What is Metazoa?
- Metazoa, also known as the animal kingdom, encompasses all animals that are multicellular and possess differentiated tissues. These organisms are characterized by their complex cellular structures and specific functions, making them distinct from single-celled organisms like protozoa. The term “Metazoa” covers a vast array of animals, from simple creatures like jellyfish to more complex mammals such as humans.
- One defining feature of metazoans is their multicellularity, meaning they are made up of many cells organized into tissues and organs. This cellular organization allows for the division of labor within the organism, where specific cells perform specific roles. For instance, nerve cells (neurons) are specialized for transmitting signals, while muscle cells are responsible for movement.
- Metazoans are heterotrophic, which means they acquire energy by consuming other organisms or organic matter. They are incapable of producing their own food, unlike plants, which use photosynthesis. Additionally, all metazoans are eukaryotic, meaning their cells contain a true nucleus and membrane-bound organelles. This is a key difference from prokaryotic organisms like bacteria, which lack these structures.
- Another hallmark of metazoans is their specialized cells, which are crucial for carrying out specific functions within the body. This specialization is what allows animals to perform complex behaviors and processes, such as digestion, reproduction, and sensory perception. For example, nerve cells help in communication between different parts of the body, while muscle cells facilitate movement.
- Examples of metazoans span a wide range of animal species. This group includes mammals like humans, dogs, and cats, birds, reptiles, amphibians, fish, insects, and more primitive forms like worms and jellyfish. Essentially, any organism you can think of, aside from single-celled organisms such as amoebas, belongs to the Metazoa group.
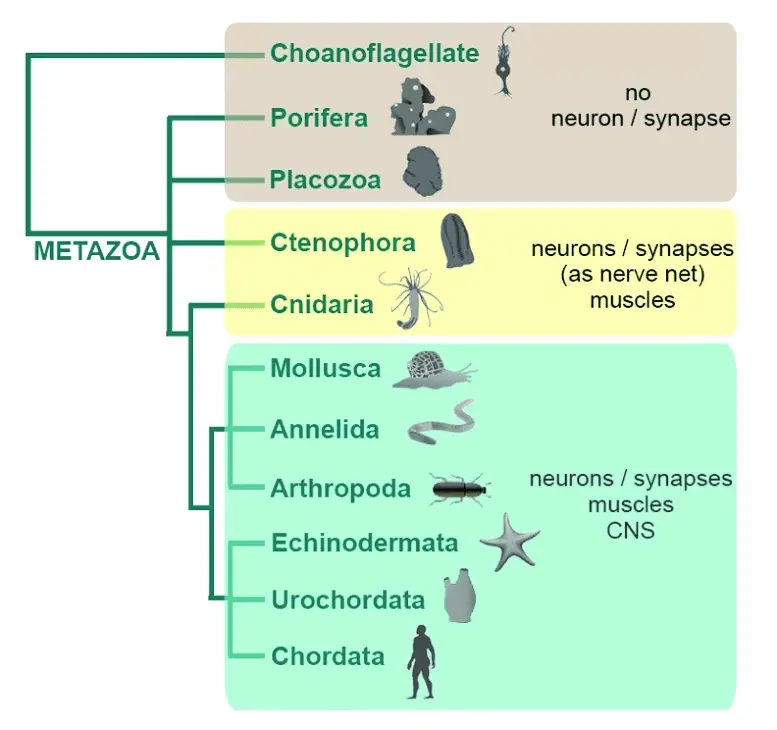
Characteristics of metazoa
Metazoa, or multicellular animals, exhibit a range of distinctive characteristics that differentiate them from other life forms. Understanding these features provides insight into their complex biological organization and functional diversity. The following points outline the key characteristics of metazoans:
- Complex Multicellular Organization: Metazoans are composed of multiple cells that are intricately organized into tissues, organs, and organ systems. This structural complexity allows for specialized functions and efficient physiological processes within the organism.
- Embryonic Development Stages: The life cycle of metazoans typically begins with a fertilized egg that undergoes a blastula stage during early embryonic development. This stage involves the formation of a hollow, spherical structure of cells, which subsequently differentiates into the various cell types that constitute the adult organism.
- Size and Nutritional Requirements: Being multicellular, metazoans are generally larger than unicellular protozoans. Their increased size necessitates higher nutritional intake, leading them to actively seek out food sources. To meet these demands, metazoans have developed advanced locomotion mechanisms.
- Advanced Locomotion Systems: The ability to move efficiently is a hallmark of metazoans. They possess contractile muscular elements and sophisticated nervous systems that facilitate coordinated movement. This enhanced locomotion capability not only aids in feeding and escaping predators but also influences their overall body shape and symmetry.
- Symmetry and Body Structure: Locomotion has a significant impact on the morphology of metazoans, resulting in specific types of body symmetry such as bilateral or radial symmetry. These symmetrical arrangements are closely linked to their movement patterns and environmental interactions.
- Cephalization and Nervous System Centralization: Most metazoans exhibit cephalization, which is the differentiation of the anterior end into a head region. This specialization is accompanied by the centralization of the nervous system within the head, enhancing sensory perception and processing capabilities. Cephalization supports more complex behaviors and interactions with the environment.
Levels of body Organisation
The levels of body organization in living organisms illustrate a progression from simple to highly complex structures as organisms evolved. These levels reflect the arrangement of biological components, ranging from basic units to intricate systems that support life. Below are the key stages of body organization:
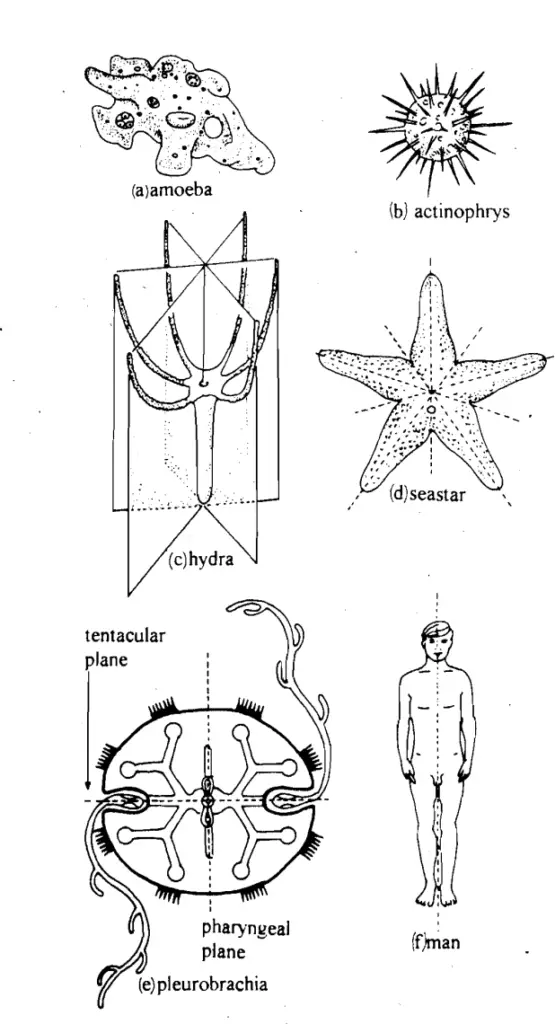
- Protoplasmic Level: The most primitive and simplest level of organization is found in unicellular organisms. At this level, the entire organism is made up of a single cell that performs all the essential life functions. These organisms, such as protozoans, operate at the protoplasmic level, where the cytoplasm carries out all functions necessary for survival, including metabolism, reproduction, and locomotion. This level forms the base of the organizational pyramid.
- Cellular Level: As organisms evolved from unicellular to multicellular forms, the next step in complexity is the cellular level of organization. In this stage, there is an aggregation of cells, but these cells are functionally differentiated. While individual cells may take on specialized roles such as reproduction or nutrient absorption, they do not yet form true tissues. Metazoans like Placozoa and Mesozoa belong to this category, with some researchers also classifying sponges (Porifera) at this level due to their variety of cell types, though they lack true tissue organization.
- Tissue Level: The tissue level of organization marks a significant advancement, where groups of similar cells work together to perform a specific function. This stage is evident in organisms such as Cnidarians (e.g., jellyfish) and Ctenophores, where cells are organized into two germ layers—ectoderm and endoderm—that form the epidermis and gastrodermis, respectively. For instance, in Cnidarians, a nerve net made up of nerve cells and their processes exemplifies tissue-level organization, enabling the organism to coordinate responses and interact with its environment.
- Organ Level: The next progression in complexity is the organ level of body organization. Here, organs are formed by multiple tissue types working together to perform a particular function. This level is observed in some Cnidarians, Ctenophores, and flatworms (Platyhelminthes), where distinct organs like the eyes and reproductive structures are present. These organs are responsible for specific tasks that contribute to the organism’s overall function and survival.
- Organ System Level: The highest level of body organization is the organ system level, where multiple organs work in concert to form systems that manage vital life processes. This level first appears in Platyhelminthes, where separate systems, such as the digestive and reproductive systems, operate independently yet harmoniously within the organism. From this point onwards, all higher animals, including mammals, exhibit the organ system level of organization, where complex systems such as the circulatory, respiratory, and nervous systems interact to sustain life.
Symmetry
In biological organisms, symmetry refers to the arrangement of body parts or organs around an axis or plane, resulting in mirror images on opposite sides. It is a fundamental aspect of an animal’s body plan, influencing both form and function. Symmetry in animals can be categorized into primary (embryonic) and secondary (adult) symmetry, which may differ as the organism matures. For example, the larval stage of a starfish exhibits bilateral symmetry, while the adult displays radial symmetry. The types of symmetry found in animals are as follows:
- Asymmetrical: Asymmetry occurs when an organism cannot be divided into identical halves by any plane. No symmetry is present in these animals, making each side unique. Examples include Amoeba and certain Porifera (sponges), which lack any consistent pattern or symmetry in their structure.
- Spherical Symmetry: Organisms with spherical symmetry can be divided into identical halves by any plane that passes through the center of the body. This form of symmetry is rare and primarily found in certain protozoans. For instance, Actinophrys and Volvox exhibit this type of symmetry, where all planes through the central point create symmetrical halves.
- Radial Symmetry: Radial symmetry is characterized by body parts arranged around a central axis, similar to the spokes of a wheel. Any vertical cut through this axis will yield two symmetrical halves. This type of symmetry is common in Cnidarians, such as jellyfish and hydras, and is adapted to sessile or slow-moving lifestyles. A modified form, pentamerous symmetry, is found in organisms like starfish, which can be divided into five symmetrical parts along specific planes.
- Biradial Symmetry: A variant of radial symmetry, biradial symmetry is found in organisms like sea anemones and ctenophores. Although these animals appear radially symmetrical, they can only be divided into two identical halves along specific planes, such as the tentacular and sagittal planes. This creates a blend of radial and bilateral features.
- Bilateral Symmetry: Bilateral symmetry is the most common type of symmetry in animals, including humans. Organisms with this form of symmetry have a single plane (sagittal) that divides the body into right and left halves, each a mirror image of the other. Bilaterally symmetrical animals, known as Bilateria, possess distinct dorsal (upper) and ventral (lower) surfaces, as well as anterior (head) and posterior (tail) ends. This symmetry is associated with more advanced movement and the development of a head (cephalization). Most animals, including vertebrates and many invertebrates, exhibit this type of symmetry. Interestingly, adult echinoderms (e.g., starfish) show radial symmetry, but their larvae are bilaterally symmetrical, reflecting their evolutionary history.
Developmental patterns
Developmental patterns in animals provide insight into the processes that shape their early embryonic development. The two primary divisions of bilaterally symmetrical animals—Protostomes and Deuterostomes—are based on these developmental characteristics. The distinction between these groups lies primarily in their cleavage patterns and the fate of the blastopore during gastrulation. The following points detail the key aspects of developmental patterns:
- Cleavage:
- In all animals, the single-celled zygote undergoes cleavage, a series of rapid cell divisions, resulting in a ball of cells called blastomeres. The first two cleavage planes are vertical, and the third is horizontal, leading to a structure of eight blastomeres.
- Radial cleavage occurs in some animals, such as echinoderms, cnidarians, and chordates. In this pattern, the blastomeres are aligned directly on top of each other, forming distinct layers. The blastomeres are indeterminate or regulative, meaning that each cell retains the potential to develop into a complete organism if separated. Therefore, the early cells’ positions do not determine their future roles.
- In contrast, spiral cleavage occurs in groups such as annelids, mollusks, and arthropods. Here, the blastomeres are arranged in a spiral pattern, with cells positioned above the furrows between others. Spiral cleavage is determinate, meaning the fate of each blastomere is fixed early in development. If separated, these cells form incomplete, defective embryos, as their developmental roles are predefined from the outset.
- Fate of the Blastopore:
- Following cleavage, the ball of cells becomes a morula and later develops into a hollow sphere called the blastula, which contains a fluid-filled cavity known as the blastocoel. As development continues, the blastula undergoes invagination, forming a double-walled structure called the gastrula. The opening of the developing digestive cavity, the blastopore, determines the eventual fate of the mouth and anus.
- In Protostomes, including platyhelminths, nematodes, annelids, arthropods, and mollusks, the blastopore becomes the mouth, with the anus forming secondarily. This characteristic defines their classification, as “protostome” translates to “mouth first.”
- In Deuterostomes, such as echinoderms, hemichordates, and chordates, the blastopore becomes the anus, and the mouth forms independently later in development. The term “deuterostome” means “mouth second,” reflecting this developmental pattern.
- Evolutionary Implications:
- The cleavage pattern and fate of the blastopore distinguish two major evolutionary lineages within the animal kingdom. Protostomes generally exhibit spiral cleavage and determinate development, while Deuterostomes demonstrate radial cleavage and indeterminate development. This distinction underscores the fundamental differences in early development between these two groups.
Germ layers
In animal development, germ layers are the primary tissue layers that form during embryogenesis, giving rise to all the organs and tissues in an organism. These layers are initially established during the formation of the gastrula from the blastula, and the three germ layers—ectoderm, endoderm, and mesoderm—each play distinct roles in forming the various structures of the body. In simpler organisms, like cnidarians and ctenophores, only two germ layers are present, while more complex animals, from platyhelminths to mammals, have all three germ layers, making them triploblastic. The following points explain the contributions of these layers to animal development:
- Diploblastic Animals:
- Organisms in the phyla Cnidaria and Ctenophora have two primary germ layers, the ectoderm and the endoderm. A mesoglea, which is a non-cellular, jelly-like substance, separates these two layers. This structure binds the ectoderm and endoderm but should not be confused with the mesoderm, which is absent in diploblastic animals.
- For example, in Hydra, a cross-section would reveal two distinct layers, with the mesoglea acting as the adhesive between them. The ectoderm forms the animal’s outer covering, while the endoderm, or gastroderm, lines the internal digestive cavity.
- Triploblastic Animals:
- All animals beyond cnidarians and ctenophores, including worms, insects, and mammals, possess three germ layers. These animals are called triploblastic because, in addition to the ectoderm and endoderm, a mesoderm develops between these two layers.
- In more complex organisms, such as a horse, the germ layers do not remain visibly distinct throughout life. Instead, during early embryonic development, the layers differentiate and give rise to specialized tissues and organs. Thus, the germ layers are most prominent during embryogenesis and are often referred to as embryonic layers.
- Fate of Germ Layers:
- Each of the three germ layers contributes to specific body structures:
- Ectoderm:
- Forms the epidermis (outer layer of skin), brain, spinal cord, nerves, and sensory structures such as the eyes and inner ear.
- It also produces various epithelial tissues, such as those lining the oral cavity, nasal passages, and anal canal, as well as external structures like hair, nails, and feathers in animals.
- Other ectodermal derivatives include glands like sweat, sebaceous, and mammary glands, and endocrine structures such as the adrenal medulla and pituitary gland.
- Endoderm:
- Gives rise to the epithelial lining of the digestive tract (excluding the mouth and anal canal), the respiratory system (including the lungs), and parts of the urinary and reproductive systems.
- It also forms the epithelial components of glands like the thyroid, thymus, and pancreas.
- Mesoderm:
- Responsible for forming the dermis (the inner layer of skin), skeletal system, muscular system, circulatory system, and connective tissues.
- It also contributes to the development of the urinary and genital systems, including the gonads and adrenal cortex.
- Additionally, the mesoderm produces mesenteries, which support the internal organs, and lines the coelom, the body cavity found in many triploblastic animals.
- Ectoderm:
- Each of the three germ layers contributes to specific body structures:
Body cavity and coelom
The body cavity, or coelom, is a critical aspect of animal anatomy, playing a significant role in the organization and function of internal systems. A coelom is defined as a large, fluid-filled cavity located between the outer body wall and the inner digestive tube. It is formed during embryonic development as a secondary cavity between two layers of mesoderm, specifically characterized by a lining known as peritoneum. This anatomical feature is crucial for many visceral functions and supports the overall complexity of animal life.
- Body Cavity Overview:
- The term “body cavity” encompasses any internal space within an organism.
- The coelom specifically refers to a true body cavity formed by the splitting of mesoderm during embryonic development.
- It contains coelomic fluid, excretory organs, and reproductive structures, with the potential for a more complex body plan than acoelomate organisms.
- Types of Coelom:
- Two main types of body cavities exist in Bilateria: primary (or pseudocoelom) and secondary (true coelom).
- Primary Coelom (Pseudocoelom):
- This cavity is derived from the blastocoel and persists throughout life.
- It is not bounded by mesoderm, allowing internal organs to remain free.
- Commonly found in worm-like organisms, including roundworms and rotifers.
- Secondary Coelom (True Coelom):
- Develops within the mesoderm, with a peritoneum lining that surrounds internal organs.
- Represents a significant evolutionary advancement, first evident in annelids.
- Primary Coelom (Pseudocoelom):
- Two main types of body cavities exist in Bilateria: primary (or pseudocoelom) and secondary (true coelom).
- Classification of Metazoa Based on Coelom:
- Metazoans can be categorized into three groups based on the presence or absence of a coelom:
- Acoelomata:
- No body cavity exists; mesoderm remains a solid layer.
- Examples include sponges, cnidarians, and flatworms.
- Pseudocoelomata:
- Characterized by a pseudocoelom, with internal organs free within this cavity.
- Examples include rotifers and nematodes.
- Coelomata:
- True coelom present, lined by mesoderm on both sides.
- This group encompasses most Bilateria, including annelids and arthropods.
- Acoelomata:
- Metazoans can be categorized into three groups based on the presence or absence of a coelom:
- Coelomic Formation in Development:
- Coelom formation occurs through various embryological processes, categorized into:
- Schizocoelomata:
- Arises by splitting mesodermal bands, common in protostomes (e.g., annelids, mollusks).
- Mesenchymal Coelomata:
- Found in Phoronida, where mesenchymal cells create the coelomic space.
- Enterocoelomata:
- Forms from mesodermal pouches derived from the archenteron, typical in deuterostomes (e.g., echinoderms, chordates).
- Schizocoelomata:
- Coelom formation occurs through various embryological processes, categorized into:
- Modifications of Coelom:
- Coelom structures vary among different animal groups, showing evolutionary adaptations:
- In some annelids, the coelom is divided into compartments by septa.
- In arthropods, the body cavity becomes a hemocoel, where blood circulates.
- Mammals exhibit a coelom divided by a diaphragm into distinct thoracic and abdominal cavities.
- Coelom structures vary among different animal groups, showing evolutionary adaptations:
Coelomic division of Metazoa
The coelomic division of Metazoa is a fundamental classification that distinguishes animals based on the presence and structure of their body cavities. Understanding this division is crucial for appreciating the evolutionary relationships and physiological adaptations among various animal groups. The classification is based primarily on the type of body cavity—if present—and its developmental origins.
- Acoelomata:
- In this category, animals lack a true body cavity. Instead, they exhibit solid mesodermal tissue throughout their bodies, without any coelomic space. The embryonic mesoderm remains solid and is often referred to as mesenchyme, which fills the spaces between the endoderm (gut wall) and ectoderm (body wall).
- Examples: This group includes simpler organisms such as Porifera, Coelenterata, Ctenophora, and Platyhelminthes.
- Additional Information: In these organisms, body functions are supported through diffusion due to the absence of a dedicated circulatory system.
- Pseudocoelomata:
- Members of this group possess a pseudocoelom, which is a “false” coelom. This body cavity is a persistent blastocoel, situated between the outer ectoderm and inner endoderm, but it is not lined by mesoderm. The structure allows for some degree of organ movement and flexibility but does not provide the same level of organization found in true coeloms.
- Examples: Common representatives include Acanthocephala, Ectoprocta, and various Aschelminthes such as Rotifera, Gastrotricha, Kinorhyncha, Nematoda, and Nematomorpha.
- Additional Information: The pseudocoelomates exhibit varying degrees of complexity and often have a hemocoel, which is a fluid-filled space that functions somewhat like a circulatory system.
- Coelomata (Eucoelomata):
- This group is characterized by the presence of a true coelom, which is a body cavity completely enclosed by mesodermal tissue on both sides. This arrangement allows for more complex organ development and physiological processes.
- Examples: All remaining phyla of Bilateria, including Annelida, Arthropoda, and Mollusca, fall under this category.
- Additional Information: The true coelom is further classified based on its embryological origins, with three primary subcategories defined by Hyman (1951):
- Schizocoelomata:
- In this group, the coelom forms through the splitting of endomesodermal bands that arise from the blastoporal region of the larva. This process creates a true coelom known as a schizocoel.
- Examples: Most protostomes, including annelids, arthropods, and mollusks, exhibit this developmental pattern.
- Mesenchymal Coelomata:
- This rare form is observed only in the phylum Phoronida, where mesenchymal cells rearrange to enclose a coelomic space, which is considered an aberrant form of a schizocoel.
- Enterocoelomata:
- In this category, the coelom arises from mesodermal pouches that form from the larval archenteron. After separating from the endoderm, these pouches fuse and expand, eventually contacting both the gut and body wall. Since this type of coelom originates from the larval enteron, it is termed an enterocoel.
- Examples: Deuterostomes such as Chaetognatha, Echinodermata, Hemichordata, Chordata, and Brachiopoda exhibit this coelomic formation.
Significance of coelom
The significance of the coelom in animal evolution is profound, reflecting an essential advancement in structural complexity and functional capabilities. The coelom, a fluid-filled body cavity found in triploblastic animals, particularly in coelomates, plays a critical role in facilitating various physiological processes. Its presence not only marks a developmental leap but also contributes to enhanced adaptability and diversity among metazoans.
- Structural Protection:
- The coelom surrounds internal organs, acting as a protective layer akin to a “water jacket.” This cushioning effect safeguards delicate structures from external mechanical shocks, which is vital for maintaining organ integrity during locomotion and environmental interactions.
- Increased Flexibility:
- The presence of a coelom enhances body flexibility. This cavity allows the digestive tract and other internal organs to move independently of the body wall. Such freedom of movement facilitates the enlargement of organs, their further differentiation, and overall increased activity, allowing for more complex behaviors and interactions with the environment.
- Hydraulic Skeleton:
- Coelomic fluid often functions as a hydraulic skeleton. This allows for effective movement and support without rigid skeletal structures. The fluid dynamics within the coelom help to maintain body shape while enabling various locomotor functions, crucial for organisms that lack hard exoskeletons or endoskeletons.
- Circulatory Medium:
- The coelomic fluid serves as a circulatory medium, aiding in the transport and distribution of nutrients and gases throughout the body. This function is particularly important for larger organisms, where simple diffusion is inadequate for sustaining metabolic needs.
- Excretory Functions:
- Reproductive Development:
- Gonads develop from the coelomic epithelium, extending into the coelom. This structural organization allows for the efficient extrusion of gametes (ova and sperm) through specialized gonoducts that connect the coelom to the external environment. Such a mechanism ensures successful reproduction and genetic diversity.
- Evolutionary Advancements:
- The evolution of the coelom marks a significant transition in the evolution of Metazoa, as evidenced by the increased size, complexity, and diversity of coelomate phyla compared to acoelomate and pseudocoelomate groups. This evolutionary milestone has allowed for a broader range of life strategies and adaptations, enabling organisms to thrive in various habitats.
Evolútion of coelom (Theories of Origin)
The evolution of the coelom in Metazoa is a significant topic in understanding the complexity of animal life. This development is linked to various evolutionary adaptations and is a matter of considerable discussion among biologists. The origins of the coelom are not directly traceable through paleontology; instead, researchers have relied on embryological evidence from contemporary metazoans. This indirect evidence has led to the formulation of several theories, each offering a unique perspective on the emergence and evolution of the coelom.
- Enterocoel Theory:
- This theory posits that the coelom originated through an enterocoelous process, suggesting that it developed from pouches of the archenteron (the primitive gut).
- Initially proposed by Lankester in 1875 and modified by subsequent researchers such as Sedgwick, Hartman, and Ramne, it argues that gastric pockets in a bilateral ancestor to coelenterates eventually separated from the digestive cavity, forming coelomic structures.
- However, the theory faces several criticisms:
- The gastric pouches observed in advanced coelenterates like Scyphozoa and Anthozoa are deemed unsuitable as ancestral structures.
- The sealing of these gastric pockets would undermine their primary role of enhancing the surface area for digestion.
- Furthermore, gastric pockets arise as evaginations of the gut endoderm rather than through the ingrowth of the body wall.
- Gonocoel Theory:
- Proposed in 1885 by Bergh, this widely accepted theory suggests that the coelom is a persistent gonadal cavity or gonocoel.
- It claims that the coelom evolved from a singular, large pair of gonads, with early theories indicating it may have initially been unsegmented.
- Supporters, such as Meyer, Bergh, and Lang, observed structures in certain species, like the nemerteans and the flatworm Procerodes lobata, that exhibited intestinal branches alternating with gonads.
- Critics of this theory argue that:
- It erroneously links coelom formation with metameric segmentation, a connection not supported phylogenetically.
- Embryological evidence suggests that gonads develop after the coelom.
- It fails to account for the numerous unsegmented coelomate phyla, which dominate the animal kingdom.
- Nephrocoel Theory:
- This theory, suggested by Lankester in 1874, asserts that the coelom originated from the expanded end of a nephridium (an excretory organ).
- However, this theory is largely discounted due to two key issues:
- Protonephridia, the simplest form of excretory system, exist in coelomates, indicating that the nephric structures can be independent of coelom formation.
- Some coelomate groups, like Echinodermata, lack any form of excretory organ, challenging the theory’s validity.
- Schizocoel Theory:
- This theory posits that the coelom arises through a mesenchymal origin, indicating that it is unrelated to the nephridia or gonads of Lower Bilateria, as well as the endodermal pouches in Radiata.
- Proponents of this theory highlight its independence from the other proposed origins of the coelom.
Cephalisation and segmentation
The concept of the body cavity and coelom is crucial to understanding the internal organization of animals, particularly in bilateral animals, which typically exhibit a structured division of the body. These cavities provide not only structural support but also contribute to various physiological functions. Below is a detailed explanation of body cavities and coelom, integrating various sources for a comprehensive understanding.
- Body cavity in bilateral animals:
- Bilateral animals often exhibit a tendency to maintain a consistent orientation during movement, with one end forward and the other towards the substratum. This leads to the development of cephalization, where the sensory organs and nervous system are concentrated at the anterior end. Consequently, this ‘head end’ typically also houses the mouth and organs involved in food capture, enabling efficient detection and processing of food. Over time, these structures, along with neurons forming the brain, evolve for faster coordination and enhanced response to environmental stimuli.
- Segmentation and its role in body form:
- Many animals show segmentation or metamerism, a division of the body into transverse compartments along the anterior-posterior axis. Segmentation can vary in complexity across species, from superficial segmentation, like that seen in tapeworms, to more intricate and true segmentation in annelids and arthropods.
- In segmented animals, the anterior and posterior regions are specialized, with prostomium at the head and pygidium or telson at the tail. Segments in between are often highly replicated, with appendages, musculature, nerves, and blood vessels present in each compartment. This setup allows for both structural and functional specialization.
- Body cavity types and their significance:
- Animals can be grouped based on the type of body cavity they possess:
- Monomeric: In this form, there is no division of the body cavity. An example is Ascaris, which exhibits a single, undivided body cavity.
- Oligomeric: In organisms like Phoronida, the body cavity is divided into distinct regions, though without divisions within the abdomen. Each body region operates independently, allowing for functional separation.
- Metameric: In this form, seen in animals like annelids and arthropods, the body is divided into distinct segments, including a head, thorax, and abdomen, with the abdomen further segmented. This segmentation allows for the regional specialization of segments.
- Animals can be grouped based on the type of body cavity they possess:
- Superficial vs. true segmentation:
- In some organisms, such as tapeworms, segmentation appears superficial, with external ring-like creases forming in the body wall to enable bending and flexibility. This superficial segmentation is an adaptation primarily for reproduction, as each segment functions independently and can be discarded after maturation.
- True segmentation, as seen in annelids, involves the development of distinct body compartments, each with its own coelomic cavity of mesodermal origin. These segments arise in linear sequence from a proliferation region near the posterior, with replication of internal structures in each segment.
- Advantages of segmentation:
- The evolution of segmentation provides animals with significant advantages, particularly in terms of locomotion and specialization. Since each body compartment can be regulated independently, segments can specialize to perform different functions. For example, in annelids, gonads may be restricted to specific segments, while in insects, appendages may be modified for distinct tasks, such as locomotion or food processing.
- Another advantage of segmentation is improved locomotory efficiency. In soft-bodied animals, the coelomic cavity serves as a hydrostatic skeleton, allowing muscles to act against the pressure of coelomic fluid. By compartmentalizing the body, segmentation enables animals to elongate or widen specific sections of the body, enhancing movement, particularly in burrowing animals like worms.
- Regional specialization in segmented animals:
- As animals evolve, regional specialization becomes more pronounced. This process can occur through:
- Restriction of certain structures to specific segments, such as the confinement of gonads to particular regions in earthworms.
- Structural divergence of segmental appendages, with some evolving for locomotion and others for specialized tasks like grasping or chewing, as seen in insects.
- Fusion of segments to form complex body parts, such as the head of Nereis, which consists of three segments, or the head of Drosophila, which is composed of five segments.
- As animals evolve, regional specialization becomes more pronounced. This process can occur through:
- Impact on locomotion:
- In segmented animals, locomotion is enhanced by the ability to control movement in individual segments. In annelids, for example, contraction of circular muscles leads to elongation of the body, while contraction of longitudinal muscles causes widening. This movement can be restricted to specific segments, allowing animals to anchor parts of their body while extending others, resulting in more efficient movement, particularly in burrowing and soft-bodied organisms.
Origin and evolution of metazoa
The body cavity and coelom represent significant evolutionary advancements in metazoans, particularly concerning their structural organization and functional capabilities. These features are crucial for understanding the complexity of multicellular organisms, including roundworms. This overview synthesizes various aspects of body cavities and coeloms in a manner beneficial for students and educators.
- Definition of Body Cavity: The body cavity, or coelom, is a fluid-filled space that lies between the body wall and the digestive tract. It serves several essential functions, including providing space for the development of internal organs, facilitating the movement of body fluids, and serving as a hydrostatic skeleton for support and movement.
- Types of Body Cavities:
- Acoelomate: Organisms, such as flatworms, that lack a body cavity. Their bodies are solid, with no space between the gut and the body wall.
- Pseudocoelomate: Organisms, like roundworms, that possess a pseudocoel, which is a body cavity that is not completely lined by mesodermal tissue. This cavity allows for greater mobility of internal organs and facilitates the circulation of nutrients and waste.
- Coelomate: Animals with a true coelom, which is entirely lined with mesoderm, providing a more complex and organized structure for their internal organs.
- Development of the Coelom:
- The formation of the coelom typically occurs during embryonic development, originating from the mesoderm layer.
- Various theories explain the evolution of multicellular organisms and the development of the coelom:
- Syncytial Theory: Proposes that ancestral metazoans arose from a multinucleate organism through compartmentalization.
- Colonial Theory: Suggests that metazoans developed from colonial flagellates that specialized into different cell types, establishing interdependencies.
- Polyphyletic Theory: Argues that metazoans originated from multiple unrelated ancestral groups.
- Functions of the Coelom in Roundworms:
- Hydrostatic Support: The pseudocoel acts as a hydrostatic skeleton, allowing roundworms to maintain their shape and enabling movement through muscle contractions.
- Organ Development: The pseudocoel provides a space for the development and organization of internal organs, facilitating their function and coordination.
- Circulation: Although roundworms lack a circulatory system, the pseudocoel allows for the diffusion of nutrients and waste products, aiding in the overall metabolic processes.
- Evolutionary Significance: The presence of a body cavity marks a significant evolutionary milestone, enabling more complex body plans and facilitating greater functional specialization. This advancement enhances the organism’s ability to interact with its environment and optimize its physiological processes.
Syncytial Theory
The Syncytial Theory presents an intriguing perspective on the origin of metazoans, positing that ancestral organisms were initially syncytial, characterized by a multinucleate structure lacking distinct cell membranes. Over time, these organisms underwent cellularization, leading to the development of true multicellular bodies. This theory has been notably supported by researchers like Hadzi and Hanson.
- Core Concept:
- The Syncytial Theory proposes that the earliest metazoans were composed of a single, multinucleate cell. This structure could have resulted from repeated nuclear divisions without corresponding cell division, leading to a syncytial arrangement.
- Subsequently, individual cell membranes formed around each nucleus, giving rise to a multicellular organism.
- Supporting Evidence:
- Advocates of the theory suggest that many modern ciliates exhibit bilateral symmetry, which aligns with the idea that the ancestral metazoan shared similar symmetry traits with contemporary acoelous flatworms.
- Key points of support for this theory include:
- Size Range: Acoelous flatworms fall within the same size range as certain ciliates, suggesting a potential ancestral link.
- Bilateral Symmetry: Acoelous flatworms display bilateral symmetry, mirroring the hypothesized characteristics of early metazoans.
- Ciliation: The presence of cilia in both acoelous flatworms and certain ciliates further supports the notion of a common ancestry.
- Syncytial Condition: Acoelous flatworms tend to exhibit conditions akin to syncytial structures, reinforcing the argument for a transition from syncytium to cellularity.
- Objections to the Theory:
- Despite its appeal, the Syncytial Theory faces several criticisms:
- Embryological Evidence: Critics argue that the embryological development of flatworms does not exhibit processes similar to cellularization, challenging the foundational premise of the theory.
- Sperm Structure: The presence of flagellated sperm in metazoans is not adequately explained by this theory, raising questions about reproductive evolution.
- Assumptions on Primitive Lineages: The theory presumes that acoelous flatworms represent the most primitive metazoans, inferring that bilateral symmetry is a more basal trait than radial symmetry. However, this assumption conflicts with the accepted understanding that radial symmetry is, in fact, a more primitive characteristic in the evolutionary hierarchy.
- Radial Coelenterates: If radial symmetry is primitive, the theory does not satisfactorily explain how radial coelenterates could have evolved from bilateral flatworms.
- Despite its appeal, the Syncytial Theory faces several criticisms:
- Implications for Evolutionary Understanding:
- The Syncytial Theory emphasizes the complexity of evolutionary pathways leading to multicellular organisms.
- It challenges researchers to explore alternative explanations for the emergence of multicellularity, considering both cellular and evolutionary factors.
Colonial Theory
The Colonial Theory is a prominent explanation regarding the origin of metazoans, proposing that multicellular organisms evolved from colonial flagellates through processes of cellular specialization and interdependence. Initially conceived by Ernst Haeckel and later modified by scientists like Metschnikoff and Hyman, this theory identifies flagellates as the most likely ancestral group for metazoans.
- Core Concept:
- The Colonial Theory suggests that the first metazoans arose from hollow, colonial flagellates. This ancestral organism exhibited traits that could facilitate the transition to multicellularity through increased specialization and cooperation among cells.
- Supporting Evidence:
- Flagellated Sperm: The presence of flagellated spermatozoa throughout the metazoan lineage indicates a possible connection to flagellates.
- Monoflagellated Cells: These cells, characterized by a single flagellum, are frequently observed in lower metazoans, such as sponges and coelenterates, suggesting an evolutionary link.
- Sperms and Eggs in Phytoflagellates: The occurrence of true sperms and eggs in phytoflagellates aligns with the reproductive strategies seen in metazoans.
- Colonial Organization: The ability of phytoflagellates to form colonies mirrors the hypothetical path to multicellularity. In particular, Volvox demonstrates differentiation between somatic and reproductive cells, although it is not the direct ancestor of metazoans.
- Choanoflagellates as Ancestors:
- Modern understanding points towards choanoflagellates as the probable ancestral group, given their similarity in mitochondrial and flagellar structures to metazoan cells. Furthermore, choanocytes, which are cells with a collar of microvilli, are present in several metazoan groups, especially sponges.
- Characteristics of the Ancestral Metazoan:
- According to the theory, the primitive metazoan would have been a round or oval hollow colonial flagellate with specific traits:
- Outer Cells: These cells would be mono-flagellated, facilitating locomotion.
- Antero-Posterior Axis: A defined anterior-posterior axis would allow for directional swimming.
- Cell Differentiation: There would be a distinction between somatic (body) cells and reproductive cells, indicating early specialization.
- According to the theory, the primitive metazoan would have been a round or oval hollow colonial flagellate with specific traits:
- Haeckel’s Blastaea:
- Haeckel introduced the term “blastaea” for this hypothetical organism, which is believed to represent the developmental stage of the blastula in modern metazoans. The progression from a colonial arrangement to a more complex multicellular structure indicates a shift toward higher organization.
- Evolution of the Gastraea:
- Haeckel suggested that the blastaea underwent invagination, creating a double-walled structure known as the gastraea, which resembles the embryonic gastrula stage. This form shows similarities to certain hydrozoan coelenterates and sponges.
- Metschnikoff’s Opposition:
- Metschnikoff critiqued Haeckel’s ideas, arguing that lower metazoans engage in intracellular digestion and therefore would not require a digestive cavity. He proposed that some cells could migrate into the interior of the blastaea, leading to a solid structure rather than a hollow one. This ingression could be considered a secondary process, contrasting with invagination.
- Planuloid Ancestor:
- Following Metschnikoff’s view, modern interpretations suggest that the evolution of metazoans began with Haeckel’s blastaea, which then transformed through cellular ingression into a solid organism. This hypothetical organism, referred to as the planuloid ancestor, would possess:
- Ovoid and Radially Symmetrical Body: This shape would facilitate movement and nutrient absorption.
- Locomotor Functions: The exterior mono-flagellated cells would enable mobility and sensory perception.
- Nutrition and Reproduction: A solid mass of internal cells would manage these vital functions without a defined mouth, allowing food to be engulfed from the exterior.
- Following Metschnikoff’s view, modern interpretations suggest that the evolution of metazoans began with Haeckel’s blastaea, which then transformed through cellular ingression into a solid organism. This hypothetical organism, referred to as the planuloid ancestor, would possess:
- Evolution of Symmetry:
- The planuloid ancestor likely gave rise to the primary radial symmetry observed in coelenterates. Subsequently, bilateral symmetry may have evolved in flatworms, leading to the diversity of metazoan phyla.
- Hyman’s Contribution:
- Hyman posited that certain ancestral planuloid organisms transitioned to a benthic lifestyle, prompting the development of distinct dorsal and ventral surfaces and the evolution of a ventral mouth. This adaptation could have led to the emergence of acoelid flatworms, considered precursors to bilateral phyla.
Polyphyletic Theory
The evolution of Metazoa represents a complex journey from simple unicellular organisms to the diverse array of multicellular life forms present today. This evolution can be understood through the examination of various phyla, including sponges, coelenterates, flatworms, and more advanced forms of life.
- Origin of Metazoa:
- The earliest metazoans, specifically sponges belonging to the phylum Porifera, are closely related to the protists. These organisms can be conceptualized as colonies of protists rather than truly multicellular entities.
- Importantly, it is widely accepted that no other group has evolved from sponges, indicating their unique position in the evolutionary history of multicellular organisms.
- Coelenterates:
- Coelenterates, which include the phyla Cnidaria and Ctenophora, are characterized as the most primitive of the Metazoa. They are diploblastic and exhibit radial symmetry.
- These organisms are believed to have evolved from a hypothetical ancestral planuloid metazoan, representing a significant step in the evolution of multicellular life.
- Flatworms (Platyhelminthes):
- Following the coelenterates, flatworms emerged from a similar planuloid ancestor. Unlike coelenterates, flatworms are triploblastic and exhibit bilateral symmetry.
- Notably, flatworms do not possess a true coelomic cavity; instead, their mesoderm is cellular, categorizing them as acoelomates. This group, along with all higher metazoans, is classified under Grade Bilateria.
- Pseudocoelomates:
- The evolution of pseudocoelomates, which includes nematodes and rotifers, is thought to have branched off from flatworms. These organisms possess a pseudocoel, a fluid-filled body cavity that plays a role in their physiology but differs from the true coelom found in more advanced metazoans.
- Eucoelomata:
- The remaining metazoan groups fall under the category of Eucoelomata, which possess a true coelom. This group includes two significant lineages:
- Molluscan-Annelidan-Arthropodan Stock: This lineage features a schizocoelous coelom, wherein the coelomic cavity forms through the splitting of mesodermal tissue. In these organisms, such as polychaete worms and earthworms, the coelom often functions as a hydrostatic skeleton.
- Echinoderm-Hemichordate-Chordate Stock: This lineage is characterized by an enterocoelic coelom, which develops from pouches of the embryonic gut. The coelom in these organisms also serves various structural and functional roles.
- The remaining metazoan groups fall under the category of Eucoelomata, which possess a true coelom. This group includes two significant lineages:
- Evolutionary Adaptations:
- Throughout evolution, the coelom has undergone significant modifications. In more primitive coelomates like polychaete and earthworms, it serves as a skeletal structure, providing support and facilitating movement.
- In contrast, the coelom in more advanced organisms, such as arthropods and mollusks, has become reduced, often represented solely by the gonadal cavity. The remaining body space, termed a hemocoel, is primarily filled with blood and serves to support the organs and tissues within the body.
- Summary of Phylogeny:
- The phylogenetic relationships among metazoans can be summarized through a series of evolutionary branches leading from simple forms like sponges and coelenterates to the more complex structures seen in higher metazoans.
- Each evolutionary step reflects adaptations that enhance survival and reproduction in various environments, showcasing the remarkable diversity and complexity of life.
Evolution of Metazoa
The evolution of Metazoa marks a significant transition in the history of life on Earth, illustrating the development of complex multicellular organisms from simpler unicellular ancestors. This evolutionary trajectory can be traced through various phyla, each contributing uniquely to the overall understanding of metazoan diversity and organization.
- Origin of Metazoans:
- Sponges, classified under the phylum Porifera, represent some of the earliest metazoans and exhibit characteristics that closely relate them to protists. They can be considered as aggregations of protists rather than fully multicellular organisms.
- It is essential to note that no other major groups have evolved directly from sponges, positioning them at a fundamental point in metazoan evolution.
- Coelenterates:
- Following the sponges, coelenterates, which comprise the Cnidaria and Ctenophora phyla, emerged as the most primitive metazoans. They are characterized by their diploblastic organization and radial symmetry.
- These organisms are believed to have evolved from an ancestral planuloid metazoan, indicating a significant evolutionary advancement that allowed for increased complexity.
- Flatworms (Platyhelminthes):
- Flatworms evolved from a similar planuloid ancestor and represent a key evolutionary branch. They are triploblastic and exhibit bilateral symmetry, a notable distinction from the radially symmetrical coelenterates.
- Flatworms lack a true coelomic cavity, being categorized as acoelomates with a cellular mesoderm, which serves as the foundational structure for more advanced metazoan forms. This group is classified under Grade Bilateria, encompassing all higher metazoans.
- Pseudocoelomates:
- The evolutionary lineage that led to pseudocoelomates, including nematodes and rotifers, likely branched off from flatworms. Pseudocoelomates possess a pseudocoel, which is a fluid-filled cavity that plays a functional role but is not a true coelom.
- Eucoelomata:
- The remaining metazoans are categorized as Eucoelomata, characterized by the presence of a true coelom. This group can be further divided into two primary lineages:
- Molluscan-Annelidan-Arthropodan Stock: This lineage exhibits a schizocoelous coelom, forming through the splitting of mesodermal tissue. In these organisms, the coelom often functions as a hydrostatic skeleton, providing structural support and facilitating movement.
- Echinoderm-Hemichordate-Chordate Stock: This lineage features an enterocoelic coelom, which develops from pouches of the embryonic gut. In these organisms, the coelom serves multiple functions, including support and protection of internal organs.
- The remaining metazoans are categorized as Eucoelomata, characterized by the presence of a true coelom. This group can be further divided into two primary lineages:
- Evolutionary Adaptations:
- The coelom has undergone significant changes throughout evolution. In more primitive forms like polychaete and earthworms, the coelom serves as a skeletal structure that aids in locomotion and organ support.
- In contrast, in more derived organisms such as arthropods and mollusks, the coelom is often reduced, represented primarily by the cavity of the gonads. The remaining body space, known as a hemocoel, is filled with blood and serves to support the internal organs.
- Phylogenetic Summary:
- The phylogenetic relationships among metazoans can be illustrated as a branching diagram, beginning with simple forms such as sponges and coelenterates and leading to the more complex structures found in higher metazoans.
- Each evolutionary stage reflects adaptations that have enhanced survival, reproduction, and ecological niches occupied by various organisms, illustrating the vast diversity and complexity of life.
Metamerism/segmentation
Metamerism, or segmentation, is a biological phenomenon characterized by the serial repetition of similar body sections along the longitudinal axis of an organism. Each segment, also known as a metamere or somite, comprises components of major organ systems, thereby contributing to the overall structural and functional organization of the animal. This systematic arrangement of segments allows for enhanced flexibility, complexity, and specialization within the body plan.
- Definition and Structure:
- Metamerism involves the repetition of organs and structures, primarily of mesodermal origin.
- Each metamere contains elements of musculature, nerves, blood vessels, and excretory organs, creating a modular body plan.
- Segmentation predominantly affects the trunk region, while the head (prostomium) and the terminal region (pygidium) are typically not segmented.
- Occurrence in the Animal Kingdom:
- Metamerism is evident in three primary phyla: Annelida, Arthropoda, and Chordata.
- In annelids and arthropods, segmentation is prominently visible in adult forms. For instance, earthworms exhibit clear external segmentation.
- Among chordates, metamerism can be observed in muscles, vertebrae, and ribs, although it may not be as visually distinct. In this group, the embryological development showcases segmentation through the formation of somites.
- Types of Metamerism:
- Homonomous and Heteronomous Metamerism:
- Homonomous metamerism refers to segments that are morphologically identical, although no truly homonomous organism exists, as certain segments, like the head and anal segment, exhibit distinct features.
- In contrast, heteronomous metamerism involves alterations among segments, such as the fusion or loss of segments, emphasizing certain organ systems while minimizing others. This condition is common in more advanced organisms, where the head typically consists of fused segments with a concentration of nervous structures.
- External and Internal Metamerism:
- External metamerism is easily observable in annelids, where the body is divided into multiple segments, each possessing similar internal organ systems.
- Internal metamerism occurs in various systems, including muscles and nerves, in vertebrates, indicating a level of organization beneath the external appearance.
- Complete and Incomplete Metamerism:
- In annelids, complete metamerism is seen, where nearly all organ systems are replicated across segments, representing a primitive condition.
- Incomplete metamerism, observed in arthropods and vertebrates, reflects a division of labor among segments, leading to specialization and differentiation of body regions.
- True Metamerism and Pseudo Metamerism:
- True metamerism is a mesodermal phenomenon prevalent in annelids, arthropods, and chordates, where the number of segments remains constant within a species, ceasing to increase upon maturity.
- Conversely, pseudo metamerism results from ectodermal segmentation, as seen in certain flatworms, where the number of segments can vary within individuals of the same species and can continue to develop throughout the organism’s life.
- Homonomous and Heteronomous Metamerism:
Origin and Evolution of Symmetry
The origin and evolution of symmetry in metazoans represent a pivotal chapter in biological development, illuminating how various body plans and organizational strategies have emerged over time. The emergence of bilateral symmetry from presumed radial ancestors is particularly significant, as it reflects fundamental shifts in organismal structure and function.
- Understanding Symmetry in Metazoans:
- The evolutionary journey of metazoans began with radially symmetrical forms, such as cnidarians and ctenophores, which exhibit a body plan organized around a central axis.
- Bilateral symmetry, characterized by a distinct left and right side, arose later in the evolutionary timeline and is often associated with increased complexity in body structure and functionality. This transition from radial to bilateral symmetry underscores a fundamental adaptation to the environment, enhancing locomotion and sensory processing.
- Key Theories Explaining Symmetry Evolution:
- Syncytial Ciliate Theory:
- Proposed by Jovan Hadzi in 1953 and later supported by Earl D. Hanson in 1958, this theory suggests that metazoans evolved from multinucleate ciliates without distinct cell membranes.
- The process of cellularization led to the development of multicellular organisms, with an ancestral body plan resembling acoelomate flatworms, which were likely bilaterally symmetrical.
- Critics of this theory argue that it overlooks the embryonic development of flatworms, fails to account for the presence of flagellated sperm in metazoans, and incorrectly implies that cnidarians’ radial symmetry evolved from a primary bilateral form.
- Syncytial Ciliate Theory:
- Colonial Flagellate Theory:
- Initially proposed by Ernst Haeckel in 1874, this theory posits that metazoans originated from colonial aggregations of protist-like cells.
- Haeckel introduced the concept of a “gastraea,” an ancestral form that evolved from hollow, spherical colonies of flagellates, leading to radial symmetry seen in cnidarians.
- This idea was further revised by scientists such as Otto Butschli and Élie Metchnikoff, who modified the understanding of gastrulation and the development of endoderm.
- One criticism of this theory is that cnidarians can form endoderm through mechanisms other than invagination, challenging the foundational concepts of Haeckel’s initial proposition.
- Modified Theories of Gastrulation:
- Metchnikoff’s Planuloid Theory: Metchnikoff’s revised theory asserted that ancestral forms underwent ingression rather than invagination, leading to a solid gastrula structure. This solid mass, referred to as the “planuloid,” was radially symmetrical, similar to the larval stages of coelenterates.
- Butschli’s Plakula Theory: Butschli proposed that metazoans evolved from a flat, disc-shaped structure with two layers of flagellated cells. This model emphasized absorption and digestion, paving the way for more complex structures.
- Hyman’s Colonial Theory: Hyman supported Haeckel’s idea but added that through multipolar ingression, the hollow blastula became solid and radially symmetrical, forming the planuloid ancestor.
- Polyphyletic Theory of Metazoan Origin:
- This theory emphasizes the independent evolution of different groups within the kingdom Animalia. It posits that flatworms arose from the cellularization of syncytial, multinucleate ciliates, while sponges evolved separately from choanoflagellates.
- According to this perspective, there are multiple origins of bilateral symmetry, challenging the notion of a single ancestral form for all metazoans.
- The Evolution of Bilateral Symmetry:
- The debate surrounding the origins of bilateral symmetry includes various perspectives on how early metazoans adapted to their environments.
- Proponents of the syncytial and plakula theories argue that bilaterality would have characterized the earliest metazoans, while supporters of the colonial theory suggest that the first bilateral organisms likely evolved from gastrula-like ancestors, which may have undergone a transition from radial to bilateral symmetry.
- The idea that the blastea, through a benthic lifestyle, invaginated and developed bilateral features encapsulates a widely discussed scenario in the evolutionary narrative of symmetry.
- Manuel M. Early evolution of symmetry and polarity in metazoan body plans. C R Biol. 2009 Feb-Mar;332(2-3):184-209. doi: 10.1016/j.crvi.2008.07.009. Epub 2008 Nov 28. PMID: 19281951.
- https://www.ekac.in/upload/online/zoology/Body%20Symmetry,Segmentation%20of%20Metazoa%20(R.Prasad).pdf
- https://www.egyankosh.ac.in/bitstream/123456789/16480/1/Unit-3.pdf
- https://www.bennington.edu/sites/default/files/sources/docs/origin%20of%20segmentation.pdf
- https://nowgonggirlscollege.co.in/attendence/classnotes/files/1621493716.pdf
- https://teachmint.storage.googleapis.com/public/346365980/StudyMaterial/529f86a1-75fc-4cbe-8c2d-6ceb9481bd0d.pdf