What are Monosaccharides?
- Monosaccharides, also known as simple sugars, are the fundamental building blocks of carbohydrates. They are single sugar molecules that serve as the simplest forms of sugar. Monosaccharides are crucial in biology and biochemistry as they form the basis for more complex sugars like disaccharides and polysaccharides.
- The term “monosaccharide” originates from the Greek words “monos,” meaning “single” or “alone,” and “sacchar,” meaning “sugar.” Essentially, monosaccharides are carbohydrates consisting of a single saccharide unit. It is important to note that the term “sugar” can encompass both monosaccharides and disaccharides.
- Monosaccharides are often referred to as simple sugars because of their basic molecular structure. They are colorless, water-soluble, and crystalline solids. However, despite their name, not all monosaccharides possess a sweet taste. Most monosaccharides can be represented by the general formula (CH2O)n, although not all compounds with this formula are monosaccharides.
- Some examples of monosaccharides include glucose (also known as dextrose), fructose (levulose), and galactose. These monosaccharides serve as the building blocks for more complex carbohydrates such as disaccharides (e.g., sucrose and lactose) and polysaccharides (e.g., cellulose and starch). The common table sugar used in daily life, sucrose, is a disaccharide composed of two monosaccharides: glucose and fructose.
- In monosaccharides, each carbon atom that carries a hydroxyl group is chiral, except for those at the end of the carbon chain. This results in various isomeric forms, which have the same chemical formula but differ in their physical structures and chemical properties. For instance, galactose and glucose are both aldohexoses, belonging to the same category of six-carbon monosaccharides. However, they possess distinct molecular arrangements and exhibit different chemical characteristics.
- One of the most significant monosaccharides is glucose, which plays a pivotal role in metabolism. Glucose acts as a key source of chemical energy in living organisms. Through processes like glycolysis and the citric acid cycle, glucose is broken down, releasing energy that is utilized by organisms for various biological functions.
- Overall, monosaccharides are the essential building blocks of carbohydrates, serving as the simplest forms of sugar. They are diverse in structure, and their arrangements contribute to their unique properties and functions within biological systems.
Definition of Monosaccharides
Monosaccharides are single sugar molecules that are the basic units of carbohydrates. They are simple sugars with a variety of structures and functions in biological systems.
Vant Hoff’s Rule of ‘n’
The number of imaginable isomers of any delivered compound relies upon the digit of the molecule’s asymmetric carbon atoms.
Based on this principle 2n is the number of possible isomers for that compound in which ‘n’ represents the number of carbon asymmetric Atoms within the compound.
Classifications of Monosaccharides
Classifications of Monosaccharides based on number of Carbon atom
Monosaccharides, the simple sugar molecules, can be classified based on different criteria. One classification method is based on the number of carbon atoms they contain, which results in various groups:
- Triose: Trioses are monosaccharides with three carbon atoms. An example is glyceraldehyde-3-phosphate, which serves as an intermediate in carbohydrate metabolic pathways.
- Tetrose: Tetroses have four carbon atoms. Examples include D-erythrose, D-threose, and D-erythrulose. Erythrose, a tetrose with one aldehyde group, is a metabolite in the Calvin cycle and the pentose phosphate pathway. Threose and erythrulose are enantiomers of erythrose, with threose being another tetrose and erythrulose being a ketotetrose due to its ketone group.
- Pentose: Pentoses consist of five carbon atoms. Ribose and deoxyribose are well-known pentoses and are important components of nucleotides and nucleic acids. Ribose is found in RNA nucleotides, while deoxyribose is found in DNA nucleotides.
- Hexose: Hexoses have six carbon atoms and include glucose, mannose, galactose, and fructose. Glucose is a crucial hexose as it serves as a metabolic intermediate in cellular respiration. Excess glucose is stored as glycogen in animals and starch in plants.
- Heptose: Heptoses contain seven carbon atoms. Examples include L-glycero-D-manno-heptose and sedoheptulose, which play early roles in lipid A biosynthesis.
- Octose: Octoses have eight carbon atoms. An example is methylthiolincosamide, the sugar component of the antimicrobial agent lincomycin A.
- Nonose: Nonoses consist of nine carbon atoms. Neuraminic acid is a synthetic nonose, and examples of naturally occurring nonoses include sialic acid, legionaminic acid, and pseudaminic acid.
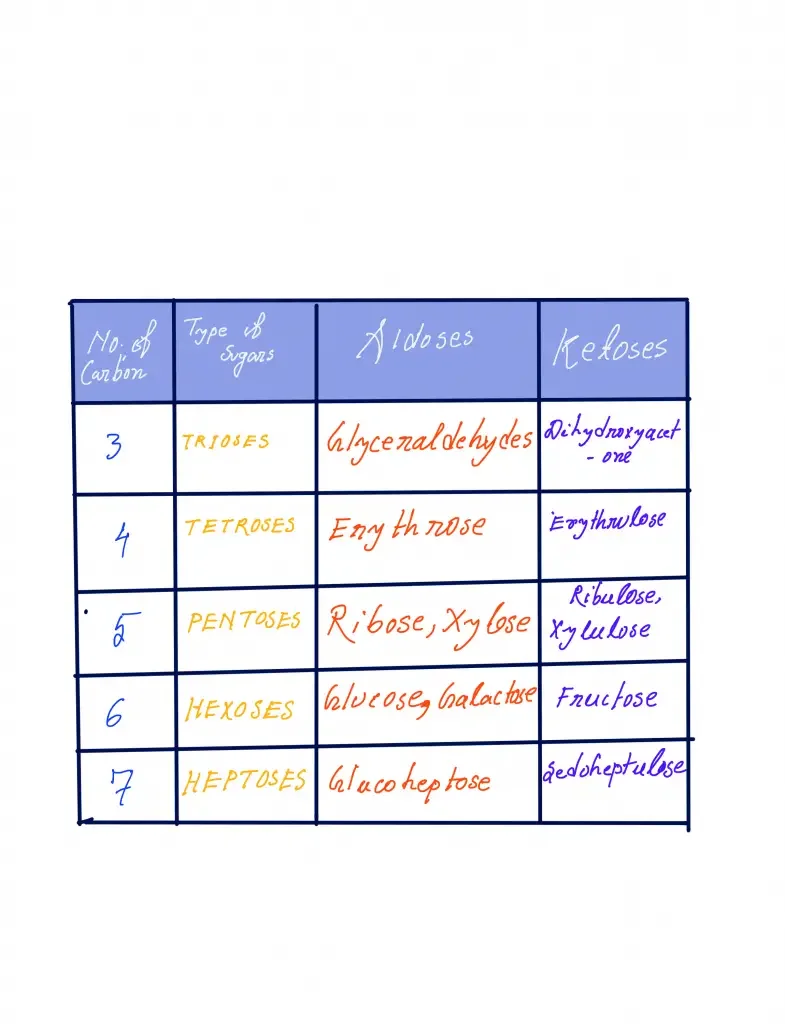
It is important to note that these are terms (e.g. triose, tetrose, pentose, etc.) are distinct from trisaccharide (tetrasaccharide), pentasacc and so on because these terms refer to the quantity of monosaccharide units within the polymer, i.e. the three monosaccharides are different from four five monosaccharides and so on.
Classifications of Monosaccharides based on the type of carbonyl group
Monosaccharides can also be classified based on the type of carbonyl group they contain:
- Aldose: An aldose is a monosaccharide that contains an aldehyde group (-CHO).
- Ketose: A ketose is a monosaccharide that contains a ketone group (C=O).
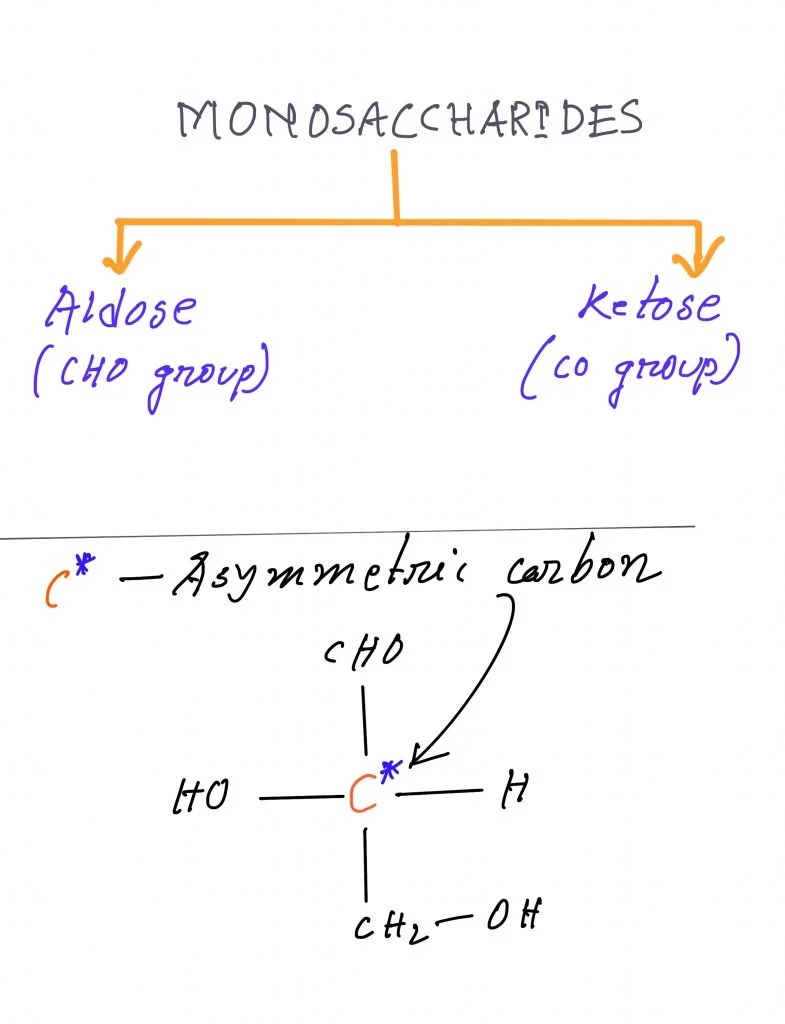
Characteristics of Monosaccharide
Monosaccharides possess several characteristic features that distinguish them within the realm of carbohydrates. These characteristics include:
- Simple Structure: Monosaccharides are the most basic and fundamental units of carbohydrates. They cannot be further broken down into simpler sugars through hydrolysis. However, they can combine with other monosaccharides to form more complex carbohydrate structures.
- Glycosidic Bonds: Monosaccharides are joined together through glycosidic bonds or glycosidic linkages. When two monosaccharides combine, they form a disaccharide. If the carbohydrate consists of three to ten monosaccharides, it is termed an oligosaccharide. Carbohydrates with a larger number of monosaccharide units are known as polysaccharides.
- Dehydration Synthesis: The process of joining monosaccharide units involves dehydration synthesis. During this process, water molecules are released as byproducts. It is important to note that this process is reversible. Complex carbohydrates can be broken down into simple sugars through processes like glycogenolysis, where stored glycogen is broken down into glucose units for energy metabolism.
- Chemical Formula and Structure: Monosaccharides have a general chemical formula of CnH2nOn, where “n” represents the number of carbon atoms. Their chemical structure is H(CHOH)nC=O(CHOH)mH. The ratio of hydrogen atoms to oxygen atoms is typically 2:1, except for deoxyribose found in DNA, which deviates from this rule. This chemical formula rule gives rise to the term “hydrates of carbon” to describe monosaccharides and other carbohydrates.
- Physical Properties: Monosaccharides are often colorless, crystalline solids. While some monosaccharides have a sweet taste, not all of them exhibit this characteristic. They can dissolve in water and may exist in the form of syrups or liquid sugar. Being organic compounds, monosaccharides contain carbon atoms covalently bonded to other atoms, particularly Carbon-Carbon (C-C) and Carbon-Hydrogen (C-H) bonds.
These characteristics collectively define monosaccharides as the simplest sugars and building blocks of more complex carbohydrates. Their chemical and structural properties contribute to their diverse functions in biological systems.
Structure of Monosaccharides
The structure of monosaccharides is characterized by their carbon backbone, functional groups, and the arrangement of atoms. Here is a description of the structure of monosaccharides:
- General Formula: Monosaccharides have the general formula (CH2O)n, where “n” represents the number of carbon atoms in the molecule. This formula indicates that monosaccharides consist of carbon, hydrogen, and oxygen atoms in specific proportions.
- Carbon Backbone: Monosaccharides have a chain-like structure composed of carbon atoms. Each carbon atom can form up to four bonds, allowing multiple carbon atoms to link together. The carbon backbone provides the foundation for the monosaccharide structure.
- Hydroxyl Groups: Along the carbon backbone, hydroxyl groups (-OH) are attached to most of the carbon atoms. These hydroxyl groups are formed when oxygen bonds with hydrogen, contributing to the overall polarity of the molecule.
- Carbonyl Group: One of the carbon atoms in the monosaccharide chain forms a double bond with an oxygen atom, creating a carbonyl group (C=O). The position of the carbonyl group determines whether the monosaccharide belongs to the aldose or ketose family.
- Aldose: If the carbonyl group is located at the end of the carbon chain, the monosaccharide is classified as an aldose. The aldehyde functional group (-CHO) is present in aldose monosaccharides.
- Ketose: When the carbonyl group is positioned within the carbon chain, the monosaccharide is classified as a ketose. The ketone functional group (C=O) is present in ketose monosaccharides.
- Ring Structure: In aqueous solutions, monosaccharides with more than five carbon atoms often adopt a ring structure. This occurs through intramolecular reactions where a hydroxyl group on one carbon atom reacts with the carbonyl group, forming a cyclic structure. The resulting ring structure contributes to the stability of the monosaccharide molecule.
- Glucose Example: Glucose, a common monosaccharide, is composed of six carbon atoms. The first carbon atom in glucose forms the carbonyl group, making it an aldose. In an aqueous solution, glucose typically exists in a ring structure. The hydroxyl group on the fifth carbon reacts with the carbonyl group, forming a stable ring structure.
Overall, the structure of monosaccharides consists of a carbon backbone, hydroxyl groups attached to the carbon atoms, a carbonyl group determining the type of monosaccharide, and the potential for ring formation in aqueous solutions. The specific arrangement of atoms in monosaccharides plays a crucial role in their biological functions and interactions.
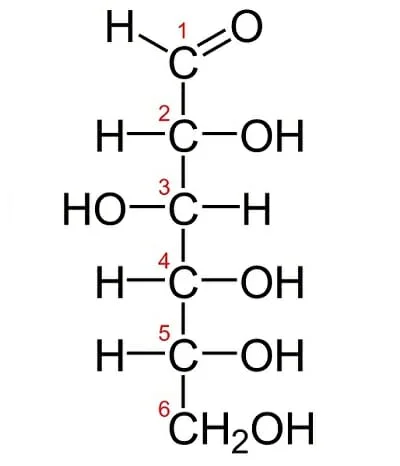
Linear-chain monosaccharides
Linear-chain monosaccharides are simple carbohydrates with a straight and unbranched carbon backbone. Here’s an explanation of their structure:
- Carbon Skeleton: Linear-chain monosaccharides have a carbon backbone without any branches. This backbone consists of carbon atoms, with each carbon atom bonded to a hydroxyl group (-OH), except for one carbon atom that forms a carbonyl group (C=O). The remaining hydroxyl group on each carbon completes the structure.
- Molecular Formula: The molecular structure of a linear-chain monosaccharide can be represented as H(CHOH)n(C=O)(CHOH)mH, where “n” and “m” represent the number of hydroxyl groups on the respective carbon atoms, and “x” represents the total number of carbon atoms in the molecule. The sum of n + 1 + m is equal to x, and the elemental formula of the monosaccharide is CxH2xOx.
- Numbering of Carbon Atoms: Conventionally, the carbon atoms in a linear-chain monosaccharide are numbered from 1 to x along the backbone. The numbering starts from the end closest to the carbonyl group (C=O).
- Aldose and Ketose: Linear-chain monosaccharides can be classified as either aldoses or ketoses based on the position of the carbonyl group.
- Aldose: If the carbonyl group is at position 1, the monosaccharide is an aldose. In this case, the compound starts with a formyl group H(C=O)− and is technically an aldehyde.
- Ketose: If the carbonyl group is between two carbon atoms, the monosaccharide is a ketose. The carbonyl group is typically located at position 2 in ketoses of biological interest.
- Greek Prefix Nomenclature: Another nomenclature system for linear-chain monosaccharides uses Greek prefixes to indicate the number of carbon atoms in the molecule (tri-, tetr-, pent-, hex-, etc.). The suffix “-ose” is used for aldoses, and “-ulose” is used for ketoses. If the carbonyl group is not at position 2 in a ketose, its specific position is indicated by a numeric infix.
- Example: H(C=O)(CHOH)4H is a pentose, H(CHOH)(C=O)(CHOH)3H is a pentulose, and H(CHOH)2(C=O)(CHOH)2H is a pent-3-ulose.
By combining these classifications, monosaccharides can be named based on the number of carbon atoms and the presence of the carbonyl group, resulting in names like “aldohexose” and “ketotriose.”
In summary, linear-chain monosaccharides have a simple structure consisting of a straight carbon backbone with hydroxyl groups attached to most carbon atoms and a carbonyl group at either position 1 (aldose) or position 2 (ketose). The nomenclature of linear-chain monosaccharides can be based on the number of carbon atoms, the presence of the carbonyl group, and the specific position of the carbonyl group in ketoses.
Open-chain stereoisomers
- Open-chain stereoisomers refer to distinct spatial arrangements of monosaccharides that have the same chain length and carbonyl position but differ in their three-dimensional orientation. The presence of a stereogenic center, specifically a chiral carbon atom bonded to four different molecular sub-structures, is essential for the existence of open-chain stereoisomers.
- In a simple open-chain monosaccharide, every carbon except the first and last atoms of the chain (and the carbon with the keto group in ketoses) is chiral and capable of forming open-chain stereoisomers. The number of chiral carbons increases with the length of the monosaccharide chain.
- Let’s consider some examples to illustrate this concept. Glycerone, also known as dihydroxyacetone, is a triketose monosaccharide with no stereogenic center. As a result, it exists as a single stereoisomer.
- On the other hand, glyceraldehyde, a triose aldose monosaccharide, possesses one chiral carbon atom (carbon 2) bonded to four distinct groups: -H, -OH, -C(OH)H2, and -(C=O)H. Therefore, glyceraldehyde exists as two stereoisomers, which are mirror images of each other, similar to a left and a right glove.
- As the number of carbon atoms increases, monosaccharides can have multiple chiral carbons, leading to a greater number of stereoisomers. The maximum number of distinct stereoisomers with the same structural diagram is limited by 2c, where c represents the total number of chiral carbons.
- To accurately represent the spatial arrangement and handedness of each chiral carbon in an acyclic monosaccharide, the Fischer projection is commonly used. In this projection, the positions (right or left) of the chiral hydroxyl groups (attached to the chiral carbons) indicate different stereoisomers.
- Most stereoisomers are chiral themselves and differ from their mirror images. In the Fischer projection, mirror-image isomers can be obtained by reversing the positions of all chiral hydroxyl groups from right to left. While mirror-image isomers are chemically identical in non-chiral environments, they often exhibit distinct biochemical properties and occur differently in nature.
- However, there are some non-chiral stereoisomers that are identical to their mirror images due to molecular graph symmetry. For example, 3-ketopentoses with a symmetrical molecular graph have two halves that are mirror images of each other. Thus, there are only three distinct 3-ketopentose stereoisomers despite having two chiral carbons.
- Distinct stereoisomers that are not mirror images of each other typically have different chemical properties even in non-chiral environments. Therefore, specific monosaccharide names are assigned to each mirror pair and non-chiral stereoisomer. For instance, the name “glucose” refers to a specific pair of mirror-image aldohexoses. In the Fischer projection, one glucose isomer has the hydroxyl on the left side of C3 and on the right side of C4 and C5, while the other isomer has the reverse pattern. These monosaccharide names are often abbreviated using three-letter codes, such as “Glu” for glucose and “Thr” for threose.
- In general, a monosaccharide with n asymmetrical carbons can have 2n stereoisomers. The number of open-chain stereoisomers for an aldose monosaccharide is one more than that of a ketose monosaccharide of the same chain length. For ketoses with more than two carbons (n > 2), there are 2(n−3) stereoisomers, while aldoses with more than two carbons (n > 2) have 2(n−2) stereoisomers. These stereoisomers are also known as epimers, representing different arrangements of -OH and -H groups at the chiral carbon atoms (excluding carbons with the carbonyl functional group).
Configuration of monosaccharides
- Open-chain stereoisomers exhibit distinct optical activity, causing a rotation in the polarization direction of linearly polarized light as it passes through them. This phenomenon is observed in glyceraldehyde, which exists as two stereoisomers: d-glyceraldehyde and l-glyceraldehyde. The d- and l- prefixes are used to identify these stereoisomers based on their rotational direction of polarized light.
- D-glyceraldehyde is dextrorotatory, meaning it rotates the polarization axis clockwise. On the other hand, l-glyceraldehyde is levorotatory, rotating the polarization axis counterclockwise. This optical activity arises due to the chirality of the molecule, specifically the arrangement of substituents around the chiral carbon furthest from the C=O group.
- The d- and l- prefixes are also employed to distinguish between other monosaccharide stereoisomers that are mirror images of each other. To determine the prefix, one focuses on the chiral carbon that is farthest from the C=O group. The four groups attached to this carbon must correspond to -H, -OH, -C(OH)H, and the remaining part of the molecule. If the molecule can be spatially rotated to align these groups in a manner similar to d-glyceraldehyde’s C2 configuration, it is assigned the d- prefix. Otherwise, it is assigned the l- prefix.
- In the Fischer projection, the d- and l- prefixes specifically indicate the configuration at the second-bottom carbon. If the hydroxyl group is on the right side, it is denoted as d-. Conversely, if the hydroxyl group is on the left side, it is labeled as l-.
- It is important to note that the d- and l- prefixes do not directly indicate the direction of rotation of polarized light. The rotation is a combined effect of the arrangement at all chiral centers within the molecule. However, it is worth noting that the two enantiomers will always rotate the light in opposite directions, albeit by the same magnitude. The d/l system provides a means to describe the stereochemistry of monosaccharides in relation to their optical activity.
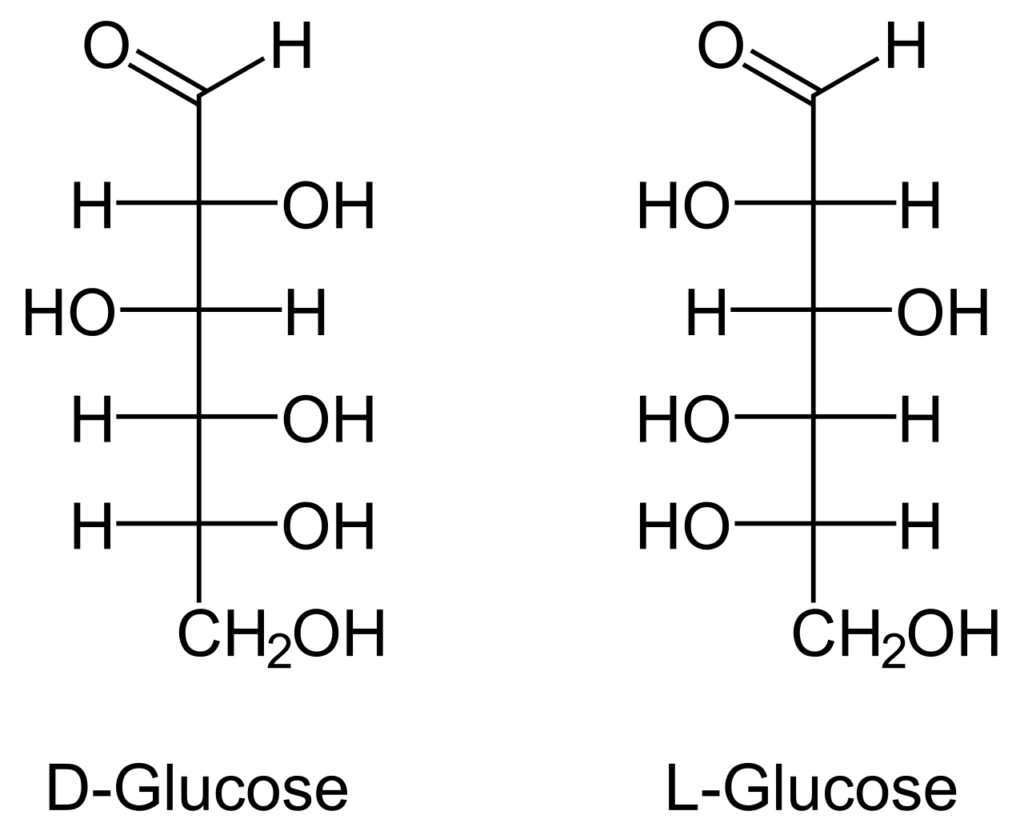
Cyclisation of monosaccharides (hemiacetal formation)
- When monosaccharides undergo a nucleophilic addition reaction between the carbonyl group and one of the hydroxyl groups within the same molecule, they can undergo cyclization, transitioning from their acyclic (open-chain) form to a cyclic form. This reaction results in the formation of a ring structure composed of carbon atoms, with a bridging oxygen atom closing the ring. The cyclic form of the molecule contains a hemiacetal or hemiketal group, depending on whether the linear form was an aldose or a ketose, respectively. It’s important to note that this cyclization reaction is reversible, allowing the molecule to convert back to its original open-chain form.
- The cyclic forms of monosaccharides typically adopt five- or six-membered rings, known as furanoses and pyranoses, respectively. These names are derived from furan and pyran, which are simple compounds sharing the same carbon-oxygen ring structure (although without the double bonds found in furan and pyran). For instance, the aldohexose glucose can undergo a hemiacetal linkage between the aldehyde group on carbon 1 and the hydroxyl group on carbon 4, resulting in the formation of a 5-membered ring known as glucofuranose. Similarly, glucose can form a hemiacetal linkage between carbons 1 and 5, resulting in a 6-membered ring called glucopyranose. Less commonly encountered are cyclic forms with a seven-atom ring, known as heptoses.
- In many cases, the cyclic forms of monosaccharides, including glucose, are more predominant in both solid-state and solution environments. As a result, the same name is often used to refer to both the open-chain and closed-chain isomers. For example, the term “glucose” can refer to glucofuranose, glucopyranose, the open-chain form, or a mixture of the three.
- Cyclization of monosaccharides introduces a new stereogenic center at the carbon atom carrying the carbonyl group. The hydroxyl group that replaces the oxygen of the carbonyl group can assume two distinct positions relative to the ring’s midplane. Consequently, each open-chain monosaccharide can yield two cyclic isomers, known as anomers, distinguished by the prefixes α- and β-. The interconversion between these two forms is facilitated by a process called mutarotation, which involves the reversal of the ring-forming reaction followed by the formation of a new ring.
- Overall, the cyclization of monosaccharides allows for the formation of cyclic structures, introducing new stereogenic centers and resulting in the coexistence of multiple isomeric forms with different ring sizes and configurations.
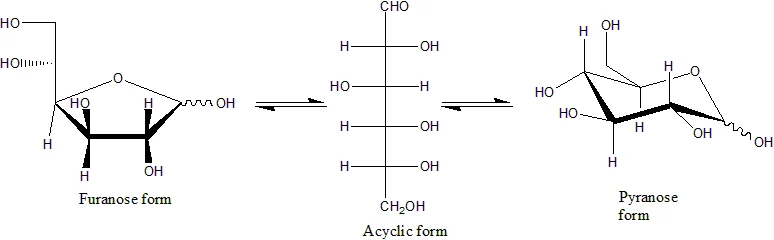

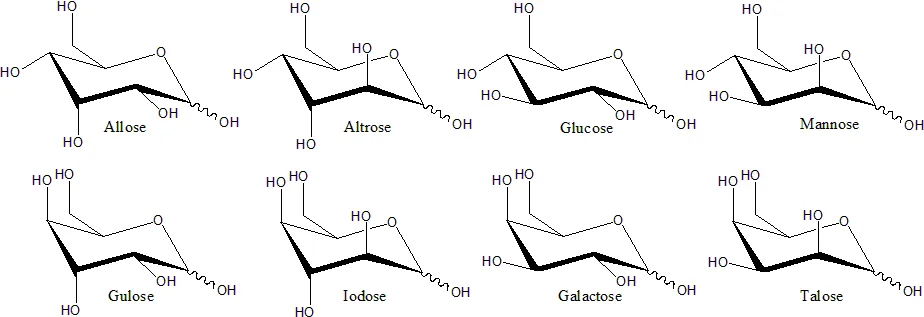
Haworth projection
- The Haworth projection is a graphical representation used to depict the stereochemical structure of cyclic monosaccharides. It provides a concise and intuitive way to visualize the spatial arrangement of atoms within the sugar ring. Specifically, the Haworth projection is commonly used to represent the pyranose form of d-aldohexoses.
- In a Haworth projection, the α-isomer of a pyranose has the hydroxyl group (−OH) attached to the anomeric carbon below the plane of the carbon atoms in the ring structure. On the other hand, the β-isomer positions the hydroxyl group above the plane of the carbon atoms. It’s important to note that the anomeric carbon is the carbon atom that becomes chiral due to the cyclization of the monosaccharide.
- Pyranose rings typically adopt a chair conformation, similar to the conformation of cyclohexane. In this chair conformation, the α-isomer positions the hydroxyl group of the anomeric carbon in an axial position relative to the ring. In contrast, the β-isomer places the hydroxyl group of the anomeric carbon in an equatorial position. It’s worth mentioning that these descriptions apply specifically to d-aldohexose sugars.
- The Haworth projection simplifies the representation of cyclic monosaccharides, allowing for a clearer visualization of the ring structure and the relative positions of substituents. By utilizing this projection, chemists and researchers can easily communicate and interpret the spatial arrangements of sugar molecules, aiding in the understanding of their biological and chemical properties.
- In summary, the Haworth projection is a useful tool for depicting the stereochemical structure of cyclic monosaccharides, particularly the pyranose forms of d-aldohexoses. It enables the clear visualization of the hydroxyl group’s orientation relative to the carbon ring, providing valuable insights into the three-dimensional structure of these important biomolecules.
Reactions Of Monosaccharides
1. Tautomerization or enolization
The process of moving hydrogen atoms to one carbon atom another in order to create enediols is called automerization. Sugars that have an omeric carbon atom are subject to tautomerization within alkaline solutions. When glucose is placed in alkaline solution for some time the sugar undergoes isomerization to produce D-fructose as well as D-mannose. This reaction– known as the Lobry de Bruyn-von Ekenstein transformation–results in the formation of a common intermediate–namely enediol–for all the three sugars. The enediols are extremely reactive so sugars in an alkaline solutions can be powerful reducers.
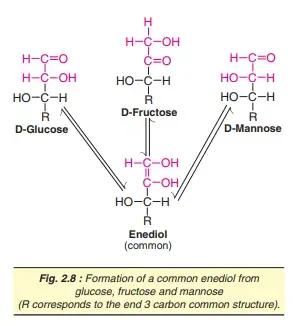
2. Reducing properties
They are classified by their ability to be either reducing or non-reducing. The property of reducing is attributed by the aldehyde free, or keto group of carbon anomeric. In the lab, a variety of tests are conducted to discover the action of sugars on reducing. They include Benedict’s test the Fehling’s test and Barfoed’s tests etc. The reduction is far more effective in the alkaline medium than the acid medium. The enediol form (explained earlier) or sugars convert cupric Ions (Cu2+) of copper sulphate cupsrous Ions (Cu+) and result in a yellow precipitate of cuprous hydroxide, or an orange crystal of copper oxide, as illustrated in the next. It should be noted that the reduction property of sugars does not allow for the identification of particular sugar since it is an all-encompassing reaction.
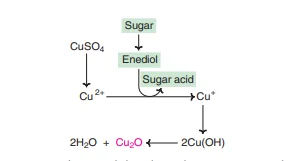
3. Oxidation
In the event of an agent used for oxidation and the type of oxidizing agent used, the terminal aldehyde (or keto) or the terminal alcohol, or both of them can be converted to oxidation. For instance, consider glucose : 1. Oxidation of the group aldehyde (CHO = COOH) leads to the formation of gluconic acid.
2. Oxidation of the terminal alcohol groups (CH2OH = COOH) results in creation of glucuronic acids.
4. Reduction
If used in conjunction with reducing agents such for sodium amalgam, the aldehyde or keto monosaccharide groups are reduced to alcohol of the same type as shown by the general formula
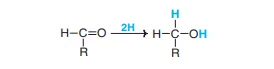
The principal monosaccharides and their alcohols are described below.
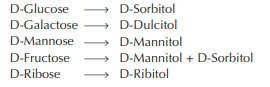
Sorbitol and dulcitol , when accumulated in tissues in huge amounts create strong osmotic reactions leading to cell swelling, and also certain pathological conditions. e.g. cataract, peripheral neuropathy, nephropathy. Mannitol can be used to decrease the intracranial pressure through forced diuresis.
5. Dehydration
In the event of treatment with sulfuric acid concentrated, the bmonosaccharides are dehydrated with an
removal of three water molecules. Therefore, hexoses produce furfural hydroxymethyl while pentoses provide furfural upon dehydration. These furfurals may condense to phenolic substances (D-naphthol) to create colour-changing products. This is the chemical foundation of the well-known Molisch test. In the case of oligo- and polysaccharides they are first hydrolyzed to monosaccharides using acid. This is later followed by dehydration.
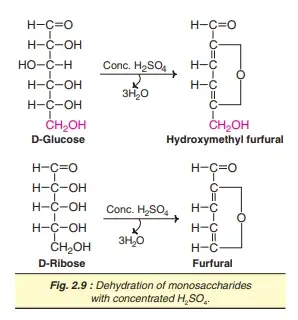
- Bial’s test: Pentoses react with powerful HCl to create furfural derivatives that then react with orcinol and form a green colored complexes. Bial’s test can be useful to detect xylose in urine when there is a need for pentosuria.

- Mucic acid test: Galactose is treated by nitric acids creates insoluble crystals of mucic acid.
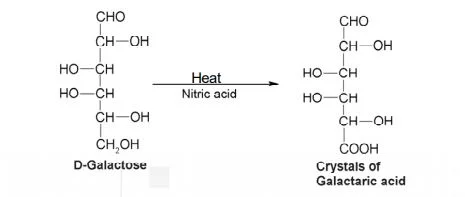
6. Osazone formation
Phenylhydrazine, a component of acetic acid when heated with sugars that reduce and forms osazones during an reaction. Based on it, two first carbons (C1 and C2) are the ones involved as part of osazone formation. The sugars that differ in arrangement on these carbons provide the same type of osazones. This is because the differences are concealed by binding to the phenylhydrazine. Therefore, fructose, glucose and mannose are the same kind of (needle-shaped) Osazones. Reduced disaccharides also produce the osazones maltose sunflower and lactose-style powderpuffs.
7. Formation of esters
Monosaccharides’ alcoholic groups are often esterified using either enzymatic or non-enzymatic processes. Esterification of carbohydrate by the phosphoric acid is a typical reaction in the metabolism. The glucose 1-phosphate and glucose 6-phosphate are excellent examples. ATP contributes the phosphate moiety during the formation of ester.
Derivatives Of Monosaccharides
There are many monosaccharides’ derivatives, some of them are essential to our health.
- Sugar acids: Oxidation of aldehyde or the primary alcohol group of monosaccharides produces sugar acids. Gluconic acid is created by the oxidation process of the aldehyde (C1 group) and glucuronic acids are produced when the principal alcohol group (C6) is converted to C6.
- Sugar alcoho: Sugar alcohols (polyols) are created by the reduction of ketoses or aldoses. For example, sorbitol can be created by removing glucose and mannitol, which is derived from mannose.
- Alditol: The monosaccharides when reduced, give polyhydroxy alcohols that are known as alditols. Ribitol is one of the constituents of flavin coenzymes. Glycerol and myo-inositol form the basis of the lipids. Xylitol is a sweetener that is used in sugar-free gums and sweets.
- Amino sugars: When one or more hydroxyl group of monosaccharides are substituted by amino-groups, the compounds created include amino sugars e.g. D-glucosamine, D-galactosamine. They are present as constituents of heteropolysaccharides. N-Acetylneuraminic Acid (NANA) is an acetylmannose derivative and the pyruvic acids. It is a key component of glycolipids and glycoproteins. The term”sialic acid” can be utilized to refer to NANA and its derivatives. Certain antibiotics have amino sugars, which could be associated with the activity of antibiotics, e.g. erythromycin.
- Desoxysugars: They are sugars with one oxygen lower than the one found within the parent molecules. The groups CHOH and Ch2OH are transformed into CH2 and then CH3 because of their absence of oxygen. D-2-Deoxyribose, the most crucial deoxysugar as it’s an essential structural component that is a component of DNA (in contrast to D-ribose found in the RNA). Feulgen staining can detect deoxyribose, which is DNA within tissues. Fucose can be described as a deoxy L-galactose that is found on blood groups antigens as well as certain glycoproteins.
- L-Ascorbic Acid (vitamin C): It is a water-soluble vitamin its structure closely is similar to that of a monosaccharide.
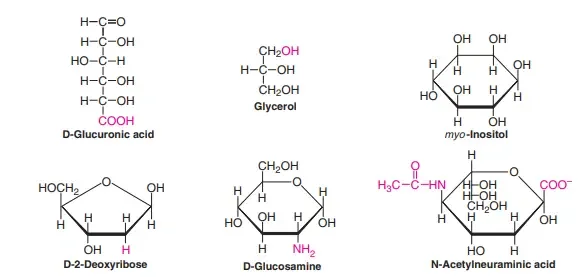
Glycosides
Glycosides form by the hemiacetal or the hemiketal hydroxyl groups (of the anomeric carbon) of one carbohydrate is reacted with a carbohydrate’s hydroxyl group or noncarbohydrate (e.g. an ethanol, methyl, or or glycerol). The bond created is known as glycosidic bond , and the non-carbohydrate component (when there is) is called an aglycone.
Monosaccharides are bonded through glycosidic bonds and result as di- or oligo, or polysaccharides (see the next section for details of structures). Naming of glycosidic bonds : The naming of glycosidic bonds relies on the links between carbon atoms as well as the state of the carbon atoms that are anomeric (D or E). For instance, lactose, which is formed by a link between C1 of E-galactose as well as C4 of glucose is referred to as E(1 or 4) glycosidic bond. The other glycosidic bond types are defined within the structure of polysaccharides and disaccharides.
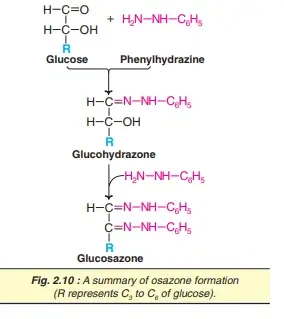
Physiologically important glycosides
- Glucovanillin (vanillin-D-glucoside) is a natural substance that imparts vanilla flavour.
- The Cardiac Glycosides (steroidal glycosides) : Digoxin and digitoxin contain the steroid aglycone, and also enhance the contraction of muscles.
- Streptomycin is an antibiotic that is used to treat tuberculosis, is glycoside.
- Ouabain hinders Na+ K+ ATPase and stops the transport activity for Na+.
- Phlorhizin can cause renal damage in laboratory animals.
Examples of Common Monosaccharides
Common monosaccharides include fructose, glucose, and galactose. These monosaccharides are considered dietary sugars as they are readily absorbed by the small intestines. They all have a hexose structure, meaning they consist of six carbon atoms with the chemical formula C6H12O6. Glucose and galactose are classified as aldoses, while fructose is a ketose.
- Glucose: Glucose is a ubiquitous monosaccharide found in nature. It can combine with other glucose units to form disaccharides such as maltose, lactose, and sucrose. Glucose is produced through photosynthesis in plants and is stored as starch or used to build cellulose and amylopectin. It serves as a vital metabolic intermediate in cellular respiration and is a major source of energy. In animals, glucose circulates in the blood and is often referred to as blood sugar. Excess glucose in animals is stored as glycogen.
- Galactose: Galactose shares a similar chemical structure with glucose but has a different orientation of hydroxyl and hydrogen atoms on carbon 4. Galactose is typically not found in a free state but rather as a component of complex biomolecules. It combines with glucose to form lactose, the disaccharide found in milk. Lactose is made up of galactose and glucose linked by a glycosidic bond. Galactose catabolism occurs through the Leloir pathway, where it is converted into glucose.
- Fructose: Fructose is known for its sweet taste and is considered the sweetest naturally occurring carbohydrate. It is found in honey, fruits, and sugar cane. Fructose is a ketonic monosaccharide, distinguished by its carbonyl group located at carbon 2. In contrast, glucose has its carbonyl group at carbon 1. Fructose occurs freely in plants or can be bonded with glucose to form the disaccharide sucrose.
These three monosaccharides, fructose, glucose, and galactose, are important components of our diet and play significant roles in energy metabolism and various biochemical processes within the body.
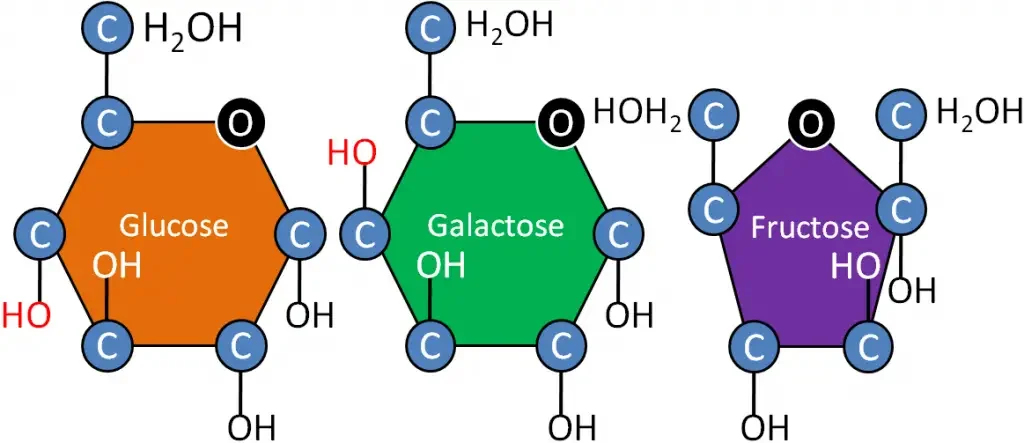
Metabolic Pathways Involving Monosaccharides
Monosaccharides play crucial roles in various metabolic pathways within the body. Some of the important metabolic pathways involving monosaccharides are:
- Glycolysis: Glycolysis is a fundamental metabolic pathway that involves the conversion of a monosaccharide, typically glucose, into pyruvate. This process occurs in the cytoplasm of cells and produces high-energy molecules such as ATP and NADH. Glycolysis is an essential pathway for energy production and is utilized by both aerobic and anaerobic organisms.
- Pentose Phosphate Pathway: The pentose phosphate pathway, also known as the hexose monophosphate shunt, is an alternative metabolic route for breaking down glucose. It generates important metabolic intermediates, such as ribose-5-phosphate, which is essential for nucleotide synthesis and other cellular processes. Additionally, this pathway produces NADPH, a reducing agent used in various biosynthetic reactions and antioxidant defense systems.
- Gluconeogenesis: Gluconeogenesis is the metabolic pathway through which non-carbohydrate precursors, such as amino acids and glycerol, are converted into glucose. This process primarily occurs in the liver and kidneys and serves as a means of maintaining blood glucose levels during fasting or prolonged periods without dietary carbohydrates. Gluconeogenesis is crucial for providing glucose as a fuel source for the brain and other glucose-dependent tissues.
- Glycogenolysis: Glycogenolysis is the breakdown of glycogen, the storage form of glucose, into individual glucose monomers. This process is regulated by the hormone glucagon and occurs mainly in the liver and skeletal muscle. Glycogenolysis allows for the release of glucose into the bloodstream, providing an immediate source of energy during times of increased demand or low blood glucose levels.
- Glycogenesis: Glycogenesis is the synthesis of glycogen from glucose molecules. It serves as a mechanism for storing glucose in the liver and skeletal muscle for later use. Glycogenesis is stimulated by the hormone insulin, which promotes glucose uptake and glycogen synthesis in response to elevated blood glucose levels.
- Fructose Metabolism: Fructose metabolism involves the utilization of fructose, a monosaccharide found in fruits and other natural sources. Fructose is metabolized differently than glucose and enters the glycolytic pathway at a later stage. It is first converted into fructose-1-phosphate and then further metabolized to produce intermediates that can be used for energy production.
- Galactose Metabolism: Galactose metabolism involves the breakdown and utilization of galactose, another monosaccharide commonly found in milk and dairy products. Galactose enters the glycolytic pathway through a series of enzymatic reactions. It is first phosphorylated to galactose-1-phosphate and then converted into glucose-6-phosphate, which can be further metabolized for energy production.
These metabolic pathways illustrate the diverse roles of monosaccharides in energy production, glucose homeostasis, and the synthesis of important cellular components. They ensure the efficient utilization and regulation of monosaccharides within the body, contributing to overall metabolic health.
Functions Of Monosaccharides
Monosaccharides, as simple sugars, serve a variety of essential biological functions in living organisms. Here are some of the key biological functions of monosaccharides:
- Energy Source: Monosaccharides, particularly glucose, are a primary source of energy for cells. Through cellular respiration, glucose is broken down to produce ATP (adenosine triphosphate), which is the energy currency of cells. Monosaccharides are metabolized in various metabolic pathways to generate energy necessary for cellular processes, growth, and maintenance.
- Structural Components: Monosaccharides contribute to the structure and function of various biological molecules. For example, they are integral components of nucleotides, the building blocks of DNA and RNA. Ribose and deoxyribose, both pentose monosaccharides, are key constituents of nucleotides, forming the backbone of nucleic acids.
- Glycosylation: Monosaccharides are involved in glycosylation, the process of attaching sugar molecules to proteins or lipids. This post-translational modification plays critical roles in protein folding, stability, cellular recognition, and signaling. Glycoproteins and glycolipids, which have monosaccharides attached to them, participate in diverse biological functions, including cell adhesion, immune response, and hormone signaling.
- Cell Recognition and Signaling: Monosaccharides are integral to cell recognition and signaling processes. Cell surface carbohydrates, such as glycoproteins and glycolipids, display specific patterns of monosaccharides that serve as recognition markers. These markers are involved in cell-cell interactions, immune response, pathogen recognition, and tissue development.
- Storage and Mobilization of Energy: Monosaccharides play a role in storing and mobilizing energy reserves in the form of glycogen. In animals, excess glucose is converted into glycogen and stored primarily in the liver and muscles. When energy demands increase, glycogen is broken down through glycogenolysis, releasing glucose monomers for energy production.
- Osmotic Regulation: Monosaccharides help regulate osmotic balance in cells and tissues. They contribute to the osmotic potential of biological fluids, affecting the movement of water and ions across cellular membranes. Maintaining proper osmotic balance is crucial for cell function and overall physiological homeostasis.
- Precursors for Biosynthesis: Monosaccharides serve as precursors for the biosynthesis of various biomolecules. They provide carbon skeletons and functional groups for the synthesis of amino acids, fatty acids, nucleotides, and other complex molecules necessary for cell growth, repair, and maintenance.
Overall, monosaccharides play vital roles in energy metabolism, structural integrity, cell-cell recognition, signaling processes, and the synthesis of essential biomolecules. Their diverse functions contribute to the proper functioning and homeostasis of living organisms.
FAQ
What are monosaccharides?
Monosaccharides are the simplest form of carbohydrates, often referred to as simple sugars. They are single sugar units that cannot be further hydrolyzed to yield simpler sugars.
What is the general formula of monosaccharides?
Monosaccharides have the general formula (CH2O)n, where “n” represents the number of carbon atoms in the molecule.
What are some examples of monosaccharides?
Common examples of monosaccharides include glucose, fructose, galactose, ribose, and deoxyribose.
What is the difference between aldoses and ketoses?
Aldoses are monosaccharides that contain an aldehyde functional group (-CHO), while ketoses contain a ketone functional group (C=O) within their carbon chain.
How do monosaccharides exist in solution?
Monosaccharides can exist in both open-chain and cyclic forms in solution due to their ability to undergo intramolecular reactions, such as hemiacetal or hemiketal formation.
What is the significance of chiral carbons in monosaccharides?
Chiral carbons are carbon atoms in monosaccharides that are bonded to four different groups. They contribute to the formation of stereoisomers, allowing for the existence of different spatial arrangements of monosaccharide molecules.
How are monosaccharides classified based on the number of carbon atoms?
Monosaccharides are classified as trioses (3 carbon atoms), tetroses (4 carbon atoms), pentoses (5 carbon atoms), hexoses (6 carbon atoms), and so on, depending on the number of carbon atoms present in the molecule.
What are anomers in monosaccharides?
Anomers are cyclic stereoisomers of monosaccharides that differ in the spatial orientation of the hydroxyl group attached to the anomeric carbon, which is the carbon involved in ring closure.
What is the role of monosaccharides in living organisms?
Monosaccharides serve as a vital energy source in cells, participating in metabolic processes like glycolysis and cellular respiration. They also play structural roles, such as forming polysaccharides like cellulose and chitin.
Can monosaccharides be found naturally in foods?
Yes, monosaccharides are found naturally in various foods. For example, fruits contain fructose, honey contains a mixture of glucose and fructose, and milk contains lactose (composed of glucose and galactose).
References
- BeMiller, James N. (2019). Carbohydrate Chemistry for Food Scientists || Monosaccharides. , (), 1–23. doi:10.1016/B978-0-12-812069-9.00001-7
- Yahia, Elhadi M. (2019). Postharvest Physiology and Biochemistry of Fruits and Vegetables || Carbohydrates. , (), 175–205. doi:10.1016/B978-0-12-813278-4.00009-9
- http://what-when-how.com/glycoconjugates-and-carbohydrates/monosaccharides-part-1-glycoconjugates-and-carbohydrates/
- https://www.brainkart.com/article/Monosaccharides_27771/
- https://biochemden.com/monosaccharide-classify/
- https://theory.labster.com/monosaccharides/
- https://noteshippo.com/derivatives-of-monosaccharides-functions-and-importance/
- http://chemistry2.csudh.edu/rpendarvis/monosacch.html
- https://www.nutrientsreview.com/carbs/monosaccharides-simple-sugars.html
- https://www.studysmarter.de/en/explanations/biology/biological-molecules/monosaccharides/
- https://www.toppr.com/guides/chemistry/biomolecule/monosaccharides/
- https://www.news-medical.net/health/Carbohydrate-Monosaccharides.aspx
- https://www.biologyonline.com/dictionary/monosaccharide
- https://biologydictionary.net/monosaccharide/
- https://www.thoughtco.com/monosaccharide-definition-and-functions-4780495
best article i have read so far on this matter!keep it up