What is Linkage?
- Genetic linkage refers to the tendency for genes located on the same chromosome to be inherited together. When genes are situated on the same chromosome and are relatively close to each other, they are likely to be passed on as a unit during inheritance, rather than assorting independently.
- In cases where genes are located on different chromosomes, they follow Mendel’s law of independent assortment, meaning their inheritance is random and not influenced by other genes. However, when genes are on the same chromosome and close to each other, they tend to remain together through generations. This phenomenon is known as linkage.
- Linkage was first observed in a classic experiment by Bateson and Punnett in 1906 with sweet peas. They found that genes for flower color and pollen shape did not assort independently but instead showed a preference for remaining together in certain combinations. This deviation from Mendelian ratios was explained by the proximity of these genes on the same chromosome.
- The concept of linkage also includes the phenomena of coupling and repulsion. Coupling occurs when linked genes tend to be inherited together in the same combination as they appeared in the parents. Repulsion, on the other hand, occurs when new combinations of linked genes appear more frequently than the parental combinations, due to the separation of gene pairs during recombination.
- Crossing over, or the exchange of chromosomal segments between homologous chromosomes during meiosis, plays a critical role in genetic linkage. This process can separate linked genes and result in recombination, leading to new combinations of alleles. The closer two genes are on a chromosome, the less likely they are to be separated by crossing over.
- Thus, linkage represents the non-independent assortment of genes that are physically connected on the same chromosome. This phenomenon affects the inheritance patterns of genes and contrasts with Mendelian principles of independent assortment.
Definition of Genetic Linkage
Genetic linkage is the tendency for genes located on the same chromosome to be inherited together due to their physical proximity.
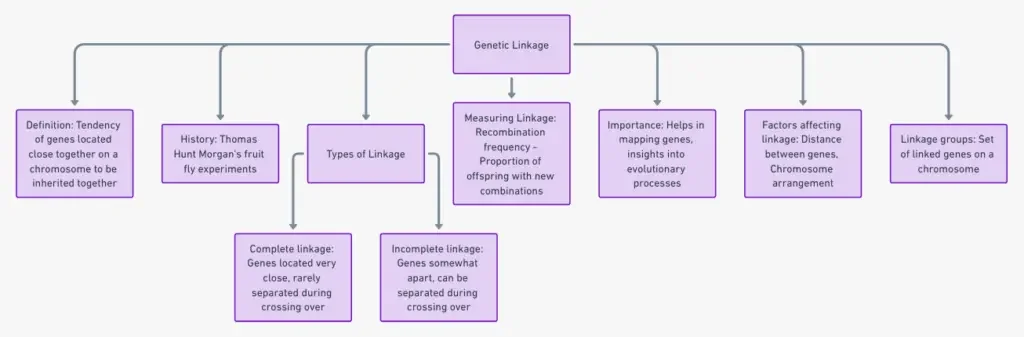
Exploring Terms Related to Genetic Linkage
- Gene Linkage:
- Gene linkage refers to the occurrence of different genes located on the same chromosome. These genes do not assort independently during meiosis but tend to be inherited together due to their physical proximity on the chromosome.
- Linkage Group:
- A linkage group consists of all the genes located on a particular chromosome. The genes within a linkage group are physically linked and therefore tend to be inherited as a unit.
- Linkage Map:
- A linkage map is a representation of the positions of genes on a chromosome, constructed based on recombinant frequencies. It provides an abstract map of chromosomal loci and helps in determining the relative distances between genes.
- Recombination:
- Recombination refers to the process by which genetic material is exchanged between homologous chromosomes during meiosis. This process leads to the formation of new allele combinations in offspring. Recombination frequency is used to estimate the distance between genes on a chromosome.
- Crossing Over:
- Crossing over is a specific type of recombination that occurs during prophase I of meiosis. It involves the exchange of chromosomal segments between homologous chromosomes, resulting in new allele combinations. This process can separate linked genes and contribute to genetic variation.
- Genetic Map:
- A genetic map is a broad term that encompasses various types of maps, including linkage maps and physical maps. It represents the positions of genes and genetic markers on chromosomes, often indicating their relative distances based on recombination frequencies.
- Recombinant Frequency:
- Recombinant frequency measures the proportion of offspring that exhibit new combinations of traits resulting from recombination. It is used to construct linkage maps and estimate the distance between genes. A higher recombinant frequency indicates that genes are located further apart on the chromosome.
- Crossover Frequency:
- Crossover frequency refers to the likelihood of crossing over occurring between two genes during meiosis. It provides an estimate of the genetic distance between genes and is used in constructing linkage maps.
- Coupling (or Cis Configuration):
- Coupling, or cis configuration, occurs when two linked genes for different traits are inherited together on the same chromosome. This results in offspring that predominantly exhibit the parental combination of traits.
- Repulsion (or Trans Configuration):
- Repulsion, or trans configuration, occurs when two linked genes for different traits are inherited separately on homologous chromosomes. This results in offspring that exhibit a mix of parental and recombinant combinations of traits.
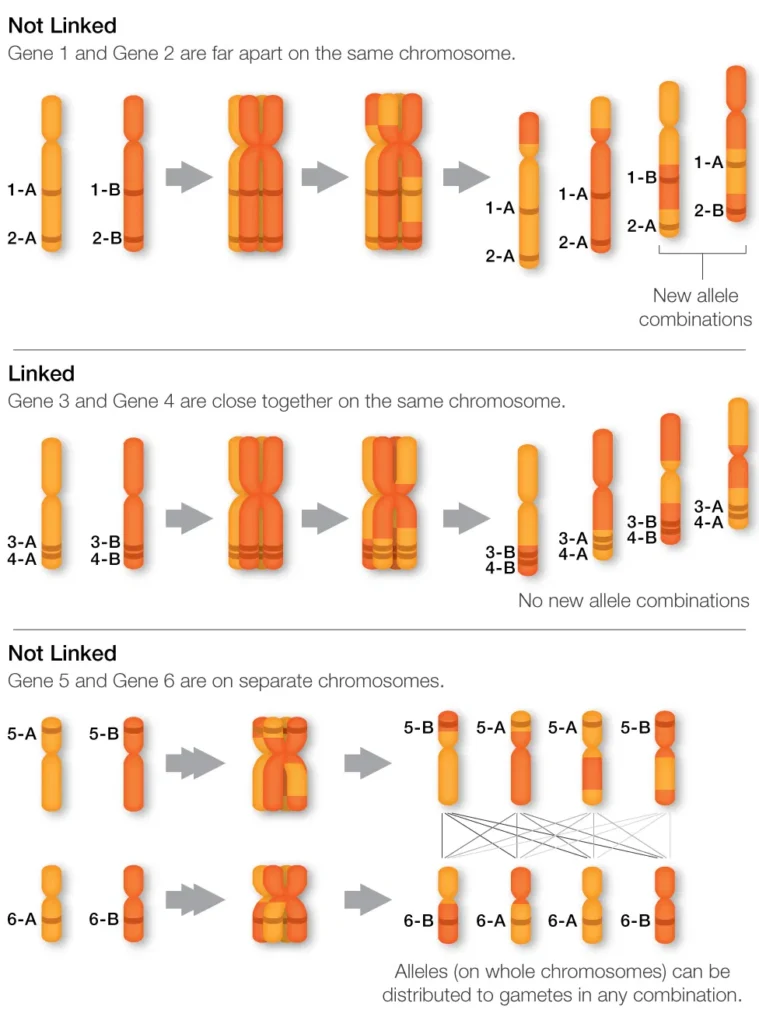
Characteristic of Linked Genes
Linked genes are genes that are located close together on the same chromosome and tend to be inherited together. Here are some key characteristics of linked genes:
- Physical Proximity: Linked genes are located close to each other on the same chromosome. Because of this proximity, they tend to not segregate independently during meiosis.
- Reduced Recombination: Linked genes have a lower chance of being separated by recombination during meiosis compared to genes that are farther apart. This is because the likelihood of a crossover event occurring between them is reduced.
- Inheritance Patterns: Linked genes often show non-Mendelian inheritance patterns. Instead of the expected 9:3:3:1 ratio seen in dihybrid crosses with independently assorting genes, linked genes might show ratios like 9:3:4 or other variations.
- Recombination Frequency: The probability that a crossover will occur between two linked genes can be used to estimate the distance between them on a chromosome. This is measured in centimorgans (cM). A recombination frequency of 1% is equivalent to a distance of 1 cM.
- Genetic Maps: Using the recombination frequencies between different pairs of genes, geneticists can create genetic maps that show the relative positions of genes on a chromosome.
- Coupling and Repulsion: When studying linked genes, two types of heterozygous gene arrangements can be observed:
- Coupling (cis configuration): Where the dominant alleles of two genes are on one chromosome and the recessive alleles are on the other chromosome.
- Repulsion (trans configuration): Where one dominant allele and one recessive allele of the two genes are found on the same chromosome.
- Incomplete Linkage: No two genes are completely linked, meaning that there is always some chance of recombination between them. The degree of linkage between two genes can vary, with some being more tightly linked than others.
- Discovery: The concept of gene linkage was first introduced by Thomas Hunt Morgan and his student Alfred Sturtevant while studying fruit flies. They noticed that certain traits were often inherited together, leading to the discovery of linked genes.
- Importance in Evolution: Linkage can slow down the process of genetic variation since linked genes are inherited together. However, recombination events can introduce new gene combinations, providing variation for natural selection to act upon.
- Disease Association: Sometimes, multiple genes that are linked can be associated with a particular disease or condition. This can be useful in genetic studies to identify regions of the genome associated with specific diseases.
What is Linkage map?
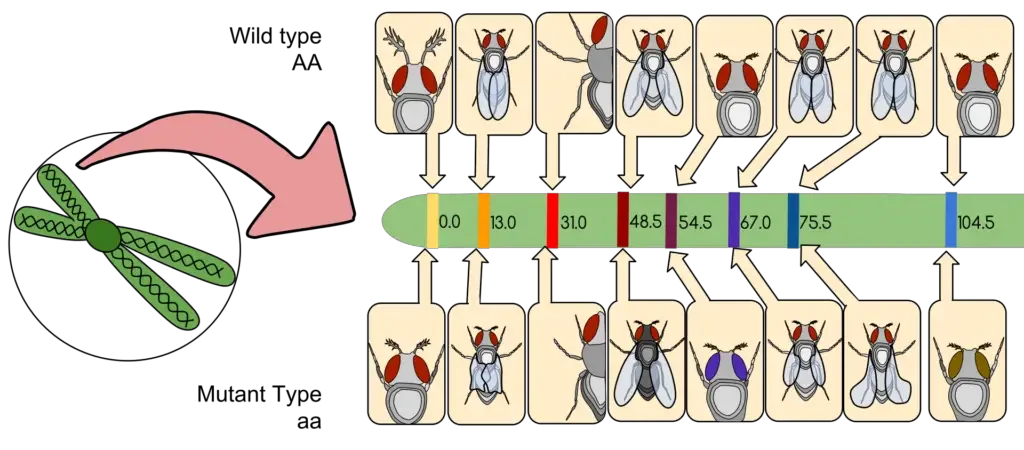
A linkage map, commonly referred to as a genetic map, is a structured representation that delineates the relative positions of genes or genetic markers on a chromosome. This positioning is not based on the precise physical locations on the chromosome but rather on the recombination frequency between these genes or markers. The foundational principle behind linkage maps is that the probability of recombination between two genetic loci is proportional to the distance separating them.
- Historical Context: The concept of the linkage map was pioneered by Alfred Sturtevant, a student under the tutelage of the eminent geneticist Thomas Hunt Morgan. Sturtevant’s groundbreaking work laid the groundwork for subsequent genetic research, emphasizing the importance of understanding gene positions relative to one another.
- Mechanistic Underpinning: The essence of a linkage map is rooted in the phenomenon of crossing over, which occurs during the prophase of meiosis. During this process, homologous non-sister chromatids undergo an exchange of chromosomal segments. The frequency of such recombination events between two markers serves as an indirect measure of their relative distance. A higher recombination frequency indicates that the markers are farther apart, while a lower frequency suggests proximity.
- Markers in Linkage Maps: Historically, the markers employed in linkage maps were discernible phenotypic traits, such as eye color or enzyme production, which were manifestations of coding DNA sequences. With advancements in molecular genetics, researchers have transitioned to using noncoding DNA sequences, like microsatellites or restriction fragment length polymorphisms (RFLPs), as markers.
- Applications and Utility: Linkage maps serve as invaluable tools for geneticists, facilitating the identification and localization of new genes or markers. Initially, data from linkage maps are employed to establish linkage groups, which are clusters of genes known to be linked. As research progresses, these groups can be expanded to encompass an entire chromosome. For well-researched organisms, these linkage groups correspond directly to individual chromosomes.
- Distinguishing from Other Maps: It is imperative to differentiate linkage maps from physical or gene maps. While linkage maps are predicated on recombination frequencies, physical maps provide a detailed representation of the actual physical distances between genes or markers on a chromosome.
Example from Scientific Literature: In a seminal research paper titled “Isozymic gene linkage map of the tomato: Applications in genetics and breeding” by Steven D. Tanksley and Charles M. Rick, the authors elucidated the linkage map of the tomato, emphasizing its implications in genetic studies and breeding practices1. Such research underscores the significance of linkage maps in understanding the genetic architecture of organisms and their subsequent applications in breeding and genetic improvement.
Chromosome Theory of Linkage
The Chromosome Theory of Linkage, developed by Thomas Hunt Morgan and A.H. Castle, is a fundamental concept in genetics. This theory provides a clear framework for understanding how genes are inherited in a linear manner along chromosomes. The following points outline the key aspects of this theory:
- Location of Linked Genes:
- Genes that exhibit linkage are located on the same chromosome. This means they are physically connected within the chromosomal structure.
- These linked genes are generally inherited together because their proximity on the chromosome keeps them together during meiosis.
- Strength of Linkage:
- The strength of linkage between genes is influenced by the physical distance separating them on the chromosome.
- Genes that are closely situated show strong linkage, meaning they are less likely to be separated during the process of genetic recombination.
- Conversely, genes that are farther apart exhibit weaker linkage. This is due to the higher probability of recombination occurring between them.
- Linear Arrangement of Genes:
- Genes are arranged in a linear sequence along the chromosome. This linear arrangement ensures that their relative positions can affect the likelihood of recombination events.
- The order of genes on a chromosome determines how they are inherited together, and any changes in this order can influence genetic outcomes.
Types of Linkage
Morgan and his colleagues identified two primary types of linkage through their investigations with Drosophila melanogaster: complete linkage and incomplete linkage. Each type has distinct characteristics and implications for genetic inheritance.
1. Complete Linkage
Complete linkage occurs when two genes are located so close to one another on the same chromosome that they are inherited together without any chance of separation during gamete formation. This condition prevents recombination between these genes, causing them to be transmitted together across generations. Complete linkage is a specific form of genetic linkage where the parental combination of traits is consistently inherited over multiple generations without the formation of recombinant types.
Characteristics of Complete Linkage
- Linked Genes: In cases of complete linkage, genes are positioned very close to each other on the same chromosome. This proximity results in the genes being passed down together during inheritance.
- Inheritance Pattern: The linked genes maintain their parental combination over two or more generations, consistently producing offspring with the same genetic traits as their parents.
- Absence of Recombination: In complete linkage, recombination between the linked genes does not occur, leading to a lack of recombinant phenotypes.
Example of Complete Linkage in Drosophila melanogaster (Fruit Fly)
- Initial Cross: A female fruit fly with red eyes and normal wings (RRWW) is crossed with a male having purple eyes and vestigial wings (rrww).
- F1 Generation: The offspring of this cross (RrWw) are all heterozygous, displaying red eyes and normal wings. These phenotypes are dominant traits.
- Test Cross: The F1 heterozygous males (RrWw) are test-crossed with homozygous recessive females (rrww). This results in only two types of offspring: one with red eyes and normal wings (RrWw) and another with purple eyes and vestigial wings (rrww), in a 1:1 ratio.
- Key Observation: There are no recombinant phenotypes, meaning no offspring exhibit red eyes with vestigial wings or purple eyes with normal wings. The frequency of recombination in this case is zero.
Mechanism Behind Complete Linkage
- Gamete Formation: In organisms where complete linkage occurs, the F1 males produce only two types of gametes: one carrying both the dominant alleles for red eyes and normal wings (RW) and the other carrying both recessive alleles for purple eyes and vestigial wings (rw). Normally, four types of gametes would be expected, but due to complete linkage, only the parental combinations are formed.
- Special Cases in Crossing Over: Although crossing over usually occurs during gametogenesis in most organisms, there are exceptions. For example, male Drosophila and female silkworms rarely, if ever, undergo crossing over during gamete formation. This lack of recombination contributes to the complete linkage phenomenon in these species.
Limitations of Complete Linkage
- Rare Occurrence: Complete linkage is rare in most organisms. Even though genes may be closely linked, some degree of recombination usually occurs due to crossing over. Total linkage, where no recombination happens at all, is an uncommon phenomenon.
- Deviation from Expected Ratios: In theoretical models, complete linkage predicts specific inheritance ratios, such as a 3:1 ratio when F1 males are crossed with F1 females. However, in practice, this ratio is not typically observed, reflecting the rarity of absolute linkage without any recombination.
2. Incomplete Linkage
Incomplete linkage refers to the phenomenon where genes located on the same chromosome, though linked, are not always inherited together due to crossing over. This results in a mixture of parental and recombinant phenotypes in the offspring, reflecting the partial separation of linked genes during gametogenesis.
Characteristics of Incomplete Linkage
- Gene Separation: Incomplete linkage occurs when genes, despite being located on the same chromosome, can be separated due to crossing over during meiosis. This crossover event occurs between homologous non-sister chromatids during the pachytene stage of Prophase I.
- Phenotypic Ratios: The presence of crossing over leads to the production of recombinant phenotypes, alongside parental phenotypes, in the progeny. This results in a deviation from the expected Mendelian ratios.
Example in Sweet Peas
- Initial Cross: Consider a cross between a homozygous dominant sweet pea plant with blue flowers and long pollen (BBLL) and a homozygous recessive plant with red flowers and round pollen (bbll).
- F1 Generation: The offspring from this cross are all hybrids with blue flowers and long pollen (BbLl), displaying the dominant traits.
- Test Cross: When these F1 hybrids (BbLl) are test-crossed with a homozygous recessive plant (bbll), four types of phenotypes appear in the F2 generation:
- Blue flowers and long pollen (BbLl)
- Blue flowers and round pollen (Bbll)
- Red flowers and long pollen (bbLl)
- Red flowers and round pollen (bbll)
- Expected vs. Observed Ratios: The observed phenotypic ratio is 7:1:1:7 rather than the expected 1:1:1:1. This deviation arises because crossing over between the linked genes has occurred, leading to recombinant types.
Detailed Observations
- Parental vs. Recombinant Phenotypes: In the F2 generation, parental phenotypes (blue flowers with long pollen and red flowers with round pollen) account for 87.4% of the progeny, while recombinant phenotypes (blue flowers with round pollen and red flowers with long pollen) constitute 12.6%.
- Linkage and Distance: The results indicate that the genes for flower color and pollen shape are linked but positioned at a greater distance on the chromosome. Due to their distance and crossing over, these genes do not always remain together during inheritance.
Mechanism of Incomplete Linkage
- Crossing Over: During meiosis, the process of crossing over can exchange genetic material between homologous chromatids. When genes are linked but not tightly, this exchange can lead to the formation of recombinant alleles, which then appear in the offspring.
- Distance Impact: The frequency of recombinant phenotypes is influenced by the distance between linked genes. Genes located farther apart on a chromosome are more likely to be separated by crossing over, resulting in a greater proportion of recombinant types.
Classification of Linkage Based on chromosomal involved
Linkage can be classified based on the chromosomal location of the genes involved. This classification helps in understanding how genes are inherited together due to their physical proximity on chromosomes. The main categories are autosomal linkage and allosomal (or sex) linkage.
1. Autosomal Linkage
- Definition: Autosomal linkage refers to the situation where genes are located on autosomes, which are chromosomes other than the sex chromosomes.
- Characteristics:
- Location: Autosomes are chromosomes that do not determine the sex of an organism. In humans, these are chromosomes 1 through 22.
- Inheritance: Genes on the same autosome are inherited together because they are physically linked. The likelihood of their recombination depends on their distance on the chromosome and the frequency of crossing over.
- Examples: Linkage studies involving traits such as flower color and seed shape in plants often involve autosomal linkage. In Drosophila melanogaster, many linkage studies are conducted on autosomes to understand the inheritance of traits such as wing shape and eye color.
2. Allosomal Linkage (Sex Linkage)
- Definition: Allosomal or sex linkage describes the situation where genes are located on sex chromosomes (X or Y chromosomes).
- Characteristics:
- Location:
- X-Linked Genes: These are genes located on the X chromosome. In humans, the X chromosome carries many genes unrelated to sex determination, and X-linked traits often exhibit different inheritance patterns in males and females.
- Y-Linked Genes: These are genes located on the Y chromosome. Y-linked traits are passed from father to son and are typically involved in determining male sex characteristics.
- Inheritance:
- X-Linked Linkage: Males have one X and one Y chromosome, while females have two X chromosomes. Therefore, X-linked traits are expressed differently in males (hemizygous) and females (homozygous or heterozygous).
- Y-Linked Linkage: Traits linked to the Y chromosome are inherited exclusively through the paternal line, affecting only males and transmitted from father to son.
- Examples:
- X-Linked: In humans, hemophilia and color blindness are examples of X-linked recessive disorders. In Drosophila, red-eye color and white-eye color are X-linked traits.
- Y-Linked: Traits such as the SRY gene, which is crucial for male sex determination, are Y-linked.
- Location:
Linkage analysis
Linkage analysis is a sophisticated genetic technique employed to identify chromosomal segments that consistently co-segregate with a specific phenotype across multiple generations within families. This method has been pivotal in mapping genes associated with both binary (dichotomous) and quantitative (continuous) traits.
Types of Linkage Analysis
- Parametric Linkage Analysis: This approach necessitates prior knowledge regarding the relationship between phenotypic and genetic similarity. The cornerstone of parametric linkage analysis is the LOD (logarithm of odds) score. The LOD score is a statistical measure that evaluates the likelihood of observing the test data under the assumption of linkage between two loci, compared to the likelihood of observing the same data by mere coincidence. A positive LOD score suggests the presence of linkage, while a negative score implies that linkage is improbable.
- Non-parametric Linkage Analysis: This method does not rely on prior assumptions about the genetic model. Instead, it evaluates the probability of an allele being identical by descent.
LOD Score Computation
The LOD score, a brainchild of Newton Morton, is computed using the formula:
LOD = Z =
Where:
θ is the recombination fraction, signifying the proportion of offspring in which recombination occurs between the genetic marker under study and the hypothetical gene associated with the disease.
Applications
Linkage analysis facilitates the identification of genetic markers in close proximity to genes that influence specific traits or diseases. For instance, through linkage analysis, researchers can pinpoint genes associated with rare genetic disorders.
Limitations
Despite its utility, linkage analysis is not devoid of limitations. It possesses both methodological and theoretical constraints that can elevate the type-1 error rate and diminish the power to map quantitative trait loci (QTL) in humans. While linkage analysis has been instrumental in identifying genetic variants linked to rare disorders, its efficacy diminishes when applied to prevalent disorders like cardiovascular diseases or various cancers. This discrepancy can be attributed to the distinct genetic underpinnings of common versus rare disorders.
Example
Consider a pedigree where a specific genetic disorder is prevalent. Through parametric linkage analysis, researchers can assess the probability that a particular genetic marker and the disease gene are linked based on the observed patterns of inheritance within the family. If the LOD score exceeds the threshold of 3.0, it provides compelling evidence for linkage, suggesting that the marker and the disease gene are located in close proximity on the chromosome.
In summary, linkage analysis is a robust genetic tool that has significantly advanced our understanding of the genetic architecture of various traits and diseases. Its precision and methodological rigor make it an indispensable technique in the realm of genetic research.
Calvin Bridges’ Experiments on Nondisjunction: Evidence for the Chromosome Theory of Heredity
Calvin Bridges, working under Thomas Hunt Morgan at Columbia University, conducted groundbreaking experiments from 1913 to 1916 that provided key evidence for the chromosome theory of heredity. Through his meticulous work with Drosophila melanogaster (the fruit fly), Bridges demonstrated how a process called nondisjunction led to unusual inheritance patterns, thus supporting the idea that genes are located on chromosomes.
Key Concepts of Bridges’ Experiments:
- Nondisjunction:
- This is a failure of chromosomes to separate properly during meiosis, leading to abnormal numbers of chromosomes in sperm or egg cells.
- Nondisjunction in Bridges’ experiments resulted in offspring displaying unexpected traits due to abnormal chromosomal compositions, providing evidence that chromosomes carry hereditary information.
- Observations in Drosophila:
- Drosophila was an ideal model organism due to its easily observable traits, like eye color, and simple chromosomal structures.
- Bridges studied eye color inheritance, focusing on sex-linked traits controlled by genes located on the X chromosome. In normal inheritance, males inherit their single X chromosome from their mothers, while females inherit one X chromosome from each parent.
- Exceptional Flies:
- Bridges noticed exceptions to the normal inheritance patterns. Specifically, he observed some male fruit flies inheriting traits from their fathers (anomalous for sex-linked inheritance) and some female flies inheriting traits solely from their mothers.
- These unusual offspring were termed “exceptional males” and “exceptional females,” signaling an error in meiosis.
- Mechanism of Nondisjunction:
- Bridges hypothesized that in exceptional cases, the X chromosomes in female flies failed to separate during meiosis. This produced eggs with either two X chromosomes or none at all.
- When fertilized by normal sperm (with either one X or one Y chromosome), this could result in:
- XXY females (which he termed exceptional females)
- XO males (which he termed exceptional males)
- Chromosomal Evidence:
- Using microscopes, Bridges could visually confirm his predictions by identifying the presence of abnormal chromosomal combinations in exceptional flies.
- His observations confirmed that females had two X chromosomes and a Y chromosome (XXY), while exceptional males had only one X chromosome (XO).
Importance of Bridges’ Work:
- Support for the Chromosome Theory of Heredity:
- Bridges’ findings provided strong evidence that genes are located on chromosomes. While Morgan and others had suggested this theory, Bridges’ work on nondisjunction gave direct, irrefutable proof.
- His research also clarified that sex in Drosophila was determined by the number of X chromosomes, not the presence of a Y chromosome as seen in humans.
- Sex-Linked Traits:
- Bridges expanded on Morgan’s earlier work on sex-linked traits, showing how nondisjunction could result in males or females inheriting traits from unusual parental combinations.
- This provided deeper insight into how genetic traits are passed on, particularly in the context of errors in cell division.
- Long-Term Impact:
- Although Morgan received the Nobel Prize in 1933 for his contributions to genetics, Bridges’ experiments were foundational in proving the role of chromosomes in heredity.
- His research helped shape the field of genetics throughout the 20th century and continues to be a cornerstone in the study of heredity.
Experimental Approach:
- Breeding Experiments:
- Bridges began by mating female white-eyed flies with red-eyed males. White eye color was a sex-linked trait found on the X chromosome.
- Exceptional flies (those with unusual inheritance patterns) appeared in low frequencies, so Bridges developed a method to increase their numbers by selectively breeding exceptional females with normal males.
- Frequency of Nondisjunction:
- Through selective breeding, Bridges found that exceptional offspring occurred more frequently in certain crosses. This allowed him to study nondisjunction more efficiently, providing more data to support his hypothesis.
- Microscopic Evidence:
- To confirm the abnormal chromosomal combinations, Bridges used microscopy to directly observe the chromosomes in exceptional offspring, further supporting his nondisjunction hypothesis.
Coupling and Repulsion Hypothesis of Linkage: By Bateson and Punnett
Bateson and Punnett, in their 1906 work on Lathyrus odoratus (sweet pea), explored the phenomenon of genetic linkage, which deviated from Mendel’s law of independent assortment. Their study focused on how certain traits were inherited together more frequently than expected.
Observations from the First Experiment
- Initial Cross: Bateson and Punnett began by crossing a plant with blue flowers (BB) and long pollen grains (LL) with a plant exhibiting red flowers (bb) and round pollen grains (ll).
- F1 Generation: As predicted by Mendelian inheritance, the F1 generation exhibited all blue flowers with long pollen grains (BbLl), as blue and long pollen are dominant traits.
- Test Cross: The F1 offspring (BbLl) were then test-crossed with a plant showing red flowers and round pollen grains (bbll). Here, instead of obtaining a 1:1:1:1 ratio of phenotypes, a ratio of 7:1:1:7 was observed.
Explanation of the Coupling Hypothesis
- Coupling: The unexpected ratio indicated that dominant alleles for blue flower color and long pollen grains (B and L) tended to stay together in the F1 progeny, while the recessive alleles for red flowers and round pollen (b and l) also remained together. Bateson and Punnett termed this phenomenon coupling. They concluded that pairs of genes from homozygous parents tend to remain linked in the offspring, resulting in a higher frequency of parental traits.
Observations from the Second Experiment
- New Cross: A second experiment was conducted, crossing a plant with blue flowers and round pollen grains (BBll) with a plant having red flowers and long pollen grains (bbLL).
- F1 Generation: All F1 progeny displayed blue flowers and long pollen (BbLl), the dominant traits.
- Test Cross: When these F1 individuals were test-crossed with a plant having red flowers and round pollen (bbll), the resulting phenotypic ratio was 1:7:7:1, rather than the expected 1:1:1:1.
Repulsion Hypothesis
- Repulsion: In this case, the results showed that when dominant alleles for blue flowers (B) and long pollen (L) came from different parents, they were less likely to be inherited together. This phenomenon, where dominant alleles from different parents avoid being linked, was termed repulsion. In this second experiment, the dominant B allele for blue flowers and the recessive l allele for round pollen tended to stay together, leading to an excess of parental phenotypes over recombinant ones.
Key Conclusions
- Linkage in Coupling: In the first experiment, the dominant alleles (B and L) for blue flowers and long pollen were linked, which Bateson and Punnett termed coupling.
- Linkage in Repulsion: In the second experiment, the dominant alleles (B and L) from different parents repelled each other, a condition described as repulsion.
- Impact on Recombination: Bateson and Punnett’s experiments showed that genetic linkage can result in a higher frequency of parental types over recombinants, deviating from Mendelian expectations.
Modern Perspective
- The coupling and repulsion hypothesis has since been replaced by our modern understanding of genetic linkage. Bateson and Punnett’s observations were foundational in recognizing that genes located close to one another on the same chromosome are more likely to be inherited together, leading to what is now known as the phenomenon of genetic linkage. Their work paved the way for later discoveries in genetics, although their initial hypothesis is no longer in use.
Importance of Linkage
The significance of linkage can be elucidated as follows:
- Genetic Mapping: Linkage serves as the foundational principle for constructing genetic maps. The frequency of recombination between genes, which is inversely proportional to the distance between them on a chromosome, provides a metric to determine their relative positions. This has been instrumental in mapping the human genome and those of numerous other organisms.Example: Alfred Sturtevant’s pioneering work on Drosophila melanogaster led to the formulation of the first genetic map, where he used recombination frequencies to deduce the relative positions of genes on the chromosome.
- Genetic Variation and Evolution: While linkage can constrain genetic variation due to the co-inheritance of linked alleles, crossing over introduces new gene combinations. This balance between linkage and recombination ensures a steady influx of genetic variation, which is vital for populations to adapt and evolve in changing environments.Example: In populations exposed to fluctuating environmental conditions, the interplay between linkage and recombination can lead to the emergence of adaptive gene combinations that confer a selective advantage.
- Genetic Disorders and Linkage Disequilibrium: Linkage disequilibrium (LD) refers to the non-random association of alleles at different loci. LD is a crucial concept in the study of genetic disorders, as certain combinations of alleles can predispose individuals to specific diseases.Example: The study of LD patterns has been pivotal in identifying genetic markers associated with complex diseases like Alzheimer’s, where specific allele combinations increase disease susceptibility.
- Breeding and Agriculture: In crop breeding, understanding linkage is paramount. Desirable traits that are linked can be co-selected, but linkage can also pose challenges when beneficial traits are linked to undesirable ones.Example: In wheat breeding, a desired trait for disease resistance might be linked to an undesired trait affecting grain quality. Breeders must employ strategies like marker-assisted selection to break this linkage and achieve the desired genetic combination.
- Molecular Evolution and Genomic Studies: Linkage can influence patterns of molecular evolution. Genes within tightly linked regions might experience reduced effective population sizes, affecting their susceptibility to genetic drift and selective pressures.Example: In genomic scans for selection, regions of reduced genetic variation might indicate selective sweeps, where beneficial mutations and linked neutral mutations increase in frequency together.
Fun Facts About linkage
- Did you know that linkage refers to the tendency of genes located close to each other on the same chromosome to be inherited together more frequently than genes that are far apart?
- Have you heard that the recombination frequency between linked genes can provide insight into their relative distances on a chromosome, with a higher frequency indicating greater distance?
- Are you aware that genes that are located far apart on the same chromosome can appear to assort independently due to frequent crossing over during meiosis?
- Did you know that complete linkage occurs when genes are so closely located on a chromosome that no recombination occurs between them, leading to a 1:1 ratio of parental phenotypes in the progeny?
- Can you believe that the concept of linkage was first demonstrated by early geneticists like Thomas Morgan, who used fruit flies to study the inheritance of traits?
- Have you ever heard that the frequency of recombination between two genes is used to construct genetic maps, helping scientists determine the physical distance between genes on a chromosome?
- Did you know that linkage can be classified into autosomal linkage, where genes are located on autosomes, and sex linkage, where genes are located on sex chromosomes?
- Are you aware that incomplete linkage occurs when genes are linked but still show some degree of recombination, resulting in a mixture of parental and recombinant phenotypes?
- Did you know that the recombination frequency cannot exceed 50%, reflecting the maximum level of independent assortment and providing a limit for gene mapping accuracy?
- Can you believe that in some organisms, such as Drosophila, the frequency of crossing over can vary significantly between sexes, affecting the observed linkage patterns?
- Have you heard that linked genes that are very close together on a chromosome have a lower probability of being separated by crossing over, resulting in a lower recombination frequency?
- Did you know that linkage disequilibrium, a term used to describe the non-random association of alleles at different loci, can provide insights into the genetic structure of populations?
- Are you aware that the study of linkage has important applications in agriculture, such as in the development of crops with desirable traits through marker-assisted selection?
- Did you know that linkage analysis can be used in human genetics to identify genes associated with complex diseases by studying the inheritance patterns of genetic markers?
- Can you believe that understanding linkage and recombination is crucial for interpreting the results of genetic crosses and for making accurate predictions about inheritance patterns?
Genetic Linkage Mindmap
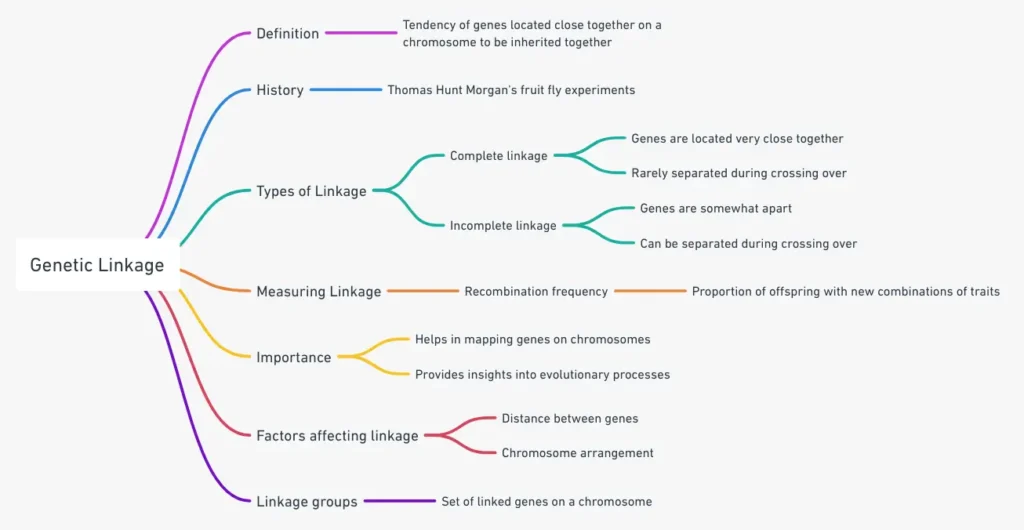
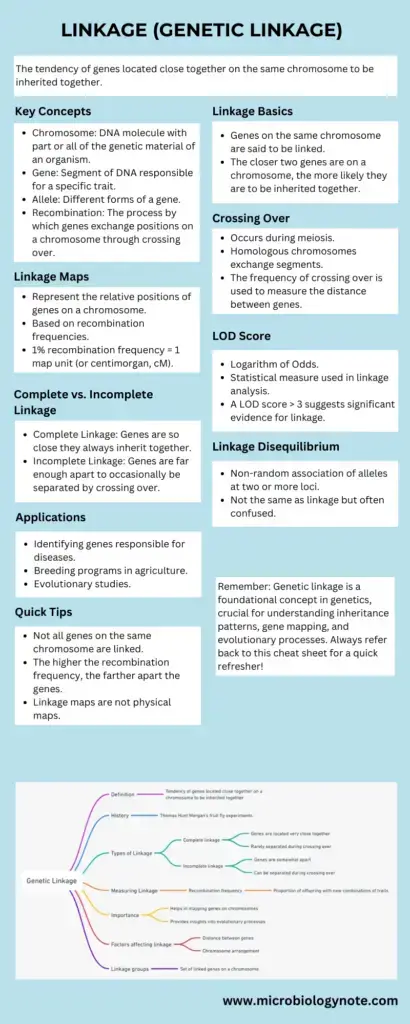
FAQ
What is genetic linkage?
Genetic linkage refers to the tendency of genes located close together on the same chromosome to be inherited together more often than expected by chance.
How was genetic linkage discovered?
Genetic linkage was first observed by Thomas Hunt Morgan and his student Alfred Sturtevant while studying fruit flies (Drosophila melanogaster). They noticed that certain genes did not assort independently, as predicted by Mendel’s laws.
What is a linkage map?
A linkage map, or genetic map, is a representation that shows the relative positions of genes or genetic markers on a chromosome based on recombination frequencies, rather than actual physical distances.
How does crossing over relate to genetic linkage?
Crossing over is the process where homologous chromosomes exchange segments during meiosis. This can separate genes that are linked, leading to recombination. The frequency of crossing over between two genes is inversely proportional to their linkage strength.
What is a LOD score in linkage analysis?
The LOD (logarithm of odds) score is a statistical measure used in linkage analysis to determine the likelihood of two genes being linked. A LOD score greater than 3 typically indicates significant evidence for linkage.
Why are some genes more tightly linked than others?
The strength of linkage between two genes is determined by their distance apart on a chromosome. Genes that are closer together are more tightly linked and have a lower recombination frequency.
What is the difference between complete linkage and incomplete linkage?
Complete linkage occurs when genes are so closely located on a chromosome that they are always inherited together. Incomplete linkage, on the other hand, refers to genes that are linked but can still be separated by crossing over due to their relative distance on the chromosome.
How do linkage maps aid in genetic research?
Linkage maps allow researchers to determine the relative positions of genes on a chromosome. This can help in identifying genes responsible for certain traits or diseases and is crucial for breeding programs and evolutionary studies.
What is linkage disequilibrium?
Linkage disequilibrium (LD) refers to the non-random association of alleles at different loci. It indicates that the alleles at two linked loci occur together more or less frequently than would be expected by chance.
Can genes on different chromosomes be linked?
No, linkage specifically refers to genes on the same chromosome. However, genes on different chromosomes can show non-random associations due to other factors, but this is not considered genetic linkage.
- Bridges CB. Non-Disjunction as Proof of the Chromosome Theory of Heredity. Genetics. 1916 Jan;1(1):1-52. doi: 10.1093/genetics/1.1.1. PMID: 17245850; PMCID: PMC1193653.
- Ganetzky B, Hawley RS. The Centenary of GENETICS: Bridges to the Future. Genetics. 2016 Jan;202(1):15-23. doi: 10.1534/genetics.115.180182. PMID: 26733664; PMCID: PMC4701082.
- https://embryo.asu.edu/pages/calvin-bridges-experiments-nondisjunction-evidence-chromosome-theory-heredity-1913-1916
- Calvin B Bridges, NON-DISJUNCTION AS PROOF OF THE CHROMOSOME THEORY OF HEREDITY, Genetics, Volume 1, Issue 1, 1 January 1916, Pages 1–52, https://doi.org/10.1093/genetics/1.1.1
- Tanksley, S.D., & Rick, C.M. (1980). Isozymic gene linkage map of the tomato: Applications in genetics and breeding.
- https://www.genome.gov/genetics-glossary/Linkage
- https://learn.genetics.utah.edu/content/pigeons/geneticlinkage/https://www.khanacademy.org/science/ap-biology/heredity/non-mendelian-genetics/a/linkage-mapping
- http://www.nature.com/scitable/topicpage/discovery-and-types-of-genetic-linkage-500
- https://bio.libretexts.org/Bookshelves/Introductory_and_General_Biology/Introductory_Biology_(CK-12)/03%3A_Genetics/3.10%3A_Genetic_Linkage
- https://www.nature.com/articles/nrg3908
- https://www.sciencedirect.com/topics/agricultural-and-biological-sciences/genetic-linkage
- https://pubmed.ncbi.nlm.nih.gov/10369304/
- https://kvmwai.edu.in/upload/StudyMaterial/Linkage_(1).pdf
- https://opengenetics.pressbooks.tru.ca/chapter/recombination/