- Nucleotides are comprised of three distinguishing elements: (1) a nitrogenous (nitrogen-containing) base, (2) a pentose, and (3) a phosphate.
- A nucleoside is the molecule without the phosphate group.
- The nitrogenous bases are derived from pyrimidine and purine, two parent molecules. The common nucleotides’ bases and pentoses are heterocyclic compounds.
- The base of a nucleotide is covalently attached to the 1′ carbon of the pentose via an N-B-glycosyl link (at N-1 for pyrimidines and N-9 for purines), and the phosphate is esterified to the 5′ carbon.
- As with O-glycosidic bond production, the N/-B-glycosyl link is created by removing water molecules (a hydroxyl group from the pentose and hydrogen from the base).
- Two primary purine bases, adenine (A) and guanine (G), and two major pyrimidines are present in both DNA and RNA.
- One of the pyrimidines in both DNA and RNA is cytosine (C), but the second major pyrimidine is different: thymine (T) in DNA and uracil (U) in RNA.
- Rarely do thymine and uracil occur in RNA and DNA, respectively.
What is Nucleotide?
- A nucleotide is a type of organic molecule made up of a nitrogenous base, a pentose sugar, and a phosphate.
- Polynucleotides, like DNA and RNA, are made up of a long chain of nucleotides with different nitrogenous bases.
- Nucleotides are needed for all metabolic and physiological processes to work.
- ATP (adenosine triphosphate) is the way that cells exchange energy.
- Nucleotides are used to make coenzymes and cofactors like NAD, NADP, FAD, coenzyme A, etc., which are needed for many metabolic processes.
- Nucleotides are made up of a base with nitrogen, a sugar with five carbons, and one or more phosphate groups.
- There are many different kinds of nucleotides in cells, and they are always switching between being free and being bound together.
- Nucleotides are the most important parts of many cellular processes, such as regulating the metabolism and storing and using genetic information.
- The small pools of free nucleotides make it easy to control the levels of nucleotides in cells. The only exception is adenosine-5′-triphosphate, which is found in higher amounts because it is the universal currency of energy.
- Inside of cells, ribonucleotides are made from simple building blocks or by recycling bases that have already been made.
- The amount of nucleotides and nucleic acids in a cell depends on how fast it grows. In mammals, the amount of nucleotides and nucleic acids as a percentage of cell weight ranges from about 1% in muscle cells to 15–40% in thymus gland cells and sperm cells.
- An oligonucleotide is a short piece of DNA or RNA. Oligonucleotides are usually made of polymers with 50 or fewer nucleotides. “Short” is a bit of a subjective term. A polynucleotide is a longer nucleic acid.
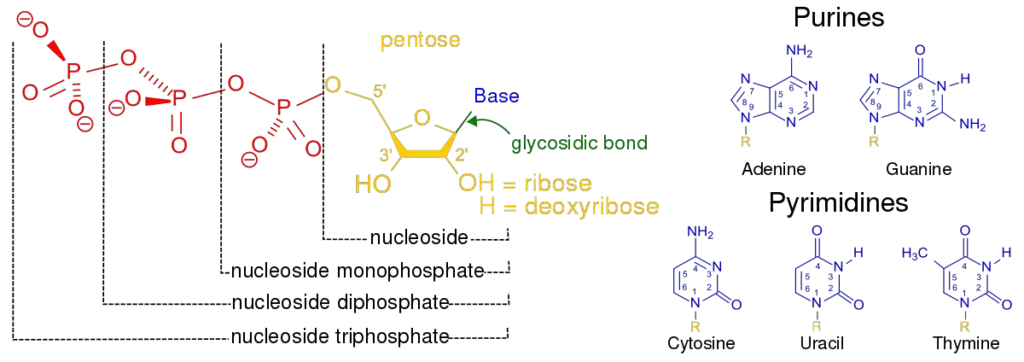
Nucleotide Structure
The structure of a nucleotide is simple, but the structure they can make when they come together is complicated. This molecule is made up of two strands that wrap around each other. Hydrogen bonds hold the structure together in the middle. Each nucleotide has a specific shape that makes this formation possible.
As has already been said, a nucleotide is mostly made of nucleobase, sugar, and phosphate. Nucleoside means “base plus sugar.” So, nucleotide is made up of a nucleoside and a phosphate.
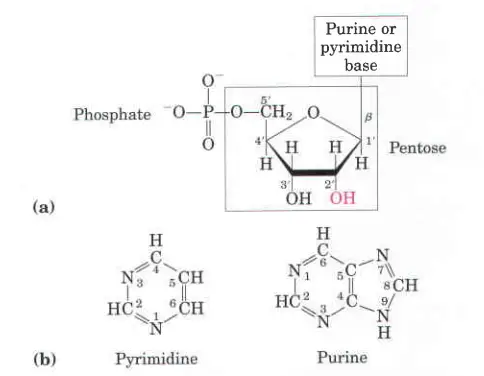
All nucleotides are made up of three main parts;
1. Nitrogenous base
- These nitrogen-containing ring compounds are made from purine or pyrimidine. They are also called heterocycles.
- In all nucleotides, the atoms in the purine and pyrimidine rings are in the same place and have the same number.
- Various chemical groups can bond to different parts of the ring in different places.
- Even though each base mostly has one structure, tautomeric forms do happen in small amounts.
- The nitrogenous base is the part of the nucleotide structure that is most important for carrying information.
- Because these molecules have different exposed functional groups, they can interact with each other in different ways.
- The idea arrangement is the one with the most hydrogen bonds between the different nucleotides. Because of the way nucleotides are built, only certain ones can interact with other ones.
- Both DNA and RNA have adenine (A) and guanine (G) as their two main purine bases, as well as two main pyrimidines.
- One of the pyrimidines in both DNA and RNA is cytosine (C), but the second major pyrimidine in DNA is thymine (T) and in RNA it is uracil (U).
- RNA and DNA don’t often have thymine or uracil.
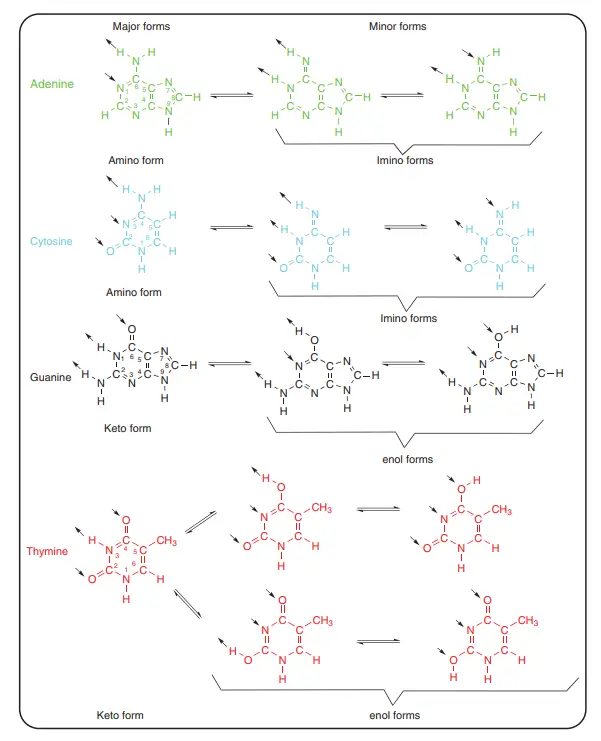
Types of Nitrogenous Base
There are present two types of base such as;
- Purine Base: The bases adenine and guanine are purines, which means they are made up of two rings.
- Pyrimidines base: Cytosine, thymine, and uracil are all pyrimidines, which means they are made up of a single ring.
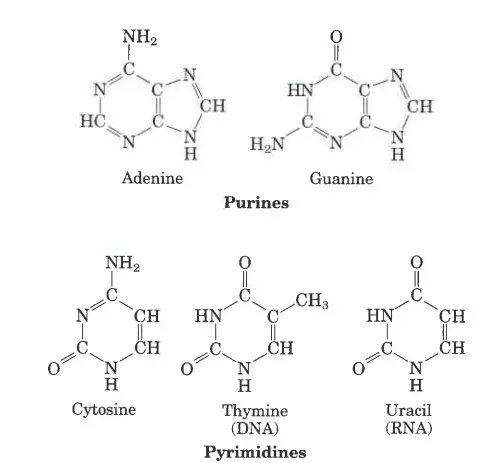
Examples of Nitrogenous Base
There are five nitrogenous bases: Adenine, Guanine, Thymine, Cytosine, and Uracil. They are compounds that have nitrogen in them and are a very important part of the genetic code. Here are the structures of the five nitrogenous bases and brief descriptions of what they do.
- Structure of Adenine: Adenine’s structure is made up of two nitrogen-containing rings. It is a purine derivative. In the nucleotide structure, it makes a double bond with thymine.
- Structure of Guanine: Again, Guanine is a purine derivative made up of two rings with nitrogen in them. In the nucleotide structure, it makes a triple bond with cytosine.
- Structure of thymine: Thymine is a type of pyrimidine that is made up of a single nitrogen-filled ring. 5-methyluracil is another name for it.
- Structure of cytosine: Like thymine, cytosine is a pyrimidine derivative. Like thymine, it has an aromatic ring with an amino and a keto group attached to it.
- Structure of Uracil: Uracil is also a pyrimidine derivative, but it can also be thought of as a form of thymine that has been stripped of its methyl group.
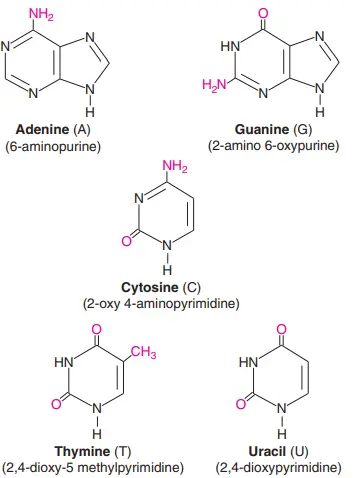
Nitrogen base | Representation | Complementary base in DNA | Chemical formula |
Adenine | A | Thymine | C5H5N5 |
Guanine | G | Cytosine | C5H5N5O |
Thymine | T | Adenine | C5H6N2O2 |
Cytosine | C | Guanine | C4H4N3O |
2. Sugar
- The sugar is the second part of the nucleotide.
- The base is linked to a sugar with five carbons. Usually, this sugar is ribose.
- Each carbon atom is given a number, and the number is followed by a prime mark so that it can be told apart from atoms in the base. For example, ribose has five carbon atoms, which are numbered from 1′ to 5′.
- The bond from the sugar’s C1′ to its base locks the sugars into a five-membered furanose ring.
- The sugar is always the same, no matter what the nucleotide is. DNA and RNA are what make the difference.
- In DNA, the sugar with 5 carbons is called deoxyribose, but in RNA, it is called ribose. This is how DNA and RNA get their full names. DNA is full name is deoxyribonucleic acid, and RNA is full name is ribonucleic acid.
- With its free oxygen, the sugar can form a bond with the phosphate group of the next molecule. The sugar-phosphate backbone is made from the bond that is made between them.
- The covalent bonds they make are much stronger than the hydrogen bonds between the two strands, so this structure makes the structure more rigid.
- When proteins need to read and change the DNA, they separate the strands and only read one side.
- When they die, the nucleotide bases that are opposite each other pull the strands of genetic material back together. The backbone of sugar and phosphate stays together the whole time.
Types of Sugars In Nucleotide
Nucleic acids have two kinds of pentoses or sugar such as;
1. deoxy-D-ribose
- The repeating parts of DNA called deoxyribonucleotides are made up of 2′-deoxy-D-ribose.
- There are four major deoxyribonucleotides (such as deoxyribonucleoside 5′-monophosphate) in the body.
- Deoxyadenosine,
- Deoxyguanosine,
- Deoxythymidine,
- Deoxycytidine.
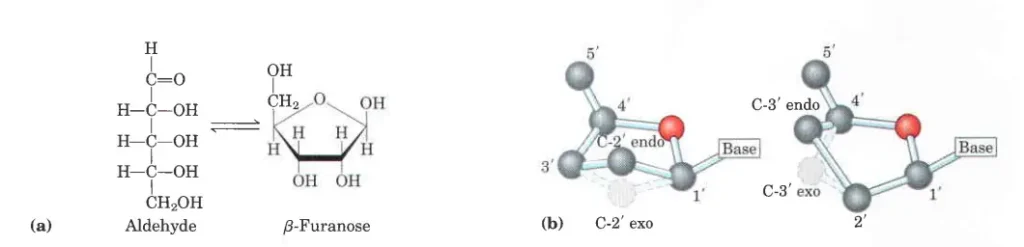
2. ribonucleotide
- RNA has o-ribose in its ribonucleotide units. Both kinds of pentoses are in their B-furanose (closed five-membered ring) form in nucleotides.
- The pentose ring is not flat; instead, it has a variety of shapes that are usually called “puckered.”
- There are four major ribonucleotides (ribonucleoside 5′-monophosphate) in the body. These are;
- Adenosine
- Guanosine
- Uridine
- Cytidine
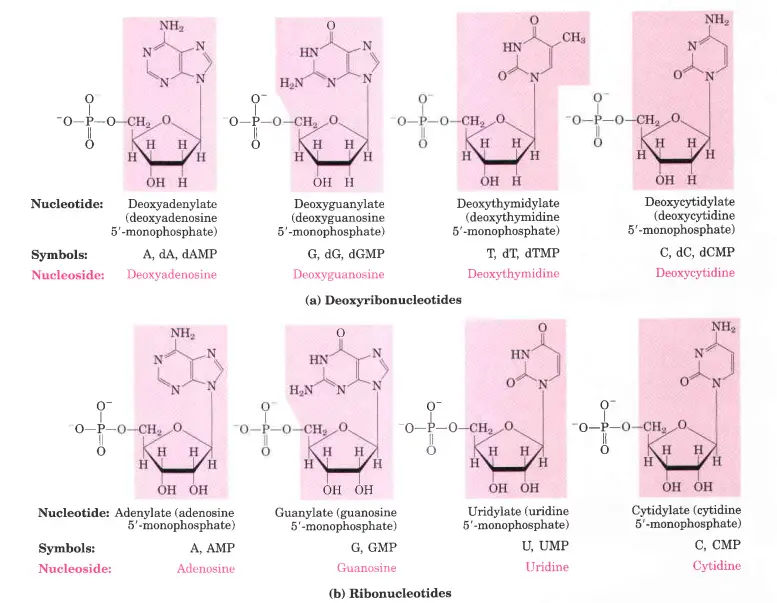
3 .Phosphate Group
- The phosphate group, which is the last part of a nucleotide’s structure, is probably something you know from ATP, another important molecule.
- Adenosine triphosphate, or ATP, is the energy molecule that most life on Earth uses to store energy and move it from one reaction to another.
- There are three phosphate groups in ATP. The bonds between them can store a lot of energy. In a nucleotide, the bonds that are made are called phosphodiester bonds because they are made between the phosphate group and the sugar molecule.
- During DNA replication, an enzyme called DNA polymerase brings together the right nucleotide bases and starts putting them in the right order along the chain it is reading.
- The job was finished by another protein called DNA ligase, which made the phosphodiester bond between the sugar molecule of one base and the phosphate group of the next.
- This makes the backbone of a new genetic molecule that can be passed on to the next generation.
- DNA and RNA hold all of the genetic information that cells need to work.
- Ester bonds connect the phosphate group to the sugar. In natural compounds, the hydroxyl at the C5′ position is the most common place where ester bonds form.
- Most of the time, one, two, or three phosphates are joined together to make mono-, di-, or triphosphates. (Note that molecules with two or three phosphates attached to different hydroxyls on the sugar are called “bis” or “tris.”
PO43- is the symbol for one phosphate group. The atom of phosphorus is in the middle. One oxygen atom is linked to both the fifth carbon in the sugar and the phosphorus atom. When phosphate groups link together to make chains, like in ATP (adenosine triphosphate), the link looks like O-P-O-P-O-P-O, with two extra oxygen atoms attached to each phosphorus, one on each side of the atom.
What are nucleosides?
- Nucleosides are glycosylamines that can be thought of as nucleotides without the phosphate group.
- A nucleoside is made up of just a nucleobase (also called a nitrogenous base) and a five-carbon sugar (ribose or 2′-deoxyribose), while a nucleotide is made up of a nucleobase, a five-carbon sugar, and one or more phosphate groups.
- In a nucleoside, the anomeric carbon is connected to the N9 of a purine or the N1 of a pyrimidine through a glycosidic bond.
- DNA and RNA are made up of parts called nucleotides.
- Nucleosides can be made from nucleotides from scratch, especially in the liver, but most of them come from eating and digesting nucleic acids. Nucleotidases break down nucleotides, like thymidine monophosphate, into nucleosides, like thymidine, and phosphate.
- The nucleosides are then broken down into nucleobases and ribose or deoxyribose by nucleosidases in the lumen of the digestive tract.
- Nucleotides can also be broken down into nitrogenous bases, ribose-1-phosphate, or deoxyribose-1-phosphate inside the cell.
Types of Nucleosides
Based on the types of nitrogenous bases they have, nucleoside derivatives can also be put into the following groups:
- Cytidine nucleotides: CTP, CDP, CMP and certain deoxy CDP derivatives of glucose, choline and ethanolamine
- Adenosine nucleotides: ATP, ADP, AMP, Cyclic AMP
- Uridine nucleotides: UDP
- Guanosine nucleotides: GTP, GDP, GMP, Cyclic GMP
- Miscellaneous : PAPS (active sulphate), SAM (active methionine), certain coenzymes like NAD+, FAD, FMN, Cobamide coenzyme, CoA
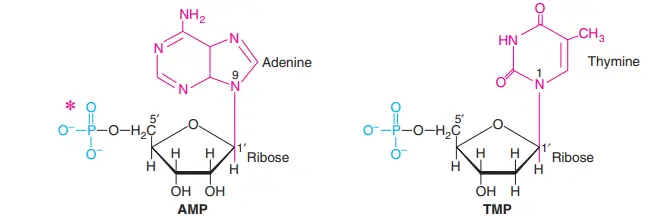
Uses
- Medicine: Several nucleoside analogues are used in medicine as antiviral or anticancer drugs. The viral polymerase adds these non-canonical bases to these compounds. In the cells, these compounds are turned on by being changed into nucleotides. Since it is hard for charged nucleotides to pass through cell membranes, they are given as nucleosides.
- Molecular biology: In molecular biology, there are many things that are like the sugar backbone. Due to the fact that RNA is not very stable and is easily broken down by water, several more stable nucleoside/nucleotide analogues that can bind to RNA are used. This is done by using a different type of sugar as the base. Locked nucleic acids (LNA), morpholinos, and peptide nucleic acids are some of these analogues (PNA).
- Sequencing: In sequencing, dideoxynucleotides are used. These nucleotides have the non-standard sugar dideoxyribose, which doesn’t have a 3′-hydroxyl group (which accepts the phosphate). DNA polymerases can’t tell the difference between it and a regular deoxyribonucleotide, so it can’t bond with the next base. This ends the chain.
List of nucleosides and corresponding nucleobases
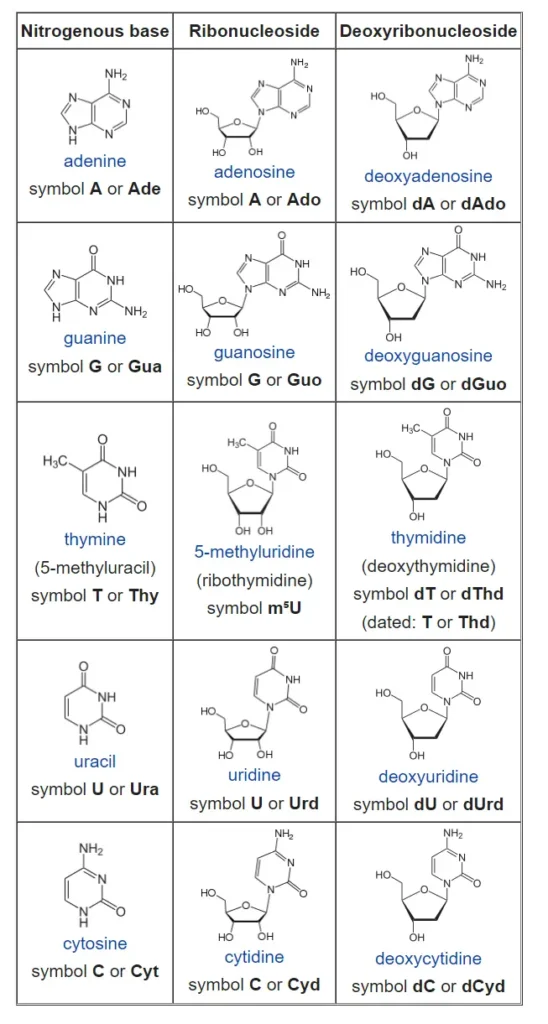
How do nucleotides and nucleosides differ?
Nucleoside = Nitrogenous base + Sugar
Adenosine, Guanosine, Thymidine, Cytidine, and Uridine are all names for nucleosides.
Nucleotide = Nucleoside + Phosphate
Nucleotides are named as Adenylic acid, Guanylic acid, Thymidylic acid, Cytidylic acid and Uridylic acid.
Nucleotides can also be called nucleoside mono, di, or triphosphate, depending on how many phosphate groups are attached to it. For example, Adenosine can be an AMP, an ADP, or an ATP (ATP).
Both DNA and RNA only have nucleotides in them.
ATP, cAMP, NAD+, NADP+, FAD, coenzyme A, etc. are all examples of nucleotides that are found in the body but are not part of DNA or RNA polynucleotide chains.
- Adenosine triphosphate (ATP): ATP is the cell’s way of paying for energy. ATP gives metabolic processes the energy they need to work. It also works as a coenzyme and is a building block for making DNA and RNA.
- Cyclic adenosine monophosphate (cAMP): cAMP is a second messenger that helps send signals. It is an important part of how carbs and fats are used by the body.
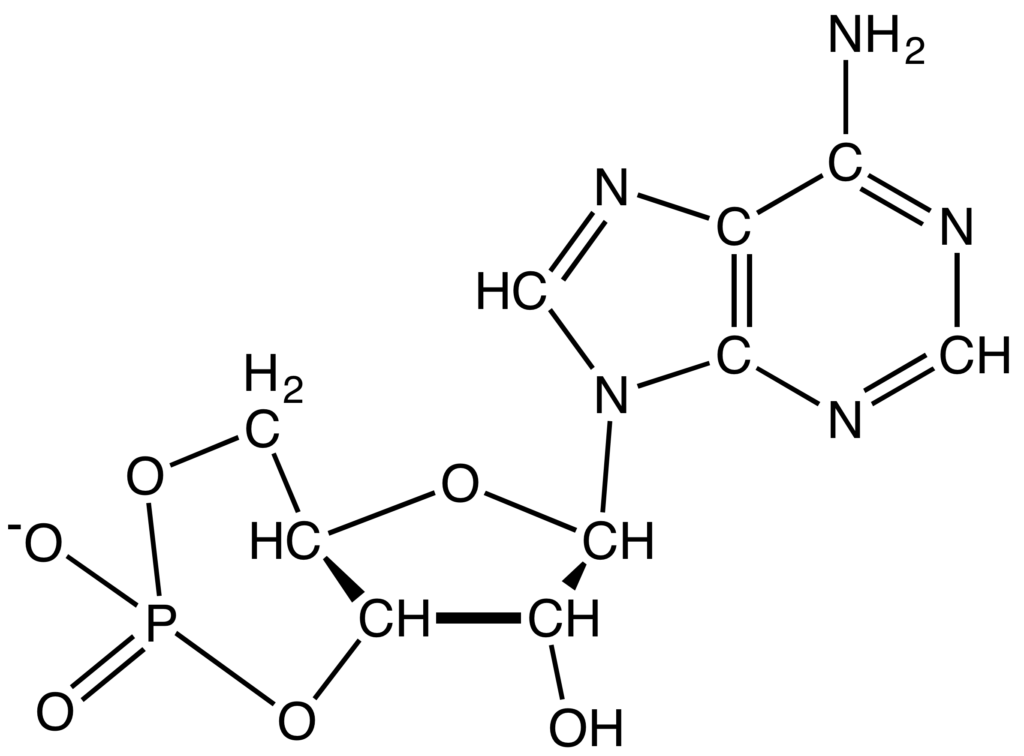
- Nicotinamide adenine dinucleotide (NAD): NAD is a dinucleotide. It is made up of two nucleotides that are connected by phosphate groups. One of the nucleotides has an adenine base, and the other has nicotinamide. They are important to the body’s metabolism and move electrons around. They come in two different forms: NAD+ and NADH. The Calvin cycle and other anabolic processes also use NADP+ (NADPH) as a cofactor.
- The FAD is an adenine dinucleotide, which is a coenzyme and a key part of many chemical reactions that enzymes take part in.
What are Dinucleotides and Oligonucleotides?
- Most of the time, 3′,5′-phosphodiester bonds hold nucleotides together in their polymeric forms. But RNA molecules with 2′,5′-phosphodiester bonds have been made chemically, and mammalian cells make oligoadenylates with a 2′,5′ linkage when they get infected by some viruses.
- Nucleic acids have a stable covalent structure, but they also take part in many chemical reactions inside the cell.
- Acid-catalyzed hydrolysis can easily break down both DNA and RNA. The glycosidic bonds in DNA are more easily broken than those in RNA, and purine nucleotides are more easily broken down than pyrimidine nucleotides.
- Because RNA has a 2′-OH group, these molecules can be broken down by water in alkaline solutions to make a mixture of 2′- and 3′-nucleotides.
- Also, a number of enzymes called nucleases have been found to break down nucleic acids (RNA or DNA) in specific ways.
- The order of the bases in each chain tells us about the primary structure of DNA and RNA polymers.
- But in polynucleotides, the different nucleotides don’t work on their own.
- Instead, they talk to each other by stacking their bases, and they are set up in a helical shape.
- Most of the time, these helices go in a right-handed direction, but certain sequences can use left-handed helices.
- There is a continuum of different structures in nucleic acids, but different secondary structures of DNA have been identified.
- The right-handed B-form is thought to be the most common form in cells. Other common forms are called A (a right-handed helix) and Z. (a left-handed helix).
- Also, the structure of DNA can be changed by its sequence because the two bases in a base pair can rotate in different ways in relation to each other and to other base pairs.
- The different, complicated shapes of nucleic acids are only possible because they can interact with water and its solutes in ways that can be changed.
- DNA has a much slower rate of metabolic turnover than RNA. This means that RNA is better at coordinating how genes are expressed and controlled.
- Due to its roles as mRNA, tRNA, and rRNA, it has been known for a long time that RNA is important for the transfer of information and the making of proteins.
- More recently, though, it has been found that smaller RNA molecules can control how genes are turned on and off.
- Even though most RNAs are made as single-stranded molecules, there is a lot of base-pairing between the strands and they have clear secondary and tertiary structures.
- Sizes of cellular RNA species range from about 20 nucleotides and up (Holbrook, 2005). But the double-stranded parts of RNA all have a helical structure that is usually right-handed and is similar to the A shape of DNA.
Properties of Nucleotides
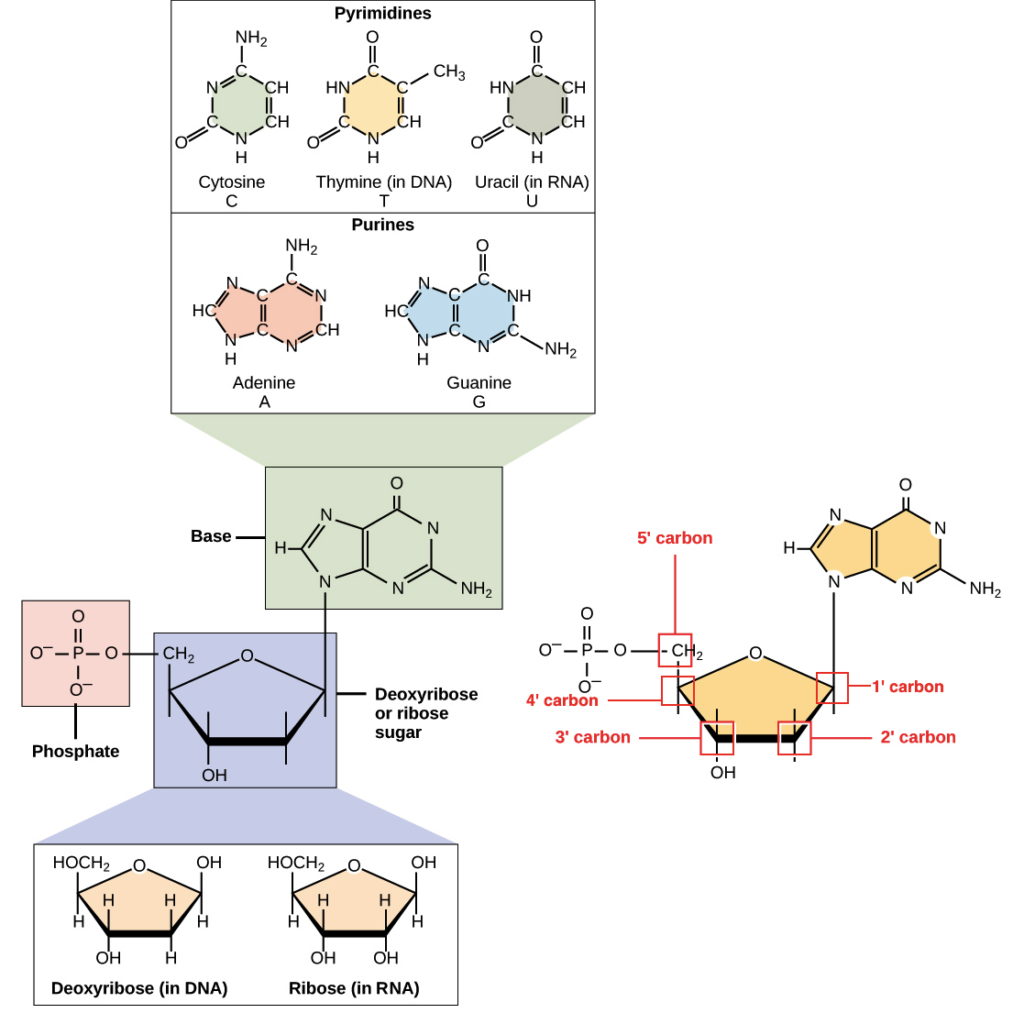
Properties of purine bases
- hardly dissolves in water
- Take in UV light at a wavelength of 260 nm (detection & quantitation of nucleotides)
- able to make hydrogen bonds.
- Aromatic bases with the numbers 1–9
- The pyrimidine ring and the imidazole ring join together to make the purine ring.
- The numbers go counterclockwise.
- Adenine: It’s made of 6-aminopurine.
- Guanine: Guanine is made up of two amino acids and six oxy purines. Can be present as lactam & lactim form
Properties of pyrimidine bases
- Body pH-soluble
- 260 nm UV light is also taken in.
- able to make hydrogen bonds.
- For pyrimidine, the base atoms that are aromatic are numbered from 1 to 6.
- The number of atoms or groups that are bonded to base atoms is the same as the number of the ring atom to which they are bonded.
- The chemical name for cytosine is 2-oxy-4-amino pyrimidine. Both lactam and lactim forms exist.
- The chemical name for thymine is 2,4-dioxy-5-methyl-pyrimidine. Only found in DNA
- The chemical name for uracil is 2,4 dioxy pyrimidine. Only found in RNA
Properties of Pentose Sugars
- A monosaccharide with five carbon atoms is called a pentose.
- With one oxygen atom attached to each carbon atom, ribose is the most common pentose.
- Deoxyribose sugar is made by taking away an oxygen atom from the sugar ribose.
- The carbohydrate’s aldehyde group reacts with nearby hydroxyl groups to make hemiacetals that are inside the molecule.
- The ring structure that is made is called a furanose because it is similar to a furan.
- The ring opens and closes on its own, letting the bond between the carbonyl group and the next-door carbon atom rotate. This gives the ring two different shapes ( and ). This is known as mutarotation.
Functions of Nucleotides
- Nucleic acid synthesis: DNA and RNA start out as nucleotides that have been turned on.
- Energy transfer: In biological systems, ATP is used as a universal currency for energy, and GTP is used in many systems that move or change the shape of macromolecules.
- Coenzymes: Some of the most important coenzymes are made up of adenine nucleotides.
- Metabolism: Nucleotide derivatives are activated intermediates in many biosynthetic reactions, and they also help to control metabolism by acting as mediators.
- Signaling processes: cyclic nucleotides and other non-enzymatic second messengers are often used in signal transduction processes (cAMP, cGMP, cyclic di-GMP). Cells can talk to their internal and external environments and to other cells through these processes, which change the way cells work and how genes are expressed.
Types of Nucleotides
Examples of nucleotides with only one phosphate group | Nucleotides with two phosphate groups | Nucleotides with three phosphate groups |
adenosine monophosphate (AMP) | adenosine diphosphate (ADP) | adenosine triphosphate (ATP) |
deoxtcytidine monophosphate (dCMP) | deoxycytidine diphosphate (dCDP) | (deoxy)thymidine triphosphate (dTTP) |
guanosine monophosphate (GMP) | (deoxy)thymidine diphosphate (dTDP) | deoxyadenosine triphosphate (dATP) |
(deoxy)thymidine monophosphate (dTMP) | deoxyguanosine diphosphate (dGDP) | deoxycytidine triphosphate (dCTP) |
cytidine monophosphate (CMP) | uridine diphosphate (UDP) | uridine triphosphate (UTP) |
deoxy guanosine monophosphate (dGMP) | deoxyadenosine diphosphate (dADP) | deoxyguanosine triphosphate (dGTP) |
deoxyadenosine monophosphate (dAMP) | cytidine diphosphate (CDP) | cytidine triphosphate (CTP) |
cyclic uridine monophosphate (cUMP) | guanosine diphosphate (GDP) | guanosine triphosphate (GTP) |
cyclic adenosine monophosphate (cAMP) | ||
cyclic cytidine monophosphate (cCMP) | ||
cyclic guanosine monophosphate (cGMP) | ||
uridine monophosphate (UMP) |
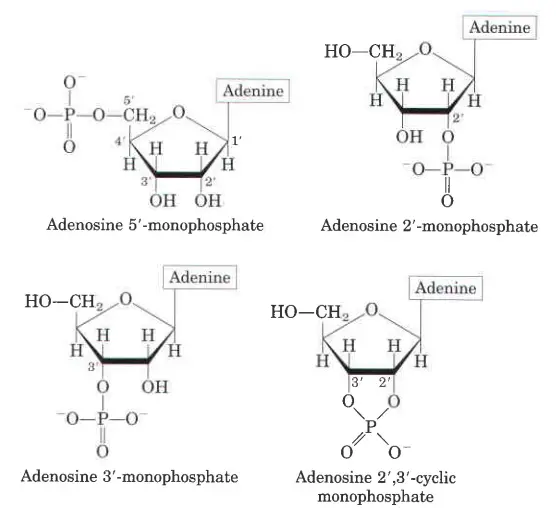
Characteristics of Nucleotides
- An organic compound called a nucleotide is made up of three parts: a nitrogenous base, a sugar with five carbons, and a phosphate group.
- It could be either ribose or deoxyribose for the sugar part. Ribose is the sugar part of nucleotides, which are the building blocks of RNA.
- DNA is made up of a sugar called deoxyribose. Each phosphate group links the sugar rings of two nucleotide monomers that are next to each other.
- Nucleic acid is held together by the phosphate groups and the sugar moles.
- In DNA, the two strands are going in different directions. This is done so that complementary pairs of bases can be made between nucleobases.
- In addition to the long chains that make up nucleic acids, nucleotides can also be found in a cyclic form.
- Cyclic nucleotides are made when the phosphate group is linked twice to the sugar moiety, specifically to the two hydroxyl groups of the sugar.
- Nucleotides do more than just make up parts of nucleic acids. They also carry energy.
- They carry chemical energy that is used by the cell to do different things. By far, the most common is Adenosine triphosphate (ATP).
Nucleotide Synthesis – De Novo Synthesis Pathway
- Nucleotides are made by the body using either the de novo synthesis pathway or the salvage pathway.
- The human liver is where the de novo synthesis of the basic nucleotides happens most of the time. In the process of making pyrimidine, the ring is made in a series of steps that start with making carbamoyl phosphate.
- First, carbamoyl phosphate is made from bicarbonate, glutamine, ATP (for phosphorylation), and a water molecule in a biochemical reaction.
- Carbamoyl phosphate synthetase II in the cytosol is the enzyme that speeds up the reaction.
- The enzyme aspartate transcarbamylase then changes the carbamoyl phosphate into carbamoyl aspartate.
- Then, the ring closes through a process called intramolecular condensation. The enzyme dihydroorotase turns carbamoyl phosphate into dihydroorotate.
- Lastly, dihydroorotate dehydrogenase, a membrane protein that is part of the inner membrane of the mitochondria, oxidises the dihydroorotate to turn it into orotate. Once the pyrimidine ring is made, a ribose phosphate called 5-phospho—D-ribosyl-1-pyrophosphate (PRPP) reacts with orotate to make orotidine-5-monophosphate (OMP).
- The enzyme OMP decarboxylase then takes OMP and breaks it down into uridine monophosphate (UMP).
- Down the biosynthetic pathway, kinases and the dephosphorylation of ATPs are used to make uridine diphosphate (UDP) and uridine triphosphate (UTP).
- With the help of the enzyme CTP synthetase, UTP can be turned into CTP through a process called amination.
- In the process of making purines, the nucleotide inosine monophosphate may be used (IMP). In turn, IMP is made from a ribose phosphate that already exists. This ribose phosphate is mostly made of the amino acids glycine, glutamine, and aspartic acid.
- Ribose 5-phosphate reacts with ATP to make 5-Phosphoribosyl-1-pyrophosphate (PRPP). PRRP is involved in the making of both purines and pyrimidines, as well as in the making of NAD and NADP and in the pathways that use them.
- When PRRP is changed into 5-phosphoribosyl amine, though, it is especially committed to purine biosynthesis (by having the pyrophosphate of PRRP replaced by the amide group of glutamine).
- Then, either adenosine monophosphate (AMP) or guanosine monophosphate is made from IMP (GMP).
Purine, Pyrimidine And Nucleotide Analogs
It is possible to change the heterocyclic ring or the sugar moiety to make purines, pyrimidines, nucleosides, and nucleotides that are similar but not the same. Some of the synthetic analogues are very useful in clinical medicine. Figure 1 shows the shapes of some analogues of purines and pyrimidines.
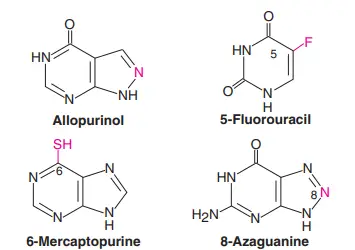
Here are some of the ways that analogues are used in pharmacology;
- Allopurinol: Allopurinol is used to treat gout and high levels of uric acid in the blood.
- 5-fluorouracil: 5-fluorouracil is used to treat cancer, along with 6-mercaptopurine, 8-azaguanine, 3-deoxyuridine, 5- or 6-azauridine, 5- or 6-azacytidine, and 5-idouracil. These chemicals get into the DNA and stop cells from making more copies of themselves.
- Azathioprine: This drug, which breaks down into 6-mercaptopurine, is used to stop the immune system from rejecting a transplant.
- Arabinosyladenine: Arabinosyladenine is used to treat viral encephalitis and other diseases of the nervous system.
- Arabinosylcytosine: Arabinosylcytosine is used to treat cancer because it stops DNA from copying itself.
- Zidovudine: Both zidovudine or AZT (3-azido-2c,3c-dideoxythymidine) and didanosine (dideoxyinosine), which are used to treat AIDS, are sugar-modified synthetic nucleotide analogues.
Degradation of Nucleotide
Purines like guanine and adenine can be broken down in the following ways:
In the case of GMP, the compound is first broken down with water and then changed into guanosine. The second one is then cut to make free guanine.
- Guanine (via guanase) » xanthine (via xanthine oxidase) » uric acid
- Adenosine »» inosine (via purine nucleoside phosphorylase) » hypoxanthine (via xanthine oxidase) » xanthine (via xanthine oxidase) » uric acid
Uric acid is created when purines are broken down. Uric acid is released into the bloodstream by the liver and other tissues in humans. It then makes its way to the kidneys. The body then gets rid of it through urine.
Purines that are broken down can be saved and used again in the following ways:
- The enzyme adenine phosphoribosyltransferase (APRT) turns adenine into adenylate so that it can be used again.
- The enzyme hypoxanthine-guanine phosphoribosyltransferase (HGPRT) gets rid of guanine and hypoxanthine by making guanylate or IMP from them.
A salvage pathway can be used to recycle pyrimidines that have been broken down. (Ref. 1) After RNA and DNA break down, the nucleobases can be used again. Here are some ways to get rid of pyrimidine:
- By deamination, cytosine is changed into uracil. Uridine is made when uracil reacts with ribose-1-phosphate. This is done by the enzyme uridine phosphorylase. Uridine is changed into uridine monophosphate by the enzyme nucleoside kinase (UMP).
- With the help of the enzyme thymidine phosphorylase and a reaction with deoxyribose-1-phosphate, thymine can be changed into thymidine. The enzyme nucleoside kinase then changes the thymidine into the thymidine monophosphate. Thymidine kinase is an enzyme of the pyrimidine salvage pathway that helps turn thymidine into thymidine monophosphate.
What is the Biological importance of Nucleotides?
- DNA and RNA are made of it. They are the building blocks of nucleic acids and transport activated metabolites during the biosynthesis process.
- Using chemicals to store energy needed for the fast-growing stages of DNA replication and RNA transcription
- Gives cells energy and does other metabolic tasks. Needed for chemical bonds in how cells respond to hormones and other stimuli from outside the cell.
- Serve as parts of the structures of enzyme cofactors and other intermediates in the metabolic process.
What is ATP?
- Adenosine triphosphate (ATP) is the energy-carrying molecule that gives living cells the power to do many things.
- ATP is also a nucleic acid, so it has a similar structure to the nucleotides that make up DNA and RNA.
- It is a nucleotide that has been phosphated.
- The nucleoside adenosine can be linked to one, two, or three phosphate groups.
- Adenosine monophosphate is made up of one phosphate group (AMP)
- Adenosine diphosphate is made up of two phosphate groups (ADP)
- Adenosine triphosphate is made up of three phosphate groups (ATP)
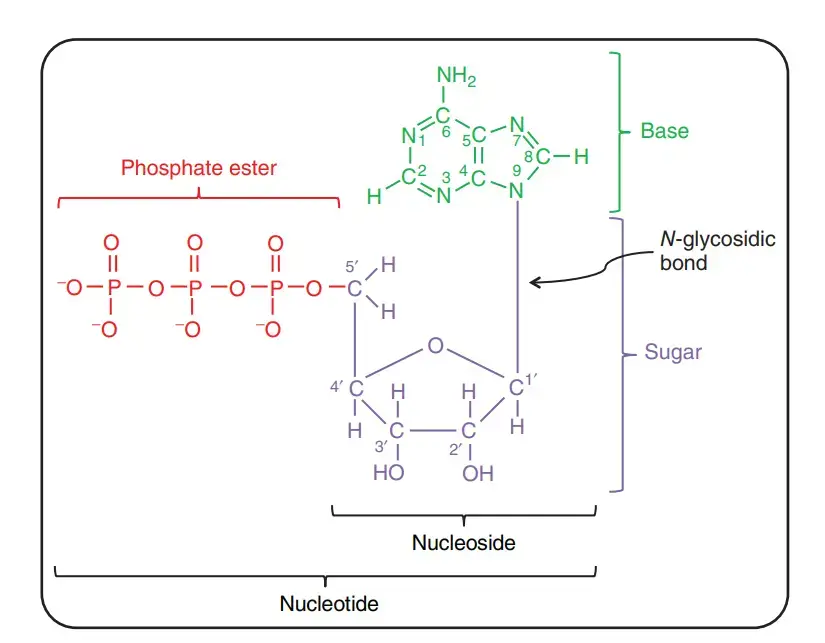
What are minor purines and pyrimidine bases?
- Even though purines and pyrimidines are the most common bases in nucleotides, both DNA and RNA also have some minor bases.
- Most of these are methylated forms of the major bases in DNA. In viral DNA, some bases may be hydroxymethylated or glucosylated. Most of the time, changed or unusual bases in DNA molecules help control or protect the genetic information.
- There are also many different kinds of minor bases in RNAs, especially in tRNAs.
- It can be hard to keep track of what the names of the minor bases are. Like the major bases, many of them have common names, like hypoxanthine.
- When an atom in a purine or pyrimidine nng is changed, it is common (and what is done here) to just write the number of the change to show where it is in the ring. For example, 5-methylcytosine, 7-methylguanine, and 5-hydroxymethylcytosine all show where the change is in the ring.
- The element (N, C, or O) to which the substituent is attached is not known. When the substituted atom is exocyclic, which means it is not part of the ring structure, the convention changes. In this case, the type of atom is named, and the position in the ring where it is attached is shown with a superscript.
- On C-6 of adenine, the amino nitrogen is written as N6. On C-6 and C-2 of guanine, the carbonyl oxygen and amino nitrogen are written as 06 and AP, respectively.
- N6-methyladenosine and M-methylguanosine are two examples of this type of name.
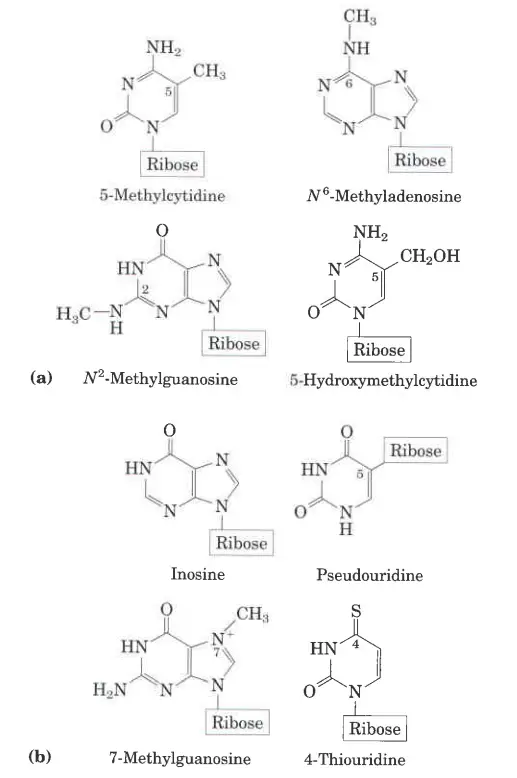
How Phosphodiester Bonds Link Successive Nucleotides in Nucleic Acids?
- Both DNA and RNA are held together by “bridges” made of phosphate groups. The 5′-phosphate group of one nucleotide is joined to the 3′-hydroxyl group of the next nucleotide to make a phosphodiester linkage.
- So, the covalent backbones of nucleic acids are made up of alternating phosphate and pentose residues, and the nitrogenous bases can be thought of as side groups that are attached to the backbone at regular intervals.
- Both DNA and RNA have backbones that like water. The sugar residues’ hydroxyl groups make hydrogen bonds with water.
- With a pK near 0, the phosphate groups are completely ionised and negatively charged at pH 7. The negative charges are usually neutralised by ionic interactions with positive charges on proteins, metal ions, and polyamines.
- In DNA and RNA, all of the phosphodiester linkages are in the same place along the chain. This gives each linear nucleic acid strand a certain polarity and different 5′ and 3′ ends.
- By definition, there is no nucleotide at the 5′ end, and there is no nucleotide at the 3′ end.
- On one or both ends, there may be other groups, most often one or more phosphates.
- The 5′ to 3′ orientation of a nucleic acid strand refers to the ends of the strand, not the way the phosphodiester bonds are arranged. Linking the nucleotides that make it up.
- The phosphodiester bonds in the covalent backbone of DNA and RNA are slowly broken down by water without the help of enzymes.
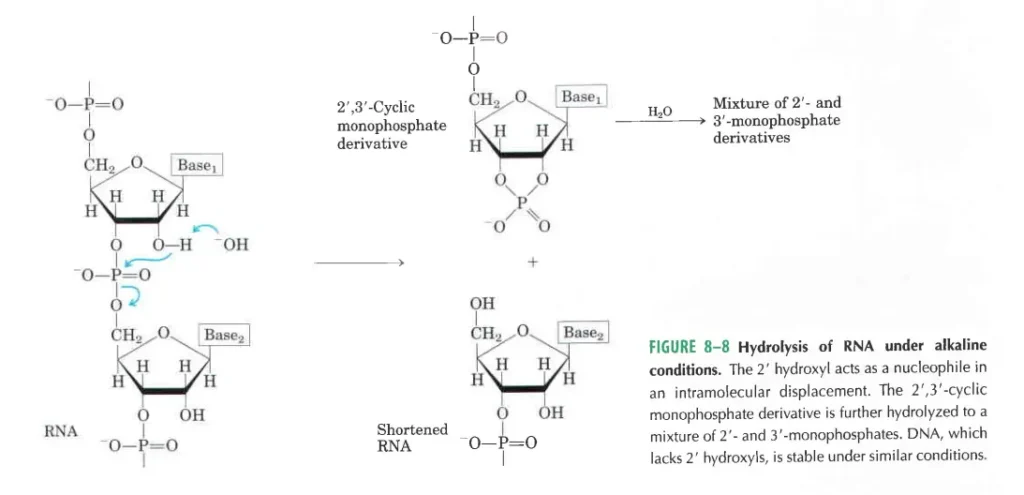
- In an alkaline test tube, RNA breaks down quickly, but DNA does not. This is because RNA’s 2′-hydroxyl groups are directly involved in the process, while DNA’s are not. When alkali reacts with RNA, the first things that come out are cyclic 2′,3′-monophosphate nucleotides. These quickly break down into a mix of 2′- and 3′-nucleoside monophosphate.
- Nucleic acids’ nucleotide sequences can be shown in a diagram, like the one below, which shows a piece of DNA with five nucleotide units.
- The phosphate groups are shown by (P), and each deoxyribose from C-1 at the top to C-5 at the bottom is shown by a vertical line (but keep in mind that the sugar is always in its closed-ring B-furanose form in nucleic acids).

- The lines that connect nucleotides are drawn diagonally from the middle (C-3,) of the deoxyribose of one nucleotide to the bottom (C-5′) of the next. These lines go through the point (P).
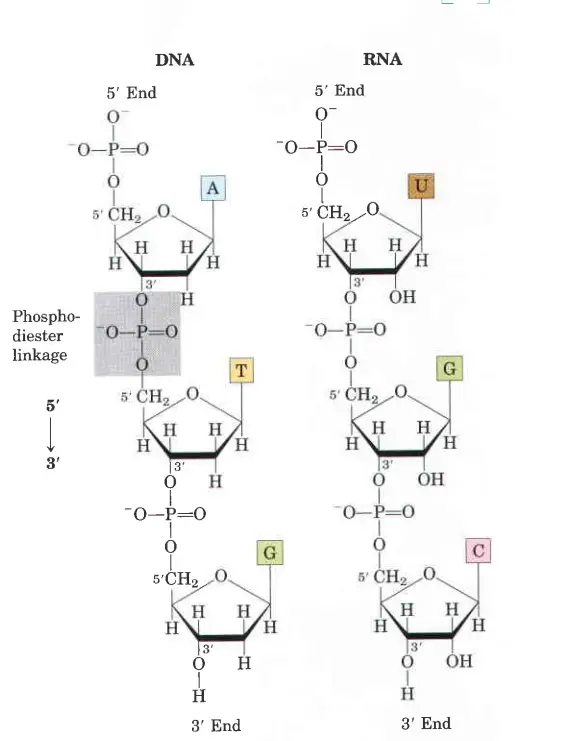
Conformational features
- The molecular shapes and conformational properties of a nucleotide’s different parts are inextricably linked.
- So, the shape of individual atoms must be thought of in the context of the whole molecule. Overall, the interactions between some of the atoms that are not bonded together make nucleotides have shapes that are not too big.
- Whether they are part of a nucleotide or stand alone, pyrimidine and purine bases can take on many of the same shapes.
- Most of the time, the bases’ heterocycles are flat. In theory, there are several tautomeric forms, but the keto and amino forms are the most common.
- Usually, the enol and imino forms don’t make up more than 0.1% of the whole, but this number can change a lot if modified bases are taken into account or if base pairs are made.
- A series of torsion angles, one for each bond, gives exact information about how a nucleotide is shaped.
- Each torsion angle shows the correct geometric relationship between four atoms in order, such as A–B–C–D. The angle is the distance between the projected bonds A–B and C–D when viewed along the central bond B–C.
- Below Figure and Table, you can see what the torsion angles of nucleotides mean.
- The position of the first and last atoms (A and D) in relation to the bond angle determines whether a torsion angle has a positive or negative value. If the smallest change needed for the front atom to cover the back atom is in a clockwise direction, the torsion angle has a positive value. If the smallest change needed is in the opposite direction, the torsion angle has a negative value.
- As is the case with most molecules that can rotate around a single bond, steric constraints tend to favour certain conformations of nucleotides.
- The Klyne–Prelog nomenclature, which was made for spectroscopists, is often used to describe ranges of torsion angle. Those of about 0° are called syn, and those of about 180° are called anti.
- (But keep in mind that syn and anti mean different things when it comes to the glycosidic bonds of nucleosides) (Figure c).
- Angles between 30° and 150° and between 210° and 330° are called clinal angles. Angles between 0° and 180° are called periplanar angles.
- Since many of the torsion angles of nucleotides depend on each other, the approximate shapes of nucleotides can be described by only four parameters: the sugar pucker, the conformation of the bond between the base and sugar (N-glycosidic bond), the orientation of C4′–C5′, and the shape of the phosphate ester bonds.
- In nucleosides, the five-membered furanose ring systems are twisted out of plane to reduce the number of unbonding interactions between their substituents that aren’t good.
- This “puckering” takes the form of an envelope (E) when four atoms are in a plane or a twist (T, also known as a “half-chair”) when two atoms next to each other are moved to opposite sides of a plane through the other three atoms (Figure b).
- C2′ and C3′ are the atoms that most often move away from the plane of the other atoms in a sugar ring.
- Its conformation is called endo if the carbon atom is above the sugar ring, toward the base and C5′, and exo if it is below the sugar ring. This method only gives a rough description of how sugar puckers. If a more precise description is needed (for example, for intermediate values), the conformation of the sugar ring can also be described using the idea of pseudorotation around the torsion angle 𝜈2 (i.e. C1′–C2′–C3′–C4′).
- When this angle is at its most positive (C2′-exo–C3′-endo is symmetrical), this is called a standard conformation (P=0).
- P can take on values between 0 and 360, and it is often shown as a circle with 0/360 at the top. Conformations in the upper, or northern, half of the circle (P=0±90∘)are labelled N, and those in the southern half (P=180±90∘) are labelled S.
- By using the idea of pseudorotation, this method can also be used to define sugars with conformation, since sugars with C2′-endo and C3′-endo are found in the south (S) and north (N) cycles, respectively.
- Most of the time, observed conformations don’t match these strict definitions, because changing between the possible conformations is easy and happens quickly. Also, the atoms that make up four-atom planes don’t always line up perfectly, and perfect envelope forms are hard to find.
- So, most conformations are twist forms, and the furanose ring’s conformation is defined by which atom moves the most (Figure b): the conformation is called C2′-endo if the endo displacement of C2′ is greater (has more puckering) than the exo displacement of C3′ (has less puckering).
- An abbreviated form of notation uses superscripts for endo atoms and subscripts for exo atoms to describe all parts of the sugar conformation. These notations come before or after the letter E or T to indicate major or minor puckering, respectively.
- For example, C2′-endo (with minor C3′-exo)=2T3 and C3′-endo (with minor C2′-exo)=3T2 are the abbreviations for the conformations shown in Figure b.
- A C2′-exo envelope is also written as 2E. The plane of the base is almost parallel to the plane of the sugar, and it cuts the angle formed by the atoms O4′-C1′-C2′ in half.
- This lets the bases take one of two main positions based on the angle 𝜒 of the glycosidic linkage: the syn conformer has the larger O2 (pyrimidine) or N3 (purine) atom above the sugar ring, while the anti conformer has the smaller H6 (pyrimidine) or H8 (purine) atom in that spot (Figure 3c).
- The exocyclic C4′–C5′ bond can turn, which lets O5′ and the 5′-phosphate move around with respect to the sugar.
- The most common rotamers of this bond (𝛾) are three shapes where all the substituents are in different places (see Figure d).
- Phosphate diesters are tetrahedral at the phosphorus, and the C5′–O5′ bond is usually in an antiperiplanar (ap) shape.
- In the same way, the C3′–O3′ bond is in the antiperiplanar to anticlinal (ac) sector (Figure d).
- In both the monomeric and polymeric states, nucleotides have these main features of their shape. But polymers have more factors to consider, such as their helical symmetry and base pairing.
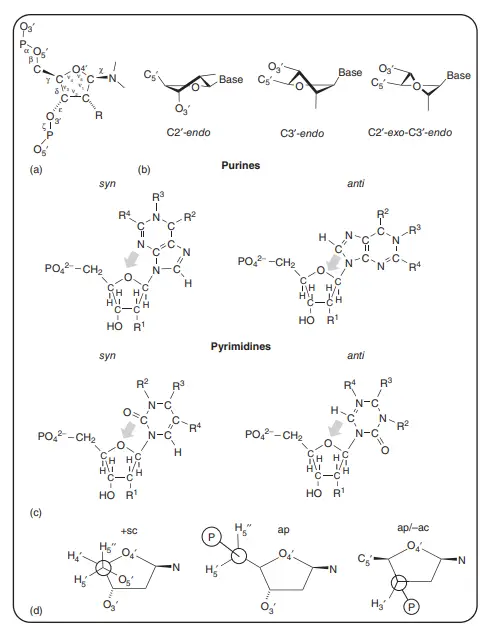
About Above Image: Nucleotides can take different shapes. (a) The IUPAC notation for the torsion angle of a polynucleotide. A common way to show the torsion angles in relation to the number of atoms in the nucleotide. (b) A picture of how furanose sugar makes your mouth pucker. Three twist (T) forms are shown in which three ring atoms (C4′, O4′, and C1′) are flat and the other two (C2′ and C3′) are on opposite sides of this plane. Any purine or pyrimidine base is called a “base.” (c) The anti and syn conformations of a purine or pyrimidine base’s glycosidic bonds. The arrow shows which base atoms are above the sugar ring furanose. R1 is H or OH, R2 is NH2 or O, R3 is H or a double bond to C6′, and R4 is H or NH2. For pyrimidine nucleotides, R1 is H or OH, R2 is H or a double bond to C4, R3 is NH2 or O, and R4 is H or CH3. (d) The best shapes for nucleotides at C4′–C5′ and C3′–O3′. In the structures, the C4′–C5′ bond has both synclinal (sc) and antiperiplanar (ap) rotamers, and the C3′–O3′ bond has the typical antiperiplanar/anticlinal (ap/ac) shape.
What do you mean by Free Nucleotides?
Diphosphate and monophosphate nucleotides can’t join together to make nucleic acid. Even so, these nucleotides are still important for how cells work.
Nucleotides can act as co-enzymes. An enzyme is a substance that is made by living things and acts as a catalyst to make a certain biochemical reaction happen. When bound to an enzyme, they can help speed up the chemical process.
What the nucleotide bonds to is one of the things that affects how the co-enzyme works. ATP, in particular, is often used as a coenzyme and is thought to be the main form of energy in living cells. Since ATP is so stable, it stays in a cell until it’s time to be used. When it’s time, it releases energy to start a chemical reaction.
Nucleotides are also important for the way cells work. This is a process that takes place in cells. Chemical reactions in the nucleotide cause the cells to break down.
This process is very important for RNA and DNA because it happens all the time in our cells, so it’s very important that it works right. If you don’t, it can lead to a number of diseases.
This reaction starts in the nucleotide, and the breakdown of the cell starts. When this happens to RNA or DNA, parts of the nucleotide can sometimes be used to make new nucleotides.

Which nucleotides are present in RNA?
RNA is made up of the following nucleotides:
- ATP: ATP stands for a nucleotide called adenosine triphosphate. It is made of ribose sugar, an adenine base, and three phosphate groups.
- CTP: Cytidine triphosphate (CTP) is a nucleotide made of ribose sugar, a cytosine base, and three phosphate groups.
- GTP : GTP stands for guanosine triphosphate, which is a nucleotide made of ribose sugar, a guanine base, and three phosphate groups.
- UTP : UTP is a nucleotide made of ribose sugar, a uracil base, and three phosphate groups.
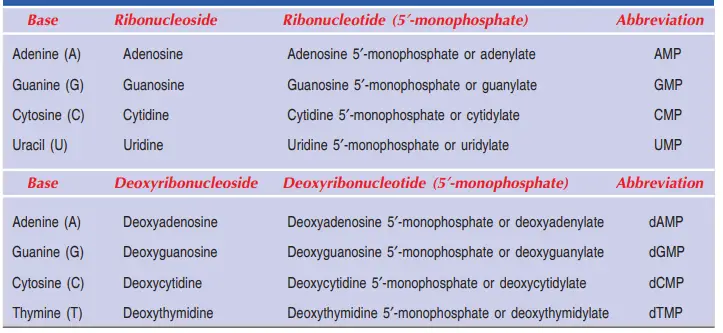
Which nucleotides are present in DNA?
Here are the DNA triphosphates that make up our nucleic bases:
- dATP: dATP stands for deoxyadenosine triphosphate, which is a nucleotide made up of three phosphate groups, an adenine base, and a deoxyribose sugar.
- dCTP: dCTP stands for deoxycytidine triphosphate, which is a nucleotide made of deoxyribose sugar, a cytosine base, and three phosphate groups.
- dTTP: dTTP stands for deoxythymidine triphosphate, which is a nucleotide made up of three phosphate groups, a thymine base, and a deoxyribose sugar.
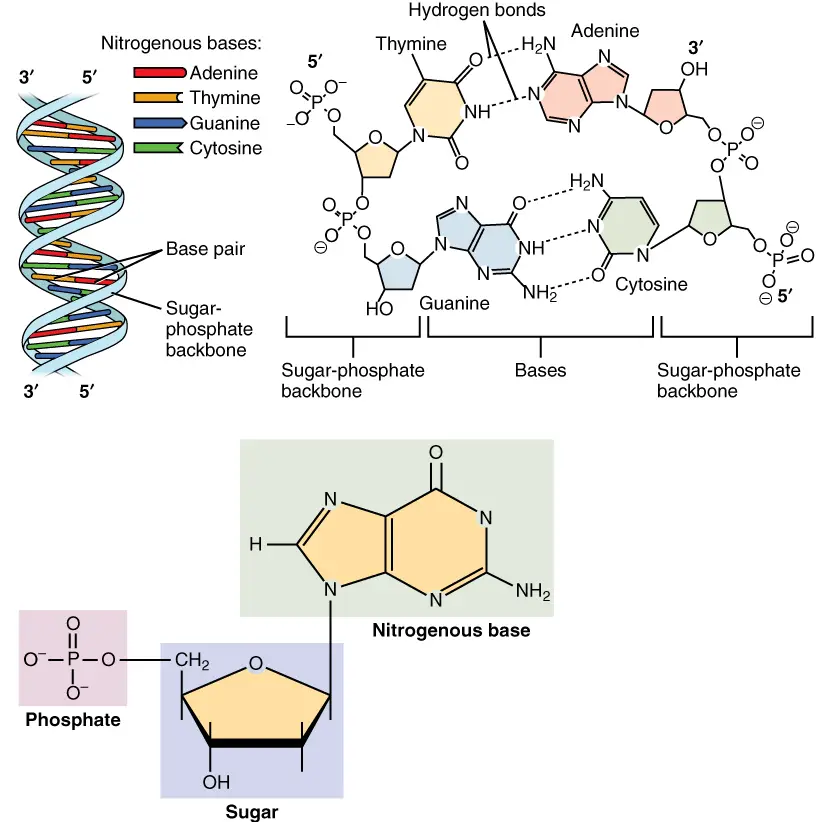
The binding of nucleotide components
The purine ring has atoms numbered from 1 to 9, and the pyrimidine ring has atoms numbered from 1 to 6. For differentiation, the carbons of sugars are shown with a prime (c) that goes with it. So, the carbons in pentose are 1c to 5c.
B-N-glycosidic bonds hold the pentoses to the nitrogenous bases. In a purine nucleoside, a covalent bond is made between the N9 of a purine ring and the C1(1′) of a pentose sugar. The glycosidic link is between the N1 of a pyrimidine and the C’1 of a pentose in pyrimidine nucleosides.
When phosphates are added to the hydroxyl groups of adenosine, 5′- or 3′-monophosphates are made. Most of the time, 5′-Hydroxyl is esterified, so 5′ is usually left out when writing the names of nucleotides. This means that AMP stands for adenosine 5c-monophosphate.
Adenosine 3c-monophosphate, on the other hand, is written as 3′-AMP. The structures of two nucleotides, AMP and TMP, were looked at.
You Need to know about Nucleotide
- Nucleosides have structures that are very similar to nucleotides, but they only have the base linked to a sugar by an N-glycosidic bond, which is also called a -glycosidic linkage.
- Since this is the case, a nucleotide can also be called a nucleoside phosphate (IUPAC-IUB, 1970, 1983).
- Nucleotides also exist in polymeric forms called nucleic acids. There are two closely related types of nucleic acids: ribonucleic acid (RNA) and deoxyribonucleic acid (DNA) (DNA).
- Because the 3′-OH of one nucleotide is linked to the 5′-phosphate of the next nucleotide with a phosphodiester linkage, the sequence of these polymeric forms has a direction.
- So, the molecule has a 5′-phosphate at one end and a 3′-OH at the other. Sequences are usually written with the nucleotide with the 5′-phosphate on the left.
- Most of the time, the deoxy forms of nucleotides and nucleosides are called out.
- At first, people thought that thymine bases only existed in DNA, and the terms thymidine, thymidine monophosphate, and thymidylate were used to refer to the deoxy form.
- But ribonucleotides with thymine as the base do exist in transfer RNA, and they have been made in the lab.
- At the moment, the terms thymidine and deoxythymidine are used interchangeably. For ribonucleotides of thymine, the prefix ribo- should be used.
- Ribothymidine should always be called that so there is no confusion. Most of the time, short forms are used to talk about nucleotides. For example, molecules with one, two, or three phosphates attached to adenosine are called AMP (adenosine monophosphate), ADP (adenosine diphosphate), and ATP (adenosine triphosphate). Their deoxy versions are called dAMP, dADP, and dATP.
- Nucleotides that don’t have a base written next to them are written as NMP, NDP, or NTP.
- There are a number of short names for polynucleotides, and most of them use a single capital letter to show the different bases of the nucleotides.
- Most of the time, the only way to tell the difference between deoxyribonucleotides and ribonucleotides is to say that the sequence is about RNA or DNA.
- Some abbreviations need to show the phosphates in the polynucleotide. For example, pApGpTpC or 5′ pApGpTpC3′-OH do this.
- For nucleic acids with repetitive sequences, there is a special way to name them. For example, a homopolymer made from deoxyadenylate is called poly(deoxyadenylate) or poly(dA), while heteropolymers with alternating sequences are called poly(deoxyadenylate-deoxythymidylate) or poly (dA-dT).
- Note that the name of a similar molecule with dA and dT spread randomly along a polymeric chain is the same, but a comma is used instead of the hyphen to make poly-dA-dT. (dA,dT).
- Hydrogen bonds can connect the bases of some nucleotides to the bases of other nucleotides. Base pairing is the name for these kinds of interactions between nucleic acid molecules that don’t involve bonds.
- Most of the time, G pairs with C and A pairs with T or U in DNA (in RNA). So, two nucleic acid polymers with complementary sequences can pair up in a very stable way. This pairing is what gives genetic material its double-stranded structure.
- Base pairing between two different strands usually happens in a way that makes the strands have opposite polarities.
- When talking about double-stranded nucleic acids, the sequence of only one strand is often given. This is because the sequence of the complementary strand is set by the rules of base pairing.
Mind map Image

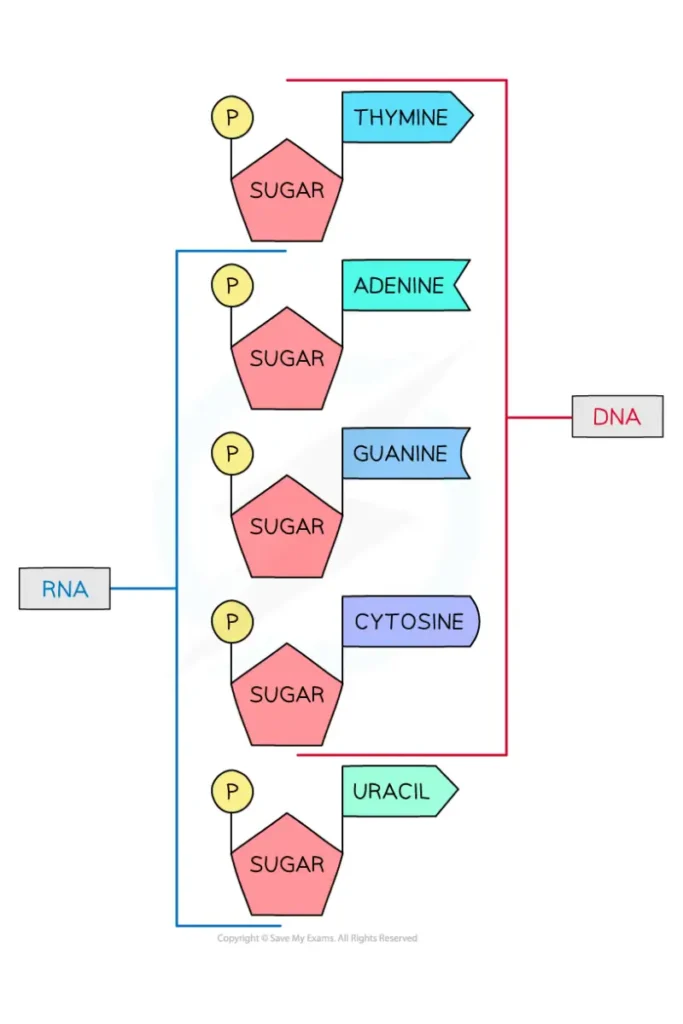
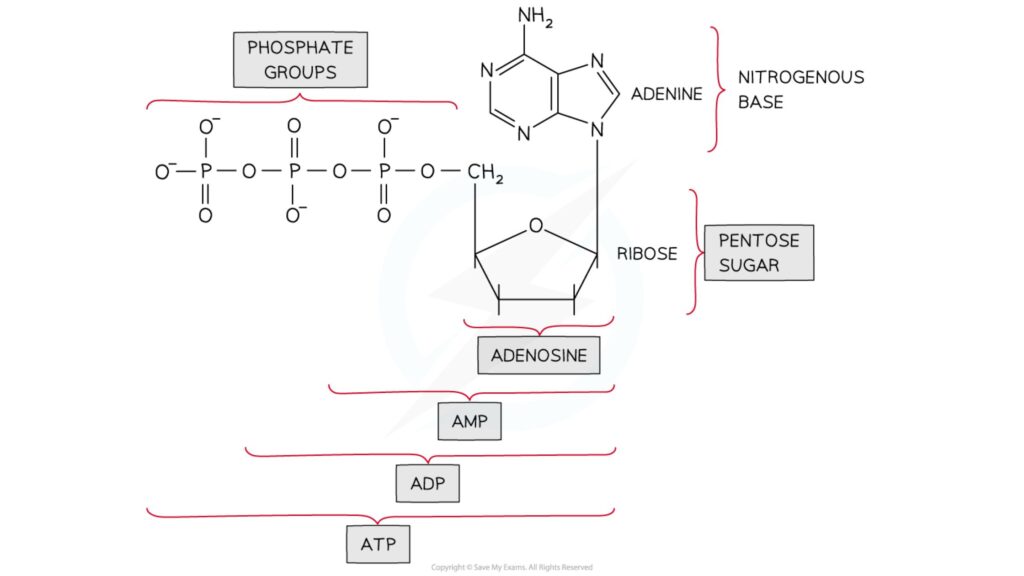
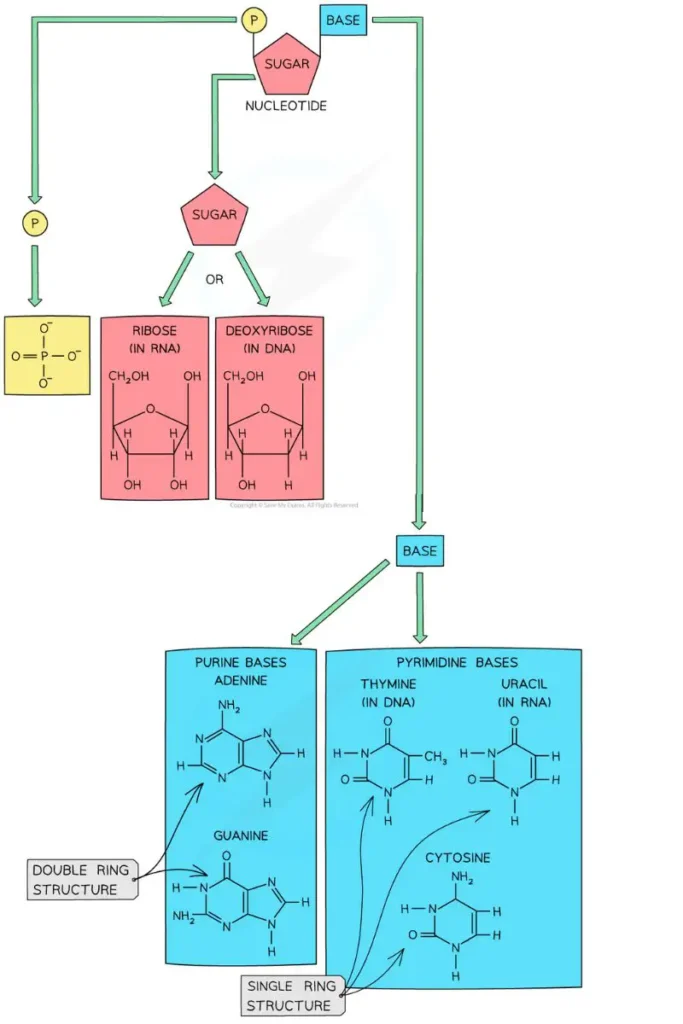

References
- Gates, Andrew & Bowater, Richard. (2015). Nucleotides: Structure and Properties. 10.1002/9780470015902.a0001333.pub3.
- https://www.albert.io/blog/what-are-the-three-parts-of-a-nucleotide/
- https://www.toppr.com/guides/chemistry/biomolecule/structure-of-nucleic-acids/
- Nucleic Acid Structure. (2021, March 22). https://chem.libretexts.org/@go/page/68870
- https://opentextbc.ca/biology/chapter/9-1-the-structure-of-dna/
- https://www.genome.gov/genetics-glossary/Nucleotide#:~:text=A%20nucleotide%20is%20the%20basic,)%20and%20thymine%20(T).
- https://en.wikipedia.org/wiki/Nucleotide
- https://biologydictionary.net/nucleotide/
- http://www2.csudh.edu/nsturm/CHEMXL153/NucleotidesCompandStruc.htm
- https://www.ncbi.nlm.nih.gov/Class/MLACourse/Original8Hour/Genetics/nucleotide.html
- https://www.khanacademy.org/science/ap-biology/gene-expression-and-regulation/dna-and-rna-structure/a/nucleic-acids
- https://en.wikipedia.org/wiki/Nucleoside
- https://atdbio.com/nucleic-acids-book/Nucleic-acid-structure
- https://blog.prepscholar.com/nucleotide-definition
- http://cyberbridge.mcb.harvard.edu/dna_1.html
- http://www.tud.ttu.ee/im/Tonu.Reintamm/shabarova/3.3.html
- http://www.gmch.gov.in/sites/default/files/documents/Nucleotide%20chemistry.pdf
- https://www.pharmacy180.com/article/nucleotide-structure-1956/
- https://knowgenetics.org/nucleotides-and-bases/
- http://www.bu.edu/aldolase/biochemistry/html_docs/Foundations%20of%20Molecular%20Biology%20JM_DTv3.pdf
- https://ib.bioninja.com.au/standard-level/topic-2-molecular-biology/26-structure-of-dna-and-rna/nucleotides.html
- https://step1.medbullets.com/biochemistry/102002/nucleic-acid-structure
- https://researchguides.library.vanderbilt.edu/c.php?g=69346&p=809171
- https://www.jenabioscience.com/nucleotides-nucleosides/nucleotides-by-structure
- https://www.biologyonline.com/dictionary/nucleotide
- https://www.thoughtco.com/what-are-the-parts-of-nucleotide-606385
- http://ndbserver.rutgers.edu/
- https://www.savemyexams.co.uk/as/biology/cie/22/revision-notes/6-nucleic-acids–protein-synthesis/6-1-structure-of-nucleic-acids–replication-of-dna/6-1-1-nucleotides/
Related Posts
- The Wobble Hypothesis – Definition, Exaplanation, Importance
- Emulsion PCR – Principle, Procedure, Advantages, Limitations, Uses
- TaqMan Probe – Definition, Principle, Applications
- Differences Between Sensitivity, Specificity, False positive, False negative
- Protein Synthesis (Translation)- Definition, Steps, Sites, Machinery