What is Sterilization?
- Sterilization is a rigorous process designed to eliminate all forms of life, including microorganisms, from surfaces, objects, or fluids. This process ensures that all biological agents, including bacteria, fungi, viruses, prions, and their spores, are completely eradicated. Sterilization is essential in various fields, particularly in healthcare, microbiology, and food processing, to prevent contamination and ensure safety.
- To achieve sterilization, microorganisms are exposed to antimicrobial agents under specific conditions. These conditions typically include precise temperature, pressure, and exposure duration. The methods used for sterilization can be categorized into several types, each with its unique mechanism.
- Heat Sterilization is one of the most commonly employed techniques. It involves using high temperatures to kill microorganisms. This can be achieved through dry heat or steam. Dry heat sterilization requires prolonged exposure to high temperatures in an oven, while steam sterilization, also known as autoclaving, utilizes pressurized steam at high temperatures to achieve sterilization.
- Chemical Sterilization involves the use of chemical agents to destroy microorganisms. These chemicals can be gases, such as ethylene oxide, or liquids, such as glutaraldehyde. Chemical sterilization is often used for items that cannot withstand high temperatures or moisture.
- Irradiation uses various forms of radiation, such as ultraviolet light or gamma rays, to sterilize objects. UV light is effective for surface sterilization, while gamma rays penetrate deeper, making them suitable for sterilizing medical supplies and food products.
- High Pressure sterilization, also known as high-pressure processing, involves applying extreme pressure to a product, which disrupts the cellular structure of microorganisms, leading to their destruction. This method is particularly useful in the food industry.
- Filtration is a method that physically removes microorganisms from liquids or gases by passing them through a filter with pores small enough to trap the microorganisms. This technique is often used for sterilizing heat-sensitive solutions.
- Unlike disinfection, sanitization, and pasteurization, which reduce the number of microorganisms to safe levels but do not eliminate all forms of life, sterilization guarantees the complete removal or destruction of all microorganisms. Therefore, an object treated through sterilization is considered sterile or aseptic, indicating it is free of all living organisms and their spores.
Physical methods of sterilization
Physical methods of sterilization involves the control of microbial growth by using Sunlight, Heat, Filtration, Radiation, etc.
Physical methods of sterilization include the following:
- Temperature/Heat Sterilization
- Desiccation.
- Osmotic pressure.
- Radiation.
- Filtration.
- Sound (sonic) waves
- Sunlight (Solar Disinfection)
- Pressure (Pascalization)
A. Heat Sterilization
Heat sterilization is a fundamental technique in microbiology and medical sterilization, notable for its efficiency in destroying microorganisms through thermal means. This method operates on the principle of using elevated temperatures to induce bactericidal effects by disrupting critical cellular components.
- Mechanism of Action
- Destruction of Cellular Components: Heat sterilization primarily targets the destruction of enzymes and other vital cellular constituents. High temperatures lead to the denaturation of proteins and nucleic acids, which are essential for microbial survival and reproduction.
- Hydrated vs. Dry Conditions: The effectiveness of heat sterilization is enhanced in a fully hydrated state. Under conditions of high humidity, lower temperatures and shorter exposure times are required to achieve effective sterilization. This is due to the predominance of denaturation and hydrolysis reactions, which are more efficient in moist environments compared to dry conditions, where oxidative changes dominate.
- Types of Heat Sterilization
- Moist Heat Sterilization: Typically conducted at temperatures ranging from 121°C to 134°C using steam under pressure (autoclaving). This method is particularly effective because steam penetrates materials more efficiently than dry heat, leading to more uniform heat distribution and quicker microbial destruction.
- Dry Heat Sterilization: Performed at higher temperatures, usually between 160°C and 180°C. Dry heat sterilization is suitable for materials that can withstand higher temperatures without undergoing thermal degradation. The process involves prolonged exposure to heat, which is effective for items that are moisture-sensitive or cannot be autoclaved.
- Considerations
- Thermal Degradation: Heat sterilization can sometimes lead to partial degradation of materials, particularly when exposed to high temperatures for extended periods. This degradation can be minimized by optimizing the temperature and exposure time. Higher temperatures with shorter exposure times generally result in less thermal damage to the product.
- Applicability: Heat sterilization is applicable to both thermostable products, which can endure high temperatures, and moisture-sensitive products, for which dry heat sterilization is employed. Each method is chosen based on the nature of the item being sterilized and its susceptibility to heat and moisture.
Destruction of Microorganisms by Heat or Temperature
In the laboratory, the Destruction of Microorganisms by heat is accomplished in these two following methods;
- Destruction of Microorganisms by Applying High Temperature.
- Destruction of Microorganisms by Applying Low Temperature.
1. Destruction of Microorganisms by Applying High Temperature.
Practical procedures by which heat is employed are conveniently divided into two categories:
- Sterilization by Moist Heat
- Sterilization by Dry Heat
a. Sterilization by Moist Heat
Moist heat sterilization is a widely utilized and effective method for eliminating microorganisms through the use of steam under pressure. This process is distinguished by its ability to achieve high levels of sterility through specific temperature and pressure conditions.
- Mechanism of Action
- Steam and Pressure: Moist heat sterilization relies on steam at temperatures between 121°C and 134°C. The steam’s high pressure increases the boiling point of water, allowing the temperature to exceed that of boiling water. This elevated temperature is crucial for effective sterilization.
- Penetration and Coagulation: High pressure facilitates the rapid and uniform penetration of heat into materials. The moisture in the steam causes the coagulation of proteins within microorganisms. This process results in an irreversible loss of microbial function and activity, effectively killing the organisms.
- Temperature-Time Cycles
- Standard Cycles: The effectiveness of moist heat sterilization is often determined by specific temperature-time cycles. For porous materials such as surgical dressings, a standard cycle involves exposure to 134°C for 3 minutes. For bottled fluids and other aqueous solutions, the standard cycle is 121°C for 15 minutes.
- High Temperature-Short Time Cycles: These cycles are beneficial as they reduce fractional degradation of materials while ensuring high levels of sterility assurance. The shorter exposure times at high temperatures enhance the inactivation of microbial contaminants.
- Autoclave Operation
- Principle and Function: The autoclave is the primary device used for moist heat sterilization. It operates by generating steam under high pressure, which is then used to sterilize various items. The autoclave’s ability to maintain high pressure and temperature ensures that even deep-seated microorganisms are effectively destroyed.
- Applications
- Clinical and Pharmaceutical Uses: Moist heat sterilization is crucial in the medical and pharmaceutical sectors. It is employed for sterilizing surgical dressings, sheets, diagnostic equipment, containers, aqueous injections, ophthalmic preparations, and irrigation fluids. It also plays a role in processing soiled and contaminated items.
- Advantages Over Dry Heat
- Effectiveness: Compared to dry heat sterilization, which relies on oxidation to kill microorganisms, moist heat sterilization is generally more effective. The coagulation of proteins caused by moist heat ensures a higher level of microbial destruction.
Sterilization by moist heat can be classified in the following groups:
- Sterilization at a temperature < 100°C
- Sterilization at a temperature of 100°C
- Sterilization at a temperature > 100°C
- Intermittent sterilization
(i) Sterilization at a Temperature Below 100°C: Pasteurization
Pasteurization is a sterilization technique employed at temperatures below 100°C, designed to reduce microbial load in various substances without reaching boiling point. This process primarily targets the elimination of non-spore-forming microorganisms.
- Mechanism of Pasteurization
- Temperature and Time: Pasteurization involves heating liquids, such as milk, to specific temperatures for defined periods. The Holder method subjects milk to 63°C for 30 minutes, while the Flash method uses a higher temperature of 73°C for 20 seconds. These conditions are sufficient to kill most pathogenic microbes without boiling the liquid.
- Microbial Reduction: The goal of pasteurization is not to achieve complete sterilization but to reduce the number of viable microorganisms to a level where they are unlikely to cause disease. This process effectively targets mesophilic, non-spore-forming bacteria, which are commonly found in foods and beverages.
- Effectiveness and Limitations
- Pathogen Control: While pasteurization is effective against many non-spore-forming bacteria, it does not eliminate all pathogenic organisms. The principle behind pasteurization is the logarithmic reduction of viable microbes, ensuring that their numbers are significantly reduced but not entirely eradicated.
- Temperature Sensitivity: Pasteurization is performed below the boiling point to prevent the curdling of milk and to preserve its nutritional quality. For instance, milk heated to temperatures above its boiling point could suffer from texture changes and nutrient loss.
- Applications
- Milk and Dairy Products: Pasteurization is commonly used in the dairy industry to ensure the safety of milk and milk products. By applying controlled heat, pathogens are eliminated, and the shelf life of these products is extended.
- Other Fluids and Equipment: Besides milk, pasteurization is applied to other fluids such as vaccines and serum, especially when dealing with non-spore-forming bacteria. Vaccines are pasteurized at 60°C for 1 hour, while serum and body fluids containing congealable proteins are processed at 56°C for 1 hour in water baths.
(ii) Sterilization at a temperature of 100°C
Sterilization at 100°C primarily involves two methods: boiling and steam sterilization. This temperature is effective for controlling microbial contamination, but it does not ensure complete sterility.
- Boiling
- Process: Boiling sterilization involves immersing items in distilled water heated to 100°C for a period of 30 to 40 minutes. The use of distilled water is crucial as hard water can lead to the formation of calcium salt films on the items, which can impede the sterilization process.
- Effectiveness: Boiling effectively eliminates most vegetative forms of bacteria and some resistant species, such as tubercle bacilli and staphylococci. However, it is not effective against bacterial spores, which may survive the boiling process. Therefore, while boiling is a useful method for disinfection, it is not sufficient for complete sterilization.
- Limitations: One significant drawback is that items sterilized by boiling can become easily recontaminated once removed from the boiling water. This limitation makes boiling unsuitable for sterilizing surgical instruments or any items requiring a high level of sterility.
- Steam Sterilization at 100°C
- Process: Steam sterilizers, such as Koch’s or Arnold’s steam sterilizer, operate at 100°C and atmospheric pressure. These devices expose materials to steam for approximately 90 minutes. This method is particularly suited for heat-labile substances that cannot withstand the higher temperatures and pressures of autoclaving.
- Effectiveness: Steam sterilization at 100°C effectively destroys most vegetative bacterial forms, including those resistant to boiling, such as certain thermophiles. However, similar to boiling, this method does not ensure the destruction of all bacterial spores.
- Applications: This method is appropriate for items that are sensitive to heat and pressure, such as certain laboratory media containing sugar or gelatin. Tyndallization, a specific application of steam sterilization, involves subjecting media to steam at 100°C for 30 minutes on three consecutive days to avoid the decomposition of sugar at higher temperatures.
(iii) Sterilization at a temperature > 100°C / steam under pressure
Sterilization at temperatures exceeding 100°C is primarily achieved through the use of steam under pressure. This method is crucial for ensuring the complete elimination of microorganisms, including bacterial spores, which are more resistant to lower temperatures.
- Steam Under Pressure
- Principle: Water typically boils at 100°C under standard atmospheric pressure (760 mm Hg). However, increasing the pressure raises the boiling point of water. This principle is harnessed in devices known as autoclaves.
- Autoclave Operation: An autoclave operates by increasing the pressure to 15 psi (pounds per square inch) or 775 mm Hg, raising the boiling point of water to approximately 121°C. This higher temperature enhances the sterilization process.
- Mechanism: Steam under pressure penetrates materials more effectively than at lower temperatures. Upon contact, the steam releases latent heat, which provides the thermal energy necessary to kill microorganisms. The condensation of steam into a liquid ensures that the microbial destruction is both thorough and uniform.
- Applications
- Contaminated Instruments: Autoclaves are employed extensively for sterilizing contaminated instruments. The high temperature and pressure ensure that all forms of microbial life, including the most resistant spores, are effectively eradicated.
- Culture Media: Autoclaves are also used for sterilizing various culture media in laboratories. This process is critical for preparing media free of contaminants that could interfere with microbial growth studies.
- Advantages of High-Temperature Sterilization
- Complete Sterility: The combination of high temperature and pressure in an autoclave provides a reliable method for achieving complete sterility. This is particularly important in medical and laboratory settings where the risk of infection or contamination must be minimized.
- Penetration Power: Steam at temperatures above 100°C has enhanced penetrating power compared to dry heat or steam at lower temperatures. This ensures that even complex or densely packed items are thoroughly sterilized.
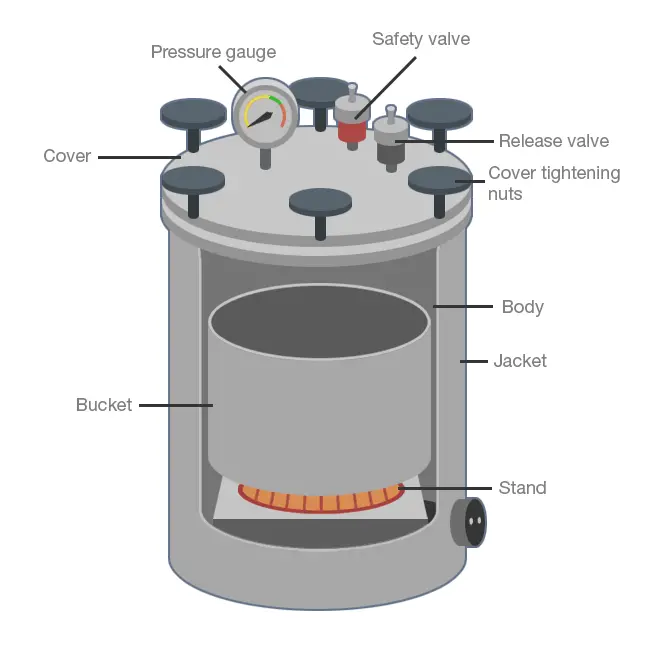
(iv) Intermittent sterilization
Intermittent sterilization, also known as tyndallization, is a method designed for heat-labile substances that cannot endure the high temperatures of conventional autoclaving. This process is particularly useful for materials such as serum, sugar solutions, and eggs, which are sensitive to continuous high heat.
- Overview of Tyndallization
- Principle: Tyndallization operates over a three-day period. It involves a series of steam treatments to ensure that all microorganisms, including heat-resistant spores, are eliminated.
- Procedure:
- Day 1: Items are exposed to steam at 100°C for 20 minutes. This initial exposure kills most vegetative forms of bacteria, yeasts, and molds. However, it does not destroy spores, which are more heat-resistant.
- Day 2: The items are left to rest, allowing any surviving spores to germinate into vegetative forms. These newly formed vegetative cells are then targeted in the subsequent steam treatment.
- Day 3: The items undergo a second exposure to steam at 100°C for 20 minutes. This final treatment ensures the destruction of the vegetative forms resulting from the previous day’s germination, effectively eliminating all spores.
- Application
- Heat-Sensitive Media: Tyndallization is commonly used to sterilize culture media that contain heat-sensitive components, such as sera (e.g., Loeffler’s serum slope), egg-based media (e.g., Lowenstein-Jensen medium), and carbohydrate solutions (e.g., serum sugars).
- Food Products: Some canned foods are also sterilized using tyndallization, especially when they contain components that cannot withstand high temperatures for prolonged periods.
- Advantages and Limitations
- Advantages:
- Preservation of Heat-Labile Substances: The process is effective for materials that might be damaged or degraded by continuous high-temperature exposure.
- Comprehensive Sterilization: By allowing spores to germinate into vegetative forms between steam exposures, tyndallization ensures that all microbial forms are addressed.
- Limitations:
- Extended Time Requirement: The process spans three days, which can be time-consuming compared to other sterilization methods.
- Inefficiency for All Spores: Although effective for many spores, some highly resistant types may not be fully eliminated.
- Advantages:
Applications of Moist Heat Sterilization
- Sterilization of Beverages
- Milk and Fresh Beverages: Moist heat sterilization is extensively used to treat milk and other fresh beverages such as fruit juices, beer, and wine. These beverages are prone to contamination during collection and processing. The process ensures that pathogenic microorganisms are eliminated, thus extending shelf life and ensuring safety for consumption.
- Hospital Disinfection
- Utilities and Equipment: In a hospital setting, moist heat sterilization is employed to disinfect utilities and equipment. This includes items such as surgical instruments, cloths, and other hospital utilities that require thorough sterilization to prevent infection and maintain a sterile environment.
- Sterilization of Heat-Resistant Materials
- Glassware and Metal Instruments: Moist heat is effective for sterilizing heat-resistant materials. This category includes glassware, metallic instruments, and some types of heat-resistant plastics. The process ensures that these items are free from all forms of microbial life before use in clinical or research settings.
- Cloth and Rubber Items: Surgical dressings and rubber gloves, which are commonly used in medical environments, can also be sterilized using moist heat. This is crucial for preventing cross-contamination and maintaining aseptic conditions.
- Paper and Some Media: Paper products and certain media that are resistant to heat can be treated to remove contaminants. This is particularly important in laboratories where contamination can impact experimental results.
- Sterilization of Heat-Sensitive Items
- Plastic Petri Plates: For heat-sensitive materials like plastic Petri plates that cannot withstand high temperatures, moist heat sterilization is used as a controlled method to ensure they are sterile without damaging their structural integrity.
- Sterilization of Culture Media
- Heat-Sensitive Media: Moist heat is also applied to sterilize heat-sensitive culture media that contain components such as sera (e.g., Loeffler’s serum slope), egg (e.g., Lowenstein-Jensen medium), and carbohydrate solutions (e.g., serum sugars). These media are essential for microbial culture and must be free from contaminants to ensure accurate experimental results.
- Canned Foods: Some canned foods that contain heat-sensitive ingredients are sterilized using moist heat to prevent spoilage and ensure safety.
Method | Recommended Uses | Limitations |
Autoclave | Sterilizing instruments, linens. utensils, and treatment trays. media and other liquids | Ineffective against organisms in materials impervious to steam; cannot be used for heat-sensitive articles |
Free-flowing steam or boiling water | Destruction of nonsporeforming pathogens, sanitizes bedding. clothing, and dishes | Cannot be guaranteed to produce sterilization on one exposure |
Pasteurization | used for sterilization of milk and other fresh beverages, such as fruit juices, beer, and wine | Doesn’t kill heat resistant pathogens. Reduction in the nutrition content, It kills pathogens. Enhances storage period. |
Steam sterilizer | used for heat-labile substances | Deleterious for heat-sensitive instruments, Microsurgical instruments damaged by repeated exposure, May leave instruments wet, causing them to rust. Potential for burns. |
Tyndallization | used most often to sterilize heat-sensitive culture media, such as those containing sera (e.g., Loeffl er’s serum slope), egg (e.g., Lowenstein–Jensen’s medium), or carbohydrates (e.g., serum sugars) and some canned foods. | – |
Approximate Conditions for Moist Heat Inactivation
Entity | Vegetative Cells | Spores |
Yeasts | 5 minutes at 50-60°C | 5 minutes at 70-80°C |
Molds | 30 minutes at 62°C | 30 minutes at 80°C |
Bacteria | 10 minutes at 60-70°C | 2 to over 800 minutes at 100°C 0.5-12 minutes at 121C |
Viruses | 30 minutes at 60°C | – |
Prions | 90 minutes at 134°C | – |
b. Dry heat sterilization
Dry heat sterilization is a technique utilized to eliminate microorganisms through the application of heat in the absence of moisture. This method is particularly suited for items and substances that are sensitive to moisture or that cannot be effectively sterilized by moist heat methods.
- Principle and Mechanism
- Heat Conduction: Dry heat sterilization operates on the principle of conduction. In this process, heat is transferred from the outer surface of an item inward until the entire item reaches the desired temperature for sterilization. Unlike moist heat, which uses steam to penetrate and kill microorganisms, dry heat relies on direct heat transfer to achieve its effects.
- Mechanism of Microbial Destruction: The effectiveness of dry heat lies in several destructive processes:
- Denaturation of Proteins: Heat causes proteins within microorganisms to denature, disrupting their structure and function.
- Protein Lysis: Continued exposure to heat can cause the breakdown or lysis of proteins.
- Oxidative Damage: Dry heat can induce oxidative stress, generating free radicals that damage cellular components.
- Cell Drying and Burning: Extended heat exposure can desiccate cells and, in extreme cases, lead to their combustion, as seen in incineration.
- Applications and Limitations
- Substances and Materials:
- Moisture-Sensitive Substances: Items such as oils, powders, and other substances that cannot withstand moisture are ideal candidates for dry heat sterilization. Moisture can either destroy these substances or fail to penetrate effectively, making dry heat a preferable option.
- Laboratory Equipment: Equipment like Petri dishes and pipettes, which may be challenging to sterilize with moist heat due to penetration issues, are suitable for dry heat methods.
- Temperature and Exposure Time: To effectively sterilize, dry heat requires higher temperatures than moist heat. Typically, temperatures range from 160°C to 180°C, with exposure times varying up to 2 hours depending on the exact temperature. This extended time is necessary to ensure that the entire item reaches the required temperature for complete microbial destruction.
- Substances and Materials:
- Industrial and Medical Applications
- Glass Bottle Sterilization: A primary industrial application of dry heat sterilization is in the preparation of glass bottles for aseptic filling. The method ensures that bottles are free from microorganisms and suitable for use in sterile environments.
- Destruction of Endotoxins: Dry heat sterilization is also effective in destroying bacterial endotoxins, or pyrogens, which are difficult to eliminate through other sterilization techniques. For depyrogenation of glass, temperatures of approximately 250°C are employed to ensure the complete destruction of these harmful substances.
- Equipment Used
- Hot Air Ovens: Hot air ovens are commonly used to provide the necessary dry heat for sterilization. These ovens generate a controlled environment of hot, moisture-free air.
- Incinerators: Incinerators are used for the complete destruction of materials through combustion, particularly for waste materials that require absolute sterility.
Sterilization by dry heat is accomplished by the following methods;
- Red Heat
- Flaming,
- Incineration
- Hot air oven.
Types of Dry Heat Sterilization
Dry heat sterilization employs heat in the absence of moisture to achieve microbial eradication. This method is suitable for materials that are sensitive to moisture or cannot withstand steam sterilization. Below are the primary types of dry heat sterilization:
- Red Heat
- Process Overview: Red heat sterilization involves heating instruments to a red-hot state using a direct flame. The intense heat ensures that microorganisms are destroyed effectively.
- Mechanism: Instruments are exposed to a Bunsen burner flame until they glow red, achieving temperatures sufficient to destroy microorganisms through protein denaturation and oxidative damage.
- Applications: This method is primarily used for sterilizing metal instruments such as incubation loops, wires, and the tips of forceps.
- Limitations: It is applicable only to items that can endure such high temperatures without being damaged. Heat-sensitive materials cannot be processed using this method.
- Process Overview: Red heat sterilization involves heating instruments to a red-hot state using a direct flame. The intense heat ensures that microorganisms are destroyed effectively.
- Flaming
- Process Overview: Flaming involves exposing metallic objects to a flame to burn off microbes and other contaminants.
- Mechanism: Before being exposed to the flame, the instrument is often dipped in alcohol or a similar flammable liquid. The subsequent flame burns off the microbes.
- Applications: Flaming is used for sterilizing smaller metallic tools and instruments in laboratory settings.
- Limitations: This method does not guarantee complete sterility as effectively as red heat sterilization. It is less thorough and is generally used for quick decontamination rather than full sterilization.
- Process Overview: Flaming involves exposing metallic objects to a flame to burn off microbes and other contaminants.
- Incineration
- Process Overview: Incineration is a process used for both sterilization and significant volume reduction of waste. It involves burning materials to ashes.
- Mechanism: Waste materials are subjected to high temperatures in an incinerator until they are reduced to ash. This process not only sterilizes but also disposes of the waste.
- Applications: Commonly employed for the disposal of hospital waste and other biological residues.
- Limitations: This method is not typically used for laboratory instruments or materials intended for reuse, due to the complete destruction of the items.
- Process Overview: Incineration is a process used for both sterilization and significant volume reduction of waste. It involves burning materials to ashes.
- Infrared Radiation (IR) Sterilization
- Process Overview: Infrared radiation sterilization uses IR radiation absorbed by materials to generate heat for sterilization.
- Mechanism: Instruments and glassware are placed in a tray and passed through an IR tunnel on a conveyor belt. The IR radiation heats the items to about 180°C over a duration of approximately 17 minutes.
- Applications: This method is suitable for mass sterilization of packaged items like syringes and catheters.
- Limitations: It is less effective for items with high heat capacity or irregular shapes that might not uniformly absorb IR radiation.
- Process Overview: Infrared radiation sterilization uses IR radiation absorbed by materials to generate heat for sterilization.
- Hot Air Oven
- Process Overview: A hot air oven utilizes dry heat for sterilization through conduction, where heat is transferred from the outer surface to the inner layers of the material.
- Mechanism: The oven consists of an insulated chamber equipped with a fan, temperature sensors, and control mechanisms. Typical sterilization parameters are 170°C for 30 minutes, 160°C for 60 minutes, and 150°C for 150 minutes.
- Applications: Hot air ovens are used for sterilizing glassware, Petri plates, powders, and other materials that cannot be sterilized by moist heat.
- Limitations: The effectiveness depends on the uniform distribution of heat and the ability of the material to withstand prolonged exposure to high temperatures.
- Process Overview: A hot air oven utilizes dry heat for sterilization through conduction, where heat is transferred from the outer surface to the inner layers of the material.
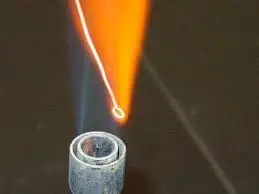
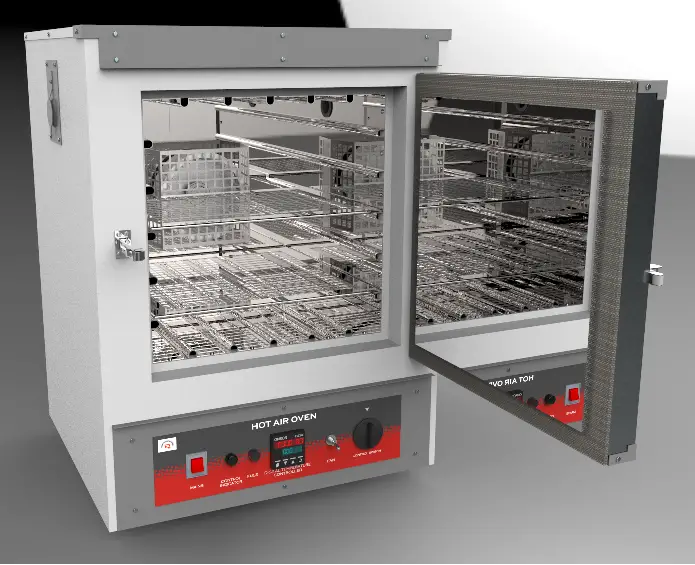
Applications of Dry heat Sterilization
The following points detail its specific applications:
- Sterilization of Glassware:
- Purpose: Glass items such as Petri dishes, flasks, pipettes, and test tubes are often sterilized using dry heat.
- Method: The heat effectively destroys microbial life without damaging the glass, which is resistant to high temperatures.
- Sterilization of Surgical Instruments:
- Purpose: Instruments like scalpels, scissors, and forceps, which are critical in clinical procedures, require thorough sterilization.
- Method: Dry heat ensures the complete eradication of pathogens from metal instruments that can withstand high temperatures without corroding.
- Sterilization of Chemicals and Oils:
- Purpose: Substances such as liquid paraffin and sulfonamide powders, which are not efficiently sterilized by moist heat, are treated with dry heat.
- Method: Dry heat is used to ensure the complete destruction of microbial contaminants in these substances, as they are less permeable to steam.
- Complete Destruction of Infectious Material:
- Purpose: Items such as syringes, needles, culture materials, dressings, bandages, bedding, animal carcasses, and pathology samples require complete destruction to prevent infection spread.
- Method: Dry heat is employed to incinerate these materials, ensuring that all microbial life is completely eradicated.
- Sterilization of Laboratory Tools:
- Purpose: Tools like inoculating loops, wire, the tips of forceps, and searing spatulas are sterilized to prevent contamination in experiments.
- Method: The application of dry heat provides a reliable method for ensuring that these tools are free from microorganisms before use.
Method | Recommended Uses | Limitations |
Hot-air oven | Sterilizing materials impermeable to or damaged by moisture, e.g., oils, glass, sharp instruments, metals | Destructive to materials which cannot withstand high temperatures for long periods |
Incineration | Recomitended Uses Disposal of contaminated objects that cannot be reused. | Size of Incinerator must be ade quate to burn largest load promptly and completely: potential of air pollution. |
Flaming | Sterilization of inoculating loop or wire, the tip of forceps, searing spatulas, etc., | – |
Incineration | used for complete destruction and disposal of infectious material, such as syringes, needles, culture material, dressings, bandages, bedding, animal carcasses, and pathology samples | not effective for metals and heat-resistant glass materials. |
2. Destruction of Microorganisms by Applying Low Temperature
Low-temperature treatment is a well-established method for controlling and preserving microorganisms. This process relies on the ability of low temperatures to slow down or halt microbial metabolism and growth. Here is a detailed explanation of how low temperatures affect microorganisms and their applications in sterilization and preservation:
Effects of Low Temperature on Microorganisms
- Metabolic Rate Reduction
- Depression of Metabolism: Low temperatures significantly reduce the metabolic rate of microorganisms. Enzymatic reactions, which are crucial for metabolic processes, slow down as the temperature decreases.
- Cease of Activity: At sufficiently low temperatures, microbial growth and metabolic activities may completely stop. Microorganisms can enter a dormant state where no detectable metabolic processes occur.
- Dormancy
- Preservation of Viability: When exposed to freezing or subfreezing temperatures, microorganisms often enter a dormant phase. During this phase, they do not exhibit any visible signs of life but retain their viability. This dormancy is crucial for the long-term preservation of cultures.
Methods of Low-Temperature Preservation
- Freeze Drying (Lyophilization)
- Process Overview: Freeze drying, also known as lyophilization, involves freezing microorganisms and then removing the ice by sublimation under vacuum. This method preserves microorganisms in a dry state, maintaining their viability over extended periods.
- Applications: Freeze-drying is commonly used for preserving bacterial cultures, yeast, molds, and some viruses. It allows for long-term storage without significant loss of viability.
- Refrigeration
- Short-Term Storage: For short-term preservation, microorganisms are often stored at refrigeration temperatures, typically between 4°C and 7°C. This temperature range is suitable for maintaining agar-slant cultures of bacteria, yeasts, and molds.
- Efficiency: Refrigeration slows down microbial metabolism but does not halt it entirely. It is effective for maintaining cultures that are intended for short-term use.
- Deep Freezing
- Extended Preservation: Deep freezing involves storing microorganisms at temperatures ranging from -20°C to -70°C. This method is suitable for the long-term preservation of many bacteria and viruses.
- Usage: Deep-freeze units are employed to store a wide variety of microbial samples, ensuring their stability and viability over extended periods.
- Liquid Nitrogen
- Ultra-Low Temperatures: Liquid nitrogen, with a temperature of -196°C, is used for ultra-low temperature preservation. This method is ideal for maintaining viruses, microorganisms, and mammalian tissue cells used in various research fields.
- Benefits: The extremely low temperatures achieved with liquid nitrogen provide excellent preservation of cellular integrity and viability.
Applications and Benefits
- Microbial Culture Preservation
- Research and Clinical Use: Low-temperature preservation is essential in research, clinical microbiology, and biotechnology. It ensures the availability of viable microbial cultures for ongoing experiments and diagnostic purposes.
- Safety and Stability
- Reduced Risk of Contamination: Storing microorganisms at low temperatures minimizes the risk of contamination and microbial overgrowth, making it a reliable method for maintaining culture purity.
- Convenience and Efficiency
- Storage Flexibility: Low-temperature methods offer flexibility in storage duration and conditions, accommodating various needs in laboratory and industrial settings.
B. Desiccation
Desiccation is a process used to control and preserve microorganisms by removing their moisture content. This method effectively halts metabolic activity and reduces microbial viability. Here is a detailed examination of how desiccation impacts microorganisms, its effectiveness, and its applications:
Effects of Desiccation on Microorganisms
- Cessation of Metabolic Activity
- Drying Process: Desiccation involves the removal of water from microbial cells. This leads to a halt in metabolic processes, as water is a crucial medium for enzymatic and biochemical reactions.
- Reduction in Viability: As the water content decreases, microbial cells become inactive, which results in a decline in the total viable population. The extent of this decline varies among different microorganisms.
- Survival Variability
- Microbial Sensitivity: Different microorganisms exhibit varying levels of resistance to desiccation. For instance, Gram-negative cocci, such as Neisseria gonorrhoeae and Neisseria meningitidis, are highly sensitive to desiccation and can die within hours. Conversely, Streptococci show greater resistance, with some surviving for weeks post-drying.
- Long-Term Viability: Mycobacterium tuberculosis, the causative agent of tuberculosis, remains viable for extended periods when dried in sputum. Moreover, spores of certain microorganisms are known to withstand desiccation indefinitely, demonstrating exceptional resilience.
Methods of Desiccation
- Lyophilization (Freeze-Drying)
- Process Overview: Lyophilization involves the extreme dehydration of microorganisms while they are in a frozen state. This is followed by placing the organisms in a vacuum to remove any remaining water via sublimation.
- Advantages: The lyophilized (dried) cultures can remain viable for many years due to the absence of moisture, which is essential for microbial activity. This method ensures long-term preservation without significant loss of viability.
Applications of Desiccation
- Pharmaceutical Industry
- Shelf Life Extension: In pharmaceuticals, lyophilization is employed to increase the shelf life of products, such as vaccines and injectable medications. By reducing the moisture content, these products are less prone to degradation and contamination.
- Preservation of Bioactive Compounds: The process helps preserve the stability and efficacy of bioactive compounds in pharmaceuticals, ensuring that products remain effective until use.
- Biotechnology
- Microbial Culture Storage: In biotechnology, desiccation techniques, including lyophilization, are used to store microbial cultures for research and industrial applications. This method facilitates the long-term preservation of microbial strains used in various biotechnological processes.
Factors Influencing Desiccation
- Microorganism Type
- Species Sensitivity: The survival rate post-desiccation is influenced by the type of microorganism. Some microorganisms are more sensitive to drying, while others can endure prolonged periods of desiccation.
- Material and Process Completeness
- Substrate and Drying Efficiency: The material in or on which microorganisms are dried affects their survival. Additionally, the completeness of the drying process plays a crucial role in determining how long microorganisms can remain viable.
- Environmental Conditions
- Exposure Conditions: The physical conditions to which dried microorganisms are exposed, such as temperature and humidity, can impact their longevity and viability.
C. Osmotic Pressure
Osmotic pressure is a fundamental concept in microbiology that describes the movement of water across a semipermeable membrane due to differences in solute concentration. This process has significant implications for microbial cell integrity and function. Here is an in-depth exploration of osmotic pressure and its effects on microorganisms:
Basic Principles of Osmotic Pressure
- Movement of Water Across Membranes
- Semipermeable Membrane: When two solutions with different solute concentrations are separated by a semipermeable membrane, water moves through the membrane from the region of lower solute concentration to the region of higher solute concentration. This movement aims to equalize the solute concentration on both sides of the membrane.
- Equalization of Concentrations
- Goal of Osmosis: The primary objective of osmotic movement is to balance solute concentrations across the membrane, resulting in equal concentrations on either side.
Effects on Microbial Cells
- Plasmolysis
- Process: In microorganisms with a solute concentration of approximately 0.95 percent, exposure to solutions with higher solute concentrations leads to the movement of water out of the cells. This process, known as plasmolysis, causes the cell to shrink as it loses water.
- Consequences: Plasmolysis results in cell dehydration, which can retard or halt metabolic processes. This effect is similar to that observed with desiccation.
- Plasmoptysis
- Process: Conversely, if microbial cells are placed in a solution with lower solute concentrations, water enters the cells. This influx of water is referred to as plasmoptysis.
- Osmotic Pressure: The entry of water into the cell increases internal pressure, known as osmotic pressure. This pressure is the result of water molecules accumulating inside the cell, exerting force against the cell membrane.
Observations and Implications
- Animal Cells vs. Microbial Cells
- Animal Cells: Animal cells, which lack rigid cell walls, exhibit more noticeable changes in shape and volume due to osmotic pressure. The absence of a rigid cell wall means these cells can experience significant deformation during plasmolysis and plasmoptysis.
- Microbial Cells: In contrast, microbial cells, particularly bacteria with rigid cell walls, do not distort as dramatically under osmotic pressure. However, changes can still occur in the cytoplasmic membrane and protoplast (the living part of the cell) during plasmolysis.
- Antimicrobial Effects
- Similar to Desiccation: The antimicrobial effects of osmotic pressure are akin to those caused by desiccation. The primary impact is on the cell’s internal environment, leading to metabolic disruption or cessation.
- Cell Wall Rigidity: Due to the rigid nature of microbial cell walls (except in protozoa), osmotic pressure does not cause the same level of structural distortion as seen in animal cells. However, the shrinkage of the protoplast from the cell wall during plasmolysis can still be observed.
D. Radiation
Radiation is a prominent method for sterilization, harnessing the power of electromagnetic waves to eliminate microorganisms. The primary types of radiation utilized for this purpose are ionizing and non-ionizing radiation. Each type possesses distinct characteristics and applications.
a. Ionizing Radiation
Ionizing radiation is a high-energy form that disrupts atomic and molecular structures by removing electrons. This type of radiation is highly effective in sterilization due to its substantial penetrating power. It can eradicate bacterial endospores and vegetative cells, though it is less effective against viruses. The main forms of ionizing radiation include X-rays, gamma rays, and cosmic rays.
- X-rays
- Characteristics: X-rays have considerable energy and penetration capabilities, making them lethal to microorganisms and higher forms of life.
- Applications: Historically, X-rays have been used experimentally to induce microbial mutations. However, they are not commonly employed for routine sterilization due to high production costs and inefficient utilization, as X-rays emanate in all directions from their source.
- Gamma Rays
- Characteristics: Gamma rays are high-energy emissions from radioactive isotopes. They are similar to X-rays but possess shorter wavelengths and higher energy levels.
- Applications: Gamma rays are highly effective for sterilizing materials of substantial thickness or volume, such as packaged foods and medical devices. Their penetrating power and microbicidal properties make them suitable for commercial sterilization.
- Cosmic Rays
- Characteristics: Cosmic rays consist of high-energy protons and atomic nuclei traveling through space at nearly the speed of light. They originate from various cosmic sources, including the Sun and distant galaxies.
- Applications: Direct sunlight, which includes cosmic rays, is naturally effective for sterilizing water in natural sources like tanks and rivers. Sunlight has a germicidal effect due to its ultraviolet and heat rays, rapidly destroying bacteria present in these environments.
b. Non-Ionizing Radiation
Non-ionizing radiation does not possess enough energy to ionize atoms or molecules. It includes forms such as ultraviolet (UV) and infrared radiation, which are utilized in different sterilization contexts.
- Ultraviolet (UV) Radiation
- Characteristics: UV radiation, particularly with wavelengths between 240–280 nm, exhibits significant bactericidal activity. It works by denaturing bacterial proteins and disrupting DNA replication.
- Applications: UV radiation is primarily used for disinfecting enclosed environments such as microbiology laboratories, inoculation hoods, laminar flow cabinets, and operating theaters. While effective against most vegetative bacteria, UV radiation is not as effective against bacterial spores, which are more resistant. UV radiation does not penetrate materials like glass, dirt films, or water very well, limiting its effectiveness in some scenarios.
- Infrared Radiation
- Characteristics: Infrared radiation has longer wavelengths than visible light and is invisible to the human eye.
- Applications: Infrared radiation is employed for the rapid and mass sterilization of disposable items such as syringes and catheters. It is particularly useful in industrial settings where high throughput is required.
Method | Recommended Uses | Limitations |
Ultraviolet light | Control of airborne infection: disinfection of surfaces | Must be absorbed to be effective (does not pass through transparent glass or opaque objects): irritating to eyes and skin; low penetration |
X-ray, gamma, and cathode radiation | Sterilization of heat-sensitive surgical materials and other medical devices. | Expensive and requires special facilities for use |
E. Filtration sterilization
Filtration is a crucial method employed for the sterilization and clarification of heat-sensitive solutions and gases. Unlike other sterilization techniques that rely on destruction of microorganisms, filtration physically removes contaminants through specific mechanisms. This process is indispensable in various scientific, industrial, and medical applications. The following details outline the fundamental aspects and types of filtration:
Types of Filtration
- Depth Filters
- Composition: Depth filters are constructed from fibrous or granular materials arranged in a thick layer. These materials form a network of small diameter channels.
- Function: The solution containing microorganisms is drawn through this layer, where particles are removed through physical screening, entrapment, and adsorption.
- Types of Depth Filters:
- Candle Filters: Made from diatomaceous earth or unglazed porcelain, these filters come in varying porosities and are commonly used for water purification.
- Asbestos Filters: Composed of asbestos materials, these filters have high adsorption capacity and are used in single-use applications. However, their use is limited due to the carcinogenic nature of asbestos.
- Sintered Glass Filters: These filters are made from powdered glass fused together. They offer low absorption and are reusable, though they are brittle and expensive.
- Membrane Filters
- Composition: Membrane filters are crafted from materials such as cellulose acetate, cellulose nitrate, polycarbonate, and polyvinylidene fluoride. They are typically circular, porous membranes with a thickness of about 0.1 mm.
- Function: Membrane filters remove microorganisms based on pore size. Commonly used pore sizes are around 0.2 µm, which is smaller than most bacteria, allowing for effective removal of vegetative cells but not viruses.
- Operation: Membranes are housed in special holders and often preceded by depth filters to remove larger particles. The filtration process involves pushing the solution through the membrane using vacuum, pressure from a syringe, or other means, collecting the filtered liquid in sterilized containers.
Filtration Mechanisms
- Sieving: Particles are removed based on size exclusion, where larger particles are retained while smaller ones pass through.
- Adsorption: Microorganisms adhere to the filter material, effectively removing them from the solution.
- Trapping: Particles are physically captured within the matrix of the filter material.
Applications of Filtration sterilization
- Sterilization of Liquids:
- Membrane Filters: Used for filtering heat-sensitive injections and ophthalmic solutions, ensuring they are free from microbial contamination without the need for heat. Membrane filters are often combined with pre-filters to enhance their capacity for handling particulate matter.
- Pressure and Vacuum Filtration: Membrane filters can be utilized in pressure-operated filter holders or vacuum filtration systems to process liquids efficiently.
- Sterilization of Gases:
- High-Efficiency Particulate Air (HEPA) Filters: These filters, consisting of pleated sheets of glass microfibers, are highly effective in removing particles, including microorganisms, from air. They are used in environments such as laminar flow cabinets and HVAC systems to ensure air cleanliness.
- Applications: HEPA filters are employed in various systems, including venting systems on fermenters, centrifuges, autoclaves, and freeze dryers.
- Additional Applications:
- Medical Gases: Filtration is used to ensure the cleanliness of medical gases, utilizing filters such as glass wool and hydrophobic membranes.
- Air Decontamination: In mechanical ventilators and microbiological safety cabinets, filters such as glass fiber and HEPA filters are used to remove contaminants from the air.
Method | Recommended Uses | Limitations |
Membrane filters | Sterilization of heat-sensitive biological fluids | Fluid must be relatively free of sus. pended particulate matter |
Fiberglass filters (HEPA) | Air disinfection | Expensive |
F. Sound (sonic) waves
Sonic waves, particularly ultrasound, are utilized as bactericidal agents through a process involving high-frequency vibrations to disrupt and eliminate microorganisms. This method leverages the physical effects of sound waves to enhance cleaning and sterilization, particularly in environments where traditional methods may be less effective.
Mechanism of Action
- Ultrasound Frequency and Medium
- Frequency Range: Ultrasound used for cleaning typically operates between 20 and 40 kHz. The effectiveness of this process is contingent on the frequency and the medium through which the sound waves are transmitted.
- Medium Choice: While ultrasound can be effective with water alone, the use of a solvent suited to the specific object and type of contamination can improve the cleaning efficacy.
- Formation of Cavitation
- Cavitation Process: As ultrasonic waves travel through a detergent solution, they generate alternating tensile and compressive forces. This creates millions of microscopic cavities within the solution.
- Cavity Collapse: When these cavities reach a critical size, they collapse violently, leading to the formation of high-energy shock waves. This collapse generates intense heat and pressure.
- Shock Wave Generation
- Temperature and Pressure: The shock waves produced can reach temperatures as high as 10,000°F and pressures as low as 10,000 PSI. These extreme conditions are sufficient to dislodge and remove microorganisms and debris from the surfaces of contaminated instruments.
Factors Influencing Effectiveness
- Sound Intensity and Duration
- Intensity: The bactericidal effect of airborne sound waves is dependent on the intensity of the sound and the duration of exposure.
- Distance: The distance between the sample and the sound source also affects the efficiency of the cleaning process.
- Impact on Microbial Structures
- Bacterial Spores: The effectiveness of sonic waves against bacterial spores varies based on the chemical composition of the spores. Lipoidal substances within the microbes absorb ultrasonic frequencies, which influences the acoustic energy absorption and removal efficiency.
- Lipid Absorption: Changes in the lipid content of the microbial cells can alter the amount of acoustic energy absorbed, affecting the overall effectiveness of the cleaning process.
Applications and Practical Use
- Healthcare Facilities
- Instrument Cleaning: This method is commonly employed in healthcare settings to clean surgical and dental instruments. The ultrasonic cleaning process is performed before the final sterilization to ensure thorough removal of contaminants.
- Enhanced Cleaning
- Accessibility: The high-energy shock waves produced by cavitation can reach and clean even the most inaccessible surfaces, making it a valuable tool in maintaining hygiene and sterility in medical environments.
Method | Recommended Uses | Limitations |
Ultrasonics | Effective in decontaminating delicate cleaning instruments | Not effective alone, but as adjunct procedure enhances effectiveness of other methods |
G. Pressure (Pascalization)
Pascalization, also known as High-Pressure Processing (HPP), is a food preservation and sterilization technique that utilizes extremely high hydrostatic pressures to enhance the safety and longevity of food products. This method is characterized by its application of pressure in the range of hundreds of megapascals (MPa), effectively targeting specific microorganisms and inactivating enzymes without the need for high temperatures.
Mechanism of Action
- Pressure Application
- High Hydrostatic Pressure: Pascalization involves the application of pressures typically between 400 and 600 MPa. This immense pressure is applied uniformly in a liquid medium, which ensures that all surfaces of the food are subjected to the same force.
- Nonthermal Treatment: Since the process operates at room temperature, it avoids the thermal effects associated with traditional heat-based sterilization methods.
- Microbial and Enzymatic Inactivation
- Microorganism Death: The high pressure induces structural changes in microbial cells, leading to their inactivation. The pressure affects cell membranes, proteins, and other cellular components, resulting in the death of various microorganisms.
- Enzyme Inactivation: Enzymes responsible for food spoilage and degradation are also inactivated under high pressure, contributing to the preservation of the food’s quality and shelf life.
- Effects on Cellular Structures
- Protein Denaturation: At pressures between 400 and 600 MPa, proteins within microorganisms are denatured. This denaturation disrupts their functional structures, leading to cell death.
- Altered Cell Morphology: The morphological structure of microbial cells is altered under high pressure, impacting their integrity and functionality. Ribosomes, essential for protein synthesis, are also destroyed.
- Membrane and Nucleic Acid Changes
- Lipid-Protein Complexes: The lipid-protein complexes within cell membranes undergo changes, resulting in increased membrane fluidity. This alteration leads to leakage of nucleic acids and other cellular contents.
- Nucleic Acid Leakage: The disruption in membrane structure facilitates the leakage of nucleic acids, further compromising the viability of the microorganisms.
Limitations and Applications
- Effectiveness Against Spores
- Pressure Tolerance of Spores: Pascalization is less effective against bacterial spores, which are known for their resistance to high pressure. However, combining high pressure with heat treatments has proven effective in inactivating these resilient spores.
- Suitability for Food Products
- Acidic Foods: The process is particularly effective for acidic foods, such as yogurts and fruits. The low pH environments in these products limit the survival of pressure-tolerant spores.
- Solid and Liquid Foods: Pascalization works efficiently for both solid and liquid food products, making it a versatile method in food processing.
- Preservation of Food Quality
- Minimal Impact on Food Properties: The treatment generally preserves the shape, color, and nutrient content of most foods. Since it does not involve thermal processing, the flavor and texture of the food are also largely unaffected.
H. Sunlight (Solar Disinfection)
Solar disinfection is an effective method for purifying water by utilizing the natural energy of sunlight. This process is primarily employed to make drinking water safe by removing or inactivating microorganisms.
Mechanism of Action
- Sunlight Utilization
- UV-A Radiation: The process relies on ultraviolet A (UV-A) radiation, which has a wavelength range of 320 to 400 nanometers (nm). This part of the solar spectrum is instrumental in disinfection.
- Reactive Oxygen Species: UV-A radiation interacts with oxygen dissolved in the water, leading to the formation of highly reactive oxygen species, such as oxygen free radicals and hydrogen peroxide.
- Microbial Inactivation
- Pathogen Damage: The reactive oxygen species produced during solar disinfection damage the cellular structures of pathogens. This includes the disruption of metabolic processes and the destruction of essential bacterial cell components.
- Heat Effect: In addition to the chemical effects of UV-A radiation, the broader spectrum of solar energy, including infrared radiation, raises the temperature of the water. This thermal effect can further contribute to the inactivation of microorganisms.
Efficiency and Limitations
- Comparison to Radiation Sterilization
- Principle Similarity: Solar disinfection shares a similar principle with radiation sterilization, utilizing UV radiation to achieve microbial inactivation.
- Efficacy and Exposure Time: Despite its effectiveness, solar disinfection is generally less efficient compared to other methods. It requires extended periods of exposure to achieve adequate disinfection, which can limit its practical application in certain scenarios.
- Economic and Environmental Considerations
- Cost-Effectiveness: Solar disinfection is a cost-effective method, as it harnesses free sunlight and does not require expensive equipment or chemicals.
- Environmental Impact: This method is environmentally friendly, leveraging natural sunlight without generating harmful byproducts.
Practical Applications
- Drinking Water Purification
- Common Use: Solar disinfection is predominantly used for purifying drinking water, especially in regions where access to advanced water treatment facilities is limited.
- Simple Implementation: The process can be implemented using basic equipment such as transparent containers or specially designed solar disinfection units, making it accessible for a wide range of applications.
- Exposure Conditions
- Optimal Conditions: For effective solar disinfection, water should be exposed to sunlight in clear, shallow layers. Cloudy or turbid conditions can reduce the effectiveness of the process.
What are Thermal Death Time and Decimal Reduction Time?
- Thermal death time refers to the shortest period of time to kill a suspension of bacteria (or spores) at a prescribed temperature and under specific conditions.
- While Decimal reduction time is another unit of measurement of the destruction of microorganisms by heat.
- From the definition of these terms, it is clear that they express a time-temperature relationship to killing. In thermal death time, the temperature is selected as the fixed point and the time varied.
- Decimal reduction time is a modification of thermal death time which measures a 90 percent rather than 100 percent kill rate.
- Thermal-death-time data and decimal-reduction-time data are extremely important in many applications of microbiology.
- The canning industry, for example, carries out extensive studies on this subject to establish satisfactory processing temperatures for the preservation of canned foods.
Physical Methods of sterilization Cheat Sheet
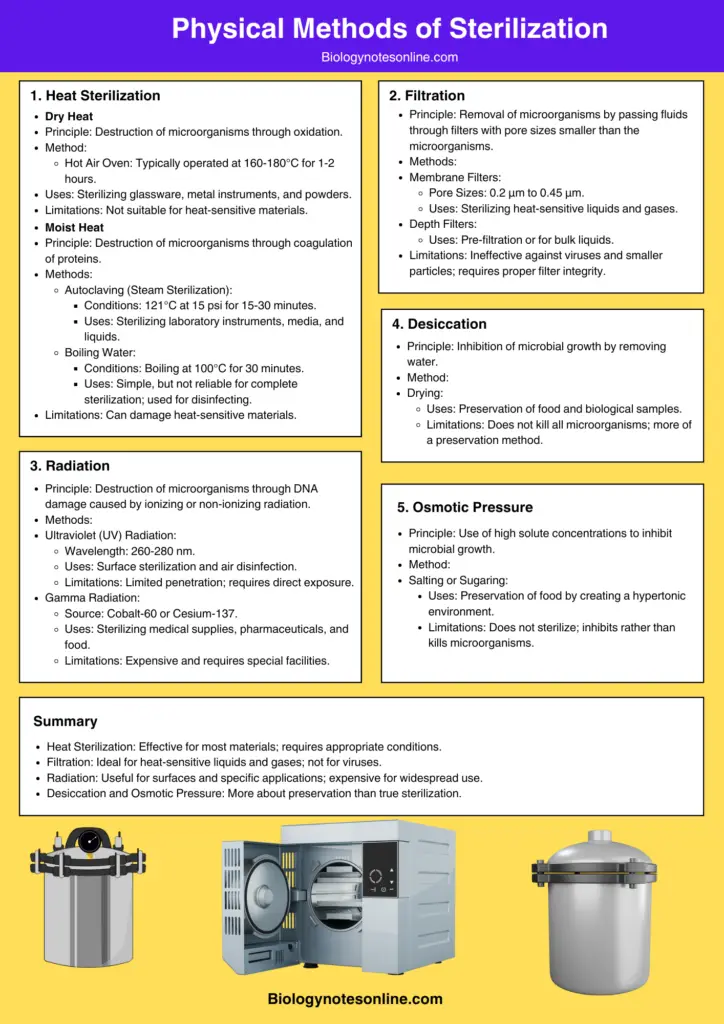
References
- Microbiology by Michael J. Pelczar, JR., E.C.S. Chan, Noel R. Krieg
- Prescott’s Microbiology
- Textbook of Microbiology and Immunology
- https://www.mvorganizing.org/what-are-the-disadvantages-of-pasteurization-of-milk/