What is Gibberellin?
- Gibberellins (GAs) represent a class of diterpenoid plant and fungal hormones, pivotal in orchestrating various developmental processes in plants, such as stem elongation, germination, dormancy, flowering, and leaf and fruit senescence. Historically, the discovery of gibberellins can be traced back to the 1930s in Japan when scientists were investigating a peculiar rice disease known as bakanae or “foolish seedling.” This disease manifested as an exaggerated elongation of rice stems and leaves, leading to their eventual collapse.
- The causative agent behind this disease was identified as the fungus Gibberella fujikuroi by Japanese scientist Eiichi Kurosawa in 1926. Subsequent research at the University of Tokyo revealed that this fungus produced a substance responsible for the symptoms of the disease. This substance was aptly named “gibberellin.” By the end of the 1930s, gibberellin had been successfully extracted from the fungus, and its application on healthy rice crops replicated the effects of the disease.
- The post-World War II era saw a surge in interest in gibberellins, especially in the West. Independent research initiatives in the United Kingdom and the United States led to the isolation of gibberellic acid, a specific derivative of gibberellin. This compound, also referred to as gibberellin A3 or GA3, soon garnered attention for its potential applications in various commercially significant plants.
- Gibberellins are synthesized within the plastids of plant cells, organelles integral to food production. Following synthesis, they are transported to the cell’s endoplasmic reticulum, where they undergo modifications and processing. To date, over 100 gibberellins have been identified, labeled as GA1, GA2, GA3, and so forth. Among these, GA3 or Gibberellic acid stands out as the most extensively researched plant growth regulator.
- Functionally, gibberellins play diverse roles in plant growth and development. They can break seed dormancy, promote cell elongation and division, and influence enzyme induction, flowering, and fruiting processes. Notably, when applied in minimal concentrations, gibberellins can induce dramatic stem elongation, transforming bushy plants into climbers. This ability to override genetic constraints in dwarf plant varieties underscores the profound impact of gibberellins on plant morphology.
- The significance of gibberellins extends beyond academic interest. The 1960s, marked by a rapid global population increase, witnessed concerns of an impending food crisis. However, the development of a high-yielding rice variety, IR8, mitigated these fears. This semi-dwarf rice variety, characterized by its short stature due to a mutation in the sd1 gene, exemplifies the influence of gibberellins on plant growth. The sd1 gene encodes for GA20ox, and its mutation results in a phenotype consistent with gibberellin deficiency.
- In conclusion, gibberellins, with their multifaceted roles in plant growth and development, have not only deepened our understanding of plant physiology but have also offered solutions to real-world agricultural challenges. Their discovery and subsequent research have bridged the gap between scientific inquiry and practical application, underscoring the importance of continued exploration in plant biology.
Definition of Gibberellin
Gibberellin is a class of diterpenoid plant hormones that regulate various developmental processes, including stem elongation, germination, flowering, and dormancy.
Structure of Gibberellin
- Gibberellins are a class of tetracyclic diterpenes characterized by their ent-gibberellane ring structure. Structurally, they can be categorized based on the number of carbon atoms they contain: either 20-carbon (C20) or 19-carbon (C19) gibberellins. The C20 gibberellins, exemplified by GA12, serve as precursors to the C19 gibberellins and inherently lack bioactivity.
- The bioactivity of C19 gibberellins is contingent upon specific structural features. For a C19 gibberellin to be bioactive, it must possess a 3β-hydroxyl group and lack a 2β-substituent. Within this category, GA1, GA3, GA4, and GA7 are recognized for their pronounced biological activity. This heightened activity likely contributed to them being among the earliest isolated gibberellins. In many plant species, GA1 is recognized as the primary active hormone. However, in certain species like Arabidopsis thaliana, GA4 exhibits greater bioactivity than GA1, suggesting its role as the dominant active hormone. Additionally, other gibberellins, such as GA3 (commonly referred to as gibberellic acid), have been identified as influential in the flowering process. Notably, GA3 is the predominant gibberellin in G. fujikuroi and is commercially produced through fungal fermentations, finding applications in horticulture, agronomy, and brewing.
- Chemically, gibberellin is a diterpenoid, a molecule that also forms the foundation for compounds like vitamins A and E. The structure of gibberellin A1, the inaugural identified gibberellin, serves as a representative example. While the core structure remains consistent across different gibberellins, variations arise from the attachment of diverse side groups. These side groups confer specificity, dictating the distinct functions of gibberellins in various plant tissues.
- In terms of biosynthesis, while gibberellin molecules can be synthesized in multiple parts of a plant, they predominantly accumulate in the roots, distinguishing them from hormones like auxin, which are more concentrated at plant apexes. Presently, over 100 distinct gibberellin molecules have been identified, underscoring their diverse roles and the intricacy of their structural variations.
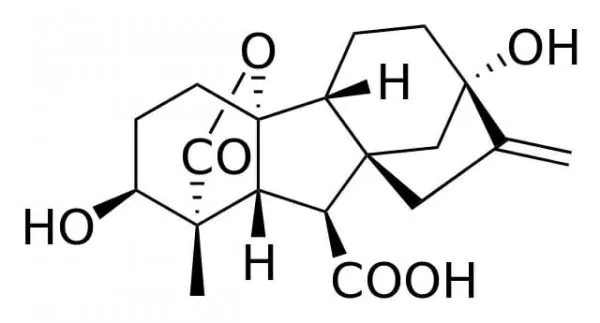
Biosynthesis of Gibberellin
Gibberellins, a class of plant hormones, play a pivotal role in various growth and developmental processes. The intricate pathway leading to the synthesis of GAs is a testament to the collaborative efforts of numerous scientists, including the likes of Jan Graebe, Jake MacMillan, Bernard Phinney, Nobutaka Takahashi, Jan Zeevaart, and their teams.
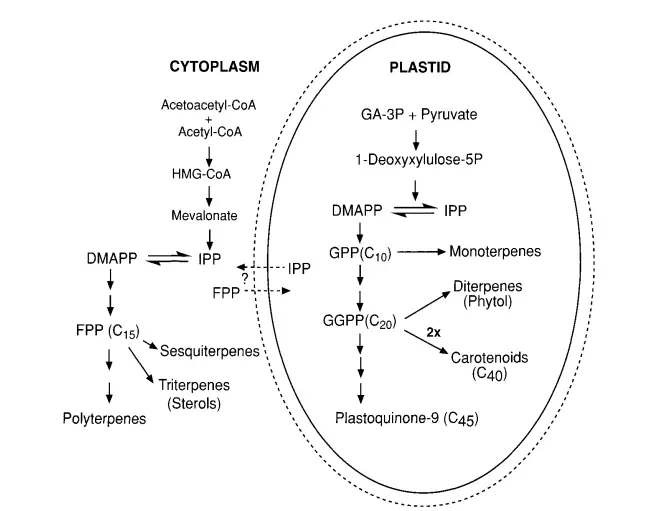
1. Initial Research and Methodology: Historically, the primary focus of GA biosynthesis research was on immature fruits or seeds, known for their rich accumulation of endogenous GAs. Radiolabeled precursors were employed to trace their incorporation into different compounds both in vivo and in vitro. The discovery that cell-free systems, derived from seeds and devoid of cellular debris, could synthesize GAs was a significant breakthrough. This in vitro synthesis, combined with advanced analytical techniques like HPLC, GC, and GC-MS, has greatly advanced our understanding of GA biosynthesis.
2. Stages of GA Biosynthesis:
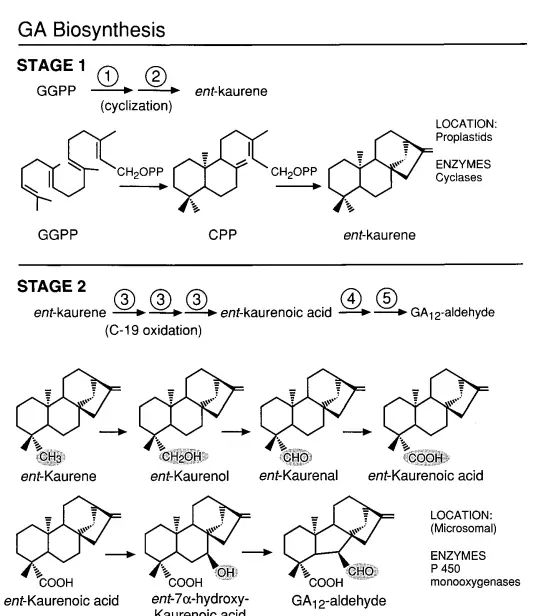
- Stage 1: Cyclization of GGPP
- The synthesis commences with the cyclization of GGPP (geranylgeranyl diphosphate) to produce the fully cyclized compound, ent-kaurene. This process involves two sequential enzymes: copalyl diphosphate synthase (CPS) and ent-kaurene synthase (KS). These enzymes are localized within plastids.
- Stage 2: Oxidation Processes
- Ent-kaurene undergoes a series of oxidations at the C-19 position, culminating in the formation of ent-7a-hydroxykaurenoic acid. This compound is then transformed into GA12-aldehyde through B ring contraction and further oxidation at C-6. The enzymes responsible for these oxidations, including ent-kaurene oxidase and ent-kaurenoic acid hydroxylase, are membrane-bound cytochrome P450 monooxygenases. These enzymes are typically associated with the endoplasmic reticulum.
- Stage 3: Diverse Pathways
- From GA12-aldehyde, the biosynthesis can diverge into multiple pathways, some of which are species-specific. Common steps include the oxidation of GA12-aldehyde to GA12, subsequent oxidations at C-20, and lactone formation. Depending on the plant species, GA12 can be hydroxylated at C-13 to produce GA53, which is then converted to GA20 and GA1. Alternatively, GA12 can lead to the formation of GA9 and GA4. The specific pathway taken is likely under developmental control, ensuring the synthesis of this crucial hormone remains flexible and adaptable.
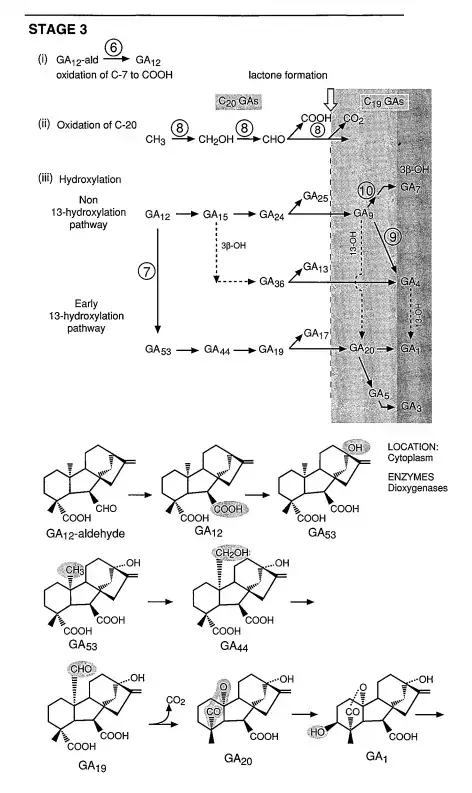
3. Enzymatic Roles in Stage 3: The early reactions in this stage, specifically the oxidation of GA12-aldehyde to GA12 and the 13-hydroxylation of GA12 to GA53, are believed to be facilitated by cytochrome P450 monooxygenases. Subsequent reactions, including C-20 oxidations and 2,3-desaturations, are orchestrated by dioxygenases that require 2-oxoglutarate and O2. These enzymes are typically found in the soluble fraction of in vitro experiments, suggesting their presence in the cytoplasm.
In conclusion, the biosynthesis of gibberellins is a complex, multi-step process that has been meticulously unraveled over the years. This pathway underscores the intricate balance and regulation required to produce these essential plant hormones.
Inhibitors of GA Biosynthesis
Gibberellin (GA) biosynthesis is a complex process that can be modulated by various synthetic compounds, commonly referred to as growth retardants. These compounds act by inhibiting GA synthesis, thereby affecting stem elongation and other growth processes.
1. Classification of GA Synthesis Inhibitors:
GA synthesis inhibitors can be categorized into three distinct classes, each targeting specific enzymes involved in the different stages of GA biosynthesis:
- Onium Compounds:
- This class includes quaternary ammonium compounds such as chlormequat chloride (CCC), mepiquat chloride, and AMO-1618, as well as phosphonium compounds like chlorphonium chloride. These compounds primarily inhibit the conversion of GGPP to ent-kaurene by targeting the enzymes copalyl diphosphate synthase and, to a lesser extent, ent-kaurene synthase.
- Nitrogen-containing Heterocyclic Compounds:
- Examples include ancymidol (a pyrimidine derivative), tetcyclacis (a norbornanodiazetine), and triazole-type compounds like paclobutrazol and uniconazole. These compounds obstruct the oxidation of ent-kaurene to ent-kaurenoic acid by inhibiting P450 monooxygenases.
- Acylcyclohexanediones:
- This group, which includes compounds like prohexadione-Ca and trinexapac-ethyl, inhibits the 2-oxoglutarate-dependent dioxygenases involved in the third stage of GA biosynthesis. Due to their structural similarity to 2-oxoglutarate, they are believed to compete for the binding site of the cosubstrate, 2-oxoglutarate.
2. Utility and Applications:
GA synthesis inhibitors serve as valuable tools for elucidating specific steps in the GA biosynthetic pathway, especially in plants where appropriate genetic mutants are unavailable. For instance, the acylcyclohexanedione BX-112, which inhibits 3β-hydroxylase, can be employed to determine which GAs actively promote stem elongation.
However, it’s crucial to recognize that these inhibitors target broad enzyme classes, implying they might influence other metabolic pathways beyond GA biosynthesis. For instance, while triazoles primarily inhibit GA synthesis, they also impact the metabolism of other hormones like abscisic acid (ABA) and brassinosteroids.
In the commercial realm, these growth retardants find applications in the agriculture and horticulture sectors. Compounds like chlormequat and mepiquat chloride are employed as antilodging agents in cereal crops and to curtail excessive vegetative growth in cotton. Acylcyclohexanediones, such as trinexapac-ethyl and prohexadione-Ca, are utilized for stem stabilization in cereal crops, growth control in turf grass, and to reduce vegetative growth in fruit trees.
In conclusion, inhibitors of GA biosynthesis play a pivotal role in both scientific research and commercial applications, offering insights into plant growth mechanisms and providing practical solutions for crop management.
Enzymes of GA Metabolism and Their Genes
The intricate process of gibberellin (GA) biosynthesis is orchestrated by a series of enzymes, the genes of which have been progressively identified and cloned over recent years.
1. Cloning and Identification of GA Enzymes:
With advancements in molecular biology, cDNAs and genes encoding the primary enzymes involved in GA biosynthesis have been successfully cloned. Various techniques, ranging from cloning from purified proteins based on amino acid sequences to homology-based techniques, have been employed. The availability of these cloned sequences has paved the way for a deeper understanding of the potential existence of different isoforms of the same enzyme, their specific localization in tissues or organs, and their relative abundance.
2. Isoforms and Their Specificity:
- GA 20-oxidases: Multiple cDNAs encoding GA 20-oxidases have been identified. These enzymes, when analyzed, appear to be products of a large gene family with several members present within a single plant species. These isoforms differ in their product outcomes and tissue specificity. For instance, while most cloned isoforms convert GA12 or GA53 to GA9 or GA20, a specific clone from pumpkin (Cucurbita maxima) produces primarily tricarboxylic GAs. In Arabidopsis, three distinct cDNAs for GA 20-oxidases have been identified, each exhibiting differential expression patterns across various organs.
- 3β-hydroxylases: Different isoforms of 3β-hydroxylases have been cloned from plants like Arabidopsis, pea, and lettuce. These isoforms exhibit organ-specific expression patterns.
- Early-stage enzymes: Unlike the later stages of GA biosynthesis, the early stages seem to have a limited number of genes encoding for enzymes like copalyl diphosphate synthase, ent-kaurene synthase, and ent-kaurene oxidase. For example, genomic analysis of Arabidopsis has revealed only one gene encoding each of these enzymes. These genes exhibit development-specific expression patterns.
3. Regulation and Expression Patterns:
The differential expression of GA biosynthesis genes across various organs suggests a nuanced regulatory mechanism. For instance, the le mutation in pea affects GA1 levels in shoot tissues, leading to dwarfism, but doesn’t impact GA content or growth in roots. In contrast, other mutations in pea, like Is, Ih, and na, which affect enzymes in the earlier stages of GA biosynthesis, result in GA deficiencies in both shoots and roots.
The identification and cloning of genes associated with GA metabolism have provided invaluable insights into the intricate mechanisms governing plant growth and development. The presence of multiple isoforms and their organ-specific expression patterns underscore the complexity and fine-tuned regulation of the GA biosynthetic pathway.
Organ and Subcellular Sites of GA Biosynthesis
Gibberellin (GA) biosynthesis, a pivotal process in plant growth and development, predominantly occurs in specific regions of the plant. This article delves into the organ-specific and subcellular sites associated with GA biosynthesis, based on empirical evidence and genetic studies.
1. Organ-Specific Sites of GA Biosynthesis:
Historically, GA biosynthesis has been associated with the younger parts of plants. Specifically:
- Shoot Apices: These are the growing tips of shoots, where new leaves and branching stems originate.
- Young Leaves: These are the newly formed leaves that haven’t reached their full size or maturity.
- Developing Fruits and Seeds: As fruits and seeds mature, they undergo rapid cell division and growth, necessitating GA biosynthesis.
- Young Roots: These are the growing parts of the root system.
Conversely, mature leaves and stems are generally considered deficient in GA synthesis. This understanding, derived from bioassays and radiolabeled precursor incorporation studies, aligns with the expression patterns of GAI and GA3 genes in Arabidopsis. Both genes predominantly express in young meristematic areas, such as root and shoot tips, procambial or cambial tissues, elongating stems, and floral parts. Their expression diminishes in older, mature plants and rosette leaves.
2. Subcellular Sites of GA Biosynthesis:
- Plastids: The initial steps of GA biosynthesis, specifically the conversion of GGPP to ent-kaurene, are believed to occur in plastids. Studies involving pea and wheat plants have shown that the incorporation of labeled GGPP into ent-kaurene is most prominent in proplastid or leucoplast fractions from areas characterized by rapid cell division and growth, such as shoot apices, intercalary meristems, and young endosperm tissue in seeds. Notably, this incorporation is absent in differentiated chloroplasts from mature leaves. Furthermore, the GAI gene encodes a protein with a plastid-targeting signal peptide, reinforcing the notion of ent-kaurene synthesis in plastids.
- Endoplasmic Reticulum (ER) and Cytosol: The subsequent stages (2 and 3) of GA biosynthesis involve enzymes located in the ER and the cytosol. Specifically, ER-bound P450 monooxygenases and cytosolic dioxygenases facilitate these stages. However, the mechanism by which ent-kaurene translocates from the plastids to the cytoplasm remains elusive.
3. Compartmentalization and Translocation:
The compartmentalization of different GA biosynthesis steps and the potential long-distance translocation of intermediate GAs within the plant are areas still under investigation.
The intricate process of GA biosynthesis is spatially regulated, with specific organs and subcellular sites playing pivotal roles. While significant strides have been made in understanding this process, certain aspects, such as the translocation of intermediates and compartmentalization, warrant further research.
Regulation Of GA Levels In The Plant
Gibberellins (GAs) are pivotal phytohormones that play a central role in plant growth and development. The precise regulation of active GAs, such as GA1 or GA4, within plant tissues is achieved through a delicate balance between synthesis, inactivation, conjugation, catabolic breakdown, and potential storage or transport mechanisms.
1. Regulation of Synthesis:
The multi-step GA biosynthetic pathway offers numerous checkpoints for regulatory control. These regulatory nodes can be influenced by developmental or environmental triggers, or even feedback mechanisms.
- Synthesis of ent-kaurene: The commitment of GGPP to CPP, catalyzed by copalyl diphosphate synthase, is a critical control point. This is evidenced by the low abundance of CPS in plant tissues and its transcript expression primarily in young, growing tissues.
- Environmental Regulation: Environmental factors, notably temperature and light, have profound effects on GA levels. For instance, in Thlaspi arvense, cold exposure followed by a return to normal temperature induces stem growth and flowering, which can be mimicked by GA application. Photoperiod also influences GA synthesis, with long days inducing flowering and stem elongation in many plants.
- Feedback Inhibition: Bioactive GAs or their metabolic products can exert negative feedback on GA synthesis. Primary targets for this feedback are GA 20-oxidases and 3β-hydroxylases. For instance, in the GA-deficient Arabidopsis mutant, gal, GA 20-oxidase gene transcript levels are elevated, but dramatically decrease upon GA3 treatment.
2. GA Conjugates:
Conjugation, which involves the addition of a sugar moiety to the GA molecule, renders the GA inactive. These conjugated GAs, known as GA-glucosyl esters, can be stored in maturing seeds or synthesized in vegetative tissues. The conjugation increases the molecule’s polarity, suggesting storage in vacuoles or potential long-distance transport in the phloem.
3. Irreversible Deactivation:
The primary mechanism for deactivating active GAs is through 2β-hydroxylation, leading to the formation of inactive GAs. This process is irreversible, and the products are the end products of GA catabolism. The slender mutant in pea, which is defective in 2β-hydroxylation, grows exceptionally tall due to an overabundance of active GA1. The wild-type gene, SLENDER, encodes a 2β-hydroxylase, which is a dioxygenase similar to GA 20-oxidases and 3β-hydroxylases.
4. Other Modifications:
Other naturally occurring modifications in GA structure include 3α-hydroxylation, resulting in 3-epi-GAs, which generally exhibit low biological activities. Methylation of C-6-COOH does not seem to occur naturally in higher plants, but artificially methylated GAs lack biological activity.
The regulation of GA levels in plants is a complex interplay of synthesis, deactivation, conjugation, and potential storage or transport mechanisms. This intricate balance ensures the appropriate physiological response to developmental and environmental cues, underscoring the significance of GAs in plant growth and development.
Catabolism & Homeostasis of Gibberellin
- Catabolism of Gibberellin: Gibberellins (GAs) undergo a series of deactivation processes to ensure that their levels are kept in check within the plant system. One of the primary mechanisms for inactivating GAs is through 2β-hydroxylation, a process catalyzed by enzymes known as GA2-oxidases (GA2oxs). Interestingly, there are variations within these enzymes; some GA2oxs target C19-GAs, while others act on C20-GAs. Another significant pathway for GA deactivation involves the cytochrome P450 mono-oxygenase, encoded by the gene “elongated uppermost internode” (eui). This enzyme transforms GAs into 16α,17-epoxides. In rice plants with eui mutations, there’s an accumulation of bioactive GAs, indicating the pivotal role of this enzyme in GA deactivation. Additionally, the genes Gamt1 and gamt2 encode enzymes responsible for the methylation of the C-6 carboxyl group of GAs, further contributing to their inactivation.
- Homeostasis of Gibberellin: Maintaining a balance in the levels of bioactive GAs is crucial for optimal plant growth and development. This balance, or homeostasis, is achieved through intricate feedback and feedforward regulatory mechanisms. In environments where GA is deficient, the expression levels of genes like AtGA20ox1 and AtGA3ox1 are elevated. However, when bioactive GAs are introduced, the expression of these genes diminishes. This showcases the feedback mechanism in play. On the other hand, genes responsible for GA deactivation, such as AtGA2ox1 and AtGA2ox2, see an increase in their expression upon the addition of GA, exemplifying the feedforward regulation.
In essence, the catabolism and homeostasis of gibberellin are sophisticated processes that ensure the plant’s growth and developmental activities are harmoniously regulated. These processes involve a series of enzymatic reactions and genetic regulations that collectively maintain the balance of this vital plant hormone.
Regulation
- Interplay with Other Hormones: Gibberellin (GA) regulation is intricately linked with other plant hormones. For instance, the auxin indole-3-acetic acid (IAA) plays a pivotal role in modulating the concentration of GA1 in elongating internodes of peas. When the apical bud, a primary source of IAA, is removed, there’s a marked decrease in GA1 concentration. Reintroducing IAA restores the GA1 levels. This auxin-GA interplay has also been observed in tobacco plants. In barley, auxin is known to enhance GA 3-oxidation while suppressing GA 2-oxidation. Furthermore, during the fruit development phase in peas, auxin has been identified as a regulator of GA biosynthesis. Another hormone, ethylene, has been found to reduce the concentration of bioactive GAs.
- Environmental Regulation: Environmental factors significantly influence GA regulation. For instance, GA concentrations play a role in light-regulated processes such as seed germination, photomorphogenesis during de-etiolation, and the photoperiodic regulation of stem elongation and flowering. Additionally, around a quarter of cold-responsive genes are associated with GA-regulated genes, indicating GA’s role in cold temperature responses. When plants experience stress, they tend to reduce their growth rate. In barley, a correlation between GA levels and the degree of stress has been suggested, indicating the hormone’s role in stress adaptation.
- Role in Seed Development: Gibberellin’s role in seed development and germination is closely tied to its interplay with abscisic acid (ABA). Typically, bioactive GAs and ABA levels exhibit an inverse relationship. The transcription factor FUS3 in Arabidopsis is upregulated by ABA and downregulated by GA, hinting at a regulatory loop that maintains the balance between GA and ABA during seed development.
In conclusion, the regulation of gibberellin is a multifaceted process, influenced by other hormones, environmental factors, and developmental stages. This intricate regulation ensures that plants can adapt and respond optimally to varying internal and external cues.
Signalling mechanism
1. Receptor Dynamics: The quest for a gibberellin (GA) receptor in the 1990s initially pointed towards a membrane-bound receptor in oat seeds. However, despite rigorous research, no such receptor has been conclusively identified. The discovery of a soluble receptor, GA insensitive dwarf 1 (GID1), has shifted the focus away from the existence of a membrane-bound counterpart.
2. GID1 and its Role: GID1, initially identified in rice, has three orthologs in Arabidopsis: AtGID1a, AtGID1b, and AtGID1c. These receptors exhibit a high affinity for bioactive GAs. When GA binds to GID1, it undergoes structural changes, leading to the formation of a GA-GID1-DELLA complex. This complex facilitates the interaction between GID1 and DELLA proteins, which are known repressors of plant development.
3. DELLA Proteins: The Repressors: Characterized by the DELLA motif, these proteins inhibit various developmental processes, including seed germination, growth, and flowering. The presence of GA promotes the degradation of DELLA proteins, liberating the cells from their inhibitory effects.
4. Targets of DELLA Proteins:
- Transcription Factors: DELLA proteins primarily target PHYTOCHROME INTERACTING FACTORs (PIFs), which are transcription factors that regulate light signaling and elongation growth. In the presence of GA, the degradation of DELLAs allows PIFs to stimulate elongation. Additionally, DELLAs repress numerous other transcription factors, influencing auxin, brassinosteroid, and ethylene signaling pathways.
- Prefoldins and Microtubule Assembly: DELLAs also interact with prefoldins (PFDs), molecular chaperones that assist in protein folding. In the absence of GA, DELLAs bind to PFDs, restricting them to the nucleus and reducing the cellular pool of β-tubulin, a crucial component of the cytoskeleton. The presence of GA promotes the degradation of DELLAs, allowing PFDs to facilitate the folding of β-tubulin, leading to cytoskeleton reorganization and cell elongation.
5. Microtubules and Hormone Transport: Microtubules play a pivotal role in membrane vesicle trafficking, essential for the correct positioning of hormone transporters, such as PIN proteins responsible for auxin movement between cells. In the absence of GA, reduced microtubule levels inhibit membrane vesicle trafficking, decreasing PIN protein levels at the cell membrane and subsequently reducing intracellular auxin levels. GA reverses this process, enhancing auxin levels within the cell.
In summary, the regulation of gibberellin operates through a complex signaling mechanism involving receptors, repressor proteins, and intricate cellular processes. This intricate regulation ensures optimal plant growth and response to environmental cues.
Gibberellin Functions
Gibberellins, a class of diterpenoid plant hormones, play a pivotal role in a myriad of plant developmental processes. Their functions are multifaceted and are intricately regulated, ensuring optimal growth and development in response to environmental cues.
- Seed Germination: Gibberellins are instrumental in the germination of certain light-sensitive seeds, such as tobacco and lettuce. In the absence of adequate sunlight, these seeds exhibit suboptimal germination. However, treatment with gibberellic acid, a type of gibberellin, can circumvent this light requirement, prompting rapid germination. Furthermore, gibberellins facilitate the digestion of the endosperm, a nutrient-rich tissue, by stimulating the aleurone layer to produce enzymes like amylases. These enzymes convert stored starches into glucose, providing the essential energy for the sprouting embryo.
- Dormancy of Buds: Buds formed during autumn often enter a dormant state, only to awaken the following spring. Gibberellins can expedite this awakening process, effectively breaking the dormancy.
- Root Growth: While gibberellins generally exhibit minimal influence on root growth, certain plants might experience growth inhibition at elevated gibberellin concentrations.
- Elongation of Internodes: One of the most pronounced effects of gibberellins is the elongation of plant internodes. In genetically dwarf plants, such as dwarf pea and maize, gibberellins can override the inherent dwarfism, leading to extended internodes. This effect is achieved through the modulation of gene transcription. Specifically, gibberellin binds to soluble protein receptors within the cell, initiating a cascade that ultimately results in the degradation of DELLA proteins. This degradation releases transcriptional inhibition, allowing for gene expression that promotes internode elongation.
- Interaction with Other Plant Hormones: Gibberellins do not operate in isolation. Their function is intricately intertwined with other plant hormones. For instance, there exists a complementary relationship between gibberellin and auxin levels. Conversely, ethylene tends to reduce gibberellin concentrations. Such interactions allow plants to maintain a delicate balance, ensuring optimal response to environmental stimuli. For example, temperature-induced changes in gibberellin levels can trigger processes like flowering and fruiting.
- Additional Roles: Beyond the aforementioned functions, gibberellins are implicated in various other plant processes, including flowering, fruiting, and senescence. Their levels and activity are often modulated by environmental factors, ensuring that plants can adapt and respond effectively to changing conditions.
In summary, gibberellins are integral to the intricate tapestry of plant growth and development. Their multifunctional nature, combined with their interactions with other hormones, underscores their significance in ensuring plants thrive in diverse environments.
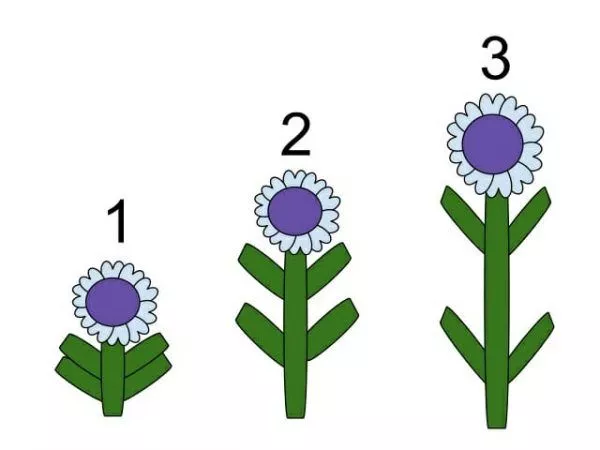
Uses of Gibberellins
Gibberellins, a class of plant growth hormones, play a pivotal role in various aspects of plant development and have found significant applications in agriculture, horticulture, and other industries. Their primary function is to stimulate cell elongation, which, in turn, leads to the overall growth of the plant, akin to how the human growth hormone, secreted by the pituitary gland, influences human growth. In plants, gibberellins are secreted by plastids, orchestrating a range of developmental processes.
- Greenhouse and Flower Industries: Gibberellins have a profound impact on the global greenhouse and flower sectors. Many dwarf plant varieties, popular in landscaping, exhibit stunted growth either due to their inability to produce gibberellins or their cells’ insensitivity to this hormone. For instance, bonsai trees, a staple in ornamental horticulture, have notably low gibberellin levels, contributing to their dwarf stature.
- Fruit Production: Farmers have harnessed the potential of gibberellins to enhance fruit production. By applying synthetic gibberellins to trees, they can stimulate the growth of buds and fruits, thereby increasing yield. This application is particularly evident in grapevines, where gibberellins are sprayed to augment the size of the grapes.
- Seed Germination: Commercially sourced from fungi, gibberellins are employed to expedite seed germination, ensuring a more uniform and efficient sprouting process.
- Flower Production in Biennial Plants: Typically, biennial plants flower under specific low-temperature conditions. However, with the application of gibberellins, these plants can be induced to flower irrespective of the prevailing temperatures, offering flexibility in cultivation practices.
- Hybridization in Cucumber Plants: Gibberellins are utilized to induce the production of all-male flowers in cucumber plants. This targeted production facilitates the extraction of pollen with desired characteristics, which is subsequently used for hybridization, leading to improved crop varieties.
- Growth Stimulation in Dwarf Varieties: Certain plant varieties are genetically predisposed to exhibit dwarfism. By administering gibberellins to these plants, their inherent growth limitations can be overcome, leading to enhanced growth and stature.
In conclusion, gibberellins, with their multifaceted roles in plant growth and development, have been harnessed in various industries to optimize plant growth, improve yields, and facilitate specific cultivation practices. Their versatility underscores their significance in modern agriculture and horticulture.
Quiz
Which of the following is NOT a primary function of gibberellins in plants?
a) Stem elongation
b) Seed germination
c) Flowering induction
d) Nitrogen fixation
Which enzyme is crucial for the conversion of GGPP to ent-kaurene in the GA biosynthetic pathway?
a) GA 20-oxidase
b) 3β-hydroxylase
c) Copalyl diphosphate synthase
d) 2β-hydroxylase
In which part of the plant are gibberellins primarily synthesized?
a) Mature leaves
b) Stems
c) Young roots
d) Flower petals
Which process renders gibberellins inactive by adding a sugar moiety?
a) Hydroxylation
b) Methylation
c) Conjugation
d) Oxidation
The slender mutant in pea plants is defective in which process?
a) 2β-hydroxylation
b) 3β-hydroxylation
c) Conjugation
d) Methylation
Which environmental factor is NOT known to influence GA levels in plants?
a) Light
b) Temperature
c) Photoperiod
d) Soil pH
Which of the following is a bioactive form of gibberellin?
a) GA1
b) GA29
c) GA34
d) GA53
The feedback inhibition of GA synthesis primarily targets which enzymes?
a) GA 20-oxidases and 3β-hydroxylases
b) 2β-hydroxylase and 3β-hydroxylase
c) Copalyl diphosphate synthase and ent-kaurene synthase
d) GA 20-oxidase and 2β-hydroxylase
Which gibberellin is known to be involved in stem elongation?
a) GA3
b) GA9
c) GA12
d) GA53
Where is the conversion of GGPP to ent-kaurene believed to occur within the cell?
a) Nucleus
b) Mitochondria
c) Plastids
d) Endoplasmic reticulum
FAQ
What are gibberellins?
Gibberellins (GAs) are a group of plant hormones that play essential roles in various growth and developmental processes, including stem elongation, seed germination, and flowering.
How many types of gibberellins are there?
Over 130 gibberellins have been identified in plants, fungi, and bacteria, but only a few are biologically active in plants.
Where are gibberellins produced in plants?
Gibberellins are primarily synthesized in young parts of the plant, such as shoot apices, young leaves, developing fruits and seeds, and young roots.
What role do gibberellins play in seed germination?
Gibberellins help break seed dormancy by stimulating the synthesis of enzymes that degrade the seed storage proteins, providing nutrients for the growing embryo.
How do gibberellins affect stem elongation?
Gibberellins promote cell elongation and division in stems, leading to increased stem length.
Are gibberellins involved in flowering?
Yes, gibberellins play a crucial role in the induction of flowering, especially in plants that require specific photoperiods to bloom.
How are gibberellin levels regulated in plants?
The concentrations of active GAs are regulated through their synthesis, inactivation by conjugation, catabolic breakdown, and feedback mechanisms.
Can gibberellins be used commercially?
Yes, gibberellins are used in agriculture to promote growth, induce flowering, and increase fruit size in certain crops.
What is the relationship between gibberellins and light?
Light, especially red light, can influence GA levels in plants, affecting processes like stem elongation and seed germination.
Are there any negative effects of excessive gibberellins in plants?
Excessive levels of gibberellins can lead to undesirable growth patterns, such as elongated stems, reduced root growth, and decreased fertility.
References
- Sponsel, V. M. (2003). Gibberellins. Encyclopedia of Hormones, 29–40. doi:10.1016/b0-12-341103-3/00108-x
- Srivastava, L. M. (2002). Gibberellins. Plant Growth and Development, 171–190. doi:10.1016/b978-012660570-9/50148-9
- AGRIOS, G. N. (2005). HOW PATHOGENS ATTACK PLANTS. Plant Pathology, 175–205. doi:10.1016/b978-0-08-047378-9.50011-7
- Bhattacharya, A. (2019). Effect of High-Temperature Stress on the Metabolism of Plant Growth Regulators. Effect of High Temperature on Crop Productivity and Metabolism of Macro Molecules, 485–591. doi:10.1016/b978-0-12-817562-0.00006-9
- Hedden, P. (2003). REGULATORS OF GROWTH | Gibberellins. Encyclopedia of Applied Plant Sciences, 1011–1019. doi:10.1016/b0-12-227050-9/00064-8
- Gao, X., Zhang, Y., He, Z., & Fu, X. (2017). Gibberellins. Hormone Metabolism and Signaling in Plants, 107–160. doi:10.1016/b978-0-12-811562-6.00004-9
- Sponsel, V. M. (2003). Gibberellins. Encyclopedia of Hormones, 29–40. doi:10.1016/b0-12-341103-3/00108-x