Microbiology of extreme environments focuses on the study of microorganisms that are capable of surviving and thriving in these harsh and inhospitable conditions. These microorganisms have evolved unique adaptations that allow them to withstand extreme temperatures, pressures, pH levels, salinity, and radiation exposure. Here are some key points about the microbiology of extreme environments:
- Extremophiles: Microorganisms found in extreme environments are often referred to as extremophiles. They have developed specialized mechanisms to tolerate or even utilize the extreme conditions in their habitats.
- Cold Environments: Microorganisms found in extremely cold environments, such as polar regions or deep ocean niches, are known as psychrophiles. They have adapted to survive and grow in temperatures below 5°C. Some examples include bacteria, fungi, and archaea that can thrive in the icy waters of Antarctica or the Arctic.
- Hot Environments: Microorganisms inhabiting hot environments, such as geothermal areas or deep-sea vents, are known as thermophiles. They can tolerate temperatures above 45°C and may even require high temperatures for their metabolic processes. Thermophilic archaea, bacteria, and some fungi are capable of thriving in these extreme heat conditions.
- Extreme pH Environments: Acidic or alkaline environments pose significant challenges to most organisms. However, acidophiles can thrive in extremely acidic environments with a pH below 5, while alkaliphiles can survive in environments with a pH above 9. These microorganisms have unique enzymes and cell membrane structures that allow them to maintain cellular function under extreme pH conditions.
- Hypersaline Environments: Microorganisms in hypersaline environments, such as salt flats or salt lakes, have adapted to high salt concentrations. Halophiles can withstand salt concentrations greater than that of seawater (greater than 3.5% salinity) by regulating their internal osmotic balance and utilizing compatible solutes.
- High-Pressure Environments: Deep-sea ecosystems and subsurface habitats experience extreme hydrostatic or litho pressure. Barophiles or piezophiles are microorganisms that can survive and thrive under these high-pressure conditions. They possess adaptations to maintain cellular integrity and prevent damage caused by pressure changes.
- Radiation Environments: Microorganisms exposed to high levels of radiation, such as deserts or mountain peaks, have developed mechanisms to withstand and repair radiation-induced damage. Some extremophiles are capable of withstanding ultraviolet or gamma radiation by producing protective pigments or DNA repair enzymes.
- Xeric Environments: Xeric or arid environments with limited water availability are home to xerophiles, which are adapted to survive in conditions of low water activity. Microorganisms in cold and hot deserts have evolved strategies to conserve water, tolerate desiccation, and utilize scarce resources.
Studying the microbiology of extreme environments not only expands our understanding of the diversity of life on Earth but also provides insights into the potential for microbial life in extraterrestrial environments. The adaptations and survival strategies of extremophiles have implications for various fields, including astrobiology, biotechnology, and environmental science.
Extreme environments definition
What are extremophiles?
Extremophiles are unique organisms that possess the remarkable ability to thrive and survive in extreme environments that are typically considered hostile to life. Here are some key points about extremophiles:
- Adaptations to Extreme Environments: Extremophiles have evolved various physiological, biochemical, and molecular adaptations that allow them to withstand and even utilize the extreme conditions of their habitats. These adaptations enable them to maintain cellular function, metabolic processes, and reproduction in environments that would be lethal to most other organisms.
- Diverse Environmental Niches: Extremophiles can be found in a wide range of extreme environments, including but not limited to extreme temperatures, high pressure, acidic or alkaline pH levels, high salt concentrations, toxic waste sites, and organic solvents. They have colonized habitats that were once thought to be inhospitable for life.
- Classification of Extremophiles: Extremophiles can be categorized into two main groups based on their relationship with extreme conditions. Extremophilic organisms require one or more extreme conditions to survive and thrive, while extremotolerant organisms can tolerate extreme conditions but may grow optimally under more moderate conditions.
- Prokaryotes and Eukaryotes: While most extremophiles are prokaryotes, belonging to the domains Bacteria and Archaea, there are also eukaryotic extremophiles. Eukaryotic extremophiles can include protists such as algae, fungi, and protozoa, as well as multicellular organisms.
- Types of Extremophiles: Extremophiles are classified based on the specific extreme conditions in which they thrive. Some examples include thermophiles and hyperthermophiles (organisms that thrive at high or very high temperatures), psychrophiles (organisms that grow in cold temperatures), acidophiles and alkaliphiles (organisms adapted to acidic or alkaline pH levels), barophiles (organisms that prefer high-pressure environments), and halophiles (organisms that flourish in high-salt environments).
- Importance and Applications: The study of extremophiles has provided valuable insights into the limits of life on Earth and the potential for life in extreme environments elsewhere in the universe. Extremophiles have also found applications in biotechnology, including the production of enzymes with industrial uses, bioremediation of contaminated sites, and as models for studying the origin and evolution of life.
Definition of extremophiles
Extremophiles are organisms that thrive and survive in extreme environments, such as high temperatures, acidic or alkaline pH levels, high pressure, or high salt concentrations, which are typically considered hostile to most other forms of life.
Characteristics of extremophiles
Extremophiles possess several unique characteristics that allow them to survive and thrive in extreme environments:
- Structural Adaptations: Extremophiles have developed specific structural features that enable them to withstand extreme conditions. These adaptations can include specialized cell membranes, protective coatings, and heat shock proteins that stabilize their cellular structures.
- Metabolic Adaptations: Extremophiles exhibit a wide range of metabolic diversity. They have evolved unique enzymatic systems that function optimally under extreme conditions. For example, thermophilic extremophiles have enzymes that are stable and active at high temperatures.
- DNA Repair Mechanisms: Extremophiles possess efficient DNA repair mechanisms to counteract the damaging effects of extreme environments, such as high levels of radiation or temperature fluctuations. These mechanisms ensure the integrity and stability of their genetic material.
- Osmoregulation: Extremophiles living in high-salt environments, known as halophiles, have developed osmoregulatory mechanisms to maintain the balance of water and ions within their cells. They accumulate compatible solutes to counteract the osmotic stress caused by high salt concentrations.
- pH Regulation: Acidophilic and alkaliphilic extremophiles have mechanisms to maintain intracellular pH within a narrow range, despite the acidic or alkaline conditions of their habitats. They utilize specific ion transporters and enzymes to maintain cellular homeostasis.
- Energy Sources: Extremophiles have adapted to use unconventional energy sources for their metabolism. Some extremophiles can derive energy from inorganic compounds, such as sulfur or iron, while others can perform chemosynthesis or survive through photosynthesis in extreme light conditions.
- Evolutionary Adaptations: Extremophiles have undergone extensive evolutionary processes to develop their unique characteristics. They have acquired genetic adaptations over long periods of time, allowing them to colonize and thrive in their extreme habitats.
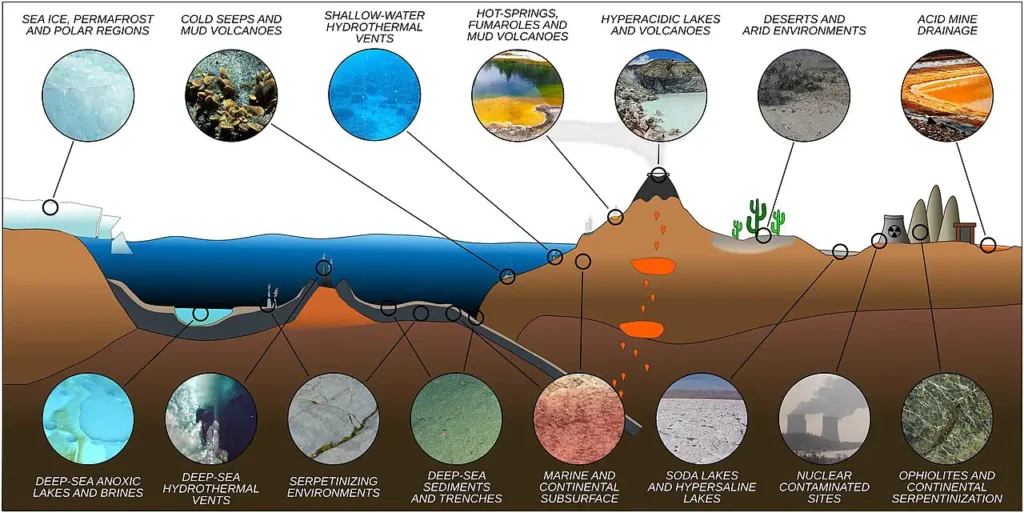
Types of extremophiles
Extremophiles are organisms that thrive and survive in extreme environments. They are classified into different types based on the specific extreme condition in which they can live and grow. Here are some types of extremophiles:
- Thermophiles: Thermophiles are organisms that thrive in high-temperature environments. They can grow optimally at temperatures above 45°C and can be found in environments such as hot springs, geothermal areas, and volcanic regions.
- Hyperthermophiles: Hyperthermophiles are a subset of thermophiles that can survive and grow at even higher temperatures, typically above 80°C. They are found in extreme environments like deep-sea hydrothermal vents and hot sulfur springs.
- Psychrophiles: Psychrophiles are organisms adapted to cold temperatures. They can thrive in environments with temperatures below 15°C, such as polar regions, glaciers, and cold deep-sea habitats.
- Acidophiles: Acidophiles are extremophiles that thrive in highly acidic environments. They can withstand low pH conditions (pH values below 3) and are found in acid mine drainage, volcanic lakes, and acidic soils.
- Alkaliphiles: Alkaliphiles are extremophiles that inhabit highly alkaline environments. They can tolerate high pH conditions (pH values above 9) and are found in alkaline lakes, soda lakes, and alkaline soils.
- Halophiles: Halophiles are extremophiles adapted to high-salt environments. They can survive in environments with high salt concentrations, such as salt flats, saline lakes, and hypersaline environments.
- Barophiles: Barophiles, also known as piezophiles or pressure-loving organisms, can thrive under high-pressure conditions, such as deep-sea trenches and hydrothermal vents.
- Xerophiles: Xerophiles are extremophiles adapted to extremely dry and arid conditions. They can survive in deserts, salt flats, and other water-deprived environments.
- Radiotolerant organisms: Radiotolerant organisms can withstand high levels of radiation, such as ultraviolet (UV) radiation or ionizing radiation. They are found in radioactive environments, deep subsurface habitats, and nuclear waste sites.
- Oligotrophs: Oligotrophs are extremophiles that can survive and thrive in nutrient-poor environments. They can adapt to low-nutrient conditions and are found in environments with limited resources, such as deep-sea sediments and caves.
These different types of extremophiles have developed specialized adaptations that allow them to survive and thrive in their extreme habitats. They provide valuable insights into the diversity of life on Earth and offer potential applications in various fields, including biotechnology, astrobiology, and environmental remediation.
Extremophiles examples
Here are some examples of extremophiles:
- Thermophiles:
- Thermus aquaticus: A thermophilic bacterium found in hot springs and known for its heat-resistant enzymes, including Taq polymerase used in PCR.
- Pyrococcus furiosus: A hyperthermophilic archaeon that thrives in deep-sea hydrothermal vents and has enzymes with high-temperature stability.
- Hyperthermophiles:
- Pyrolobus fumarii: A hyperthermophilic archaeon found in hydrothermal vents and capable of growing at temperatures up to 113°C.
- Thermococcus litoralis: A hyperthermophilic archaeon that can grow at temperatures exceeding 90°C and is found in deep-sea hydrothermal environments.
- Psychrophiles:
- Psychrobacter arcticus: A psychrophilic bacterium found in polar regions and cold environments, capable of growth at temperatures close to 0°C.
- Chlamydomonas nivalis: A psychrophilic algae species that thrives in snowfields and is responsible for the pink or red coloration known as “watermelon snow.”
- Acidophiles:
- Acidithiobacillus ferrooxidans: An acidophilic bacterium found in acid mine drainage and plays a role in bioleaching of minerals.
- Sulfolobus acidocaldarius: An acidophilic archaeon that thrives in acidic hot springs, with an optimum growth pH of around 2-3.
- Alkaliphiles:
- Natronobacterium gregoryi: An alkaliphilic bacterium found in soda lakes with a high pH, often containing high concentrations of sodium carbonate.
- Natronomonas pharaonis: An alkaliphilic archaeon found in soda lakes, capable of withstanding high pH and high salt concentrations.
- Halophiles:
- Haloquadratum walsbyi: A halophilic archaeon found in salt flats and hypersaline environments, with a square-shaped cell morphology.
- Halobacterium salinarum: A halophilic archaeon commonly found in salt ponds and produces red pigments known as bacteriorhodopsins.
- Barophiles:
- Methanocaldococcus jannaschii: A barophilic archaeon that thrives in deep-sea hydrothermal vents and can withstand high pressures of the ocean depths.
- Moritella marina: A barophilic bacterium found in the deep-sea environment, including hydrothermal vents and deep-sea sediments.
These are just a few examples of extremophiles across different categories. Extremophiles have been discovered in diverse habitats worldwide, showcasing the remarkable adaptability of life to extreme conditions.
Extreme environment examples
Extreme environments refer to habitats that exhibit harsh or extreme conditions beyond what is considered optimal for most organisms to survive and thrive. Here are some examples of extreme environments:
- Polar Regions: The Arctic and Antarctic regions are characterized by extremely low temperatures, icy conditions, and long periods of darkness. These environments pose significant challenges for life due to the extreme cold, limited resources, and harsh weather conditions.
- Deserts: Deserts are characterized by arid and dry conditions, with very low precipitation and high temperatures. Organisms living in deserts must be adapted to extreme heat, intense sunlight, and limited water availability.
- Deep-sea hydrothermal vents: These deep-sea environments are characterized by high temperatures, extreme pressure, and the presence of toxic chemicals and minerals. Despite these challenging conditions, unique ecosystems thrive around hydrothermal vents, supported by chemosynthetic bacteria that convert chemicals into energy.
- Volcanic regions: Volcanic environments, such as volcanic craters and lava fields, have high temperatures, toxic gases, and rugged terrain. Some extremophiles can tolerate the extreme heat, acidity, and heavy metal-rich soils found in these regions.
- High-altitude mountains: The high-altitude regions of mountains, such as the Himalayas or the Andes, present extreme cold temperatures, low oxygen levels, and harsh climatic conditions. Organisms in these environments must withstand low temperatures, strong winds, and limited resources.
- Deep-sea trenches: Deep-sea trenches, like the Mariana Trench, are the deepest parts of the oceans. These environments are characterized by extreme pressure, total darkness, and cold temperatures. Organisms found in these trenches have adapted to survive under immense pressure and scarce food resources.
- Acidic or alkaline environments: Certain lakes, ponds, and geothermal areas can have extreme pH levels, either highly acidic or strongly alkaline. These environments pose challenges for life due to the chemical imbalances and extreme conditions that organisms must tolerate.
- Space: Outer space represents the ultimate extreme environment, characterized by vacuum, extreme temperatures, radiation, and lack of atmospheric protection. Astrobiology explores the potential for life to exist or survive in space or on other celestial bodies.
These extreme environments provide unique challenges and opportunities for studying extremophiles and understanding the limits of life on Earth and beyond. They offer insights into the diversity and adaptability of organisms and have implications for astrobiology, biotechnology, and our understanding of the fundamental principles of life.
A. Microorganisms in extreme temperature
Microorganisms that can survive in extreme temperatures are known as thermophiles (organisms that thrive in high temperatures) and psychrophiles (organisms that thrive in low temperatures).
Examples of thermophilic microorganisms include:
- Thermus aquaticus: a bacterium that can grow at temperatures up to 80°C and is commonly used in DNA amplification (PCR) in molecular biology.
- Pyrococcus furiosus: an archaeon that can grow at temperatures up to 100°C and is commonly used in biotechnological applications.
- Geobacillus stearothermophilus: a bacterium that can grow at temperatures up to 75°C and is commonly used in industrial processes such as the production of detergents and enzymes.
Examples of psychrophilic microorganisms include:
- Psychrobacter arcticus: a bacterium that can grow at temperatures as low as -10°C and is commonly found in Arctic and Antarctic regions.
- Colwellia psychrerythraea: a bacterium that can grow at temperatures as low as -1.5°C and is commonly found in cold marine environments.
- Chlamydomonas nivalis: a green alga that can grow at temperatures as low as -20°C and is commonly found in snow and ice.
These microorganisms have evolved a range of adaptations that allow them to survive and thrive in extreme temperature conditions, including the production of specialized enzymes and membrane proteins that are able to function at high or low temperatures. Some thermophilic and psychrophilic microorganisms have been harnessed for biotechnological applications, such as the production of enzymes and other biomolecules for industrial and medical purposes.
1. Psychrophile
- Psychrophiles, also known as cold-loving organisms, are adapted to thrive and grow at low temperatures. They have an optimum growth temperature greater than 15°C and a maximum growth temperature greater than 20°C. These microorganisms have successfully colonized various permanently cold environments, ranging from the deep sea to mountain and polar regions.
- The prevalence of permanently cold environments, such as ocean waters, permafrost, glaciers, Antarctic rocks, snowfields, and polar ice caps, provides widespread suitable habitats for psychrophiles. Some of these organisms are also referred to as psychrotolerant or psychrotrophs, depending on their optimal growth temperature.
- Psychrophiles have overcome two primary challenges associated with growth at low temperatures. Firstly, the biochemical reactions in their cells are significantly affected by the decrease in temperature. Secondly, the viscosity of aqueous environments poses challenges for cellular processes. Nevertheless, psychrophiles have developed adaptations to thrive under these conditions.
- Prokaryotic psychrophiles exhibit varying requirements and tolerances for oxygen, including strictly aerobic, strictly anaerobic, and facultative species. Apart from extreme cold, many psychrophiles can tolerate or even require other extreme environmental conditions for their growth and survival. For example, deep-sea psychrophiles may rely on high pressures, leading to their classification as barophilic psychrophiles.
- Psychrophiles represent an intriguing group of organisms that have successfully adapted to the challenges posed by low-temperature environments. Their ability to thrive in these extreme conditions expands our understanding of the diversity of life on Earth and the incredible adaptability of microorganisms.
Psychrophile Adaptations
Psychrophiles, organisms that thrive in cold environments, have evolved specific modes of adaptation to survive and function optimally in these extreme conditions. Some key aspects of their adaptation include:
a. Proteins:
- Psychrophiles produce proteins and enzymes that are specifically adapted to function at low temperatures.
- Cold-active enzymes in psychrophiles often have a higher proportion of α-helix structures and a lower proportion of β-sheet structures, which provides greater flexibility and stability in cold temperatures.
- They also produce antifreeze proteins that bind to ice crystals, lowering the freezing point and preventing ice formation within the organism.
b. Membrane:
- The cytoplasmic membranes of psychrophiles contain a higher amount of unsaturated fatty acids. These fatty acids help maintain a semi-fluid state of the membrane at low temperatures.
- Psychrophiles may also have larger lipid head groups, increased protein content, and non-polar carotenoid pigments in their membranes to enhance fluidity and maintain functionality in cold conditions.
- Some psychrophiles have lipids with polyunsaturated fatty acids and long-chain hydrocarbons, which further contribute to membrane fluidity.
c. Genome structure:
- Psychrophiles have distinctive genomic characteristics, including high G+C genomic regions that code for informational proteins like tRNAs, elongation factors, and RNA polymerases.
- These organisms often possess redundant genes, with multiple copies of tRNA species for amino acid biosynthesis and an increased variety and number of chaperones to assist in protein folding and stability.
Overall, the adaptation of psychrophiles involves modifications in protein structure, membrane composition, and genome organization to ensure their survival and functionality in cold environments. These adaptations enable them to maintain crucial cellular processes, such as protein function, membrane integrity, and genetic information processing, at low temperatures.
Psychrophile Examples
There are many examples of psychrophiles, which are microorganisms that thrive in extremely cold environments. Here are a few examples:
- Psychrobacter: This genus of bacteria is commonly found in cold environments, such as glaciers, sea ice, and Arctic soils. Some species of Psychrobacter are known to cause food spoilage in refrigerated food products.
- Colwellia: These bacteria are commonly found in deep sea sediments and are able to survive at temperatures as low as -1.5°C. They have been found to produce a variety of enzymes that are useful in biotechnology, including cold-active lipases and proteases.
- Chlamydomonas nivalis: This single-celled algae is commonly found in snow and ice in polar and alpine environments. It is able to survive and carry out photosynthesis in temperatures as low as -20°C.
- Polaromonas vacuolata: This bacterium is commonly found in Arctic and Antarctic soils and is able to survive at temperatures as low as -5°C. It produces a variety of enzymes, including cold-active proteases and cellulases.
- Methanogenium frigidum: This methanogenic archaeon is found in permafrost soils in the Arctic and is able to survive and produce methane at temperatures as low as -16°C.
These are just a few examples of the many different types of psychrophiles that have been identified. Their ability to survive and thrive in extremely cold environments is fascinating and has important implications for biotechnology, medicine, and our understanding of the limits of life on Earth.
2. Thermophile
- Thermophiles, which literally means heat lovers, are organisms that thrive and grow at temperatures above those that sustain most life forms (typically above 25–40°C). These organisms exhibit maximum growth rates at temperatures above 45°C.
- Thermophiles can be found in various environments with extreme temperatures, including terrestrial and submarine habitats. They can exist in diverse locations ranging from surface soil to compost piles. However, they are particularly prevalent in areas such as thermal vents, hot springs, and boiling steam vents.
- Thermophiles can be further classified into different groups based on their temperature preferences. Facultative thermophiles are capable of thriving at both high and moderate temperatures, while obligate thermophiles specifically require high temperatures for growth.
- Similar to psychrophiles, thermophiles possess physiological and molecular adaptations that enable them to survive in environments with elevated temperatures. These adaptations allow them to withstand conditions that would normally denature proteins, cell membranes, and genetic material.
- The study of thermophiles provides valuable insights into the mechanisms of heat resistance and the potential applications of extremophiles in various industries. Understanding their unique adaptations may contribute to advancements in fields such as biotechnology and enzyme engineering, as thermophilic enzymes have desirable properties for industrial processes.
Thermophile Adaptations
Thermophiles, organisms that thrive at high temperatures, have specific modes of adaptation that allow them to survive in these extreme conditions. These adaptations are observed in their proteins, cell membranes, and nucleic acids.
- Proteins: Thermophilic enzymes and proteins are much more stable to heat than those found in mesophiles (organisms that grow at moderate temperatures). This heat stability is achieved through critical amino acid substitutions in the enzymes, which enable them to fold in a way that maintains their structural integrity at high temperatures. Additionally, thermophiles produce certain solutes, such as diinositol phosphate and monosylglycerate, which help stabilize proteins against thermal degradation.
- Cell membrane: The stability of the cell membrane is crucial for thermophiles. These organisms typically have cytoplasmic membranes that are rich in saturated fatty acids. The presence of saturated fatty acids allows the membrane to remain stable and functional at high temperatures. Compared to unsaturated fatty acids, saturated fatty acids provide a stronger hydrophobic environment, which contributes to membrane stability.
- Nucleic acid: Thermophiles have specific adaptations related to their nucleic acids, particularly DNA. They possess unique DNA binding proteins that arrange the DNA into globular particles, making it more resistant to melting under high temperatures. Additionally, thermophiles have a specialized enzyme called DNA gyrase, which introduces positive supercoils in DNA. This supercoiling provides additional stability to the DNA molecule and enhances heat resistance.
These adaptations in proteins, cell membranes, and nucleic acids enable thermophiles to thrive in high-temperature environments. By understanding these mode of adaptations, scientists can gain insights into the mechanisms of heat stability and potentially apply this knowledge in various fields, including biotechnology and industrial processes.
Thermophile Examples
There are many examples of thermophiles, which are microorganisms that thrive in high-temperature environments. Here are a few examples:
- Thermus aquaticus: This bacterium was first discovered in the hot springs of Yellowstone National Park, and is commonly used in molecular biology for its ability to withstand high temperatures. It produces an enzyme called Taq polymerase, which is used in the polymerase chain reaction (PCR) technique to amplify DNA.
- Pyrococcus furiosus: This hyperthermophilic archaeon is found in deep-sea hydrothermal vents and can grow at temperatures up to 100°C. It produces a variety of enzymes that are useful in biotechnology, including thermostable DNA polymerases and cellulases.
- Sulfolobus solfataricus: This thermophilic archaeon is commonly found in acidic hot springs and can grow at temperatures up to 80°C. It produces a variety of enzymes, including sulfur-reducing enzymes and DNA repair enzymes.
- Thermus thermophilus: This bacterium is commonly found in hot springs and geysers and can grow at temperatures up to 80°C. It produces a variety of enzymes, including thermostable DNA polymerases and proteases.
- Aquifex aeolicus: This bacterium is commonly found in deep-sea hydrothermal vents and can grow at temperatures up to 95°C. It is unique in that it is a chemolithotroph, meaning it can use inorganic compounds as its energy source.
These are just a few examples of the many different types of thermophiles that have been identified. Their ability to survive and thrive in extremely hot environments is fascinating and has important implications for biotechnology, medicine, and our understanding of the limits of life on Earth.
3. Hyperthermophile
- Hyperthermophiles are a group of organisms that thrive in extremely high temperatures, exceeding 80°C. These organisms, also known as extreme thermophiles, have adapted to survive and grow in environments where other thermophiles cannot.
- Hyperthermophiles have an optimum temperature of growth around 80°C, but they can endure even higher temperatures, exceeding 100°C. They are capable of withstanding extreme conditions such as high pH levels and high pressure, which are commonly found in their natural habitats. Most hyperthermophilic organisms belong to the archaea domain, with only a few species being bacterial.
- These organisms are predominantly found in environments such as hot springs and boiling steam vents, where temperatures reach extreme levels. These habitats provide the necessary conditions for hyperthermophiles to thrive, as they can withstand the intense heat and other harsh environmental factors that would be inhospitable to most other life forms.
- Hyperthermophiles have unique adaptations that allow them to survive in these extreme conditions. Their proteins and enzymes are highly stable at high temperatures, often containing specific amino acid substitutions that confer thermal stability. Their cell membranes are composed of lipids rich in saturated fatty acids, providing stability and protection against heat-induced damage. Additionally, their nucleic acids possess specialized proteins and enzymes that contribute to their heat resistance.
- Studying hyperthermophiles and understanding their adaptations to extreme heat has significant scientific and practical implications. These organisms offer insights into the fundamental principles of life and the limits of biological processes. Additionally, their unique enzymes and proteins have potential applications in biotechnology, such as the development of heat-stable enzymes for industrial processes.
- Overall, hyperthermophiles are remarkable organisms that have evolved to thrive in some of the most extreme environments on Earth. Their ability to survive and function at such high temperatures showcases the diversity and resilience of life in challenging conditions.
Hyperthermophile Adaptations
Hyperthermophiles have evolved a number of adaptations that enable them to survive in extremely hot environments. Some of the key modes of adaptation include:
- Thermophilic enzymes: Hyperthermophiles produce enzymes that are stable at high temperatures, allowing them to carry out essential metabolic processes even in extreme heat. These enzymes have evolved to function optimally at high temperatures, with modifications to their structure and amino acid composition that allow them to maintain stability and activity.
- Heat-shock proteins: Hyperthermophiles also produce heat-shock proteins, which protect other cellular components from damage caused by high temperatures. These proteins help to prevent denaturation of proteins and maintain the structural integrity of cellular membranes.
- Membrane modifications: Hyperthermophiles have evolved modifications to their cellular membranes that make them more stable at high temperatures. These modifications may include changes to the lipid composition of the membrane, as well as the presence of specialized lipids or proteins that stabilize the membrane.
- DNA stabilization: Hyperthermophiles have also evolved mechanisms to protect their DNA from damage caused by high temperatures. These mechanisms may include specialized DNA-binding proteins that help to stabilize the double helix, as well as DNA repair enzymes that can fix any damage that does occur.
- Unique metabolic pathways: Some hyperthermophiles have developed unique metabolic pathways that allow them to thrive in high-temperature environments. For example, some bacteria use chemolithotrophy, a metabolic process that involves the oxidation of inorganic compounds such as hydrogen, sulfur, and iron for energy.
Hyperthermophile Examples
Hyperthermophiles are a diverse group of microorganisms that are found in a range of high-temperature environments, including hydrothermal vents, geothermal pools, and deep-sea thermal vents. Some examples of hyperthermophiles include:
- Pyrococcus furiosus: This archaeon is found in hydrothermal vents and has an optimal growth temperature of 100°C. It is known for its ability to produce a range of heat-stable enzymes, including DNA polymerase, which is used in molecular biology research.
- Thermus aquaticus: This bacterium is found in geothermal pools and has an optimal growth temperature of around 70°C. It is notable for its production of Taq polymerase, a heat-stable enzyme used in the polymerase chain reaction (PCR) technique.
- Sulfolobus acidocaldarius: This archaeon is found in acidic geothermal pools and has an optimal growth temperature of around 80°C. It has a unique metabolism that involves the oxidation of sulfur compounds for energy.
- Aquifex aeolicus: This bacterium is found in deep-sea thermal vents and has an optimal growth temperature of around 85°C. It is notable for its simple genome and ability to use hydrogen gas as a source of energy.
- Methanocaldococcus jannaschii: This archaeon is found in deep-sea thermal vents and has an optimal growth temperature of around 85°C. It is known for its ability to produce methane and for its unusual nucleic acid composition, which includes modified bases that help to stabilize DNA and RNA at high temperatures.
Differences Between Psychrophile, Thermophile, and Hyperthermophile
Characteristic | Psychrophile | Thermophile | Hyperthermophile |
---|---|---|---|
Temperature Range | -15°C to 20°C | 45°C to 80°C | 80°C to 115°C |
Optimal Temperature | 15°C to 20°C | 60°C to 65°C | 85°C to 113°C |
Membrane Lipids | High proportion of unsaturated fatty acids | High proportion of saturated fatty acids | High proportion of saturated fatty acids with branched chains |
Enzyme Structure | Flexible and more active at lower temperatures | Rigid and more active at higher temperatures | Rigid and stable at high temperatures |
Metabolic Rate | Slow and reduced at low temperatures | High and fast at high temperatures | Very high and very fast at very high temperatures |
Habitat | Cold environments such as glaciers, Arctic and Antarctic regions | Hot springs, geothermal vents, compost heaps | Hydrothermal vents, deep-sea hydrothermal vents, volcanic vents |
Psychrophiles are microorganisms that can thrive in very low temperatures, between -15°C to 20°C, with an optimal temperature range of 15°C to 20°C. They have a high proportion of unsaturated fatty acids in their membrane lipids, which help maintain membrane fluidity in cold temperatures. Their enzymes are flexible and more active at lower temperatures, and their metabolic rate is slow and reduced at low temperatures. They are commonly found in cold environments such as glaciers, Arctic and Antarctic regions.
Thermophiles are microorganisms that can thrive in high temperatures, between 45°C to 80°C, with an optimal temperature range of 60°C to 65°C. They have a high proportion of saturated fatty acids in their membrane lipids, which help maintain membrane stability at high temperatures. Their enzymes are rigid and more active at higher temperatures, and their metabolic rate is high and fast at high temperatures. They are commonly found in hot springs, geothermal vents, and compost heaps.
Hyperthermophiles are microorganisms that can thrive in very high temperatures, between 80°C to 115°C, with an optimal temperature range of 85°C to 113°C. They have a high proportion of saturated fatty acids with branched chains in their membrane lipids, which help maintain membrane stability and prevent oxidation at high temperatures. Their enzymes are rigid and stable at high temperatures, and their metabolic rate is very high and very fast at very high temperatures. They are commonly found in extreme environments such as hydrothermal vents, deep-sea hydrothermal vents, and volcanic vents.
B. Microorganisms in extreme pH
Microorganisms that can survive in extreme pH conditions are known as acidophiles and alkaliphiles. Acidophiles can grow and reproduce in environments with pH levels below 4, while alkaliphiles can grow in environments with pH levels above 9.
Examples of acidophilic microorganisms include:
- Acidithiobacillus ferrooxidans: a bacterium that can oxidize iron and sulfur compounds, and is commonly found in acidic mine drainage.
- Picrophilus oshimae: a thermophilic archaeon that can grow at pH levels as low as 0.7.
- Lactobacillus acidophilus: a bacterium commonly found in the human gastrointestinal tract that is able to survive and grow in acidic conditions.
Examples of alkaliphilic microorganisms include:
- Bacillus pseudofirmus: a bacterium that can grow in alkaline environments up to pH 11.5.
- Natronomonas pharaonis: an archaeon that can grow in environments with pH levels up to 11.
- Spirulina platensis: a cyanobacterium that is able to grow in alkaline conditions and is commonly found in soda lakes.
These microorganisms have evolved a range of adaptations that allow them to survive and thrive in extreme pH conditions, including the production of specialized enzymes and membrane proteins that are able to function at high or low pH levels. Some acidophilic and alkaliphilic microorganisms have been harnessed for biotechnological applications, such as the production of biofuels and the treatment of acidic or alkaline wastewater.
1. Acidophile
- Acidophiles are organisms that have the ability to survive and thrive in highly acidic conditions, typically at pH 2.0 or lower. They are found in a variety of natural and man-made environments characterized by extreme acidity, such as acidic lakes, hydrothermal systems, acid sulfate soils, sulfidic regoliths, and metal and coal mine-impacted areas.
- These environments are formed through the oxidation of metal and sulfidic minerals, leading to the formation of highly acidic conditions. Acidophiles have adapted to these extreme habitats and can tolerate the low pH levels.
- Among the acidophiles, the most extensively studied organisms are prokaryotes that oxidize reduced iron and sulfur. They play a crucial role in the oxidative dissolution of metal sulfide minerals, such as pyrite (FeS2), which leads to the severe acidification of the environment in which they thrive.
- Acidophilic bacteria, often in close association with chemolithotrophic primary producers, are commonly found in extremely acidic environments. They exhibit diverse physiological characteristics, including being aerobic, facultative anaerobic, chemolithotrophic, and heterotrophic. Additionally, acidophilic organisms can include photoautotrophic eukaryotes, predatory protozoa, and other types of microorganisms.
- Acidophiles are represented across all three domains of life: archaea, bacteria, and eukaryotes. However, archaea, specifically the group of acidophilic archaea, are the most predominant among acidophilic organisms.
- The study of acidophiles and their adaptations to extreme acidity provides insights into the limits of life on Earth and expands our understanding of microbial diversity and resilience.
Acidophile Mode of adaptation
Acidophiles have developed several strategies to adapt to the harsh acidic environments in which they live. Some of the key adaptations include:
- Maintaining pH homeostasis: Acidophiles have developed various mechanisms to maintain pH homeostasis within their cells. For example, they may use proton pumps or transporters to expel excess protons from their cytoplasm or use intracellular buffering systems to maintain a neutral pH.
- Modifying cellular membranes: Acidophiles may modify their cellular membranes to protect them from the corrosive effects of acidic conditions. They may produce specialized lipids or proteins that can resist acid hydrolysis or maintain membrane integrity in acidic environments.
- Producing acid-resistant enzymes: Acidophiles produce enzymes that are adapted to function in acidic environments. These enzymes often have a unique amino acid composition, which allows them to maintain their structure and function under acidic conditions.
- Neutralizing mechanisms: Some acidophiles produce molecules that help to neutralize acidic conditions. For example, they may produce ammonia, bicarbonate, or other basic compounds that can raise the pH of their environment.
- Slow growth rates: Acidophiles often have slow growth rates compared to other microorganisms. This may be because the harsh conditions they live in make it difficult for them to carry out essential metabolic processes at a fast pace.
Overall, acidophiles have developed a range of adaptations that allow them to survive and thrive in acidic environments. These adaptations have important implications for our understanding of the diversity of life on Earth and the potential for life in extreme environments.
Acidophile Examples
Acidophiles can be found in a wide range of environments, including soils, water bodies, and extreme habitats such as acid mine drainage systems. Some examples of acidophilic microorganisms include:
- Acidithiobacillus ferrooxidans: A. ferrooxidans is a Gram-negative bacterium that is commonly found in acidic environments such as mine drainage systems. It is able to oxidize iron and sulfur compounds, and its metabolism is highly dependent on low pH conditions.
- Picrophilus oshimae: P. oshimae is an extreme acidophile that has been isolated from acidic hot springs in Japan. It is a member of the archaea domain and is known for its ability to survive in environments with a pH as low as 0.7.
- Ferroplasma acidiphilum: F. acidiphilum is a highly acidophilic archaeon that is commonly found in acid mine drainage systems. It is able to oxidize iron and sulfur compounds and has been shown to play a key role in the formation of acid mine drainage.
- Thiobacillus thiooxidans: T. thiooxidans is a Gram-negative bacterium that is commonly found in acidic environments such as mine drainage systems. It is able to oxidize sulfur compounds and has been shown to play a key role in the biogeochemical cycling of sulfur.
- Acidobacterium capsulatum: A. capsulatum is a Gram-negative bacterium that is commonly found in acidic soils. It is able to metabolize a wide range of organic compounds and has been shown to play an important role in soil ecology.
These examples demonstrate the diversity of acidophilic microorganisms and their ability to thrive in highly acidic environments.
2. Alkaliphile
Alkaliphiles are microorganisms that are adapted to live and grow in alkaline environments, typically with a pH greater than 9.0. They are found in a variety of habitats, including soils, lakes, and soda lakes. Some of the key characteristics of alkaliphiles include:
- pH homeostasis: Like acidophiles, alkaliphiles have developed mechanisms to maintain pH homeostasis within their cells. They may use proton pumps or transporters to expel excess hydroxide ions from their cytoplasm or use intracellular buffering systems to maintain a neutral pH.
- Membrane modifications: Alkaliphiles may modify their cellular membranes to protect them from the effects of high pH. For example, they may produce specialized lipids or proteins that can resist alkali hydrolysis or maintain membrane integrity in alkaline environments.
- Alkaline-tolerant enzymes: Alkaliphiles produce enzymes that are adapted to function in alkaline conditions. These enzymes may have a unique amino acid composition, which allows them to maintain their structure and function under high pH conditions.
- Sodium metabolism: Many alkaliphiles use sodium ions as a key component of their metabolism, rather than relying on protons as is common in most other microorganisms. They may use specialized transporters to import sodium ions into their cells or sequester them in specialized compartments.
- Slow growth rates: Alkaliphiles often have slower growth rates than other microorganisms. This may be because the high pH conditions they live in make it difficult for them to carry out essential metabolic processes at a fast pace.
Overall, alkaliphiles have developed a range of adaptations that allow them to survive and thrive in alkaline environments. These adaptations have important implications for our understanding of the diversity of life on Earth and the potential for life in extreme environments.
Alkaliphile Mode of adaptation
Alkaliphiles have evolved a number of strategies to adapt to life in high-pH environments:
- pH homeostasis: One of the key adaptations of alkaliphiles is the ability to maintain a stable internal pH despite the high external pH. This is achieved through a variety of mechanisms, such as the production of proton pumps or the use of intracellular buffering systems.
- Membrane modifications: Alkaliphiles often modify their cellular membranes to maintain their structural integrity in alkaline conditions. For example, they may produce specialized lipids or proteins that are resistant to alkali hydrolysis.
- Enzyme adaptation: Alkaliphiles produce enzymes that are adapted to function at high pH values. These enzymes often have a unique amino acid composition that enables them to maintain their structure and function under alkaline conditions.
- Sodium metabolism: Many alkaliphiles use sodium ions as a key component of their metabolism, rather than relying on protons as most other microorganisms do. They may use specialized transporters to import sodium ions into their cells or sequester them in specialized compartments.
- Slow growth rates: Alkaliphiles often have slower growth rates than other microorganisms. This may be because the high pH conditions they live in make it difficult for them to carry out essential metabolic processes at a fast pace.
Overall, alkaliphiles have developed a range of strategies to cope with the challenges of life in high-pH environments. These adaptations allow them to thrive in habitats that would be inhospitable to most other microorganisms.
Alkaliphile Examples
There are many examples of alkaliphilic microorganisms, including:
- Natronomonas: This is a genus of bacteria that is found in highly alkaline soda lakes. They are able to grow in environments with a pH as high as 12.
- Bacillus pseudofirmus: This is a Gram-positive, spore-forming bacterium that is found in soda lakes and other alkaline environments. It is able to grow at pH values up to 11.5.
- Natronobacterium: This is a genus of halophilic bacteria that are able to grow in highly alkaline environments, such as soda lakes. They are able to grow at pH values up to 10.5.
- Halomonas: This is a genus of halophilic bacteria that are found in a variety of environments, including soda lakes and hypersaline soils. Some species are able to grow at pH values up to 10.5.
- Spirulina: This is a genus of cyanobacteria that is found in many alkaline lakes and ponds around the world. They are able to grow at pH values up to 11.
These are just a few examples of the many alkaliphilic microorganisms that have been discovered. Their ability to thrive in extreme environments has important implications for our understanding of the diversity of life on Earth and the potential for life in other extreme environments, such as those found on other planets.
Differences Between Acidophile, and Alkaliphile
Characteristic | Acidophile | Alkaliphile |
---|---|---|
pH Range | Below 5.5 | Above 8.5 |
Optimal pH | 2.0 to 5.5 | 9.0 to 11.5 |
Membrane Lipids | High proportion of branched chain fatty acids | High proportion of straight chain fatty acids |
Enzyme Structure | Acid-stable enzymes | Alkaline-stable enzymes |
Metabolic Rate | Slow growth rate due to proton influx | Fast growth rate due to high pH |
Habitat | Acidic environments such as acid mines, acid soils, and acidic hot springs | Alkaline environments such as soda lakes, high carbonate soils, and alkaline soils |
Acidophiles are microorganisms that can thrive in acidic environments with a pH below 5.5, and have an optimal pH range of 2.0 to 5.5. They have a high proportion of branched chain fatty acids in their membrane lipids, which help maintain membrane stability in acidic conditions. Their enzymes are acid-stable, allowing them to function in acidic environments. Their metabolic rate is slow due to the influx of protons in acidic environments. They are commonly found in acid mines, acid soils, and acidic hot springs.
Alkaliphiles are microorganisms that can thrive in alkaline environments with a pH above 8.5, and have an optimal pH range of 9.0 to 11.5. They have a high proportion of straight chain fatty acids in their membrane lipids, which help maintain membrane stability in alkaline conditions. Their enzymes are alkaline-stable, allowing them to function in alkaline environments. Their metabolic rate is fast due to the high pH, which promotes enzyme activity. They are commonly found in soda lakes, high carbonate soils, and alkaline soils.
C. Microorganisms in extreme low humidity/water activity
Microorganisms that can survive in environments with extreme low humidity or water activity are known as xerophiles. There are several types of xerophilic microorganisms, including:
- Bacteria: Many types of bacteria can survive in low-moisture environments, including spore-forming bacteria such as Bacillus and Clostridium species, as well as non-spore-forming bacteria such as Staphylococcus and Pseudomonas species.
- Fungi: Some fungi, such as Aspergillus and Penicillium species, are able to grow and reproduce in very low-moisture environments, such as dried fruits, spices, and grains.
- Yeasts: Certain yeasts, such as Saccharomyces and Candida species, can survive in environments with low water activity, such as in sugar-rich foods like honey and molasses.
- Algae: Certain types of algae, such as Chlorella and Scenedesmus species, are able to survive in extreme low-moisture environments, such as desert soils.
- Archaea: Some archaea, such as Haloquadratum and Halobacterium species, can thrive in hypersaline environments with very low water activity, such as salt flats and salt lakes.
These microorganisms have evolved a range of adaptations that allow them to survive and thrive in environments with very low water activity, including spore formation, osmotic regulation, and membrane modifications. They play important roles in nutrient cycling and ecosystem functioning in arid habitats, and some have also been harnessed for industrial and biotechnological applications.
1. Xerophile Definition and Characteristics
Xerophiles are microorganisms that are adapted to living in environments with very low water activity, typically below 0.85. These environments include deserts, salt flats, and other arid habitats.
Xerophiles have a number of characteristic adaptations that allow them to survive and thrive in these harsh environments. These adaptations include:
- Spore formation: Many xerophilic microorganisms are able to form spores, which are highly resistant to desiccation and can survive for extended periods of time in dry environments.
- Osmotic regulation: Xerophiles often produce solutes such as trehalose and glycine betaine, which help to maintain their cellular hydration levels and prevent damage to their cellular components.
- Membrane modifications: Xerophiles may produce specialized lipids or proteins that are able to maintain the integrity of their cellular membranes in dry environments.
- Metabolic adaptation: Xerophiles often have slower metabolic rates than other microorganisms, which allows them to conserve energy and resources in low-water environments.
Overall, xerophiles have evolved a number of strategies to cope with the challenges of life in dry environments. These adaptations allow them to survive in habitats that would be inhospitable to most other microorganisms.
Xerophile Mode of adaptation
Xerophiles have evolved a number of adaptations to survive in environments with very low water activity. These adaptations include:
- Spore formation: Many xerophilic microorganisms are able to form highly resistant spores that can survive extended periods of desiccation.
- Osmotic regulation: Xerophiles often produce solutes such as trehalose and glycine betaine, which help to maintain cellular hydration levels and prevent damage to cellular components.
- Membrane modifications: Xerophiles may produce specialized lipids or proteins that can maintain the integrity of cellular membranes in dry environments.
- Metabolic adaptation: Xerophiles often have slower metabolic rates than other microorganisms, which allows them to conserve energy and resources in low-water environments.
- DNA repair mechanisms: Xerophiles may have specialized DNA repair mechanisms that can prevent DNA damage caused by desiccation.
- Protective coatings: Some xerophilic microorganisms produce protective coatings or biofilms that can help to prevent desiccation and maintain hydration levels.
These adaptations allow xerophiles to survive and thrive in environments that would be inhospitable to most other microorganisms. However, xerophiles are also vulnerable to other stresses, such as high temperatures, radiation, and nutrient limitation, and may have additional adaptations to cope with these stressors as well.
Xerophiles examples
Examples of xerophilic microorganisms include:
- Aspergillus penicillioides: a fungus that can grow on dried fruits, spices, and other low-moisture foods.
- Xeromyces bisporus: a yeast that can grow in sugar-rich, low-moisture environments, such as honey.
- Salinibacter ruber: a bacterium that can live in extremely salty, low-moisture environments, such as salt flats.
- Haloquadratum walsbyi: a square-shaped archaeon that can survive in hypersaline environments, such as the Dead Sea.
- Chroococcidiopsis spp.: a group of cyanobacteria that can survive in hot, dry environments, such as desert soils and rocks.
- Staphylococcus xylosus: a bacterium that can survive in low-moisture environments, such as cured meats.
These microorganisms have evolved a range of adaptations that allow them to thrive in environments with very low water activity, including spore formation, osmotic regulation, and membrane modifications, among others. They play important roles in nutrient cycling and ecosystem functioning in arid habitats, and some have also been harnessed for industrial and biotechnological applications.
xerophiles bacteria examples
Xerophiles are organisms that are adapted to survive and thrive in extremely dry or desiccated environments. These environments can include arid regions, deserts, and places with very low water availability. While xerophiles can include various types of organisms, including plants and animals, I’ll provide some examples of xerophilic bacteria:
- Deinococcus radiodurans: This bacterium is known for its remarkable ability to withstand extreme conditions, including desiccation. It can survive extreme levels of radiation, dehydration, and oxidative stress. Deinococcus radiodurans is often used as a model organism for studying mechanisms of DNA repair and protection against desiccation.
- Chroococcidiopsis: This genus of cyanobacteria is capable of withstanding extreme desiccation and surviving in harsh environments, such as deserts and rocky regions. Chroococcidiopsis forms dormant, resistant cells called akinetes that protect the organism during dry periods and enable it to revive when favorable conditions return.
- Xeromyces bisporus: Although primarily known as a fungus involved in food production (commonly known as the button mushroom), Xeromyces bisporus has adaptations that allow it to thrive in dry environments. It can tolerate low water availability and has mechanisms to regulate water loss.
- Nostoc commune: This cyanobacterium is capable of surviving in diverse habitats, including arid regions. It forms colonies known as gelatinous masses or “star jelly” that can endure periods of desiccation and revive when conditions become favorable again.
- Haloarcula marismortui: This halophilic bacterium is adapted to saline environments with low water availability. It is often found in highly salty areas, such as salt flats or hypersaline lakes. Haloarcula marismortui has evolved mechanisms to survive in these conditions by maintaining high intracellular salt concentrations to counterbalance osmotic stress.
These examples highlight some of the bacteria that have adapted to thrive in xeric environments. Xerophiles possess unique physiological and biochemical characteristics that allow them to withstand extreme dryness and minimize water loss, enabling them to survive in environments where water availability is limited.
D. Microorganisms in extreme salinity
Microorganisms that can survive in extreme salinity are called halophilic microorganisms. They are typically found in environments with high salt concentrations, such as salt lakes, salt pans, and saline soils.
Examples of halophilic microorganisms include:
- Halobacterium salinarum: This bacterium is commonly found in salt lakes and other highly saline environments. It is able to survive in salt concentrations up to 25%.
- Haloquadratum walsbyi: This archaeon is commonly found in salt pans and other highly saline environments. It is able to survive in salt concentrations up to 35%.
- Dunaliella salina: This microalga is commonly found in salt ponds and other highly saline environments. It is able to survive in salt concentrations up to 35%.
- Salinibacter ruber: This bacterium is commonly found in salt pans and other highly saline environments. It is able to survive in salt concentrations up to 30%.
Halophilic microorganisms have developed several adaptations to survive in high salt concentrations. For example, they may produce compatible solutes such as betaine or trehalose to balance the concentration of solutes inside and outside the cell, or they may have specialized ion transport systems to regulate ion homeostasis.
1. Halophile
Halophiles are microorganisms that are adapted to live in environments with high salt concentrations, typically above 3% NaCl. They have evolved various strategies to cope with the osmotic stress caused by the high salt concentration.
Some common characteristics of halophiles include:
- Salt tolerance: Halophiles have adapted to survive and grow in environments with high salt concentrations by developing various mechanisms to cope with the osmotic stress. Some halophiles accumulate intracellular solutes such as potassium and glycine betaine to balance the osmotic pressure between their cytoplasm and the environment.
- Enzyme stability: The high salt concentrations in their environment can affect the stability and function of proteins and enzymes. Halophiles have developed enzymes that are stable in high salt concentrations, such as halophilic proteases and lipases.
- Pigmentation: Some halophiles produce pigments such as carotenoids and bacteriorhodopsin that protect their cells from UV radiation and oxidative stress.
- Habitat: Halophiles are commonly found in saline environments such as salt lakes, salt pans, and saline soils. Some halophiles are also found in marine environments such as salt marshes, estuaries, and the Dead Sea.
- Diversity: Halophiles include bacteria, archaea, and eukarya. Some well-known halophiles include Halobacterium salinarum, Halococcus morrhuae, and Salinibacter ruber.
Halophile Mode of adaptation
Halophiles have developed various strategies to adapt to high salt concentrations, which can cause osmotic stress and damage to their cells. Some of the modes of adaptation observed in halophiles include:
- Accumulation of compatible solutes: Halophiles can accumulate organic molecules such as amino acids, betaines, and sugars within their cells to balance the osmotic pressure between their cytoplasm and the environment. These compatible solutes help to protect the cells from dehydration and maintain the structural integrity of proteins and enzymes.
- Modification of membrane lipids: The high salt concentrations in the environment can disrupt the structure and function of cell membranes. Halophiles have adapted to this challenge by modifying their membrane lipids with a higher proportion of branched-chain fatty acids or by synthesizing novel lipids that are more stable in high salt concentrations.
- Production of salt-in proteins: Some halophiles produce specialized proteins known as salt-in proteins that can bind and sequester ions within their cells. These proteins help to maintain the osmotic balance and protect the cells from high salt concentrations.
- Reduction of water loss: Halophiles can reduce the amount of water loss from their cells by producing a protective exopolysaccharide matrix or by forming biofilms that trap water and nutrients within the community.
- Efficient transport systems: Halophiles have evolved efficient transport systems to take up and regulate the levels of essential nutrients such as nitrogen, phosphorus, and carbon in their saline environments.
Overall, halophiles have evolved multiple mechanisms to adapt and thrive in high salt environments, making them a unique group of microorganisms with potential applications in biotechnology and bioremediation.
Halophile Examples
Halophiles are found in a wide range of environments, including salt flats, salt pans, saline soils, and hypersaline lakes. Some examples of halophiles include:
- Haloarchaea: These are a group of halophilic archaea that are commonly found in hypersaline environments such as the Dead Sea, the Great Salt Lake, and salt pans. Haloarchaea are known for their ability to produce pigments such as bacteriorhodopsin and halorhodopsin that enable them to capture light energy and generate ATP.
- Halobacteria: These are another group of halophilic bacteria that are commonly found in salt lakes, salt pans, and saline soils. Halobacteria are known for their ability to produce bacterioruberin, a red pigment that protects them from oxidative stress.
- Dunaliella: These are unicellular green algae that are found in high-salt environments such as salt flats and brine pools. Dunaliella are known for their ability to produce large amounts of beta-carotene, a pigment that gives them a reddish-orange color and has applications in the food and cosmetic industries.
- Salinibacter: This is a halophilic bacterium that was discovered in a salt crystallizer pond in Spain. Salinibacter is notable for its ability to survive in extremely high salt concentrations (up to 30% NaCl) and for its unique genomic features, including a high proportion of genes involved in DNA repair.
These are just a few examples of the diverse range of halophiles that have adapted to life in high-salt environments.
E. Microorganisms in extreme sugar concentrations
Microorganisms that can survive in extreme sugar concentrations are called osmophilic microorganisms. They are typically found in environments with high concentrations of sugar, such as sugarcane juice, honey, and molasses.
Examples of osmophilic microorganisms include:
- Zygosaccharomyces rouxii: This yeast is commonly found in sugarcane juice and other high-sugar environments. It is able to survive in sugar concentrations up to 70%.
- Aspergillus niger: This fungus is commonly found in molasses and other sugar-rich environments. It is able to survive in sugar concentrations up to 70%.
- Saccharomyces cerevisiae: This yeast is commonly used in the production of bread, beer, and wine. It is able to survive in sugar concentrations up to 25%.
- Lactobacillus acidophilus: This bacteria is commonly found in honey and other high-sugar environments. It is able to survive in sugar concentrations up to 80%.
Osmophilic microorganisms have developed several adaptations to survive in high sugar concentrations. For example, they may produce osmolytes such as trehalose or glycerol to balance the concentration of solutes inside and outside the cell, or they may have thicker cell walls to prevent water loss.
1. Osmophile
Osmophiles definition: Osmophiles are microorganisms that are adapted to environments with high osmotic pressure, typically caused by high sugar or salt concentrations. These microorganisms are commonly found in environments such as honey, fruit juices, and molasses.
Characteristics of osmophiles include:
- Adaptation to high osmotic pressure: Osmophiles are able to survive and grow in environments with high osmotic pressure, which can cause dehydration and damage to other microorganisms.
- Production of compatible solutes: Osmophiles may produce compatible solutes such as glycerol or proline to balance the concentration of solutes inside and outside the cell.
- Tolerance to low water activity: Osmophiles are able to survive and grow in environments with low water activity, which can limit the availability of water for cellular processes.
- Resistance to antimicrobial agents: Osmophiles may have increased resistance to antimicrobial agents such as antibiotics, which can make them difficult to control in certain environments.
Osmophiles have developed several adaptations to survive in high-sugar or high-salt environments, including the production of compatible solutes and the regulation of ion homeostasis. These adaptations allow osmophiles to thrive in environments that would be inhospitable to other microorganisms.
Osmophile Mode of adaptation
Osmophiles have developed several adaptations to survive in environments with high osmotic pressure caused by high sugar or salt concentrations. Some of the ways in which osmophiles adapt to these conditions include:
- Production of compatible solutes: Osmophiles may produce compatible solutes such as glycerol, betaine, or proline to balance the concentration of solutes inside and outside the cell. These compatible solutes help to protect the cell from dehydration and maintain cellular function in high osmotic environments.
- Regulation of ion homeostasis: Osmophiles regulate the balance of ions inside and outside the cell to maintain osmotic equilibrium. They may have specialized transporters to take up or expel ions as needed to maintain proper ion concentrations.
- Structural adaptations: Osmophiles may have specialized cell wall or membrane structures to protect the cell from damage in high osmotic environments. For example, some bacteria may have a thicker cell wall or a more rigid outer membrane to prevent dehydration.
- Metabolic adaptations: Osmophiles may have specialized metabolic pathways to utilize the available nutrients in high-sugar or high-salt environments. For example, some yeasts may have adapted to utilize alternative carbon sources besides glucose, such as fructose or sucrose.
These adaptations allow osmophiles to survive and thrive in environments with high osmotic pressure, which can limit the availability of water and nutrients for cellular processes.
Osmophile Examples
- Zygosaccharomyces rouxii: This yeast is commonly found in high-sugar environments such as dried fruits and syrups.
- Saccharomyces cerevisiae: This yeast is commonly used in fermentation processes for beer and wine production, which involve high-sugar environments.
- Staphylococcus xylosus: This bacterium is commonly found in high-sugar environments such as honey and maple syrup.
F. Microorganisms in extreme pressure
Microorganisms that are adapted to survive in high-pressure environments are known as barophiles or piezophiles. These organisms have developed several adaptations to withstand the extreme pressures found in deep-sea environments and other high-pressure habitats. Some of the ways in which barophiles adapt to these conditions include:
- Membrane adaptations: Barophiles may have specialized membrane structures that are more rigid and less fluid to withstand the increased pressure. They may also produce more saturated fatty acids, which can help to stabilize the membrane.
- Enzyme adaptations: Barophiles may have enzymes that are more stable and functional under high pressure conditions. They may also have specialized enzymes that can function in the absence of oxygen, which is often limited in deep-sea environments.
- Structural adaptations: Barophiles may have specialized cell wall structures or other features that can withstand high pressure. For example, some deep-sea bacteria have developed pili or other appendages that help them attach to surfaces and resist the force of water currents.
- Osmotic adaptations: Some barophiles may have adapted to maintain a balance of osmotic pressure inside and outside the cell in high-pressure environments. This may involve producing compatible solutes or regulating ion concentrations.
These adaptations allow barophiles to thrive in environments with extreme pressure, such as deep-sea hydrothermal vents or sub-seafloor sediments. These environments are often characterized by limited resources, high salinity, and low temperature, and the ability of barophiles to survive under these conditions makes them important models for understanding the limits of life on Earth and the potential for life on other planets or moons.
1. Piezophile
Piezophile, also known as barophile or hyperpiezophile, is a type of extremophile that can thrive in environments with extremely high pressure, such as the deep ocean. These microorganisms have adapted to survive under immense pressure, which would be lethal to most other life forms.
Characteristics of piezophiles include:
- Pressure tolerance: Piezophiles are able to tolerate the extremely high pressure found in deep-sea environments, often up to 1,000 times greater than atmospheric pressure.
- Slow growth rate: Piezophiles often have a slow growth rate due to the energy required to maintain cellular structure under high pressure.
- Specialized membrane composition: The membranes of piezophiles are highly specialized to maintain structural integrity under high pressure.
- Enzymatic adaptations: Piezophiles have adapted their enzymes to function optimally under high pressure, often using more flexible protein structures.
- Limited genetic diversity: Piezophiles have limited genetic diversity due to the harsh conditions of their environment.
Piezophiles play an important role in the deep-sea ecosystem by breaking down organic matter and recycling nutrients. They are also of interest to biotechnology researchers due to their unique adaptations and potential applications in various industries.
Piezophile Mode of adaptation
Piezophiles, also known as barophiles or hyperpiezophiles, have adapted to survive in environments with extreme pressure, such as the deep ocean. Their mode of adaptation includes several strategies:
- Maintaining structural integrity: Piezophiles have adapted their cell membranes to maintain structural integrity under high pressure. This allows them to protect their internal structures and prevent damage.
- Adjusting enzyme function: Piezophiles have adapted their enzymes to function optimally under high pressure. These enzymes often have more flexible protein structures that can accommodate the pressure changes.
- Conserving energy: Piezophiles often have a slower growth rate due to the energy required to maintain cellular structure under high pressure. They conserve energy by limiting their metabolic activity.
- Reducing genetic diversity: Piezophiles have limited genetic diversity due to the harsh conditions of their environment. This allows them to maintain stable populations and adapt more efficiently to their surroundings.
Overall, piezophiles have developed a range of adaptations that allow them to survive and thrive in high-pressure environments. These adaptations help them maintain their cellular structure, conserve energy, and function optimally under extreme conditions.
Piezophile Examples
Piezophiles are found in a variety of deep-sea environments, including hydrothermal vents, seafloor sediments, and oceanic trenches. Some examples of piezophilic microorganisms include:
- Shewanella piezotolerans: A Gram-negative bacterium found in deep-sea sediments that can grow at pressures up to 50 MPa.
- Colwellia psychrerythraea: A Gram-negative bacterium found in deep-sea sediments and hydrothermal vents that can grow at pressures up to 70 MPa.
- Methanocaldococcus jannaschii: A thermophilic archaeon found near hydrothermal vents that can grow at pressures up to 120 MPa.
- Photobacterium profundum: A Gram-negative bacterium found in the deep ocean that can grow at pressures up to 70 MPa.
- Moritella sp.: A Gram-negative bacterium found in sediments in the Pacific Ocean that can grow at pressures up to 80 MPa.
These piezophilic microorganisms have adapted to the high-pressure conditions of their environment and are able to thrive in the deep-sea where other life forms cannot survive.
G. Microorganisms in rocks
Microorganisms are found in many different environments, including within rocks. These microorganisms can survive and thrive within the tiny spaces and crevices that exist within the rock matrix.
One type of microorganism commonly found within rocks is bacteria. Bacteria are able to adapt to extreme environments, including those with high temperatures, low oxygen levels, and acidic conditions. Some bacteria are also capable of breaking down minerals within the rock, releasing nutrients that other microorganisms can use for growth.
Another type of microorganism commonly found within rocks is fungi. Fungi are able to grow within the porous spaces within rocks, where they can break down organic matter and absorb nutrients. Some fungi are also capable of breaking down minerals within the rock, releasing nutrients that other microorganisms can use.
Microorganisms within rocks can play important roles in geologic processes, including the weathering and erosion of rocks. They can also impact the formation of minerals and contribute to the development of soil. Additionally, microorganisms within rocks may have important applications in bioremediation and the development of new technologies.
1. Endolith
- An endolith is a microorganism that lives inside rocks, deep in the Earth’s crust, or in other extreme environments where life is difficult to sustain. Endoliths are able to survive in these harsh conditions due to their ability to use various energy sources, such as minerals, sunlight, or organic matter.
- Endoliths can be found in a wide variety of environments, including deserts, glaciers, deep-sea sediments, and even within the walls of nuclear reactors. They are able to survive in these environments due to their ability to adapt to extreme conditions, such as high levels of radiation, extreme temperatures, and low nutrient availability.
- Endoliths are typically very small, ranging in size from a few micrometers to a few millimeters in diameter. They often live in colonies or communities, which can help protect them from environmental stressors. Some endoliths are also able to form biofilms, which are protective layers that help shield them from the surrounding environment.
- Overall, endoliths are an important area of study for astrobiology, as they may provide insight into the potential for life on other planets or moons with harsh environments.
Endolith Mode of adaptation
Endoliths have several modes of adaptation that allow them to survive in extreme environments. These include:
- Utilizing mineral resources: Endoliths can use minerals in rocks as a source of energy and nutrients. They can break down minerals using specialized enzymes or obtain energy by oxidizing iron or sulfur compounds.
- Using sunlight: Some endoliths have adapted to use light as a source of energy. They can do this by living in thin cracks in rocks or by producing pigments that allow them to harvest light energy.
- Surviving extreme temperatures: Endoliths can survive in extreme temperatures by slowing down their metabolism and reducing their energy consumption. Some endoliths have also evolved heat-shock proteins that help protect their cellular structures from damage.
- Forming protective biofilms: Endoliths can form biofilms, which are thin layers of cells that protect them from environmental stressors. Biofilms can also help endoliths retain moisture and nutrients in environments with low availability.
- Repairing DNA damage: Endoliths are exposed to high levels of radiation in some environments, which can damage their DNA. However, some endoliths have evolved mechanisms to repair this damage and continue to grow and reproduce.
Overall, endoliths have developed diverse adaptations to survive in extreme environments, making them fascinating subjects for scientific study.
Endolith Examples
Endoliths can be found in a variety of environments around the world. Here are a few examples:
- Antarctic Dry Valleys: In this extremely cold and dry region of Antarctica, endoliths can be found living in rocks and soil. Some of the most common types of endoliths in this environment are cyanobacteria, which are able to survive by using photosynthesis to generate energy.
- Deep sea sediments: In the deep sea, endoliths can be found living within rocks and sediments. These organisms are able to survive in complete darkness and extreme pressure. Some types of endoliths found in deep sea sediments include bacteria, fungi, and archaea.
- Sahara Desert: Endoliths can also be found living in the hot and dry Sahara Desert. These organisms live within rocks and use the minerals within the rocks as a source of energy. One common type of endolith found in the Sahara is the cyanobacterium Chroococcidiopsis.
- Nuclear reactors: Endoliths have even been found living within the walls of nuclear reactors, where they are exposed to high levels of radiation. These organisms are able to survive by repairing DNA damage and using radiation as a source of energy. Some of the endoliths found in nuclear reactors include bacteria and fungi.
Overall, endoliths are able to survive in a wide range of extreme environments, making them a fascinating area of study for scientists interested in astrobiology and extremophile organisms.
2. Hypolith
- Hypoliths are a type of microorganism that live underneath translucent rocks in arid and semi-arid environments. They are commonly found in regions such as deserts, dry river beds, and rocky landscapes.
- Unlike endoliths, which live inside rocks, hypoliths grow on the underside of rocks and rely on the sunlight that passes through the rock for energy. They are typically small, ranging in size from a few millimeters to a few centimeters in diameter.
- Hypoliths are able to survive in these harsh environments due to their ability to adapt to low water availability, high temperatures, and intense UV radiation. They are also able to utilize nutrients in the soil and rock substrate, and some species can even fix nitrogen from the atmosphere.
- Hypolith communities can be very diverse, with different species of bacteria, fungi, and algae coexisting in a single location. They often form colorful mats or patterns on the undersides of rocks, which can be visible from a distance.
- Overall, hypoliths are an important area of study for understanding how microorganisms are able to adapt to extreme environments, and they may have implications for astrobiology and the search for life on other planets.
Hypolith Mode of adaptation
Hypoliths have several modes of adaptation that allow them to survive in arid and semi-arid environments. These include:
- Utilizing sunlight: Hypoliths grow on the undersides of translucent rocks and rely on the sunlight that passes through the rock for energy. They can use photosynthesis to generate energy, and some species have evolved to be more efficient at using light energy in these low-light conditions.
- Reducing water loss: Hypoliths are adapted to low water availability and have evolved to reduce water loss through mechanisms such as small size, thick cell walls, and specialized water-storage structures.
- Nitrogen fixation: Some hypoliths are able to fix nitrogen from the atmosphere, which allows them to utilize this essential nutrient even in environments with very low nitrogen levels.
- Tolerating extreme temperatures: Hypoliths are able to survive in environments with high temperatures by regulating their metabolism and reducing their energy consumption. Some species are also able to produce specialized proteins that protect their cellular structures from damage.
- Using soil and rock substrate: Hypoliths can utilize nutrients in the soil and rock substrate, including minerals and organic matter, to obtain energy and nutrients.
Hypolith Examples
Hypoliths can be found in a variety of arid and semi-arid environments around the world. Here are a few examples:
- Mojave Desert: Hypoliths are common in the Mojave Desert, where they grow on the undersides of translucent quartz rocks. The hypoliths in this region are dominated by cyanobacteria, which are able to use photosynthesis to generate energy.
- Atacama Desert: The Atacama Desert in Chile is one of the driest places on Earth, but hypoliths are able to survive in this harsh environment. The hypoliths in this region are dominated by bacteria and are able to tolerate high levels of UV radiation.
- Antarctica: Hypoliths have even been found in the extreme environments of Antarctica, where they are able to survive in ice-free regions of the continent. The hypoliths in this region are dominated by cyanobacteria, which are able to use photosynthesis to generate energy even in low-light conditions.
- Namib Desert: The Namib Desert in southern Africa is home to a diverse community of hypoliths, including bacteria, fungi, and algae. These hypoliths are able to survive in the harsh desert environment by utilizing nutrients in the soil and rock substrate.
Overall, hypoliths are able to thrive in a wide range of arid and semi-arid environments, making them a fascinating area of study for scientists interested in extremophile organisms and the role they play in maintaining ecosystem function.
H. Microorganisms in heavy metals
- Microorganisms are capable of interacting with heavy metals in various ways. Some microorganisms can tolerate and even thrive in high concentrations of heavy metals, while others are able to transform or remove heavy metals from their environment.
- One way that microorganisms interact with heavy metals is through the process of bioremediation. Bioremediation is the use of microorganisms to degrade, transform, or remove pollutants from the environment. Some microorganisms are able to use heavy metals as an energy source, converting them into less toxic forms.
- Other microorganisms are able to immobilize heavy metals by adsorbing them onto their cell surfaces or by precipitating them out of solution. This can help to reduce the toxicity of heavy metals in the environment.
- Microorganisms can also play a role in the natural cycling of heavy metals in the environment. For example, some microorganisms are able to dissolve or mobilize heavy metals from rocks or minerals, making them available for other organisms to use. Other microorganisms are able to transform or detoxify heavy metals in the soil, making them less harmful to plants and other organisms.
- In addition to their natural interactions with heavy metals, microorganisms are also being used in various industrial and technological applications. For example, some microorganisms are being used in the development of biosensors for detecting heavy metal contamination in water or soil. Others are being used in the development of new materials, such as nanoparticles, that have potential applications in environmental remediation.
- Overall, microorganisms play a complex and important role in the cycling and transformation of heavy metals in the environment, and their interactions with these pollutants have important implications for both environmental health and industrial applications.
1. Metallotolerant
Metallotolerant organisms are those that are able to tolerate high levels of heavy metals in their environment. These organisms have developed a variety of adaptations that allow them to survive and even thrive in environments that are toxic to other organisms.
Some characteristics of metallotolerant organisms include:
- Tolerance to high levels of heavy metals: Metallotolerant organisms are able to survive in environments with high concentrations of heavy metals, often by developing specialized mechanisms for detoxifying or immobilizing these metals.
- Adaptations to low-nutrient environments: Many metallotolerant organisms live in environments that are low in nutrients, such as mine tailings or contaminated soils. As a result, they have evolved to be able to survive in these harsh environments with limited resources.
- Ability to form biofilms: Metallotolerant organisms are often able to form biofilms, which are complex communities of microorganisms that adhere to surfaces. Biofilms can provide protection from toxic metals and help to facilitate the exchange of nutrients and genetic material among the community members.
- High genetic diversity: Metallotolerant organisms are often highly diverse genetically, which allows them to adapt to changing environmental conditions and maintain population stability over time.
- Potential for bioremediation: Some metallotolerant organisms have the ability to degrade or immobilize heavy metals in contaminated environments, making them useful for bioremediation and environmental cleanup efforts.
Overall, metallotolerant organisms are a fascinating group of organisms that have evolved unique adaptations for survival in toxic environments. They have important implications for environmental health and industrial applications, and their study can help us to better understand the diversity and resilience of life on Earth.
Metallotolerant Adaptations – How are metallotolerant adapted to their environment?
Metallotolerant organisms have developed a variety of adaptations that allow them to survive and even thrive in environments with high levels of heavy metals. Some of the common modes of adaptation include:
- Detoxification mechanisms: Metallotolerant organisms are able to detoxify heavy metals by converting them into less toxic forms. This can involve the production of enzymes that break down heavy metals, or the use of specialized transport proteins that pump heavy metals out of the cell.
- Metal sequestration: Some metallotolerant organisms are able to immobilize heavy metals by binding them to specific proteins or peptides. This can prevent the toxic metals from interacting with cellular components and causing damage.
- Biofilm formation: Metallotolerant organisms often form biofilms, which are complex communities of microorganisms that adhere to surfaces. Biofilms can provide protection from toxic metals and help to facilitate the exchange of nutrients and genetic material among the community members.
- Genetic diversity: Metallotolerant organisms often have high genetic diversity, which allows them to adapt to changing environmental conditions and maintain population stability over time. This genetic diversity can be the result of mutation and selection, as well as the exchange of genetic material through horizontal gene transfer.
- Utilization of alternative energy sources: In environments with high levels of heavy metals, traditional energy sources such as glucose may be scarce. Metallotolerant organisms have adapted to these conditions by utilizing alternative energy sources, such as sulfur or iron, to fuel their metabolic processes.
Overall, metallotolerant organisms have developed a variety of unique and complex adaptations that allow them to survive in toxic environments. Their study can help us to better understand the diversity and resilience of life on Earth, and may have important implications for bioremediation and environmental cleanup efforts.
Metallotolerant Examples
There are many examples of metallotolerant organisms, including:
- Acidithiobacillus ferrooxidans: This bacterium is able to tolerate high levels of heavy metals, such as iron and copper, and is commonly found in acidic mine drainage environments. It is known for its ability to oxidize sulfur and iron, and is often used in bioremediation efforts to clean up contaminated soils.
- Pseudomonas aeruginosa: This common soil bacterium is able to tolerate high levels of cadmium and other heavy metals, and is known for its ability to form biofilms. It has been studied for its potential to remove heavy metals from contaminated soils and water.
- Arbuscular mycorrhizal fungi: These fungi form symbiotic relationships with plant roots and are able to tolerate high levels of heavy metals in the soil. They have been studied for their potential to help plants grow in contaminated environments, and to remove heavy metals from the soil.
- Chlorella vulgaris: This green algae is able to tolerate high levels of heavy metals, such as copper and zinc, and has been studied for its potential to remove heavy metals from contaminated water.
- Geobacter sulfurreducens: This bacterium is able to tolerate high levels of heavy metals, such as iron and uranium, and is known for its ability to use metals as electron acceptors in its metabolic processes. It has been studied for its potential to clean up contaminated groundwater.
Overall, metallotolerant organisms are found in a wide range of environments and play important roles in biogeochemical cycles and environmental health. Their study can help us to better understand the diversity and resilience of life on Earth, and may have important implications for bioremediation and environmental cleanup efforts.
I. Microorganisms in extreme Radiation
Microorganisms in extreme radiation environments, such as nuclear waste repositories and outer space, are known as radioresistant organisms. These organisms have developed unique adaptations that allow them to survive in environments with high levels of ionizing radiation. Some of the characteristics of radioresistant microorganisms include:
- DNA repair mechanisms: Radioresistant microorganisms have developed sophisticated DNA repair mechanisms that allow them to quickly repair the damage caused by ionizing radiation. This can include the production of specialized enzymes that repair DNA double-strand breaks, or the use of error-prone DNA polymerases that can bypass damaged regions of DNA.
- Protective pigments: Some radioresistant microorganisms produce pigments, such as carotenoids, that protect their cells from radiation damage. These pigments are able to scavenge free radicals and reactive oxygen species that are produced by ionizing radiation.
- Thick cell walls: Radioresistant microorganisms often have thick cell walls that provide additional protection from ionizing radiation. These cell walls may contain high levels of compounds such as melanin or other radiation-absorbing pigments.
- Spore formation: Some radioresistant microorganisms are able to form spores, which are highly resistant to radiation and other environmental stressors. Spore formation allows the microorganisms to survive in extreme environments for long periods of time, and may facilitate their dispersal to new locations.
- Metabolic flexibility: Radioresistant microorganisms are often able to survive on a wide range of energy sources, including organic and inorganic compounds. This metabolic flexibility allows them to adapt to changing environmental conditions, and may help them to survive in environments with limited nutrients.
Examples of radioresistant microorganisms include Deinococcus radiodurans, which is able to withstand extremely high levels of ionizing radiation, and Thermococcus gammatolerans, which is able to tolerate high levels of both radiation and heat. The study of radioresistant microorganisms has important implications for fields such as bioremediation, astrobiology, and radiation therapy.
1. Radiophile
Radiophiles, also known as radiotrophic organisms, are a group of microorganisms that are able to use ionizing radiation as an energy source. They are typically found in environments with high levels of radiation, such as radioactive waste sites, nuclear reactors, and hot springs. Some of the characteristics of radiophiles include:
- Ability to use radiation as an energy source: Radiophiles are able to use the energy from ionizing radiation to power their metabolic processes. They do this by using pigments or other mechanisms to capture the energy from the radiation and convert it into a usable form.
- Resistance to radiation damage: Radiophiles have developed mechanisms to protect themselves from the harmful effects of ionizing radiation, such as DNA damage and cell death. This can include specialized repair enzymes, antioxidant molecules, or thick cell walls.
- Adaptability to extreme environments: Radiophiles are often able to survive in environments with high levels of radiation, extreme temperatures, and low nutrient availability. They have developed adaptations to allow them to thrive in these challenging conditions.
- Wide range of metabolic pathways: Radiophiles are able to use a wide range of energy sources and metabolic pathways to power their growth and reproduction. They may use photosynthesis, chemosynthesis, or other processes to generate energy.
- Role in bioremediation: Radiophiles have been studied for their potential to clean up radioactive waste sites and contaminated environments. They are able to use the energy from radiation to break down and detoxify harmful compounds, making them an important tool in bioremediation efforts.
Examples of radiophiles include Deinococcus radiodurans, which is known for its extreme resistance to radiation and ability to survive in harsh environments, and Cryptococcus neoformans, a fungus that is able to use melanin pigments to capture the energy from ionizing radiation. The study of radiophiles is an important area of research, with potential applications in biotechnology, bioremediation, and radiation medicine.
Radiophile Mode of adaptation
Radiophiles, also known as radiotrophic organisms, have developed unique adaptations to thrive in environments with high levels of ionizing radiation. Some of the ways in which radiophiles have adapted to their environment include:
- Pigment production: Some radiophiles produce pigments, such as melanin, that are able to capture the energy from ionizing radiation and convert it into a usable form. These pigments act as a type of natural solar panel, allowing the microorganisms to power their metabolic processes.
- DNA repair mechanisms: Radiophiles have developed specialized mechanisms to repair DNA damage caused by ionizing radiation. These mechanisms can include the production of specialized enzymes, such as photolyases, that are able to repair DNA damage caused by ultraviolet radiation.
- Antioxidant production: Radiophiles produce high levels of antioxidants, such as carotenoids, to protect themselves from the damaging effects of free radicals produced by ionizing radiation. These antioxidants scavenge free radicals and prevent them from causing damage to the cell.
- Thick cell walls: Some radiophiles have developed thick cell walls that provide additional protection from ionizing radiation. These cell walls may contain high levels of compounds such as melanin or other radiation-absorbing pigments.
- Metabolic flexibility: Radiophiles are often able to use a wide range of metabolic pathways to generate energy, allowing them to adapt to changing environmental conditions. Some radiophiles are able to use photosynthesis, while others use chemosynthesis or other energy-generating pathways.
Overall, radiophiles have developed a range of adaptations that allow them to thrive in environments with high levels of ionizing radiation. These adaptations have important implications for fields such as bioremediation, radiation medicine, and astrobiology.
Radiophile Examples
There are several examples of radiophiles, which are microorganisms that are able to use ionizing radiation as an energy source. Some of the most well-known examples include:
- Deinococcus radiodurans: This bacterium is known for its extreme resistance to ionizing radiation and ability to survive in harsh environments. It is able to repair DNA damage caused by radiation and can withstand doses of radiation that would be lethal to most other organisms.
- Cryptococcus neoformans: This fungus is able to use melanin pigments to capture energy from ionizing radiation and use it for growth and metabolism. It is also able to produce antioxidants that protect it from the damaging effects of radiation.
- Thermus scotoductus: This bacterium is able to grow at high temperatures and is often found in hot springs and other extreme environments. It is able to use ionizing radiation as an energy source, and can produce pigments that protect it from the harmful effects of radiation.
- Cupriavidus metallidurans: This bacterium is known for its ability to tolerate high levels of heavy metals, but it is also able to use ionizing radiation as an energy source. It is able to repair DNA damage caused by radiation and can survive in environments with high levels of both radiation and heavy metals.
- Rhodopseudomonas palustris: This bacterium is able to use photosynthesis to generate energy, but it is also able to use ionizing radiation as an energy source when light is not available. It is able to produce pigments that allow it to capture energy from radiation and use it for growth and metabolism.
These examples of radiophiles illustrate the wide range of microorganisms that are able to use ionizing radiation as an energy source, and the unique adaptations they have developed to thrive in environments with high levels of radiation.
Applications of Extremophiles
Extremophiles, or microorganisms that are adapted to live in extreme environments, have a wide range of applications in various fields. Some of the most important applications of extremophiles include:
- Bioremediation: Extremophiles are often used in bioremediation efforts to clean up contaminated environments. For example, some extremophiles are able to break down toxic chemicals or heavy metals in polluted soil or water.
- Industrial processes: Extremophiles are used in various industrial processes, such as the production of enzymes or the fermentation of food and beverages. Some extremophiles are able to produce enzymes that are stable at high temperatures or extreme pH levels, making them useful in industrial settings.
- Medical research: Extremophiles are being studied for their potential medical applications, such as the development of new antibiotics or treatments for diseases. Some extremophiles produce compounds that are effective against pathogenic bacteria or viruses.
- Astrobiology: Extremophiles are being studied for their potential applications in astrobiology, the study of life in the universe. Scientists are interested in extremophiles because they may provide clues to the types of organisms that could survive in extreme environments on other planets or moons.
- Environmental monitoring: Extremophiles can be used as bioindicators to monitor environmental conditions in extreme environments. For example, the presence of certain extremophiles can indicate the presence of certain minerals or other conditions in a particular environment.
- Energy production: Extremophiles are being studied for their potential in bioenergy production. Some extremophiles, such as those found in geothermal environments, are able to produce hydrogen gas or other biofuels through their metabolic processes.
- Agriculture: Extremophiles are being investigated for their potential to improve crop yields and resistance to stress. For example, some extremophiles produce compounds that can protect plants from drought, salinity, or other environmental stressors.
- Biotechnology: Extremophiles are used in biotechnology for the production of novel compounds or materials. For example, some extremophiles produce unique proteins or enzymes that can be used in various applications, such as drug discovery or bioplastics production.
- Exploration and mining: Extremophiles are being studied for their potential to aid in the exploration and mining of mineral resources. Some extremophiles are able to survive in environments with high levels of metals or minerals, and could be used to extract valuable resources from these environments.
- Education: Extremophiles can be used as teaching tools to help students learn about the diversity of life on Earth and the adaptations organisms have to survive in extreme environments. They can also inspire curiosity and interest in science and research.
FAQ
What are extremophiles?
Extremophiles are microorganisms that can survive and thrive in extreme environments that are hostile to most other forms of life, such as high temperatures, high pressure, low temperatures, high salinity, acidic or alkaline conditions, or high levels of radiation.
Why are extremophiles important?
Extremophiles are important because they represent a vast and largely untapped reservoir of biological diversity, and their unique adaptations to extreme environments make them valuable resources for scientific research and practical applications in areas such as bioremediation, biotechnology, energy production, and medicine.
How do extremophiles survive in extreme environments?
Extremophiles have evolved a variety of adaptations to survive in extreme environments, such as the ability to produce heat shock proteins, enzymes that function at high temperatures or extreme pH levels, and membranes that are resistant to harsh conditions.
Where are extremophiles found?
Extremophiles are found in a variety of extreme environments around the world, including hot springs, deep sea hydrothermal vents, polar ice caps, salt flats, and acidic or alkaline lakes.
Can extremophiles be harmful to humans?
While some extremophiles produce compounds that are toxic to humans, the vast majority of extremophiles are harmless to humans and many have potential medical applications, such as the production of novel antibiotics or treatments for diseases.
Can extremophiles survive in space?
Some extremophiles have been shown to survive in simulated space conditions and are being studied for their potential applications in astrobiology, the study of life in the universe.
Are extremophiles rare?
Extremophiles are not rare, but they are often difficult to study and isolate due to the extreme conditions in which they live.
How are extremophiles studied?
Extremophiles are studied using a variety of techniques, such as culturing in the laboratory, genetic sequencing, and imaging with microscopy.
Can extremophiles be engineered for specific applications?
Yes, extremophiles can be genetically engineered to produce specific compounds or enzymes for various applications, such as bioremediation or biotechnology.
What is the future of extremophile research?
Extremophile research is a rapidly growing field, and ongoing studies are uncovering new and innovative ways to harness the unique properties of these organisms for practical use in a variety of fields. The future of extremophile research holds exciting potential for new discoveries and applications.
References
- Rampelotto PH. Extremophiles and extreme environments. Life (Basel). 2013 Aug 7;3(3):482-5. doi: 10.3390/life3030482. PMID: 25369817; PMCID: PMC4187170.
- Gómez, F. (2011). Extreme Environment. In: , et al. Encyclopedia of Astrobiology. Springer, Berlin, Heidelberg. https://doi.org/10.1007/978-3-642-11274-4_566
- D’Amico S, Collins T, Marx JC, Feller G, Gerday C. Psychrophilic microorganisms: challenges for life. EMBO Rep. 2006 Apr;7(4):385-9. doi: 10.1038/sj.embor.7400662. PMID: 16585939; PMCID: PMC1456908.
- De Maayer P, Anderson D, Cary C, Cowan DA. Some like it cold: understanding the survival strategies of psychrophiles. EMBO Rep. 2014 May;15(5):508-17. doi: 10.1002/embr.201338170. Epub 2014 Mar 26. PMID: 24671034; PMCID: PMC4210084.
- Berenguer, J. (2011). Thermophile. In: , et al. Encyclopedia of Astrobiology. Springer, Berlin, Heidelberg. https://doi.org/10.1007/978-3-642-11274-4_1583
- Berezovsky IN, Shakhnovich EI. Physics and evolution of thermophilic adaptation. Proc Natl Acad Sci U S A. 2005 Sep 6;102(36):12742-7. doi: 10.1073/pnas.0503890102. Epub 2005 Aug 24. PMID: 16120678; PMCID: PMC1189736.
- Sterner R, Liebl W. Thermophilic adaptation of proteins. Crit Rev Biochem Mol Biol. 2001;36(1):39-106. doi: 10.1080/20014091074174. PMID: 11256505.
- Holden, J. F., Summit, M., & Baross, J. A. (1998). Thermophilic and hyperthermophilic microorganisms in 3–30°C hydrothermal fluids following a deep-sea volcanic eruption. FEMS Microbiology Ecology, 25(1), 33–41. doi:10.1111/j.1574-6941.1998.tb00458.x
- Coker JA. Extremophiles and biotechnology: current uses and prospects. F1000Res. 2016 Mar 24;5:F1000 Faculty Rev-396. doi: 10.12688/f1000research.7432.1. PMID: 27019700; PMCID: PMC4806705.
- Johnson DB, Ghauri MA, Said MF. Isolation and characterization of an acidophilic, heterotrophic bacterium capable of oxidizing ferrous iron. Appl Environ Microbiol. 1992 May;58(5):1423-8. doi: 10.1128/aem.58.5.1423-1428.1992. PMID: 1622207; PMCID: PMC195620.
- Johnson, D. B., & Schippers, A. (2017). Editorial: Recent Advances in Acidophile Microbiology: Fundamentals and Applications. Frontiers in Microbiology, 8. doi:10.3389/fmicb.2017.00428
- Mirete, Salvador & Morgante, Verónica & Gonzalez-Pastor, Eduardo. (2017). Acidophiles: Diversity and Mechanisms of Adaptation to Acidic Environments. 10.1007/978-3-319-48327-6_9.
- Mamo, G., & Mattiasson, B. (2020). Alkaliphiles: The Versatile Tools in Biotechnology. Advances in Biochemical Engineering/Biotechnology. doi:10.1007/10_2020_126
- https://nap.nationalacademies.org/read/9895/chapter/6
- https://www.sciencedirect.com/topics/engineering/extreme-environment
- https://space.nss.org/life-in-extreme-environments/
- https://oceanservice.noaa.gov/facts/extremophile.html
- https://www.northumbria.ac.uk/research/idrts/extreme-environments/
- https://www.oxfordbibliographies.com/display/document/obo-9780199830060/obo-9780199830060-0152.xml
- https://learn.genetics.utah.edu/content/astrobiology/environments/
- https://www.researchgate.net/publication/12097896_Life_in_extreme_environments
- https://www.researchgate.net/publication/12097896_Life_in_extreme_environments
- https://serc.carleton.edu/microbelife/extreme/environments.html
- https://www.sciencelearn.org.nz/videos/2094-what-is-an-extreme-environment