, as the pH of the dye affects the dye’s colour.
- These colours (e.g., neutral red, bromothymol blue, etc.) are added to nutrient agar media with inadequate buffering.
- Depending on the nature of a reaction, the change in colour of a dye near a colony indicates the ability of colonial cells to create either organic acid(s) or amine(s).
- Such colonies are subcultured to produce stock cultures. However, additional testing is required because inorganic acids or bases are also possible metabolic products of microbial development. In other words, these strategies do not guarantee success.
- This differential approach can also be used to determine whether or not microorganisms are capable of specific microbial transformations, such as —7 Oestrogen production by bioconversion.
B. Secondary Screening
Primary screening merely enables the detection and isolation of microorganisms with potentially interesting industrial applications; secondary screening is required for any systematic screening programme designed to isolate industrially useful microorganisms. In addition, primary screening does not provide a great deal of information necessary for establishing a new fermentation process. Secondary screening aids in the identification of truly beneficial microorganisms during fermentation processes. This can be accomplished by carefully considering the following secondary screening considerations:
- It is quite beneficial for separating microorganisms with actual commercial value from the numerous isolates obtained during primary screening. Simultaneously, microorganisms of little utility in the fermentation process are removed. It is recommended to eliminate inferior cultures as quickly as possible, as such research are laborious and costly.
- It gives information regarding whether or not a microorganism’s product is novel. This can be performed by the use of paper, thin layer, or other chromatographic techniques. The considered substance is compared to previously known compounds.
- It provides a sense of the economic value of a fermentation process employing a freshly found culture. Regarding the economic status picture, it is possible to do a comparative analysis of this process with processes that are already known.
- It provides information regarding the probable product yields of certain iso-lates. Consequently, this is beneficial for selecting effective cultures for fermentation operations.
- It determines the optimal circumstances for the growth or accumulation of a given culture’s output.
- It gives information regarding the effect of various medium components. This information is useful for building a media that may be economically appealing.
- It identifies widespread genomic instability in microbial cultures. This type of information is crucial, as microorganisms prone to mutation or change may lose their ability to accumulate fermentation products to their optimum capacity.
- It provides information regarding the quantity of products produced during a single fermentation. Additional major or minor products are of exceptional value, as their recovery and sale can significantly enhance the economic status of the primary fermentation.
- It is made available information regarding the product’s solubility in various organic solvents. This information is useful for the recovery and purification of the product.
- During secondary screening, the chemical, physical, and biological properties of a product are also determined. In addition, it indicates whether a product produced in the culture broth exists in multiple chemical forms.
- This determines whether a culture is homofermentative or heterofermentative.
- The structure of the product has been determined. The structure of the product may be simple, complex, or even macromolecular.
- If certain types of products (such as antibiotics) are to be used for therapeutic purposes, their toxicity for animals, plants, and humans must be determined.
- It reveals whether microorganisms are able to undergo chemical transformations or even destroy their own fermentation products. Microorganisms that produce the adaptive enzyme decarboxylase, for instance, can remove carbon dioxide from an amino acid, leaving behind an organic amine.
- It provides information regarding the chemical stability of the fermented product.
Thus, secondary screening provides answers to numerous concerns that emerge during the final sorting of microorganisms with industrial applications. This is achieved by conducting research on agar plates, in flasks or tiny bioreactors holding liquid media, or by employing a mix of these methods.
For an understanding of the sequence of events throughout a screening programme, a specific example of antibiotic-producing Streptomyces species may be used. In a primary screening programme, those streptomycetes able to generate antibiotics are discovered and separated. Antimicrobial streptomycetes are subjected to a preliminary secondary screening in which their inhibitory spectra are obtained. A *giant-colony* approach is utilised to accomplish this. Each streptomycal isolate is streaked across the centres of the nutritive agar plates in a thin band. The plates are then incubated until streptomycete growth occurs.
Now, the test organisms are streaked from the margins of the plates to the streptomycete growth, but not touching it. The plates are incubated once more. At the conclusion of incubation, growth inhibitory zones are determined in millimetres for each test organism. Thus, the’microbial inhibition spectrum’ investigation greatly facilitates the elimination of ineffective cultures. Ultimately, streptomycete isolates with intriguing microbial inhibition spectra require additional testing. With the possibility that streptomycetes produce antibiotics with poor solubility in water, the initial secondary screening is conducted in a manner beyond the scope of this book.
Further screening is conducted using liquid media in flasks, as these experiments yield more information than those conducted on agar media. In addition, it is recommended to use accurate assay procedures (e.g. paper disc-agar diffusion test) to precisely assess the quantities of an antibiotic present in culture fluid samples. Thus, each streptomycete isolate is investigated in Erlenmeyer flasks equipped with baffles utilising a variety of liquid media.
These cultures of streptomycetes are injected into sterile liquid media. The flasks containing the seeds are then incubated at a steady temperature. Typically, these cultures are incubated at temperatures close to room temperature.
In addition, these flasks are aerated by placing them on a mechanical shaker, as the growth of streptomycetes and the production of antibiotics are enhanced in aerated flasks as opposed to stationary ones. Regular samples are collected under aseptic circumstances and analysed in a laboratory for quality control. Important tests include the following:
- checking for contamination
- checking of pH
- estimation of critical nutrients
- assaying of the antibiotic
- other determinations, if necessary
The results of the aforementioned tests indicate which medium is optimal for antibiotic production and at what stage in the growth of the culture on the various media the antibiotic yields are greatest. After completing all required routine tests in the screening for a streptomycete that is truly useful for a fermentation process, additional determinations are made. The following are:
- Evaluation of fermentation media from which the greatest antibiotic yields can be achieved.
- Determine whether or not the antibiotic is new.
- It is determined how much antibiotics have accumulated in the culture broth.
- Effect of various bioparameters on streptomycete culture growth, fermentation process, and antibiotic accumulation.
- S. Antibiotic solubility in a variety of organic solvents. Additionally, it must be established if antibiotics are absorbed by adsorbent materials (e.g. ion-exchange resins or activated carbon). This understanding is crucial for recovering and purifying an antibiotic from the fermenting broth.
- On mice or other laboratory animals, toxicity testing are undertaken. Antibiotics are also evaluated for potential harmful effects on humans, animals, and plants.
- Cultures of streptomycetes are characterised and classified by species.
- An unique streptomycete culture is the focus of more research. For instance, mutation and other genetic studies are conducted to improve strains.
In conclusion, tests are devised and executed so as to produce production streptomycete strains at the lowest possible cost. Similar screening and analytical procedures could be used for isolating microbial isolates essential for the manufacture of various industrial chemical compounds.
2. Strain Development
- It is of the utmost importance that the industrial fermentation process become increasingly economical. This depends greatly on the efficacy of the production strain utilised in the fermentation process.
- Therefore, a person desiring to begin a fermentation industry or compete with other industries must acquire an effective strain.
- Clearly, the utilisation of a high-yielding strain is the most crucial aspect of any fermentation procedure. Typically, newly isolated strains obtained through screening techniques are inefficient for industrial fermentation processes.
- Regarding the yield of a particularly desirable compound, therefore, such strains require improvement. This is accomplished through the use of physical or chemical methods to produce mutant fermentation strains. These mutants can be categorised into two major groups:
- auxotrophic mutants
- mutants resistant to analogues
- Typically, microorganisms possess regulatory systems that regulate the amount of metabolites generated. Therefore, microorganisms cannot produce an excess of the metabolites that would exceed the needs of the cells.
- Clearly, inhibition of these regulatory mechanisms is required to generate strains that produce larger yields of the desired metabolites.
- Strain improvement may employ microbial cultures with multivalent processes, coordinated repression, or feed-back inhibition.
- Then, mutants that have lost the capacity to produce one of the end products capable of feed-back inhibition or repression are sought.
- Consider a case in which three end products (E.P., E.P., and E.P.) are generated from an intermediary molecule via a branched biosynthetic pathway (A).
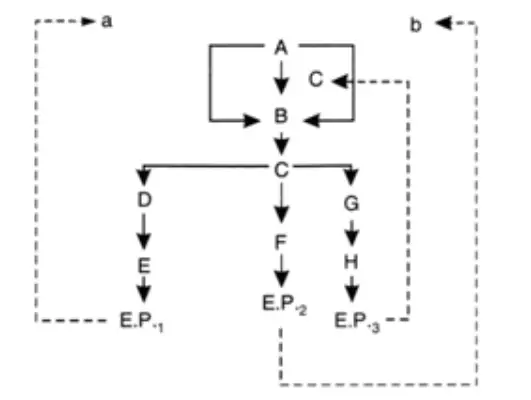
- There are two primary regulating processes, which are distinct from one another. There may be three different isoenzymes (a, b, and c) capable of catalysing the first pathway reaction (A to B).
- And each is capable of being inhibited or repressed by one of the three final products. With a multivalent or concerted regulatory system, repression is only evident when all three end products are present.
- It should be emphasised that there is just one enzyme for the A-to-B conversion. Additionally, it should be emphasised that there is occasionally a cumulative effect of the three end products depicted schematically.
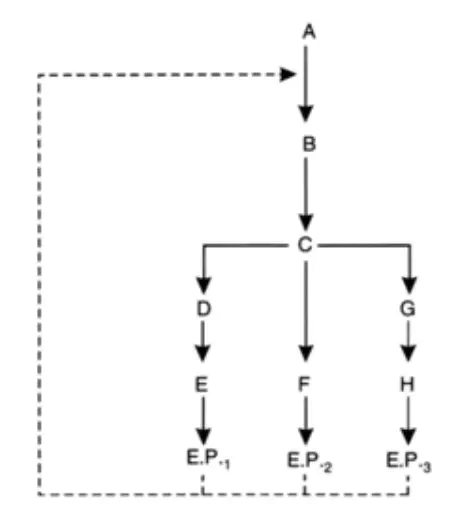
Different types of industrially important mutants have been summarized as under:
- A mutant strain of Corynebacterium glutamicum can excrete around 60 g of lysine per litre in a glucose- and mineral-based medium. This strain requires homoserine. The wild strain of this bacteria, on the other hand, does not require homoserine and does not excrete lysine. In a wild strain, the initial few reaction steps of the production of lysine and threonine have a similar metabolic route. A combination of lysine and threonine that regulates the activity of aspartate kinase inhibits this pathway via feed-back. However, a mutant strain that requires homoserine can no longer produce threonine. In addition, feed-back inhibition no longer takes place, and lysine accumulates in the medium. The optimal medium for lysine synthesis contains 400 ug of homoserine per millilitre and a high concentration of biotin (20 ug/ml). Methionine can be shown to augment threonine-induced inhibition. Methionine interacts with a regulatory region on aspartate kinase in a competitive manner. The ratio of threonine to methionine is also significant.
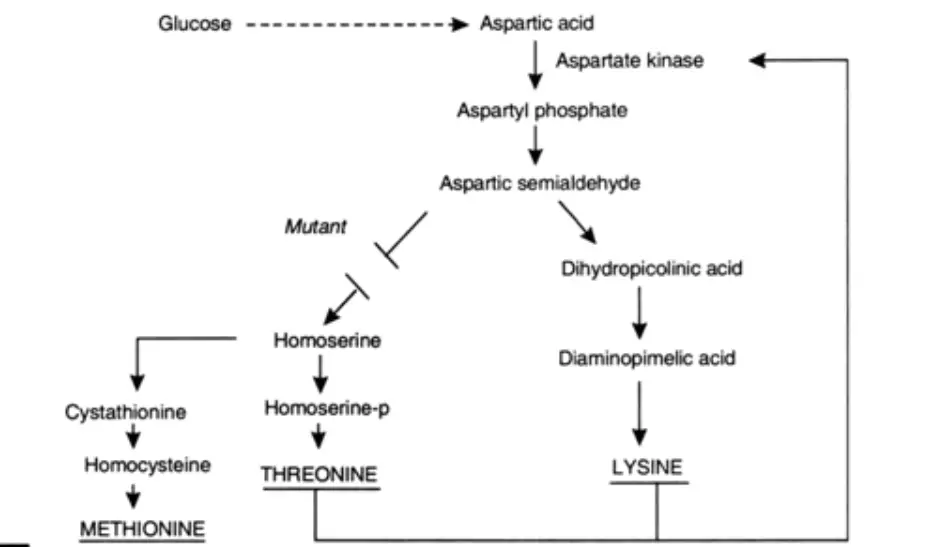
- Notably, the biosynthetic pathway to lysine is identical in wild strains of Escherichia coli, but its regulation mechanism is distinct. In this instance, there are three aspartate kinases, each of which is independently regulated by lysine, methionine, or threonine. This regulatory system is depicted in Figure, where E.P is lysine. Lysine also inhibits dihydropicolinate synthetase via feedback inhibition.
- Mutant strains that lack an enzyme in a biosynthetic route are also beneficial, as they can be used to produce an intermediate metabolite of that pathway. A mutant strain of Corynebacterium glutamicum may serve as an illustration of this. The biosynthesis of the amino acid arginine is depicted in Figure as a biosynthetic pathway. Now, a mutant strain that lacks the ornithine-acting enzyme will excrete ornithine as long as just enough arginine is provided for growth, without enough to cause feedback inhibition. Ornithine is produced optimally in a medium containing 200ng of arginine per millilitre and Sug of biotin per liter. In addition, this medium should contain an abundance of glucose and ammonium salts.
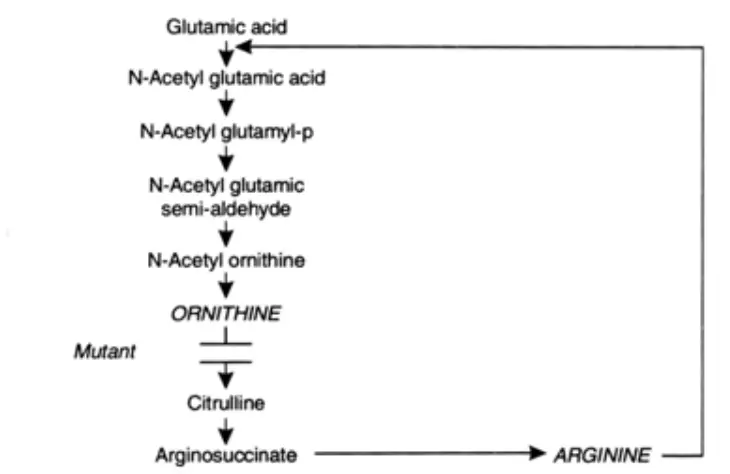
- Some mutant strains contain enzymes that provide resistance to feedback control. Regarding the regulatory mechanism of feed back inhibition, the interaction between an enzyme’s end product and regulatory site alters the enzyme’s structure. Consequently, the enzyme is rendered ineffective. A mutant strain containing an enzyme with an altered regulatory site is producible. This modified regulatory site is incapable of interacting with the inhibitor. Consequently, feed back inhibition does not occur.
- It is also possible to employ an analogue in the selection of economically significant strains. An analogue can interact with the regulatory site underlying feedback inhibition. Such an analogue typically has a toxic effect. Additionally, this toxicity eradicates all sensitive mutant cells within a population. -amino B-hydroxyvaleric acid, for instance, is the analogue of threonine. Using this antimetabolite, the following two stages of mutant strain selection are possible:
1. During the preparation of a nutrient agar medium poured into a sterile petri dish, threonine, an analogue of an amino acid, is added. The medium is then allowed to solidify at an angle, as illustrated below:
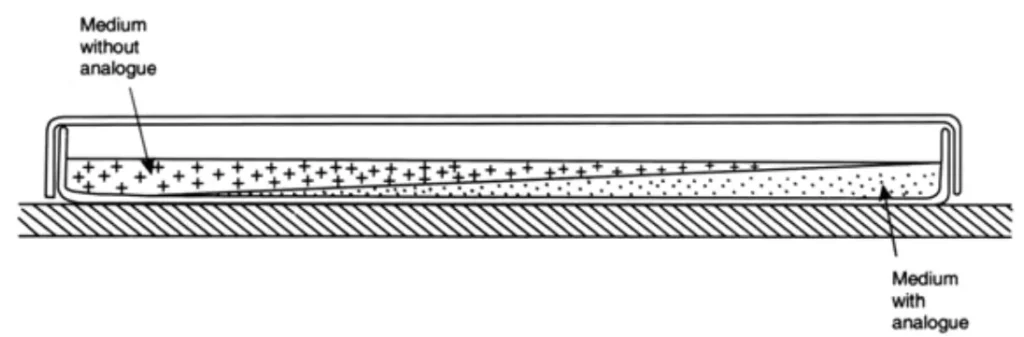
- After the wedge has hardened, a second layer of the same medium, without analogue, is poured on top and allowed to harden with the plate level as follows:
- After a period of time, an analogue diffuses into the upper layer of the medium. This results in the formation of a concentration gradient at the surface.
- Now, a culture that has been treated with a mutagen is spread across this medium’s surface.
- Then, any mutants with resistance to high concentrations are selected.
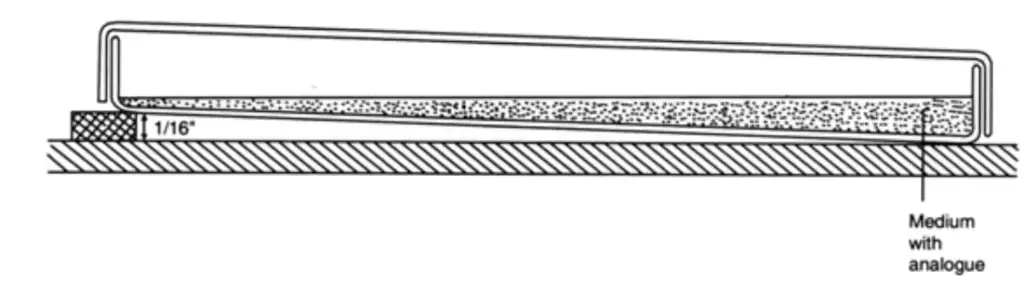
2. The final step is to hunt for resistant mutants capable of generating threonine.
This can be achieved by inoculating point cultures of mutants onto an agar medium that has been seeded with a threonine-dependent culture. It is possible for seeded cultures (cultures requiring threonine) to grow around each colony of threonine-excreting mutant strain. The quantity of threonine generated by mutants determines the diameter of the zone of seeded culture growth. Thus, it is possible to obtain analogue-resistant mutant strains that produce greater amounts of threonine. Using the method described above, a mutant strain of Brevibacterium flavum capable of excreting up to 12.6 g of threonine per litre is created.
- Mutant strains may undergo reversion on occasion, as mutations are not always stable. As a result, revertants may arise in a mutant strain’s microbial population. The revertant strain possesses a different enzyme than that which was lost as a result of the earlier mutation. Additionally, the enzyme is resistant to feedback inhibition. The threonine deaminase of a revertant strain of the bacterial species Hydrogenomonas is an illustration of this. The situation is depicted as follows:
- The wild strain lacked a need for isoleucine. Moreover, this amino acid was not produced.
- The auxotrophic mutant’s growth and reproduction required isoleucine.
- The revertant strain did not require isoleucine. In addition, this amino acid was also created.
- In the fermentation industry, constitutive mutants are important because they can be used to increase the yield of specific enzymes. In the absence of inducing substrates or other substrates that provide resistance to catabolite repression, these mutants produce specific enzymes.
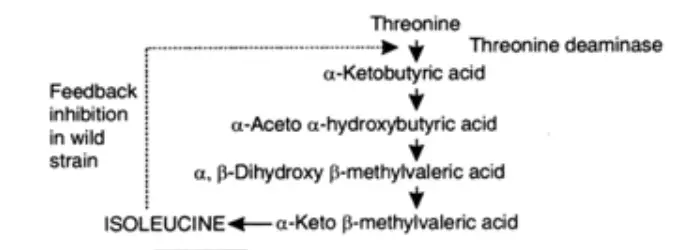
There are numerous techniques for selecting these mutants. Some simple methods have been briefly discussed here:
- On a medium containing a carbon source with the following two qualities, microbial cells are grown.
- It should not serve as an inducer substrate for a specific enzyme.
- It should function as a substrate for the same enzyme. For instance, phenyl B-galactoside can be used to identify constitutive mutants for B-galactosidase excretion. By limiting the concentration of an inducing substrate (Lactose) during the continuous culturing of Escherichia coli, a constitutive mutant was created. This mutant strain generates 25% of its total protein as the enzyme B-galactosidase.
- In a cyclical way, the microbial culture is alternately grown with and without an inducing substrate. For instance, the microbial culture may be cultivated cyclically in the presence of glucose and then lactose. After a given number of cycles, the fraction of constitutive mutants in the bacterial population will increase.
- In the selection of constitutive mutants, inhibitors of the inducer in the medium may also be utilised. For instance, 2-nitrophenyl B-fucoside is a lactose inhibitor and can be used to select mutants with constitutive B-galactosidase production. Therefore, Escherichia coli mutants generate B-galactosidase without induction if the medium contains lactose as the sole carbon source.
- It is feasible to isolate constitutive mutants that provide tolerance to toxins. Mutants are constitutive for an enzyme that eliminates environmental toxins. When a culture of the photosynthetic bacterium Rhodopseudomonas spheroides is repeatedly exposed to 0.1 M H,O, 25% of the surviving mutants are constitutive mutants for catalase production.
- Toxic antimetabolites can be utilised to select constitutive mutants with the capacity to produce a higher yield of enzymes involved in the biosynthesis of the metabolite in question. Mutants of Lactobacillus case resistant to dichloroamethopterin, for instance, produce eighty times more thymidylate synthetase than the parent culture. In addition to the various techniques for inducing high-yielding mutant strains, there is an additional technique for obtaining high-yielding strains. In this instance, the genetic makeup of the microbial cells is altered.
This is achieved by transferring all or a portion of the DNA from the donor culture to the recipient culture. Again, there are numerous methods for transferring genetic material. They are listed as follows:
- transformation
- transduction
- lysogeny
- conjugation
- parasexuality
3. Preservation of Microorganisms
Every microbiologist in practise has felt the need to preserve the viability of the microorganisms with which they work. In addition, all cultural characteristics of a culture must be preserved as they existed at the time of preservation. The nature of the work being performed will determine whether the preservation requirement is very brief (e.g., a few days) or permanent. If a culture is to be deposited in one of the service culture collections with the goal of preserving something of scientific value “forever,” long-term preservation is required. Numerous techniques for preserving microorganisms have been developed. It should be noted that there are numerous types of microorganisms. Therefore, there are two factors to consider when selecting a preservation method for a given culture. The following are:
- the period of preservation desired.
- the nature of a culture to be preserved.
Any biologist working with living organisms must, however, at least temporarily maintain his own cultures during the course of his research and preserve them until they are ready to be deposited in one of these major collections. This depositing of important strains is highly desirable because in the past, many organisms that were the subject of intensive research were discarded at the conclusion of the work or upon the biologist’s death. Thus, a significant amount of valuable material has been lost. There are three fundamental objectives for maintaining and preserving microorganisms. The following are:
- To keep cultures alive.
- Uncontaminated
- As physically and physiologically healthy as possible, retaining their original properties until they are deposited in major collections. For extremely long-term preservation involving stocks of the strains (rather than single specimens of each strain) and where withdrawals from stocks are made on a regular basis, a fourth objective is to have adequate stocks and systems for replenishing stocks as needed. Obviously, this fourth objective is the responsibility of service culture collections, but the other three are the responsibility of any maintenance and preservation program.
The operation of the collection and the maintenance procedures are intended to reduce the following risks to which the cultures are exposed:
- Transfer selection can be repeated on either a mutant strain or a purely vegetative, non-sporulating type. Therefore, the transfer should be performed as much as feasible by a specialist with an eye for the wild strain. However, the risk decreases as the number of transfers decreases.
- Occasionally, some strains tend to become attenuated under artificial culture conditions.
- Others degrade into damp, slimy, decomposed mycelium or spores. Simmons (1963) suggests that this may be the result of viral infections, and there is substantial evidence to support his claim.
- Only adaptable strains survive the maintenance processes to which the microorganism (e.g., the fungus) is subjected. These may possess a very typical quality.
- Cultures are susceptible to contamination, mite infection, and unfavourable circumstances of temperature, light, and humidity, etc., which are responsible for their contamination. The latter may result from equipment failure or an insufficient understanding of the body.
- There must be adequate documentation of the strains. In a culture collection with a long history, strains may survive several generations of microbiologists; therefore, a clear description of the cultural characteristics supported by dried cultures should be provided at the time of depositing them in order to preserve them in their original state.
A. Serial Subculture
- This is the most popular and straightforward approach for sustaining microbial cultures. Microbes are cultured on agar slants and moved to fresh media before depleting nutrients or drying out.
- A notable exception is aerobic Streptomyces spp., for which drying out of the medium has been found to be effective, providing the initial growth produced aerial hyphae.
- The drying of the medium appeared to promote sporulation, and the preserved specimen became a dried out strand of agar coated with viable spores that remained viable at ambient temperature for several years.
- For some microbial cultures, no other methods have been discovered to be adequate, however for the vast majority of species, alternative techniques are available. There are numerous considerations to keep in mind when selecting an appropriate media.
- Solid media should be chosen over liquid media so that the growth of a contamination may be monitored more easily. Nevertheless, bacteriophages are frequently maintained successfully as suspensions in liquid mediums.
- Also, anaerobes, particularly Clostridium spp., are routinely maintained in a liquid medium (for instance, Robertson’s cooked beef). Some maintenance specialists favour stab cultures.
- However, there do not appear to be any published data indicating that these are superior to slope cultures. Obviously, if the microorganism is oxygen-sensitive, a stab culture would be recommended as an added precaution during bench handling.
- While a rich media may provide optimal early growth for heterotrophs, it runs the danger of collecting hazardous metabolic byproducts.
- Therefore, the best media for growth may not necessarily be the greatest for microorganism maintenance and preservation. In addition to a proper substrate, additional variables influence the growth of cultures intended for storage. These include light intensity, temperature, humidity, standard growth conditions, transfer method, culture vessel, and storage.
- The appropriate duration for subculture may range from one week to several years. Under normal circumstances, cultures must be regrown fairly frequently.
- With a large collection, this requires considerable effort. In addition, every time a culture is handled, the aforementioned dangers exist as a possibility.
- In order to reduce the frequency of handling cultures, it is desirable to increase the time between subculturing. There are numerous ways to achieve this.
B. Preservation by overlaying cultures with mineral oil
This preservation method is an adaptation of serial subculture technique. It was first widely employed by Buell and Weston. Of 2000 fungus strains maintained under oil for 10 years at the C.M.I., only 45 were lost.
This method is inexpensive and simple because it does not require any specialised knowledge or equipment, such as a centrifuge, desiccator, or vacuum pump. The steps involved in this method are:
- First, an agar slant contained within a screw-cap tube is inoculated with a specific culture.
- Inoculated agar slants are incubated until healthy growth appears.
- A healthy agar slant culture (from the previous step) is covered with sterile mineral oil to a depth of approximately 1 cm, above the agar slant. When using a short slant of agar, less oil is required.
- The culture from step (in) can then be stored at room temperature. However, viability is improved when stored at lower temperatures (such as 15°C).
The used oil should be of high grade. British pharmacopoeia therapeutic paraffin oil with a specific gravity between 0.865 and 0.885 is acceptable. At the C.M.1, oil is sterilised in McCartney bottles for 15 minutes at 151b/in? Simmons (1963) also emphasises the importance of high-quality oil, early sterility, and dryness.
The oil covering stops the culture from drying out. The oil permits slow gas diffusion, thus growth proceeds at a slower rate. This may induce alteration as a result of adaptation to oil increase. Some fungus isolates appear stable, and C.M.1 survivals of more than 20 years have been recorded. Others evolve swiftly, resulting in a standard culture within a few months (e.g. Fusarium species).
The oil depth of 1 centimetres is crucial (Fennel, 1960), as the transfer of oxygen through layers of mineral oil beyond 1 centimetres becomes less favourable. If less oil is used, mycelium may become exposed, allowing the cultures to dry (Dade, 1960; Fennel, 1960). If McCartney bottles are used, the rubber liners should be removed from the metal caps because oil tends to degrade rubber, which can be hazardous to cultures.
Advantages
This method has the following advantages:
- Practically every tested bacterial species or strain survives longer in oil than in control tubes devoid of oil. Certain bacterial species have been successfully preserved for 15 to 20 years.
- When desired, transplants can be prepared without compromising the viability of the stock cultures.
- The method is especially advantageous when working with unstable variants in which sporadic transfers to fresh media or growth in mass cultures results in alterations to the strains’ developmental stages.
- This appears to be an ideal storage method for a busy laboratory with limited funds and a relatively small collection. Numerous employees have reported using the oiled fungus culture maintenance method.
C. Lyophilization or freeze-drying
The most effective method for the long-term preservation of microorganisms is lyophilization. It is used universally to preserve bacteria, viruses, fungi, sera, toxins, enzymes, and other biological substances. Although it is a convenient method for preserving a large number of cultures, it is not the ideal method for storing yeasts with completely unaltered properties. Raper and Alexander were the first to apply lyophilization on a large scale to microfungi in 1942. In 1945, they successfully processed cultures at the Northern Regional Research Laboratory in Peoria and presented their findings.
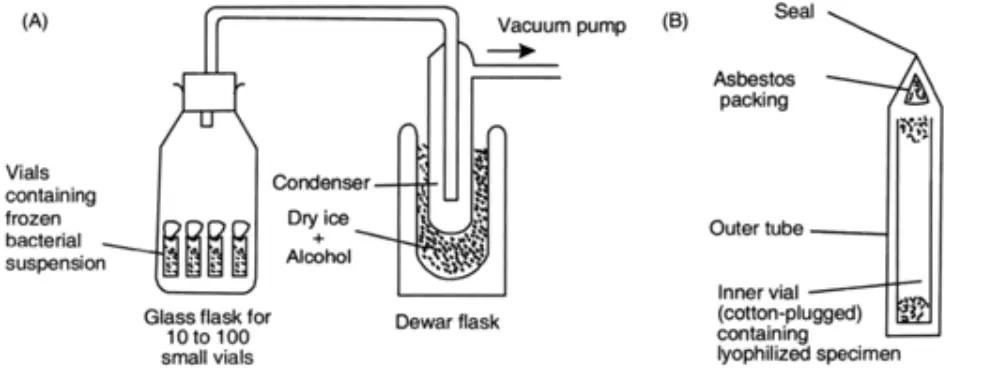
(A) Small cotton-plugged vials holding frozen suspensions of microorganisms are placed in a condenser-connected glass flask. The condenser is coupled to a high-vacuum pump, and this setup causes the cultures to dry out.
(B) Once the cultures have been desiccated as described in (A), the vials are removed, placed separately in a bigger tube, covered with asbestos packing, and vacuum-sealed.
****
Steps of Lyophilization or freeze-drying
Perhaps the most prevalent kind of suspended metabolism is lyophilization. It involves drying cultures or a suspension of spores from a frozen state under reduced pressure. There are numerous types of devices available for this purpose. This can be achieved in numerous ways. These are the primary steps involved in this method:
- In an appropriate protective medium, a cell or spore suspension is created (at the Commonwealth Mycological Institute). 10% skim milk and 5% inositol in water that has been purified are deemed appropriate).
- The suspension from I is dispensed in minute amounts into glass ampoules using a sterile procedure.
- The ampoules are attached to a high vacuum system that typically includes a desiccant (such as phosphorus pentoxide, silica gel, or a freezing trap) and immersed in a freezing combination of dry ice and alcohol (-78°C).
- After activating the vacuum pump and evacuating the ampoules until drying is complete, the ampoules can be sealed.
Methodological specifics differ from laboratory to laboratory. For the processing of a few ampoules, relatively basic equipment can be created, although complicated machinery are available for larger-scale operations. At the C.M.I, a two-stage centrifugal freeze drier is utilised. In this case, cooling is achieved through evaporation, and no freezing mixture is necessary. Instead of vacuum sealing, the little vials can be filled with sterile dry nitrogen once they have been dried.
The methods of revitalization vary from laboratory to laboratory. In the case of fungi, dry pellets can be dissolved in a suitable solvent before being streaked over agar. A amount of sterile water equal to the initial volume of the spore suspension is added to the ampoule at room temperature at the C.M.l. The ampoule is then left for 20 to 30 minutes to allow the water to be progressively absorbed before streaking. This delay appears to result in more satisfying civilizations. The level of vitality is evaluated visually or by counting spores.
Factors affecting
- Among the variables that influence the survivability of freeze-dried cultures are: the chemical composition of the protective (suspending) media;
- Addition of specific compounds to the culture suspension prior to freeze-drying to protect the culture from the toxicity of oxygen and moisture when stored in unsealed ampoules; Sealing the ampoules after freeze-drying to prevent access to oxygen and moisture.
- Insufficient elimination of oxygen and moisture on the freeze-dried culture’s survival rate.
- Temperature for storing freeze-dried and sealed cultures.
Advantages Lyophilization
This approach has the following benefits:
- As the ampoules are sealed, there is no possibility of mite contamination or infection.
- The prepared ampoules are simply stored, are not easily fractured, and maintain viability for many years for the majority of species (more than 20 years in case of many bacterial species).
- There are fewer opportunities for cultural features to undergo transformation (i.e. they remain unchanged during storage period).
- Due to the small size of glass ampoules, it is possible to store hundreds of lyophilized cultures in a small space. In addition, the tiny size of the ampoules makes them suitable for mailing.
- Lyophilization reduces the number of required transfers.
D. Storage at very low temperatures or Nitrogen Storage
This process is also known as cryogenic storage. It is comparable to lyophilization, an effective approach for the long-term storage of microorganisms. Many specimens that cannot be lyophilized have also been successfully preserved with this technique. Suspended metabolism is responsible for microorganism maintenance.
Steps for Storage at very low temperatures or Nitrogen Storage
- In an appropriate suspending media (10% glycerol is employed at the Commonwealth Mycological Institute), the culture is suspended as a cell or spore suspension, as finely broken-up particles of mycelium, or as a piece of fungus mycelium. As dimethyl sulfoxide is less comfortable to handle than glycerol, glycerol should be used routinely as a suspension medium and dimethyl sulfoxide should only be utilised when revivals are weak.
- Ampoules are filled with the suspension created in step I (These must be resistant to cold-shock). At the A..C.C., Wheaton ‘Goldband’ prescored borosilicate glass ampoules with a capacity of 1.2 millilitres were deemed acceptable. The manufacturers of ampoules caution against overfilling them (less than 0.5 ml, being recommended).
- The culture suspension-filled ampoules are frozen and securely sealed. Hwang (1966) suggests precooling to 7°C prior to freezing. The ampoules can be frozen by plunging them directly into the liquid nitrogen, suspending them briefly over the liquid nitrogen, and then lowering them into the liquid nitrogen, or by utilising controlled cooling. Choice of freezing method is influenced by a number of interdependent aspects, including the microorganisms to be frozen, the degree of resurrection required, and the equipment available.
- The frozen ampoules manufactured as described in (ii) are often clipped on metal (aluminium) canes, one on top of the other, with six on each cane. The canes are then placed in metal boxes or canisters (aluminium) that accommodate around 20 canes. These are perforated to allow liquid nitrogen to flow freely. The cultures are revived by removing them from the container, rapidly thawing them, and then cultivating them as usual.
Precautions
Ampoules that are defective or cracked are hazardous. Because liquid nitrogen can potentially permeate and fill the ampoules. These ampoules (filled with liquid nitrogen) may explode upon thawing due to the rapid expansion of the nitrogen into gas. Nitrogen in storage may also become contaminated. If thawing is performed in water and an explosion occurs, the water will tend to retain glass particles. When thawing ampoules, however, it is recommended that gloves and an eye or face shield be worn. Nitrogen should be handled with caution due to the potential of cold burns. Any metal components in direct contact with liquid nitrogen should be handled with extreme caution. As the gas is odourless and colourless, there is a minimal risk of asphyxiation if it is handled in a confined environment. Consequently, the presence of nitrogen in the atmosphere may be unnoticeable. Therefore, the nitrogen containers should be stored in a room with adequate ventilation.
Advantages of Nitrogen Storage
- It is an effective preservation method.
- There is no subculturing required.
- The cultural attributes have not changed. This could be crucial when storing highly specialised strains used in industrial processes.
- Since the ampoules are sealed, they are not susceptible to contamination or infection by mites.
- Living material that would not normally grow in a culture or be preserved in a culture collection can be maintained in a viable state.
Disadvantages of Nitrogen Storage
- The method is expensive because expensive equipment is required.
- A reliable nitrogen supply is required.
- Due to the use of explosive gas, there is a possibility of a minor accident occurring.
E. Other Methods of Storage for Fungi
No laboratory can afford lyophilization or storage of liquid nitrogen. Many technicians lack the time required for the periodic transfer to fresh media and find oil storage to be untidy. In modern times, when personnel safety is of paramount importance, the spatter produced by oily needles during sterilisation is undesirable. Therefore, numerous alternative preservation methods have been developed.
Soil cultures
This technique is utilised specifically for the preservation of sporulating microorganisms (e.g. Bacillus, Penicillium, Aspergillus and Streptomyces). The steps involved in this procedure are as follows:
- First, a spore suspension is prepared, which may require a specific medium.
- The preparation and distribution of tubes containing a mixture of soil (20%), sand (78%) and calcium carbonate (2%) (a few grammes per tube). They are chilled after being steriled for 8 to 15 hours at 130°C.
- A spore suspension is applied to the sterilised soil from step I and allowed to grow for approximately ten days.
- Under vacuum, the inoculated tubes from step (iii) are stored in a desiccator. This is done in order to evaporate extra water. The tubes are then sealed.
- The culture tubes are stored in a refrigerator between 5 and 8 degrees Celsius. Thus, spores can be maintained without germination for several years.
Silica gel cultures
- A spore suspension in 5% skim milk is added to screw-capped bottles of anhydrous silica gel.
- This is let to dry for approximately 14 days.
- The caps are then tightened. The bottles are often stored in a refrigerator, however keeping at room temperature is also acceptable.
- Prior to initiating the procedure, it is necessary to take the precaution of thoroughly cooling everything. This is due to the release of heat when water is introduced to silica gel.
- Exceptional revivals are obtained over the course of several years. This is an adaptation of the Roberts approach employed by Ogata (1962) for the maintenance of Neurospora genetic stocks.
Water storage
There have been reports of surprising success (Boeswinkel, 1976; Marx and Daniel, 1976) in storing fungal cultures in water. The following are the steps involved in this straightforward procedure:
- In a Petri dish, the fungus is grown on a poor medium and then chopped into little pieces.
- Using sterile technology, the fragments are transferred to small screw-capped bottles of sterile distilled water.
- The cap is tightened.
- The cultures are ideally preserved at a cool temperature.
The storage of spores in sand, the drying of cultures on filter paper, and the sealing of tubes with paraffin wax are additional methods of preservation.
4. Preparation Of Inoculum
- The number of microbial cells or spores necessary to inoculate large quantities of production medium in a fermentor (e.g., a 30,000-gallon tank) is significant, as a loopful of culture cannot satisfy the need to complete the fermentation run in the lowest time possible.
- Therefore, microorganisms from the stock culture are sequentially transferred to a specialised inoculum medium, with the volume increasing at each stage.
- Except for the initial step of inoculation (i.e., inoculation from a stock culture), all phases involve the transfer of between 0.5 and 5% of the inoculum from the preceding step.
- During inoculum establishment, the inoculum media and incubation conditions are such that rapid cell or spore development is favoured without or with negligible product accumulation.
- For instance, specific sporulating media is employed in the formation of inoculum during penicillin fermentation. This sporulating media encourages the rapid synthesis of spores but not penicillin.
- In terms of chemical makeup, inoculum media and production media are often distinct. In fermentations when enzymatic adaptation is sought, it is crucial that the inoculum medium contains an inciting substrate.
- This precaution avoids a pronounced lag phase in the production tank immediately following inoculation, allowing the organism to acclimatise. These media typically include a significantly lower concentration of the principal nutritional carbon source.
- In certain fermentations, it is preferable to separate the microbial cells or spores from the inoculum medium prior to using them to inoculate a production tank. This can be achieved as follows: I Exhaustion of a carbon source from the inoculum media just before to harvesting the inoculum can cause particular bacteria to flocculate and settle from the medium. Either the cells or the supernatant liquid can then be removed from the bottom of the culture vessel. OR (ii) The inoculum cells may be isolated from the inoculum medium through centrifugation, sedimentation, or a similar technique.
- Unfortunately, if the second approach is utilised, any inoculum medium particles will accompany the cells. In constructing the inoculum media, therefore, it is recommended to employ only fully soluble components.
- This precaution provides the inoculum cells without particle attachment to the inoculum medium. This approach of utilising only the inoculum cells is advantageous when substantial inoculum additions (e.g. 20%) are necessary.
- This minimises excessive dilution of the production medium, unintended pH changes, and unwanted matter carryover. The following are examples of high-level inoculum addition: I bisulfite-yeast-glycerol fermentation; and (ii) α, €-diaminopimelic acid-Enterobacter aerogenes-L-lysine fermentation.
- There is a chance that a microbial strain will suffer mutation during inoculum preparation, as microbial cells must pass through multiple generations.
- If the microorganism used in the fermentation process is not a mutant strain, there is no major issue. The rationale for this is because a particular mutation happens infrequently, and when it does, it is typically damaging to the microbial cell or provides no selective benefit to the microbial cell in its growing competition with wild (non-mutated) cells.
- In contrast, if a mutant fermentation strain is used in the fermentation process, it becomes a major issue. The explanation for this is the frequency with which reverse mutations occur.
- Due of the high frequency of back mutations, the final inoculum may contain a large proportion of back mutants. This can be circumvented by utilising growth media and incubation conditions that favour the growth of the mutant strain.
- During inoculum preparation, a mutant strain of Escherichia coli that requires L-lysine for growth and multiplication should not deplete its supply of this amino acid. If the inoculum medium exhausts its L-lysine supply, a high frequency of back mutations develops.
- As a result, the proportion of back mutants to mutant cells in the inoculum increases dramatically. In other words, the back mutant becomes dominant within the inoculum’s cellular population.
- Therefore, it is of the utmost significance that these modified fermentation strains be carefully preserved in stock-cultures so that as few back mutants as possible will arise in the inoculum.
- The procedure for transferring microbial growth from stock-cultures to liquid inoculum medium and initiating development in the liquid inoculum media relies on the microorganism engaged in the fermentation process. For the transfer in the liquid inoculum medium, bacterial cells or spores may be suspended in a sterile diluent (such as tap water).
- In addition, bacterial spores (e.g. Clostridium spores) frequently require “heat stunning” to promote spore germination. This is illustrated by the highly heat-resistant spores of Clostridium acetobutylicum.
- Heat cannot be used to germinate heat-sensitive spores of fungi and actinomycetes.
- The alternate approach for germinating such spores is to employ a media that can promote germination, and then transfer the germinated spores to the standard inoculum medium.
- Fungi and actinomycetes spore suspensions are prepared by adding an appropriate diluent to sporulated agar growth and then scraping the spores loose with a sterile loop.
- In some instances, a small amount of a non-toxic wetting agent must be added to the diluent to ensure the uniform distribution of spores in the diluent. In contrast, certain stationary fungal fermentations (e.g., Aspergillus niger- citric acid fermentation) require the spores to float on the surface of the liquid medium.
- In the case of non-sporulating or inadequately sporulating fungi or actinomycetes, fractured hyphae are utilised to transport the inoculum to the liquid media.
- Hyphae are fragmented by vigorously scraping the agar surface with a sterile loop, or by another means. Contamination must be monitored at each stage of inoculum development.
- In fermentations utilising the hyphae of fungi and actinomycetes, severe bacterial contamination is occasionally observable as general turbidity or even foaming.
- Typically, contamination is detected by microscopic examination and plating samples of the inoculum on a suitable medium that allows the growth of suspected contaminating microorganisms.
- To determine the presence of facultative anaerobes in inoculum for anaerobic fermentation, streaked plates should be incubated under both anaerobic and aerobic conditions.
- In the case of some fermentations (such as Clostridium acetobutylicum-acetone-butanol fermentation), a distinctive fermentation pattern indicates the presence of contamination.
- It may be concluded that inoculum manufacturing is a crucial aspect in both commercial production and scientific research, and that the quality and repeatability must be maintained.