- Mycobacterium tuberculosis (M. tuberculosis), also known as Koch’s bacillus, is the primary causative agent of tuberculosis (TB), a severe respiratory disease that continues to be a significant global health challenge. It is estimated that around one-third of the world’s population is infected with this bacterium, leading to approximately 2 million deaths annually. Transmission occurs through inhalation of aerosols, and while the bacterium can infect a person, the disease itself may remain dormant for years. This latency is particularly dangerous for individuals with compromised immune systems, such as AIDS patients, who are at greater risk of developing active TB.
- M. tuberculosis is an intracellular pathogen from the order Actinomycetales. Its cell wall is complex and rich in mycolic acids, peptidoglycan, and arabinogalactan, which contribute to its virulence and make it difficult to target with standard treatments. The waxy coating of mycolic acid also renders the bacterium resistant to Gram staining, so specialized acid-fast stains like Ziehl-Neelsen or fluorescent stains, such as auramine, are required for proper identification under a microscope.
- The physiology of M. tuberculosis is highly aerobic, meaning it thrives in oxygen-rich environments like the lungs, which it primarily targets. Once inside the host, the bacterium can cause either pulmonary TB, affecting the lungs, or extrapulmonary TB, which can spread to other organs. Symptoms often vary widely, leading to frequent misdiagnoses and treatment delays. Diagnosing TB typically involves methods like the tuberculin skin test, acid-fast staining, culture, and advanced molecular techniques such as polymerase chain reaction (PCR).
- The M. tuberculosis genome, sequenced in 1998, is high in guanine and cytosine (G/C) content and encodes numerous proteins associated with its pathogenicity and immune evasion. Treatment of tuberculosis includes a range of antibacterial drugs, while preventive vaccines, such as BCG (Bacillus Calmette–Guérin), offer partial protection, especially for young children.
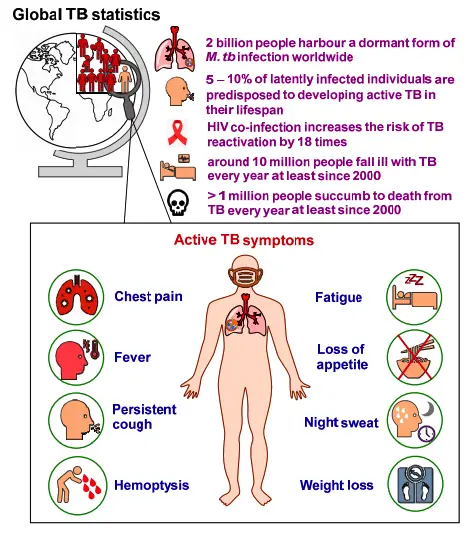
Classification of Mycobacterium tuberculosis
The genus Mycobacterium comprises over 70 species, several of which are pathogenic to humans. The most notable among these is Mycobacterium tuberculosis, the bacterium responsible for tuberculosis (TB). Understanding the classification of M. tuberculosis and its related species is crucial for medical research and public health.
- Historical Context:
- The journey of understanding Mycobacterium began in 1874 when Hansen identified Mycobacterium leprae.
- In 1882, Robert Koch isolated the mammalian tubercle bacillus, confirming its role as the causative agent of tuberculosis, fulfilling all criteria of Koch’s postulates.
- The Mycobacterium tuberculosis Complex:
- The M. tuberculosis complex currently refers to several closely related species capable of causing tuberculosis in humans and other mammals. This complex includes:
- Mycobacterium tuberculosis: Known as the human type, this species is the primary cause of TB worldwide.
- Mycobacterium bovis: Originally associated with cattle, this species can infect humans, particularly those with close contact with livestock.
- Mycobacterium africanum: This species is a hybrid between human and bovine types, mainly affecting individuals in tropical Africa.
- Mycobacterium microti: Primarily pathogenic to small mammals like goats, this species does not typically cause infections in humans.
- The M. tuberculosis complex currently refers to several closely related species capable of causing tuberculosis in humans and other mammals. This complex includes:
- Saprophytic Mycobacteria:
- Beyond the pathogenic species, there exists a third group known as saprophytic mycobacteria. These organisms are commonly found in various environments and can be isolated from:
- Cold- and warm-blooded animals.
- Skin ulcers.
- Environmental sources such as soil and water.
- Initially referred to as atypical environmental or opportunistic mycobacteria, these species are now classified as mycobacteria other than typical tubercle (MOTT).
- Beyond the pathogenic species, there exists a third group known as saprophytic mycobacteria. These organisms are commonly found in various environments and can be isolated from:
Culture of Mycobacterium tuberculosis
The cultivation of Mycobacterium tuberculosis (M. tuberculosis) is crucial for diagnostic and research purposes, given its status as the primary pathogen responsible for tuberculosis. This bacterium has distinct growth characteristics that necessitate specific culture techniques.
- Growth Conditions:
- M. tuberculosis is classified as an obligate aerobe, meaning it requires oxygen for growth. Its optimal growth temperature is around 37°C, and it fails to grow at temperatures below 25°C or above 40°C.
- The ideal pH for growth ranges from 6.4 to 7.0, providing a narrow window for cultivation.
- Growth Rate:
- This slow-growing bacillus has a generation time of approximately 14 to 15 hours, which requires extended incubation periods to observe growth.
- Typically, colonies can appear within 2 weeks, though some cultures may necessitate up to 8 weeks for visibility.
- Culture Media: M. tuberculosis can be cultivated in a variety of enriched solid and liquid media.
- Solid Media:
- Various solid media types are employed for culturing:
- Egg-containing media: Examples include Lowenstein–Jensen (LJ) medium, Petragnani, and Dorset egg mediums.
- Blood-containing media: Such as Tarshis medium.
- Serum-combining media: For example, Loeffler’s serum slope.
- Potato-based media: Including Pawlowsky medium.
- On solid media, M. tuberculosis colonies appear dry, rough, raised, and irregular with a wrinkled surface.
- Among these, the LJ medium is the most commonly used and is recommended by the International Union against Tuberculosis (IUAT). This medium consists of coagulated whole egg, asparagines, malachite green, mineral salts, and glycerol or sodium pyruvate. Malachite green effectively inhibits non-mycobacterial growth. Notably, adding 0.75% glycerol promotes M. tuberculosis growth while inhibiting M. bovis. After 6 to 8 weeks of incubation, colonies on LJ medium turn yellowish or buff in color and are characterized by their tenacious nature.
- Various solid media types are employed for culturing:
- Liquid Media:
- Commonly used liquid media include Soloac’s solutions, Dubos medium, and Middlebrook’s and Beck’s media.
- In liquid cultures, growth typically begins at the bottom of the container and later spreads to the sides, forming a surface pellicle. In Dubos medium containing Tween 80, M. tuberculosis exhibits diffuse growth. In glycerol broth, a whitish, wrinkled pellicle forms on the surface, accompanied by a granular deposit at the bottom.
- Virulent strains of M. tuberculosis tend to form long serpentine cords in liquid media, whereas avirulent strains display a more dispersed growth pattern.
- It is important to note that liquid media are not routinely used for the culture of the bacilli but are instead reserved for specific applications, such as preparing mycobacterial antigens for vaccines and assessing antibiotic sensitivity.
- Solid Media:
Biochemical Reactions of Mycobacterium tuberculosis
These biochemical reactions provide insights into the metabolic capabilities of the bacterium and its pathogenic characteristics.
- Niacin Test:
- One significant biochemical test is the niacin test, which identifies niacin-positive strains of M. tuberculosis, particularly those affecting humans.
- Human strains of M. tuberculosis yield a positive result in this test, producing niacin as a metabolic by-product when cultured on egg-containing solid media.
- In contrast, Mycobacterium bovis strains are niacin-negative, aiding in differentiation between these two species. Additionally, M. simiae and some strains of M. chelonae also exhibit a positive response in the niacin test.
- Catalase Activity:
- Typically, M. tuberculosis is catalase positive, indicating the presence of the catalase enzyme that breaks down hydrogen peroxide into water and oxygen.
- However, a notable characteristic is that the bacterium can lose this catalase activity when it develops resistance to isoniazid (INH). Catalase-negative strains of M. tuberculosis are linked to reduced virulence, particularly in guinea pigs, highlighting the importance of this biochemical property in assessing pathogenicity.
- Amidase Activity:
- The bacterium exhibits amidase activity, which refers to its ability to produce amidase enzymes such as nicotinamidase and pyrazinamidase.
- These enzymes facilitate the cleavage of various amide substrates, playing a role in the bacterium’s metabolism and potentially contributing to its virulence.
- Nitrate Reduction Test:
- M. tuberculosis is also positive for the nitrate reduction test. It possesses the enzyme nitrate reductase, allowing it to reduce nitrate to nitrite.
- This biochemical reaction is essential for differentiating it from other mycobacterial species, such as M. bovis and M. avium, which do not produce nitroreductase and thus yield a negative result in this test.
- Neutral Red Test:
- Both M. tuberculosis and M. bovis are positive for the neutral red test, demonstrating their ability to bind neutral red dye in an alkaline buffer solution. This property can assist in distinguishing these species from others during laboratory testing.
- Arylsulfate Test:
- Conversely, M. tuberculosis is negative for the arylsulfate test, which can further aid in distinguishing it from other mycobacterial species.
Geographical Distribution of Mycobacterium tuberculosis
The geographical distribution of Mycobacterium tuberculosis reflects a significant global health challenge, with a staggering one-third of the world’s population currently infected. The burden of tuberculosis (TB) is disproportionately high in developing countries, where nearly two-thirds of all cases are reported. This distribution highlights critical disparities in public health and access to treatment.
- Developing Countries: The majority of tuberculosis cases arise from developing nations, including India, Pakistan, China, Bangladesh, the Philippines, Indonesia, Thailand, and Congo. These regions face unique challenges, including limited healthcare infrastructure, socioeconomic factors, and high population density, which facilitate the spread of the disease.
- India’s Burden: India stands out as a focal point in the fight against tuberculosis. Approximately 40% of the Indian population is infected with M. tuberculosis. This staggering statistic underscores the urgent need for targeted public health interventions. In India alone, nearly 15 million individuals suffer from active TB, with 3 million classified as highly infectious open cases, posing a substantial risk to community health. Moreover, the country sees around half a million TB-related deaths annually.
- Global Mortality Rates: On a global scale, tuberculosis claims the lives of approximately 3 million individuals each year. This alarming mortality rate emphasizes the critical importance of robust surveillance and treatment strategies, particularly in high-burden countries. Furthermore, around 2 billion people worldwide are estimated to have latent tuberculosis, which signifies an ongoing reservoir of potential infection.
- Risk Factors: The geographical distribution of TB is not uniform; various factors contribute to the vulnerability of specific regions. High rates of poverty, malnutrition, and co-infections such as HIV significantly increase susceptibility to tuberculosis. These interconnected challenges require comprehensive approaches that address the broader determinants of health.
- Public Health Implications: Understanding the geographical distribution of M. tuberculosis is essential for effective public health planning. Tailored strategies that consider the unique epidemiological profiles of affected regions can enhance control measures, improve diagnosis and treatment accessibility, and ultimately reduce the incidence and prevalence of tuberculosis.
Reservoir, Source, and Transmission of Mycobacterium tuberculosis
Understanding the reservoir, source, and transmission pathways of Mycobacterium tuberculosis (M. tuberculosis) is essential for tackling tuberculosis (TB) as a public health concern. Human beings serve as the sole reservoir for this pathogen, which has significant implications for infection control strategies. The transmission of TB relies heavily on the infectiousness of individuals, influenced by various factors including bacillary load and lesion morphology.
- Reservoir and Source: M. tuberculosis has humans as its only reservoir. The infectiousness of an individual largely determines the potential for disease transmission. Infectiousness is primarily influenced by the bacillary load found in pulmonary lesions, particularly in those with cavitary lesions, which can harbor 100 to 10,000 bacilli. Individuals who are untreated are more infectious compared to those receiving antitubercular therapy, as treatment reduces the bacillary load in the lungs.
- Modes of Transmission: The primary mode of acquiring M. tuberculosis is through inhalation of aerosolized droplets. Patients with pulmonary tuberculosis expel these droplets into the environment through actions such as coughing, sneezing, or even speaking. Each cough can release up to 3,000 infectious droplet nuclei into the air. These droplet nuclei are quite small, measuring 5 micrometers or less, and may contain approximately 1 to 10 tubercle bacilli. Although theoretically, a single bacillus can initiate infection, practically, an exposure to 5 to 200 inhaled bacilli is usually necessary for infection to occur. The small size of these droplets allows them to remain suspended in the air for extended periods, enhancing the risk of transmission in poorly ventilated or small spaces.
- Alternative Transmission Pathways: While M. tuberculosis primarily spreads through aerosols, M. bovis, a related bacterium, can be transmitted to humans through the ingestion of raw milk from infected cows. In animals, M. bovis is spread via aerosolized bacilli expelled during coughing. However, person-to-person transmission of M. bovis is rare.
- Risk Factors for Infection: The risk of acquiring TB infection is influenced by various factors:
- HIV Co-Infection: Individuals infected with both HIV and M. tuberculosis exhibit significantly higher rates of TB than those infected with M. tuberculosis alone.
- Immunosuppressive Conditions: Factors such as steroid therapy, cancer chemotherapy, and malignancies increase susceptibility to TB. Undernutrition, which can impair cellular immunity (CMI), also heightens the risk, particularly among impoverished populations.
- Genetic Factors: Certain human leukocyte antigen (HLA) types may predispose individuals to a greater risk of acquiring TB, and the presence of the Bcg gene can also play a role in susceptibility.
- Nontubercular Infections: Other infections, such as pertussis, varicella, and measles, may reactivate dormant M. tuberculosis infections.
- Infection in Other Mammals: M. tuberculosis is primarily a human pathogen, although it can infect other primates, dogs, and some mammals that have close contact with infected humans. Experimental infections indicate that M. tuberculosis can be highly infectious in guinea pigs and hamsters, while being nonpathogenic for bovines, goats, cats, and rabbits. Conversely, M. bovis is more pathogenic in animals and can cause disease in cattle and primates, along with certain experimental models.
- Strain Variability: Different strains of M. tuberculosis, such as African strains or M. africanum, are predominantly found in Africa, while certain Asian strains, characterized by low virulence for guinea pigs and resistance to specific drugs, are mainly isolated from South India and other Asian regions.
Habitat of Mycobacterium tuberculosis
Mycobacterium tuberculosis primarily inhabits human hosts, where it serves as the only known reservoir, but this resilient bacterium can thrive in various environments, both within and outside the human body. Below is an exploration of its habitat and survival mechanisms, focusing on its preferred environments and adaptability across diverse conditions.
- Human Host (Primary Reservoir): The human body provides the main reservoir for M. tuberculosis. Once inhaled, it predominantly infects the lungs, as it requires high oxygen levels, making the respiratory system a prime site for colonization. However, it is not restricted to the lungs and can infect other parts of the body, leading to either pulmonary or extrapulmonary tuberculosis.
- Types of Pathogens: M. tuberculosis is generally considered an obligate pathogen, meaning it typically relies on a host organism for survival and reproduction. While its primary mode is pathogenic, it can also exhibit facultative or opportunistic characteristics under specific circumstances, allowing it to persist and adapt even when conditions are not ideal for growth.
- Infection Mechanism: In human hosts, M. tuberculosis often targets mono-nuclear phagocytes, particularly macrophages, which are immune cells responsible for engulfing and destroying pathogens. However, M. tuberculosis has evolved mechanisms to survive and multiply within these cells, making it challenging for the immune system to eliminate.
- External Survival: Besides thriving in human hosts, M. tuberculosis demonstrates remarkable resilience in external environments. It can persist for weeks on inanimate surfaces, including dust, carpets, and clothing. In sputum (saliva and mucus), it can survive for extended periods, providing a reservoir for transmission and reinfection.
- Environmental Presence: Though primarily a human pathogen, M. tuberculosis can also be found in natural environments, such as soil and water, indicating its capacity to survive in varying conditions. Its resilience in these environments suggests potential transmission routes beyond direct human-to-human contact, although human infection primarily results from inhaling airborne droplets.
- Transmission through Milk: Another transmission route, though less common, involves the consumption of unpasteurized milk. This mode primarily affects individuals who consume milk from infected animals, underscoring the importance of pasteurization as a preventive measure.
Through its adaptability, Mycobacterium tuberculosis remains a formidable pathogen, capable of enduring diverse environments, both within human hosts and externally, which contributes to its widespread prevalence and persistence across various settings.
Morphology of Mycobacterium tuberculosis
Mycobacterium tuberculosis (M. tuberculosis), the bacterium responsible for tuberculosis, exhibits unique morphological traits that contribute to its pathogenicity and resilience. Below is a detailed look at its structure and composition, which play crucial roles in its survival and ability to infect hosts.
- Shape and Size: M. tuberculosis cells are rod-shaped, typically appearing straight or slightly curved. They measure about 0.5 µm in width and around 3 µm in length, although microscopic analysis often shows a slight variation, with lengths averaging around 2.71 ± 1.05 µm. This bacterium can appear singly, in pairs, or in small clumps.
- Non-Spore Forming and Non-Motile: Unlike some bacteria, M. tuberculosis does not form spores, and it is non-motile, meaning it lacks the structures, like flagella, needed for active movement. Additionally, it does not have a capsule.
- Acid-Fast Properties: The bacterium’s cell wall contains high levels of mycolic acids, a type of fatty acid, which gives it its characteristic waxy coat and contributes to its acid-fast nature. This property makes M. tuberculosis neither Gram-positive nor Gram-negative; instead, it requires specialized staining methods. When acid-fast staining is performed, it appears bright red or deep purple against a green or blue background.
- Cell Wall Composition: The cell wall is particularly lipid-rich, with mycolic acids comprising 50-60% of its composition. This thick lipid layer includes waxes and fatty acids, which wrap tightly around the bacterial cell, enhancing its ability to resist various environmental stresses. The outer membrane surface area measures about 3.04 ± 1.33 µm², while the plasma membrane has a surface area of approximately 2.67 ± 1.19 µm².
- Intracellular Growth: M. tuberculosis is capable of growing within host cells, particularly within macrophages, which are immune cells tasked with destroying pathogens. This intracellular capacity makes it highly adept at evading immune responses, allowing it to persist within the host for extended periods.
- Resistance Features: The structural composition of M. tuberculosis contributes to its high resistance to disinfectants, detergents, common antibiotics, osmotic lysis, and other environmental stresses. Its waxy outer layer also resists stains and dyes, making it harder to detect and eliminate.
- Cellular Components: Detailed measurements of M. tuberculosis cells reveal a cell volume averaging around 0.293 ± 0.113 fl, with its outer membrane and periplasm also contributing to its structure. Inside, the cytoplasm contains ribosomes in high density, with an average count of 1672 ± 568 ribosomes, providing essential protein synthesis functions to support its growth and persistence.
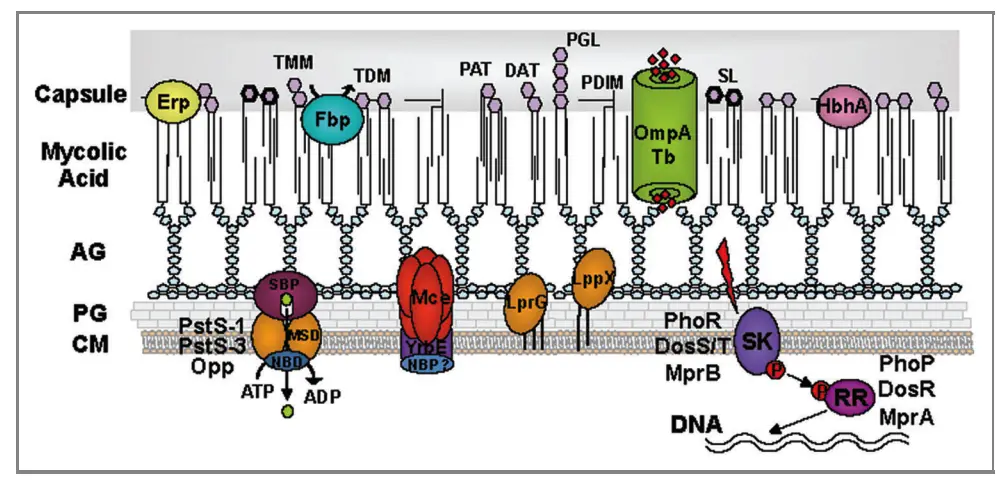
Cell Wall Components and Antigenic Structure of Mycobacterium tuberculosis
The cell wall of Mycobacterium tuberculosis (M. tuberculosis) is a complex structure crucial for the bacterium’s pathogenicity and survival. It comprises multiple layers, each contributing to its unique characteristics and ability to provoke an immune response.
- Layer Composition:
- The cell wall consists of four primary layers:
- Peptidoglycan Layer: This innermost layer is covalently linked with arabinogalactan, a polysaccharide. Its terminal ends attach to the mycolic acid layer.
- Arabinogalactan Layer: This polysaccharide layer provides structural integrity and is critical in linking the peptidoglycan to the mycolic acid.
- Mycolic Acid Layer: This layer, containing free lipids, glycolipids, and peptidoglycolipids, constitutes nearly 60% of the dry weight of the cell wall. It is crucial for several properties of M. tuberculosis.
- Mycosides: This outer layer includes various lipids that enhance the bacterium’s resistance to environmental stresses.
- The cell wall consists of four primary layers:
- Functionality of the Cell Wall:
- The mycolic acid fraction confers several important characteristics to M. tuberculosis:
- Acid Fastness: This property allows the bacterium to resist decolorization by acids, a feature that aids in its identification in clinical specimens.
- Slow Growth: The unique structure of the cell wall slows the permeation of nutrients, contributing to the bacterium’s lengthy generation time.
- Antibiotic Resistance: The lipid-rich cell wall provides a barrier against commonly used antibiotics, complicating treatment efforts.
- Detergent Resistance: The cell wall’s composition also grants resistance to detergents, further protecting the bacterium in hostile environments.
- Resistance to Acids and Alkalis: This unusual resilience helps M. tuberculosis survive in varying pH conditions.
- Clumping or Cord Formation: The cell wall’s structure leads to the formation of clumps or cords, influencing how the bacteria aggregate in tissue.
- The mycolic acid fraction confers several important characteristics to M. tuberculosis:
- Antigenic Structure:
- Strains of M. tuberculosis display antigenic homogeneity and share similarities with M. bovis and M. microti, while remaining distinct from other mycobacterial species. The antigenic structure comprises two main types of antigens:
- Cell Wall Insoluble Polysaccharide Antigens: These antigens, including arabinogalactans and lipoarabinomannan, contribute to group specificity. They play a critical role in the bacterium’s interaction with the host immune system.
- Cytoplasmic Soluble Protein Antigens: These antigens confer type specificity and include several notable proteins: antigen 5, antigen 6, antigen 14, antigen 19, antigen 33, antigen 38, and antigen 60. These proteins are essential in eliciting specific immune responses in infected individuals.
- Strains of M. tuberculosis display antigenic homogeneity and share similarities with M. bovis and M. microti, while remaining distinct from other mycobacterial species. The antigenic structure comprises two main types of antigens:
Molecules Involved in Pathogenesis and Virulence of Mycobacterium tuberculosis
Mycobacterium tuberculosis (MTB) employs a variety of molecules within its cell envelope, secretion systems, and proteins to establish and sustain infection, contributing significantly to its pathogenesis and virulence. These molecules aid in immune evasion, intracellular survival, and host tissue invasion. Key components include complex lipids, lipoproteins, and specialized secretion systems that modulate the host’s immune response. Below are essential molecules and mechanisms in MTB virulence.
Mycobacterial Lipids
- Lipoarabinomannan (LAM): This glycolipid helps stabilize the mycobacterial cell wall. It inhibits phagosome maturation in macrophages by blocking phagolysosome fusion, enabling intracellular survival. Variants like mannose-capped LAM (ManLAM) differ by strain, affecting interferon-γ production and adherence to macrophages. LAM also triggers pro-inflammatory cytokines, such as TNF-α and IL-10, contributing to immune modulation.
- Lipomannan (LM): Present alongside LAM, LM activates macrophages through Toll-like receptors (TLR2 and TLR4). This molecule can both stimulate immune responses (e.g., TNF-α production) and inhibit them, depending on its acylation state, by reducing NO production in macrophages. LM’s structural changes can weaken mycobacterial resistance, increasing susceptibility to antibiotics and macrophage-mediated killing.
- Phosphatidylinositol Mannosides (PIMs): These glycolipids, precursors of LAM and LM, promote macrophage uptake of MTB through mannose receptors and influence immune response by recruiting natural killer T-cells. Higher-order PIMs are associated with virulence in macrophages by affecting cytokine release, such as TGF-β and reactive oxygen species.
- Trehalose-6,6’-dimycolate (TDM): Known as cord factor, TDM is highly toxic and inflammatory. It prevents phagosome-lysosome fusion and drives cytokine responses in macrophages, contributing to granuloma formation and maintenance, a hallmark of TB pathology. TDM-coated particles cause delayed phagosome maturation, essential for MTB survival.
- Phthiocerol Dimycocerosate (PDIM) and Phenolic Glycolipids (PGL): PDIM stabilizes the mycobacterial envelope, enhancing resistance to host defenses. It prevents phagosome acidification, protects against reactive nitrogen species, and supports intracellular survival. PGL, particularly in M. leprae, displays neurotoxic properties, leading to demyelination.
Secretion Systems
- Twin-Arginine Transporter (TAT): This system exports folded proteins, including those involved in cell wall stability and virulence. TAT-mediated secretion of phospholipases and beta-lactamase suggests potential as a target for TB drug development. Proteins secreted via TAT are crucial for cell wall integrity and beta-lactam antibiotic resistance.
- ESX Transporters:
- ESX-1: Critical for cytosolic contact during infection, this system disrupts host cell membranes and supports MTB dissemination by inducing host cell lysis. It also participates in genetic exchange through conjugation.
- ESX-3: Key in zinc and iron uptake, ESX-3 hinders phagosome maturation, impairing antigen presentation in macrophages. Components like EsxG and EsxH activate inflammatory responses and are linked with macrophage cytokine production.
- ESX-5: Found only in slow-growing mycobacteria, ESX-5 is pivotal for exporting PE/PPE proteins. It is activated under phosphate limitations, affecting cell wall integrity and immune response by inducing inflammasome activity.
PE and PPE Proteins
- PE-PPE and PE-PGRS: These protein families facilitate MTB’s entry and persistence in host cells by modulating immune responses. PE-PGRS proteins interact with TLR2, affecting cytokine secretion and macrophage necrosis, supporting intracellular survival. Mutations in PE-PGRS genes lead to reduced virulence, impaired lung colonization, and limited tissue damage in models.
Lipoproteins
- LpqH (19 kDa protein): This lipoprotein triggers T-cell proliferation and DC maturation. By engaging TLR2, LpqH promotes autophagy and antigen presentation, playing a role in adaptive immunity activation. It also induces apoptosis in macrophages through caspase-dependent pathways.
- LppX: Associated with PDIM release, LppX is essential for full virulence, particularly affecting lipid distribution on the cell surface.
- Mpt83: This glycoprotein enhances MTB adhesion to host cells and triggers pro-inflammatory cytokines like IL-6 and TNF-α. It is involved in immune responses in individuals vaccinated with BCG.
- LprG: Essential for phagosome maturation arrest, LprG controls lipid localization in the cell wall. Mutants lacking LprG show reduced survival and virulence in host environments.
- RpfB: This lipoprotein stimulates immune responses during MTB reactivation, driving Th1-type immunity via TLR4.
These diverse molecular strategies allow MTB to evade the immune system, persist within host cells, and cause the characteristic granulomatous inflammation associated with TB. Understanding these molecules’ roles may provide pathways for novel therapeutic targets and vaccine development.
Non-Protein Virulence Factors in Mycobacteria
Non-protein virulence factors play a critical role in the survival and pathogenicity of Mycobacterium tuberculosis (Mtb) and related species. These components, which include lipids, glycolipids, glycans, nucleic acids, and metabolites, contribute to the bacteria’s ability to evade host defenses and promote infection. Understanding these factors offers insight into the complex interplay between pathogens and their hosts.
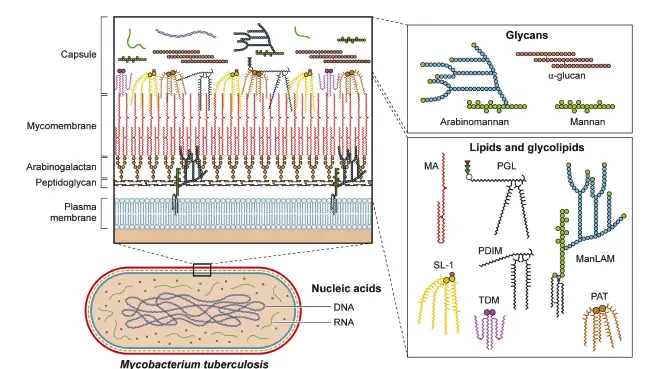
- Lipid and Glycolipid Functions
Bacterial outer membranes and cell walls often shed various components that significantly influence host biology. For example, lipopolysaccharides (LPS) from gram-negative bacteria activate host responses via pattern recognition receptors, such as Toll-like receptor 4 (TLR4). In Mtb, mycolic acids, which are long-chain fatty acids, are integral to the outer membrane. They inhibit TLR2, thereby reducing pro-inflammatory interleukin-8 (IL-8) production and modulating host immune responses. Additionally, free mycolic acids enhance the recruitment of macrophages that support Mtb survival. - Trehalose Conjugates
Trehalose monomycolate (TMM) and trehalose dimycolate (TDM) are prominent glycolipids in the Mtb cell envelope. TDM, known as cord factor, is notable for inducing filamentous growth patterns and triggering immune responses via Monocyte-Inducible C-type Lectin (Mincle) receptors in macrophages. This interaction enhances the production of pro-inflammatory cytokines and may modify immune responses through structural alterations during Mtb infection. - Phthiocerol Dimycocerosates (PDIM)
PDIMs are another critical class of lipids found in the outer membrane of mycobacteria. They mask pathogen-associated molecular patterns (PAMPs), allowing Mtb to evade detection by host immune receptors. After phagocytosis, PDIMs have been shown to disrupt phagosome integrity, thus inhibiting autophagic degradation of the bacteria. - Phenolic Glycolipids (PGL)
PGLs, formed from PDIM linked to sugars, have been implicated in enhancing virulence among certain Mtb lineages. PGL activates the cytosolic surveillance pathway and promotes the secretion of MCP-1, a chemokine that recruits macrophages conducive to Mtb survival. Furthermore, PGL has been associated with antagonistic interactions against TLR2, further complicating host immune responses. - Sulfolipids
Sulfolipid-1 (SL-1) is a prevalent lipid in Mtb, playing a less direct role in virulence but is involved in stimulating cough reflexes in infected hosts. The regulation of SL-1 production during different infection stages highlights its potential role in facilitating transmission of the pathogen. - Phosphatidylinositol Mannosides (PIMs)
PIMs and their derivatives, such as lipomannan (LM) and lipoarabinomannan (LAM), are essential for Mtb’s cell envelope integrity and immune evasion. They engage scavenger receptors, inhibiting nitric oxide production in macrophages and preventing the degradation of Mtb within phagolysosomes. This mechanism underscores the importance of these glycolipids in establishing a replicative niche within host cells. - Glycans
Glycans such as peptidoglycan and arabinogalactan contribute significantly to the structural integrity of the Mtb cell envelope. Glycans within the bacterial capsule interact with host cells, potentially facilitating the initial infection stages. α-Glucan, a key capsular component, has been shown to modulate both innate and adaptive immune responses. - Nucleic Acids
Mtb employs a specialized Type VII secretion system, ESX-1, to breach phagosomal membranes and access the cytosol. Within the cytosol, mycobacterial DNA can activate the host cyclic GMP-AMP synthase (cGAS) pathway, leading to interferon-beta (IFN-β) production. While this may aid Mtb in establishing infection, it can also trigger host autophagy mechanisms that facilitate bacterial clearance. Additionally, Mtb secretes RNA into the host cytoplasm, further influencing immune signaling pathways.
Protein Virulence Factors in Mycobacterium tuberculosis
The study of protein virulence factors in Mycobacterium tuberculosis (Mtb) reveals critical insights into how this obligate intracellular pathogen manipulates host immune responses to ensure its survival. Mtb has evolved a sophisticated arsenal of proteins that target various host cellular mechanisms, which can be categorized based on their specific functions and interactions with the host.
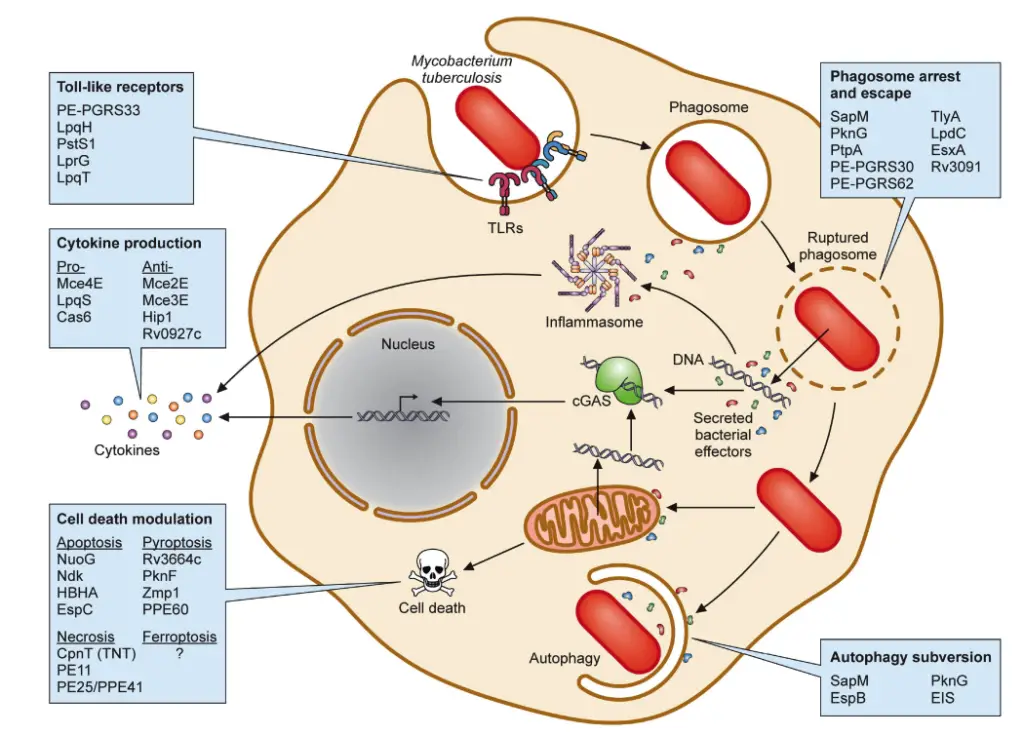
- Modifiers of TLR2 Activity
Toll-like receptor 2 (TLR2) plays a pivotal role in recognizing Mtb through its cell wall ligands. Upon TLR2 activation, signaling cascades involving the adapter protein MyD88 lead to the production of pro-inflammatory cytokines such as TNF-α, IL-1β, and IL-12. Mtb employs several mechanisms to exploit this pathway. For instance, the PE and PPE protein families, comprising a significant portion of the Mtb genome, interact with TLR2 to modulate the host immune response. Notable members include PE-PGRS33, which activates pro-inflammatory cytokines through TLR2 in a calcium-dependent manner. Other lipoproteins like LprG, LpqH, and LpqT also engage TLR2 but exhibit varying effects on immune responses, complicating the understanding of their roles. - Regulators of Cytokine Production
Mtb further manipulates host responses by regulating cytokine production. Proteins within the mammalian cell entry (Mce) operons inhibit key signaling pathways, leading to decreased levels of pro-inflammatory cytokines such as TNF-α and IL-6. For example, Mce2E (LprL) and Mce3E (LprM) interfere with the activation of the ERK and JNK MAPK pathways, while the serine hydrolase Hip1 suppresses pro-inflammatory cytokine production in dendritic cells. Conversely, lipoprotein LpqS stimulates TNF-α and IL-12 production, highlighting the complexity of Mtb’s manipulation of cytokine responses. - Phagosome Maturation Arrest and Phagosome Escape
Following phagocytosis, Mtb resides within phagosomes, which normally mature and fuse with lysosomes for degradation. Mtb employs several strategies to inhibit this maturation process. Proteins such as SapM and PknG prevent phagosome-lysosome fusion by targeting specific host proteins, thereby facilitating bacterial survival. SapM dephosphorylates phosphatidylinositol 3-phosphate (PI3P) in phagosomal membranes, while PknG interferes with Rab7L1, crucial for phagosome maturation. Similarly, PtpA disrupts the phagosome-lysosome fusion by interacting with the vacuolar ATPase complex, underscoring the pathogen’s adeptness at evading host defenses. - Subversion of Autophagy
Autophagy serves as a defense mechanism against intracellular pathogens, including Mtb. Mtb has developed strategies to counteract autophagic clearance, with proteins like SapM and PknG also implicated in autophagy modulation. SapM inhibits the fusion of autophagosomes with lysosomes, while PknG enhances autophagy induction yet simultaneously prevents maturation, thereby promoting bacterial survival. Another virulence factor, EIS, disrupts autophagy by activating the PI3K/AKT/mTOR pathway, which further hinders the autophagic process. - Modulation of Cell Death Pathways
Mtb can influence various cell death pathways, including apoptosis and pyroptosis. While apoptosis can be detrimental to Mtb, several virulence factors, such as NuoG and Ndk, inhibit this process, allowing the bacteria to persist within host cells. In contrast, other factors like the heparin-binding hemagglutinin antigen (HBHA) can induce apoptosis. Similarly, pyroptosis, a form of programmed cell death that restricts Mtb growth, is inhibited by Mtb proteins like Rv3364c and PknF, which dampen inflammasome activation.
Pathogenesis of Mycobacterium tuberculosis
Mycobacterium tuberculosis (M. tb) is the causative agent of tuberculosis (TB), a disease characterized by its complex pathogenesis and unique interactions with the host’s immune system. Understanding the intricate mechanisms by which M. tb establishes infection, survives within the host, and ultimately leads to disease progression is critical for effective treatment and prevention strategies.
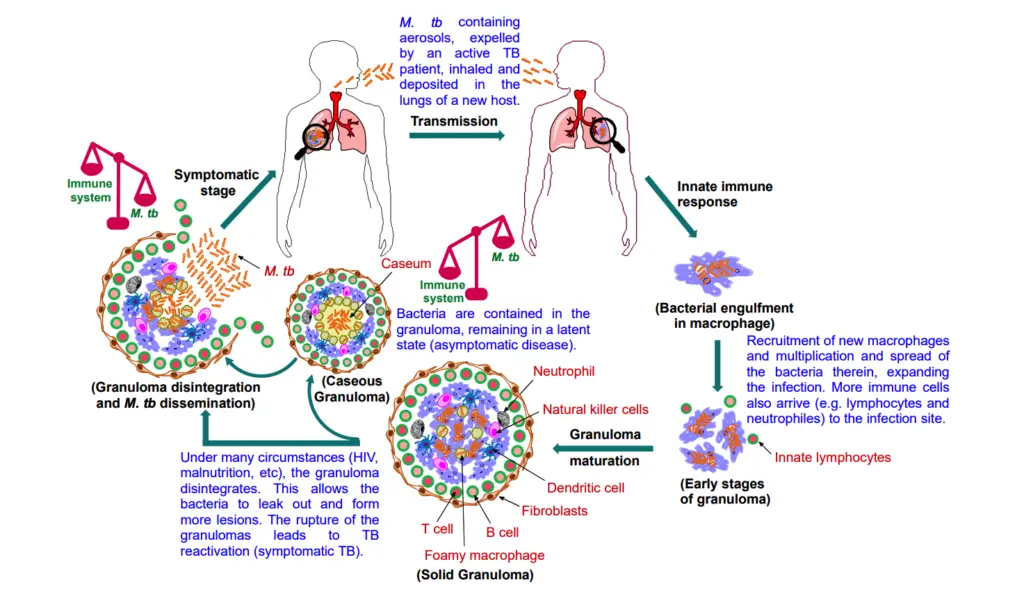
- Transmission and Initial Infection: TB is primarily transmitted through aerosol droplets expelled by individuals with active TB during coughing, sneezing, or talking. When a susceptible host inhales these droplets, the bacteria traverse the respiratory tract and reach the alveoli in the lungs.
- Innate Immune Response: Upon reaching the lungs, M. tb encounters the host’s innate immune system. Alveolar macrophages, the first line of defense, attempt to phagocytize and eliminate the bacteria. However, if these macrophages are unable to contain the infection, M. tb can multiply intracellularly. This replication allows the bacteria to escape and infect additional macrophages, perpetuating the cycle of infection.
- Cell-Mediated Immunity: As the infection progresses, lymphocytes are recruited to the site, initiating a cell-mediated immune response. This influx of immune cells aims to sequester the bacteria, leading to the formation of a granuloma, a hallmark of TB. At this stage, many individuals remain asymptomatic, with the potential for the bacteria to be completely eliminated or to enter a latent phase.
- Granuloma Formation: The granuloma serves as a protective structure that confines the bacilli. Although it restricts bacterial spread, the granuloma does not fully eradicate the pathogen. M. tb has evolved mechanisms to evade destruction by blocking phagolysosomal fusion and modulating the host’s immune responses, thereby establishing a niche for survival. The bacteria can persist in a non-replicating or slowly replicating state within the granuloma for extended periods, rendering the host non-infectious.
- Macrophage Differentiation and Lipid Metabolism: As granulomas mature, macrophages differentiate into foamy macrophages, characterized by the accumulation of lipid droplets. This transformation is influenced by M. tb’s disruption of host lipid metabolism, leading to the formation of foam cells that support bacterial persistence. The necrosis of immune cells at the granuloma’s center forms caseous (cheese-like) necrotic tissue, which becomes a reservoir for the bacteria.
- Progression to Active TB: In immunocompromised individuals, various factors such as HIV co-infection, malnutrition, or immune-suppressive therapies can reactivate dormant M. tb within granulomas. As the granuloma liquefies and cavitates, the bacteria proliferate, leading to symptomatic active TB. The transmission cycle restarts as infectious bacilli are expelled from the lungs into the environment.
- Granuloma Dynamics: Granulomas evolve through stages, including solid, necrotic, and caseous forms. Solid granulomas typically arise in the early stages of infection and are characterized by low bacterial loads, while caseous granulomas develop later and indicate tissue necrosis and a higher bacterial burden. The structural integrity of granulomas is crucial in determining the disease’s progression; as granulomas deteriorate, they facilitate bacterial dissemination through the lungs and into the bloodstream.
- Clinical Implications: Understanding the pathogenesis of M. tb, particularly the interplay between the bacilli and the host immune response, is vital for developing effective therapeutic strategies. The challenge of targeting the persistent bacteria within granulomas remains a significant hurdle in TB management, underscoring the need for continued research into novel treatment approaches.
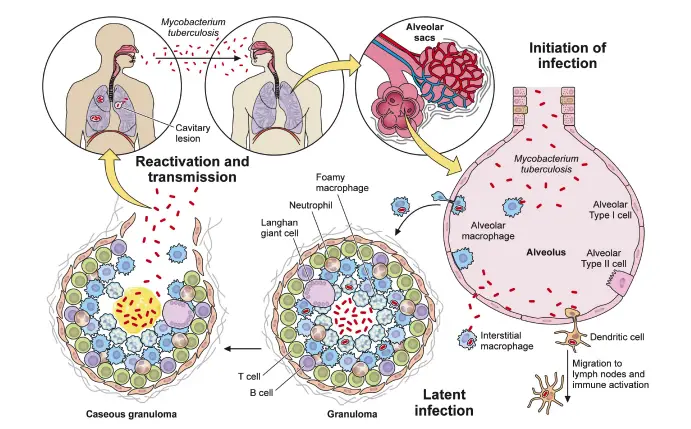
Immune system evasion
Mycobacterium tuberculosis (MTB) demonstrates remarkable capabilities to evade the host immune response, particularly through its residence within immune cells. Unlike many pathogens that are cleared through direct attack, MTB cleverly utilizes macrophages as a niche for survival. The bacterium employs a range of strategies to thwart the microbicidal mechanisms of macrophages, significantly impacting the host’s immune response.
- Phagosome-Lysosome Fusion Disruption:
- MTB can inhibit the fusion of the phagosome with lysosomes, preventing its degradation. Phosphatases like PtpA and SapM play critical roles in this process. PtpA interferes with vacuolar H+-ATPase, crucial for phagosome acidification, by dephosphorylating its substrates. This results in an environment that supports bacterial survival.
- SapM dephosphorylates phosphatidylinositol 3-phosphate (PI3P) on the phagosome membrane, which is essential for acquiring lysosomal constituents. The absence of SapM leads to impaired growth in macrophages, indicating its importance in maintaining the phagosome’s functionality for the bacterium.
- Inhibition of Phagosome Maturation:
- MTB secretes various macromolecules to disrupt the maturation of the phagosome. Factors such as LAM (lipoarabinomannan), TDM (trehalose dimycolate), and the Esx-1 secretion system are implicated in this arrest. This process is not uniform across different mycobacterial species, showcasing the complexity of immune evasion mechanisms.
- Resistance to Reactive Oxygen and Nitrogen Species:
- Upon phagocytosis, macrophages produce reactive oxygen and nitrogen species (ROS and RNS) to eliminate pathogens. MTB exhibits a remarkable ability to resist these toxic agents. It utilizes proteins like Ndk, which has nucleoside diphosphate kinase activity and is secreted by the bacteria. Ndk interferes with Rho GTPases, leading to decreased phagolysosome fusion and reduced production of ROS.
- Additionally, the bacteria employ a variety of protective enzymes, including superoxide dismutase (SOD) and catalase, to detoxify ROS. For instance, SOD converts superoxide radicals into hydrogen peroxide, while catalase degrades hydrogen peroxide, providing a significant survival advantage within the host.
- Mechanisms to Inhibit Apoptosis:
- MTB can also evade programmed cell death through apoptosis, a vital innate defense mechanism of macrophages. The bacterium promotes survival through various means, including neutralizing ROS and modulating macrophage signaling pathways.
- Key factors such as the nuoG gene and serine/threonine kinases like PknE are essential for the bacterium’s ability to inhibit apoptosis. The NuoG protein plays a significant role in suppressing host cell apoptosis, allowing MTB to persist in the host despite immune challenges.
- Interaction with Host Signaling Pathways:
- MTB also engages with host cellular signaling pathways to manipulate immune responses. For example, it can downregulate the production of pro-inflammatory cytokines like TNF-α and IL-6, further contributing to its survival within the host.
Epidemiology of Mycobacterium tuberculosis
Mycobacterium tuberculosis (M. tuberculosis) is a significant global health concern, with approximately 30% of the world’s population estimated to be infected. This bacterium primarily causes tuberculosis (TB), a condition especially prevalent in developing nations. The relationship between M. tuberculosis and HIV is particularly alarming, as TB is the most frequently encountered opportunistic infection among individuals with HIV.
- Prevalence and Risk: The lifetime risk of progressing from M. tuberculosis infection to active tuberculosis disease is estimated to be between 10% and 20% for those infected solely with the bacterium. However, this risk is substantially heightened in individuals co-infected with HIV. Approximately 8 million individuals are affected by both M. tuberculosis and HIV, leading to a significantly increased risk of developing TB.
- Incidence Rates: In the year 2000, the global landscape of TB saw 8,311,000 new cases, translating to an incidence rate of about 137 cases per 100,000 people. Alarmingly, among these new cases, 511,000 were found in patients co-infected with HIV. This indicates a concerning trend in the dual burden of these infections, particularly in vulnerable populations.
- Trends Over Time: Despite various public health efforts aimed at controlling and preventing tuberculosis, there was an observed increase in the total number of new TB cases, averaging 1.8% annually between 1997 and 2000. This persistent rise is troubling, given the availability of effective therapies for TB.
- Mortality Rates: Tuberculosis continues to be one of the leading causes of morbidity and mortality, particularly among HIV-positive individuals in underdeveloped regions. In the year 2000, TB infection was responsible for 1,839,000 deaths globally, with 12% of these fatalities occurring in individuals with HIV. Moreover, tuberculosis accounted for approximately 11% of all AIDS-related deaths in the same year.
- Geographical Distribution: The burden of tuberculosis is disproportionately concentrated in specific regions, with the highest rates of infection reported in parts of Africa, Southeast Asia, and South America. This geographical disparity highlights the need for targeted interventions and resources in these areas to address the TB epidemic effectively.
Clinical Syndromes of Mycobacterium tuberculosis
Mycobacterium tuberculosis (M. tuberculosis) primarily manifests as pulmonary infection but can also affect other parts of the body, leading to various clinical syndromes. The clinical presentation is heavily influenced by the site of infection and can vary significantly among individuals.
Pulmonary Tuberculosis
- Typical Symptoms:
- Common signs include a productive cough, fever, and weight loss.
- Patients may experience hemoptysis (coughing up blood), chest pain, night sweats, fatigue, and anorexia.
- The sputum produced may be scanty, bloody, or purulent, often associated with cavitary lesions in the lungs.
- Forms of Pulmonary Tuberculosis:
- Progressive Pulmonary Disease: A severe and worsening form of the disease.
- Pleural Involvement: Can lead to pleuritis or pleural effusion.
- Reactivated Pulmonary Disease: Occurs when dormant bacteria re-emerge.
- Complications:
- Possible complications include relapse of the disease, formation of aspergillomas (fungal infections within a pre-existing lung cavity), bronchiectasis, broncholithiasis (calcified material in the bronchi), and fibrothorax (fibrosis of the pleural space).
Extrapulmonary Tuberculosis
Extrapulmonary tuberculosis arises from the hematogenous spread of M. tuberculosis during its initial multiplication in the lungs. The manifestations vary depending on the organ system affected.
- Genitourinary Tuberculosis:
- This is one of the most common forms of extrapulmonary tuberculosis.
- Symptoms typically include dysuria (painful urination), increased frequency of urination, and flank pain.
- In men, it may present as epididymitis or a scrotal mass, while women may experience pelvic inflammatory disease. This condition can account for approximately 10% of female sterility.
- Tubercular Meningitis:
- Considered one of the most severe complications of tuberculosis.
- Patients may suffer from persistent or intermittent headaches, indicating possible central nervous system involvement.
- Gastrointestinal Tuberculosis:
- The clinical manifestations depend on the specific site affected in the gastrointestinal tract.
- For instance, infections in the stomach or duodenum may present as abdominal pain similar to peptic ulcer disease, whereas infections in the large intestine can lead to abdominal pain and diarrhea.
- Skeletal Tuberculosis:
- The spine is the most commonly affected site, leading to Pott’s disease, which involves vertebral collapse and deformity.
- Tubercular Lymphadenitis:
- Often referred to as scrofula, this condition primarily affects lymph nodes in the neck, particularly along the sternocleidomastoid muscle.
- Typically unilateral, it presents with minimal pain.
- Other Conditions:
- Other manifestations may include miliary tuberculosis (disseminated spread of tuberculosis), cutaneous tuberculosis, and tuberculosis affecting the middle ear and ocular structures.
Laboratory Diagnosis of Tuberculosis
The laboratory diagnosis of tuberculosis (TB) is crucial for accurate identification and subsequent treatment of the disease. Several methods are employed to detect Mycobacterium tuberculosis, each with its specific applications and methodologies.
Specimen Collection and Processing
- Specimen Types: Various specimens can be used for TB diagnosis, including:
- Sputum
- Bronchial washings
- Brushings or biopsies
- Early morning gastric aspirates
- Cerebrospinal fluid (CSF)
- Urine
- Processing Techniques:
- For specimens collected from nonsterile sites, such as sputum, they should be liquefied with N-acetyl-L-cysteine and decontaminated with sodium hydroxide. This process helps eliminate non-tuberculous bacteria and fungi, facilitating the isolation of mycobacteria.
- Specimens from sterile sites, like CSF, do not require decontamination and can be directly centrifuged and cultured.
Direct Detection Methods
- Microscopy:
- The Ziehl–Neelsen (ZN) staining technique is commonly used to detect the acid-fast property of mycobacteria in sputum and other clinical materials. Red bacilli become visible against a contrasting background.
- Fluorescence microscopy, utilizing the same principle of acid-fastness, offers a less labor-intensive alternative for identifying mycobacteria.
- Culture:
- Due to the presence of various bacteria and fungi that can overgrow mycobacteria, specimens like sputum undergo decontamination before culturing. Mycobacteria are resistant to certain acids and disinfectants, allowing them to survive this process.
- The inoculated Lowenstein Jensen (LJ) medium reveals distinct colonies described as dry, rough, and buff-colored, indicative of mycobacterial growth.
- Liquid media with radiometric growth detection, such as BACTEC 460 TB, enhance the culture method’s efficiency.
- Biochemical Analysis:
- A series of biochemical tests are employed to characterize mycobacterial strains:
- Niacin accumulation test: positive
- Arylsulphatase test: positive
- Neutral red test: positive
- Catalase peroxidase test: peroxidase positive and weakly catalase positive
- Amidase test: positive
- Nitrate reduction test: positive
- Tween 80 hydrolysis: variable results
- Susceptibility tests, including sensitivity to pyrazinamide and resistance to thiophene-2-carboxylic acid hydrazide (TCH), are also conducted.
- A series of biochemical tests are employed to characterize mycobacterial strains:
- Animal Inoculation:
- Historically, guinea pig inoculation was a standard method for diagnosing TB, but it is now considered obsolete due to advancements in laboratory techniques.
- Serodiagnosis:
- Enzyme-linked immunosorbent assay (ELISA) techniques measure specific antibody titers (IgM, IgA, and IgG) against mycobacterial antigens for rapid diagnosis.
- Latex agglutination tests, using latex particles coated with antibodies to M. tuberculosis, exhibit a sensitivity of 94.4%, although their specificity remains low.
- Antigen-Protein Detection:
- Recent advancements allow for the detection of microbial components, such as tuberculostearic acid extracted from the mycobacterial cell wall, using gas chromatography or mass spectrometry in clinical samples.
- Molecular Techniques:
- The introduction of hybridization assays and nucleic acid amplification tests has improved the early identification of M. tuberculosis complex in liquid cultures.
- Polymerase chain reaction (PCR) sequencing, which amplifies mycobacterial DNA using genus-specific primers, is employed to confirm identification through nucleotide sequence comparison with reference sequences, particularly the 16S rRNA gene.
Indirect Detection Methods
- Tuberculin Test:
- The purified protein derivative (PPD) is administered to assess hypersensitivity to mycobacterial antigens.
- The results are interpreted based on induration measured at the injection site after 72 hours, with different thresholds for individuals at varying risk levels for TB.
- Interferon-Gamma Release Assay (IGRA):
- IGRAs provide an alternative diagnostic method, particularly in individuals with BCG vaccination or those exposed to nontuberculous mycobacteria.
- These assays measure the immune response by detecting interferon-gamma released from sensitized CD4 T cells in response to specific antigens from M. tuberculosis. Two commonly used assays include:
- Quantiferon-Gold In-Tube Test (QFT-GIT): An ELISA that detects interferon-gamma in whole blood.
- T-SPOT.TB: An ImmunoSpot assay utilizing purified peripheral blood mononuclear cells.
Treatment of Tuberculosis
The treatment of tuberculosis (TB) is structured around a core regimen of anti-TB agents, specifically designed to combat the disease effectively. Following the guidelines established by the World Health Organization (WHO), treatment protocols emphasize both the duration and the composition of medication to maximize efficacy and minimize resistance.
- Core Anti-TB Agents: The primary medications employed in the initial treatment of TB are:
- Isoniazid (INH)
- Rifampin (RIF)
- Pyrazinamide (PZA)
- Ethambutol (EMB)
- Standard Treatment Regimen: The WHO recommends that all new TB patients, regardless of the site or severity of their infection and in the absence of any documented drug resistance, should undergo a comprehensive treatment protocol. This regimen consists of:
- An intensive 2-month phase: Administering a combination of rifampicin, isoniazid, pyrazinamide, and ethambutol.
- A continuation 4-month phase: Followed by rifampicin and isoniazid alone.
- Potential Side Effects: It is important to note that isoniazid can lead to mild psychiatric disturbances and peripheral neuropathy, particularly in patients with a history of alcohol use. To mitigate these side effects, healthcare providers often prescribe pyridoxine (vitamin B6) as a preventive measure.
- Resistance to Treatment: Drug resistance poses a significant challenge in TB treatment. Resistance may occur in two main forms:
- Acquired Resistance: This develops during therapy, often due to the use of substandard medications or lack of proper supervision.
- Primary Resistance: Patients may also present with resistance from the outset due to infection with resistant strains.
- Types of Resistant Strains:
- Multidrug-Resistant Tuberculosis (MDR-TB): This is characterized by resistance to at least isoniazid and rifampicin, with or without resistance to other drugs.
- Extensively Drug-Resistant Tuberculosis (XDR-TB): This more severe form of TB is defined by resistance to at least isoniazid, rifampicin, a fluoroquinolone, and any injectable agent.
- Second-Line Drugs: In cases of drug resistance, second-line medications are employed. These include:
- Fluoroquinolones: Such as moxifloxacin, levofloxacin, or gatifloxacin.
- Injectable Agents: Options include amikacin, kanamycin, or capreomycin.
- Additional Core Second-Line Agents: This category encompasses drugs like ethionamide, prothionamide, cycloserine, terizidone, clofazimine, or linezolid, which may be used in combination.
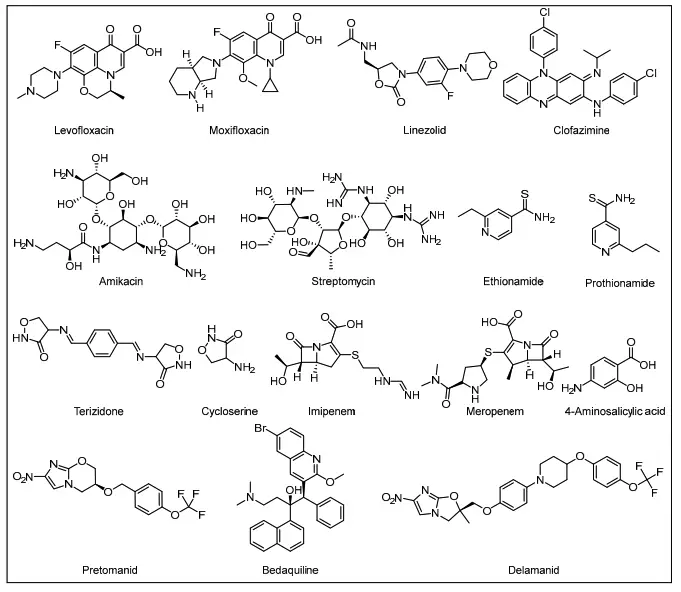
Current Treatment Regimen for Drug-Sensitive (DS) TB
The treatment regimen for drug-sensitive tuberculosis (DS-TB) is crucial in controlling and eliminating the disease. Current guidelines advocate for a specific combination of four first-line antibiotics, each playing a vital role in effectively targeting Mycobacterium tuberculosis (M. tb).
- Combination of Antibiotics: The primary drugs used in the treatment of DS-TB include:
- Isoniazid (INH)
- Rifampicin (RIF)
- Pyrazinamide (PZA)
- Ethambutol (EMB)
- Duration and Administration: The treatment protocol spans at least 6 months and is structured into two distinct phases:
- Initial Phase: The first 2 months involve administering all four antibiotics concurrently. This phase aims to reduce the bacterial load significantly.
- Continuation Phase: The following 4 months involve using only isoniazid and rifampicin to eliminate any remaining dormant bacteria, ensuring a comprehensive eradication of the infection.
- Mechanisms of Action: Each antibiotic operates through different mechanisms to combat M. tb:
- Isoniazid (INH): This drug targets the synthesis of mycolic acids, which are essential components of the bacterial cell wall. It interferes with the formation of the mycobacterial outer coating, effectively disrupting its structural integrity.
- Rifampicin (RIF): By binding to the beta subunit of bacterial RNA polymerase, rifampicin inhibits the transcription process, blocking the early stages of gene expression and leading to bacterial cell death.
- Pyrazinamide (PZA): A prodrug activated by the pyrazinamidase enzyme, PZA converts into pyrazinoic acid (POA) within the TB granuloma. Although its precise mode of action remains somewhat elusive, it effectively targets bacteria hiding in these protected niches.
- Ethambutol (EMB): This bacteriostatic agent inhibits the synthesis of arabinogalactan and lipoarabinomannan, two critical components of the mycobacterial cell wall. By targeting arabinosyltransferases EmbA, EmbB, and EmbC, EMB disrupts cell wall formation.
- Adverse Effects: While this four-drug regimen is effective, it is not without complications. Patients may experience several side effects, including:
- Liver Dysfunction
- Peripheral Neuropathy
- Erythromelalgia
- Ocular Toxicity
- Central Nervous System (CNS) Toxicity
- Gastrointestinal (GI) Intolerance
- Skin Rash
- Challenges in Treatment: These side effects can lead to poor patient compliance, exacerbated by the high pill burden and extended duration of therapy. Additionally, the overuse and misuse of antibiotics contribute significantly to the emergence of drug-resistant strains of M. tb.
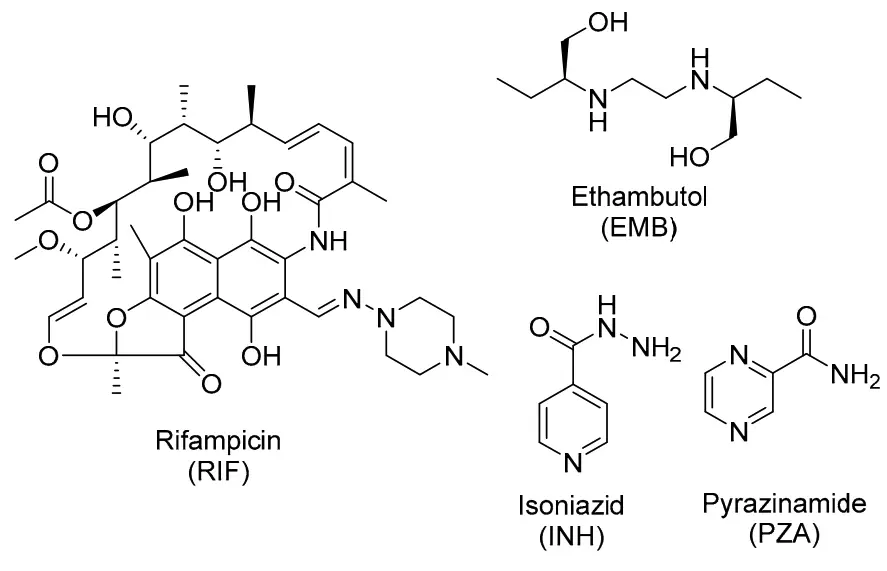
Identification of Mycobacterium tuberculosis
Identifying Mycobacterium tuberculosis is crucial for accurate diagnosis and effective treatment of tuberculosis (TB). Various laboratory techniques and biochemical tests are employed to differentiate M. tuberculosis from other mycobacterial species. This identification process relies on culturing the organism, observing its growth characteristics, and performing a series of specific tests.
- Growth Characteristics: M. tuberculosis grows slowly, typically requiring 4 to 6 weeks for noticeable colonies to form. It is weakly catalase positive and nitrate positive, with a distinct ability to reduce niacin.
- Biochemical Tests for Identification:
- Niacin Test: This test involves adding sterile normal saline to colonies of M. tuberculosis cultured in Löwenstein-Jensen (LJ) medium. After incubation, a portion of the fluid is mixed with alcoholic aniline and 10% cyanogen bromide. A positive result, indicated by a yellow color development, is absent in M. tuberculosis, as it does not produce yellow color.
- Arylsulfatase Test: This test detects the enzyme arylsulfatase. Cultures are grown in a medium containing tripotassium phenolphthalein disulfate. Following the addition of sodium hydroxide, a pink color indicates a positive reaction. M. tuberculosis is arylsulfatase negative, distinguishing it from some other mycobacterial species that are positive.
- Neutral Red Test: This test evaluates the ability of certain mycobacteria to bind neutral red dye in an alkaline solution. Following the inoculation and incubation process, a development of pink or red-stained colonies suggests a positive result. Both M. tuberculosis and M. bovis are neutral red test positive.
- Catalase Test: This assay detects the presence of the catalase enzyme. Mixing hydrogen peroxide and Tween 80 with mycobacterial colonies allows observation for bubble formation, indicating a positive result. Both M. tuberculosis and M. bovis show weakly positive results.
- Amidase Test: This test measures the ability to split amides, such as acetamide and nicotinamide. Following a series of chemical additions and incubation, a blue color indicates a positive test. M. tuberculosis is amidase positive.
- Nitrate Reduction Test: This test identifies the presence of nitrate reductase. After incubation and addition of specific reagents, the development of pink or red color indicates a positive result. M. tuberculosis is positive, in contrast to M. bovis and M. avium, which are negative.
- Tween 80 Hydrolysis Test: This test demonstrates lipase production. By observing color changes in a solution containing Tween 80, researchers can determine positive results. M. tuberculosis displays variable outcomes, unlike M. kansasii, which consistently produces a positive test within hours.
- Specialized Tests:
- NAP Differentiation Test: The addition of p-nitro acetyl-amino-hydroxyl-propionophenone (NAP) selectively inhibits the growth of M. tuberculosis, M. bovis, and M. africanum, helping to differentiate these species from others.
- DNA Probes: Nucleic acid hybridization using DNA probes offers a rapid identification method. These probes can detect the M. tuberculosis complex with 100% sensitivity and specificity when a sufficient number of bacilli are present. However, this test does not differentiate among M. tuberculosis, M. bovis, and M. africanum.
- Pyrazinamide Test: M. tuberculosis is sensitive to pyrazinamide, with a positive pyrazinamidase test, while M. bovis is negative for this enzyme.
- TCH Susceptibility Test: In this assessment, M. tuberculosis shows resistance to 10 µg/mL of thiosemicarbazone (TCH), contrasting with M. bovis, which is susceptible.
Each of these tests plays a critical role in the laboratory identification of M. tuberculosis, ensuring timely and accurate diagnosis for effective patient management.
Challenges to the Global Control of TB
Tuberculosis (TB) remains a significant global health challenge, exacerbated by various interconnected factors that hinder effective control measures. These challenges include the emergence of drug-resistant TB (DR-TB), the impact of HIV co-infection, and the repercussions of the COVID-19 pandemic. Understanding these factors is crucial for developing strategies to combat TB on a global scale.
- Drug-Resistant TB Crisis:
The rise of drug-resistant TB presents a formidable barrier to effective treatment. Multidrug-resistant TB (MDR-TB) is characterized by resistance to both isoniazid (INH) and rifampicin (RIF), the most potent first-line anti-TB medications. In 2021, an estimated 450,000 new cases of MDR-TB were reported, with cure rates substantially lower than those for drug-sensitive TB (DS-TB). The treatment for MDR-TB often involves an 18–20 month regimen that requires multiple drugs, creating a more complex treatment landscape. The inclusion of newer agents such as bedaquiline (BDQ) and delamanid (DLM) marks a significant advancement, yet these treatments are not universally accessible, limiting their efficacy against MDR-TB strains. Furthermore, extensively drug-resistant TB (XDR-TB), which resists fluoroquinolones and injectable second-line agents, drastically reduces treatment options and is associated with extremely high mortality rates. Consequently, the complexity and duration of treatment contribute to poor patient adherence, fostering ongoing transmission and perpetuating the global TB crisis. - TB and HIV Co-Infection:
Co-infection with HIV presents additional challenges to TB control. The presence of HIV dramatically increases the risk of TB progression, with co-infected individuals experiencing an 18-fold greater likelihood of developing active TB. This interplay complicates treatment regimens due to overlapping toxicities and drug interactions. For instance, RIF can significantly reduce the effectiveness of several HIV medications by inducing cytochrome P450 enzymes, which in turn accelerates the metabolism of these drugs. As a result, achieving optimal therapeutic levels becomes challenging, complicating the management of both infections and reducing adherence to treatment protocols. The need for tailored treatment plans highlights the necessity of addressing co-morbidities in TB control strategies. - Impact of the COVID-19 Pandemic:
The COVID-19 pandemic has severely impacted TB control efforts worldwide, reversing years of progress. In 2020 and 2021, there was a notable increase in TB deaths, primarily due to delays in diagnosis and treatment. Reports indicate that nearly half of the active TB cases went unreported during this period, exacerbating community transmission. The WHO estimated that TB-related deaths surged to approximately 1.6 million in 2021, compared to 1.5 million in 2020, with the highest burdens occurring in countries like India, Indonesia, the Philippines, and Myanmar. This shift underscores the urgent need to reinvigorate TB control initiatives. The WHO’s End TB Strategy aimed to achieve a 90% reduction in incidence and a 95% reduction in deaths by 2035, but the setbacks caused by COVID-19 necessitate immediate and innovative solutions to realign global TB targets.
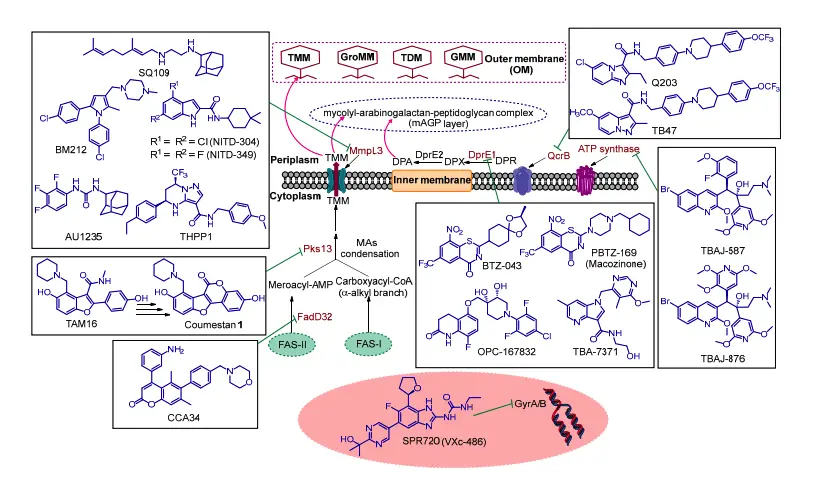
- Weekes, C., & Kotra, L. P. (2007). Mycobacterium Tuberculosis Infections. xPharm: The Comprehensive Pharmacology Reference, 1–7. doi:10.1016/b978-008055232-3.60889-x
- Smith I. Mycobacterium tuberculosis pathogenesis and molecular determinants of virulence. Clin Microbiol Rev. 2003 Jul;16(3):463-96. doi: 10.1128/CMR.16.3.463-496.2003. PMID: 12857778; PMCID: PMC164219.
- Rahlwes KC, Dias BRS, Campos PC, Alvarez-Arguedas S, Shiloh MU. Pathogenicity and virulence of Mycobacterium tuberculosis. Virulence. 2023 Dec;14(1):2150449. doi: 10.1080/21505594.2022.2150449. PMID: 36419223; PMCID: PMC9817126.
- Forrellad MA, Klepp LI, Gioffré A, Sabio y García J, Morbidoni HR, de la Paz Santangelo M, Cataldi AA, Bigi F. Virulence factors of the Mycobacterium tuberculosis complex. Virulence. 2013 Jan 1;4(1):3-66. doi: 10.4161/viru.22329. Epub 2012 Oct 17. PMID: 23076359; PMCID: PMC3544749.
- Echeverria-Valencia, G., Flores-Villalva, S., & Espitia, C. I. (2018). Virulence Factors and Pathogenicity of Mycobacterium. InTech. doi: 10.5772/intechopen.72027
- Heemskerk D, Caws M, Marais B, et al. Tuberculosis in Adults and Children. London: Springer; 2015. Chapter 2, Pathogenesis. Available from: https://www.ncbi.nlm.nih.gov/books/NBK344406/
- Alsayed SSR, Gunosewoyo H. Tuberculosis: Pathogenesis, Current Treatment Regimens and New Drug Targets. Int J Mol Sci. 2023 Mar 8;24(6):5202. doi: 10.3390/ijms24065202. PMID: 36982277; PMCID: PMC10049048.
- Alva A, Aquino F, Gilman RH, Olivares C, Requena D, Gutiérrez AH, Caviedes L, Coronel J, Larson S, Sheen P, Moore DA, Zimic M. Morphological characterization of Mycobacterium tuberculosis in a MODS culture for an automatic diagnostics through pattern recognition. PLoS One. 2013 Dec 16;8(12):e82809. doi: 10.1371/journal.pone.0082809. PMID: 24358227; PMCID: PMC3865090.
- https://www.meddean.luc.edu/lumen/meded/medicine/pulmonar/diseases/tbthc1.htm
- https://en.wikipedia.org/wiki/Mycobacterium_tuberculosis
- https://microbewiki.kenyon.edu/index.php/Mycobacterium_tuberculosis
- https://www.testing.com/tests/acid-fast-bacillus-afb-testing/
- https://www.slideshare.net/slideshow/mycobacterium-tuberculosis-seminar/10548463