What is Flagella?
- A flagellum, derived from the Latin word “flagellum,” meaning whip or scourge, is a slender, hair-like organelle found in various organisms, playing a pivotal role in motility. This remarkable biological structure consists of a complex assembly of proteins, lipids, and other molecular components. Flagella are responsible for facilitating the movement and propulsion of cells and microorganisms, showcasing the intricate design of nature.
- The flagellum exhibits extraordinary mechanical precision, capable of achieving rapid rotational speeds, often reaching several hundred revolutions per second. This rotation generates a whip-like motion, propelling the microorganism forward with impressive speed and agility. This remarkable feat allows bacteria to navigate toward favorable environments, evade harmful substances, and search for nutrients, all essential for their survival.
- Flagella are not homogenous structures; rather, they exhibit considerable diversity in their types and assembly complexity across various organisms. The primary purpose of flagella remains the same – cellular movement. However, the detailed structures and mechanisms differ among bacteria, archaea, and eukaryotes.
- In bacterial flagella, these helical filaments, composed of flagellin proteins, rotate like screws, propelling the bacterium in a corkscrew-like manner. The filament is connected to a rotary motor at its base, passing through protein rings embedded in the cell membrane.
- In contrast, archaeal flagella are nonhomologous and exhibit distinct features from bacterial flagella. They are also filamentous structures protruding outside the cell, but unlike bacterial flagella, the filaments rotate as a single assembly, rather than independently.
- Eukaryotic flagella, found in certain animal cells, plant cells, and protists, are made up of microtubules surrounded by the plasma membrane. They enable cells to move in a whip-like fashion, contributing to various physiological functions, including cell motility and sensory perception.
- Beyond motility, flagella serve other crucial functions in some cells. These functions include attachment to surfaces or host tissues, capturing food particles, sensory perception, and signal transduction. In sensory cells like rod photoreceptor cells in the eye, olfactory receptor neurons in the nose, and kinocilium in the cochlea of the ear, flagella play a pivotal role in detecting environmental changes such as light, odor, and sound.
- Flagella are not confined to a single group of organisms; they are found in diverse microscopic and macroscopic life forms, including bacteria, fungi, algae, and certain animals. The study of flagella offers valuable insights into molecular biology, evolution, and the mechanisms governing bacterial cell movement and pathogenicity. Furthermore, the efficiency and precision of flagellar motion have inspired scientists and engineers to develop micro-robotic systems capable of navigating complex environments, with potential applications in fields such as medicine, environmental monitoring, and nanotechnology.
- In summary, the flagellum is a fascinating and multifunctional organelle found in various organisms, serving as a biological motor for cellular movement and sensory perception. Its diverse structures and mechanisms across different domains of life exemplify the elegance and efficiency of nature’s design, making it a subject of significant scientific interest and innovation.
Definition od Flagella
Flagella are slender, whip-like appendages extending from certain cells, primarily serving the purpose of motility and, in some organisms, functioning as sensory organelles. They are found across various organisms, from bacteria and archaea to eukaryotic cells.
Types of Flagella
Flagella, whip-like appendages found in various organisms, play a pivotal role in motility and sensory functions. These structures, while sharing a common name, exhibit distinct characteristics across different domains of life. Here, we delve into the three primary types of flagella, elucidating their unique features and functions.
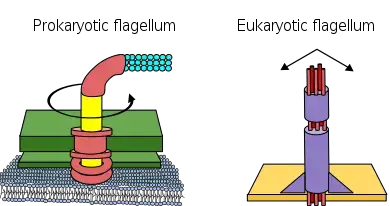
- Bacterial Flagella: Bacterial flagella are characterized by their helical coil, extending slightly longer than their archaeal and eukaryotic counterparts. With a diameter of approximately 20 nanometers, these flagella are slenderer than eukaryotic flagella. The number and length of flagella vary among bacterial species. While primarily facilitating locomotion, bacterial flagella can also serve as sensory structures, detecting environmental changes. Notably, their filaments typically adopt a left-handed helix, propelling the bacteria through counter-clockwise rotation.
- Archaeal Flagella: Distinct from bacterial flagella, the archaeal flagellum is a specialized motility structure. Despite similarities in assembly with bacterial flagella, their composition is markedly different. Present in diverse archaeal groups, including halophiles, methanogens, and thermophiles, these flagella are thinner in diameter compared to bacterial flagella. The proteins within archaeal flagella form a right-handed helix, resulting in a clockwise rotation that propels the cell at a relatively faster pace. Additionally, the hooks in archaeal flagella exhibit variability in length across species. Furthermore, the switching mechanism and sensory control of archaeal flagella differ from those in bacteria.
- Eukaryotic Flagella: Eukaryotic flagella, found in various algae and specific animal cells like sperm, are multifunctional structures involved in motility, feeding, and reproduction. In certain algae, they also act as sensory antennae. These flagella differ significantly from bacterial flagella in terms of architecture, composition, mechanism, and assembly. Comprising several hundred distinct proteins, eukaryotic flagella are more complex than the bacterial variants, which contain around 30 proteins. Their motility is driven by dynein-dependent microtubule sliding, contrasting with the rotary motor mechanism observed in bacterial flagella. The genesis of eukaryotic flagella stems from the microtubule-based centriole, from which the axoneme extends. This centriole often serves as the flagella’s basal body in eukaryotic cells.
Bacterial Flagella
- Structure: Comprises a filament, hook, and a flagellar basal body. The rotary motion of the flagellum propels the bacterium.
- Function: Essential for cell motility, chemotaxis, and biofilm formation.
- Variants:
- Assembly: Sequential addition of protein subunits at the flagellar filament’s growing tip.
- Evolutionary Aspect: Gene duplication can lead to the diversification of flagella.
- Example: Escherichia coli (E. coli), a gram-negative bacterium, possesses peritrichous flagella, enabling rapid, directed movement. The flagellar motor in E. coli uses the proton motive force.
Archaeal Flagella
- Structure: Similar to bacterial flagella but distinct in composition.
- Function: Enables movement in archaea.
- Composition: Composed of archaellin, unlike bacterial flagella which have a complex protein sequence.
- Mechanism: Uses ATPases for rotation, differing from the proton or sodium ion gradient in bacterial flagella.
Eukaryotic Flagella
- Structure: Comprises microtubule-based structures called flagellar axonemes.
- Function: Involved in cell motility, sensory perception, fluid movement, and reproductive processes.
- Motion: Exhibits a beating motion, contrasting the rotational motion of bacterial and archaeal flagella.
- Example: Sperm cells in animals have a flagellum that propels them through the female reproductive tract. The flagellum’s structure, with microtubules and molecular motors, ensures efficient movement.
In summary, flagella, though present across various organisms, exhibit distinct structural and functional characteristics. These differences highlight the evolutionary adaptations that organisms have undergone to thrive in their respective environments.
In conclusion, while flagella across different domains share a common purpose of facilitating movement and sensory functions, their structural and functional nuances underscore the diversity and adaptability of life forms across the evolutionary spectrum.
Bacterial flagella arrangement
There are five types of pattern in bacterial flagella such as;
1. Atrichous
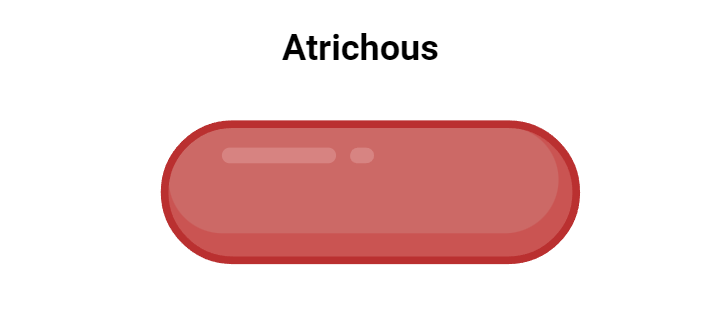
In the vast realm of bacterial diversity, the presence or absence of flagella often determines the motility of these microorganisms. Atrichous bacteria stand out as a unique category within this spectrum, characterized by their lack of flagella. The term “atrichous” is derived from the Greek prefix “a-“, signifying “without,” and “trichos,” translating to “hair.”
- Characteristics and Examples: Atrichous bacteria, devoid of flagella or analogous motile structures, are inherently non-motile. Notable examples of such bacteria include species from the genera Streptococcus and Lactobacillus. While these bacteria lack the means for active movement, they employ alternative mechanisms, such as passive diffusion or active transport, to navigate their environment.
- Ecological Significance: Despite their non-motile nature, atrichous bacteria flourish in diverse habitats. For instance, Lactobacillus species predominantly inhabit the human gastrointestinal tract, where they play a pivotal role in gut homeostasis and overall health. Their presence aids in digestion, nutrient absorption, and the fortification of the gut’s defense mechanisms.
- Adaptive Evolution: The evolutionary trajectory leading to the absence of flagella in atrichous bacteria is intriguing. It is postulated that this absence is an adaptive response tailored to their specific ecological niches. Bacteria residing in environments with minimal external stimuli might not necessitate active movement for survival. Conversely, those adapted to specialized habitats might have evolved distinct movement strategies, more attuned to their environmental context.
- Case Study – Lactobacillus lactis: Lactobacillus lactis, an exemplary atrichous bacterium, thrives in dairy environments. While it lacks flagella, its metabolic activities are crucial for the fermentation processes in cheese and yogurt production. Its non-motile nature does not impede its significance in both natural ecosystems and industrial applications.
In summation, atrichous bacteria, despite their lack of flagella, remain integral to various ecological and industrial processes. Their existence underscores the myriad ways in which microorganisms adapt and thrive in diverse environments.
2. Monotrichous
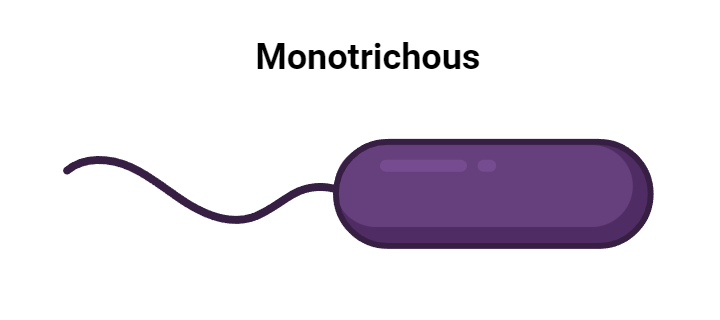
In the diverse world of bacterial motility, the monotrichous arrangement stands out due to its simplicity. Monotrichous bacteria are characterized by the presence of a solitary flagellum on each cell. When this lone flagellum is located at one of the poles of the bacterial cell, it is specifically termed a “monotrichous polar” arrangement.
Mechanism of Movement: The motility mechanism of monotrichous flagella is streamlined yet effective. The movement is orchestrated by various chemoreceptors that detect environmental changes and subsequently modulate the cell’s motility. These sensory receptors perceive external alterations, leading to the establishment of a transmembrane electrochemical gradient of ions. This gradient serves as the driving force for the bacterial flagellar motor.
Upon activation, the motor generates a thrust, which is relayed to the hook and subsequently to the filament. This results in a counterclockwise rotation of the flagellum, propelling the bacterium forward in a motion termed a ‘run’. The directionality of the bacterial movement in a monotrichous configuration is primarily governed by this counterclockwise rotation. Interestingly, when the flagellum rotates in the opposite direction, it pulls the bacterium backward, facilitating a change in orientation or ‘tumble’.
Exemplary Bacteria with Monotrichous Arrangement: Several bacterial species exhibit the monotrichous arrangement of flagella. Notable examples include:
- Vibrio cholerae: The causative agent of cholera, this bacterium uses its monotrichous flagellum for swift movement in aquatic environments.
- Campylobacter spp.: These bacteria are commonly associated with gastrointestinal infections in humans and navigate the gut environment using their single flagellum.
- Caulobacter crescentus: A model organism for studying bacterial cell cycle and differentiation, it possesses a single polar flagellum that aids in its movement.
In conclusion, the monotrichous arrangement offers a unique and efficient mode of motility for certain bacteria. Despite having just one flagellum, these bacteria exhibit remarkable agility and responsiveness to their surroundings, underscoring the adaptability and diversity of bacterial locomotion mechanisms.
3. Lophotrichous
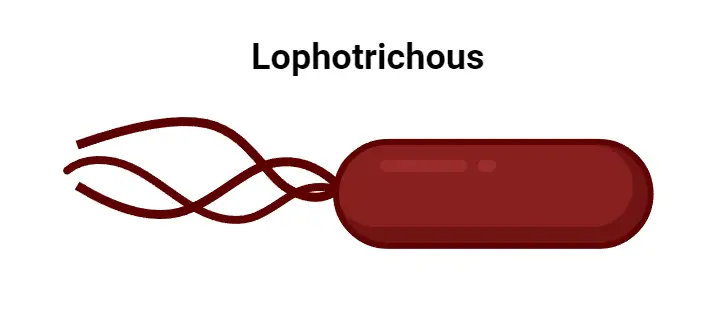
In the realm of bacterial motility, the lophotrichous configuration represents a unique arrangement where multiple flagella emerge from a singular point on the bacterial cell, typically at one of its poles. This clustered arrangement not only enhances the bacterium’s propulsion but also provides a distinct advantage in navigating diverse environments.
Structural Characteristics: Lophotrichous bacteria are characterized by a tuft of flagella that originate from the same site, often the polar end of the cell. This cluster of flagella is anchored within a specialized region of the cell membrane, termed the “polar organelle,” which provides structural support and ensures coordinated movement.
Mechanism of Movement: The motility mechanism of lophotrichous bacteria is intriguing. While the movement is initiated by environmental stimuli, similar to monotrichous bacteria, the presence of multiple flagella necessitates synchronized motion for efficient propulsion. Each flagellum is powered by its own motor, but the collective response to environmental cues ensures that all motors are activated simultaneously. This results in a unified thrust, propelling the bacterium forward.
However, directional changes present a challenge. To alter its course, the bacterium must coordinate the separation of its flagella in response to environmental stimuli. This orchestrated movement allows the bacterium to navigate its surroundings with precision.
Exemplary Bacteria with Lophotrichous Arrangement: Several bacterial species exhibit the lophotrichous configuration:
- Spirillum: A spiral-shaped bacterium, Spirillum uses its tuft of flagella for efficient movement in aquatic habitats.
- Pseudomonas fluorescens: Known for its biocontrol properties and ability to suppress plant diseases, this bacterium employs its lophotrichous flagella for effective navigation within its environment.
In summation, the lophotrichous arrangement offers bacteria a unique motility advantage, allowing for powerful propulsion and precise navigation. The coordinated movement of the clustered flagella underscores the intricate regulatory mechanisms that govern bacterial locomotion, highlighting the adaptability and sophistication of these microorganisms.
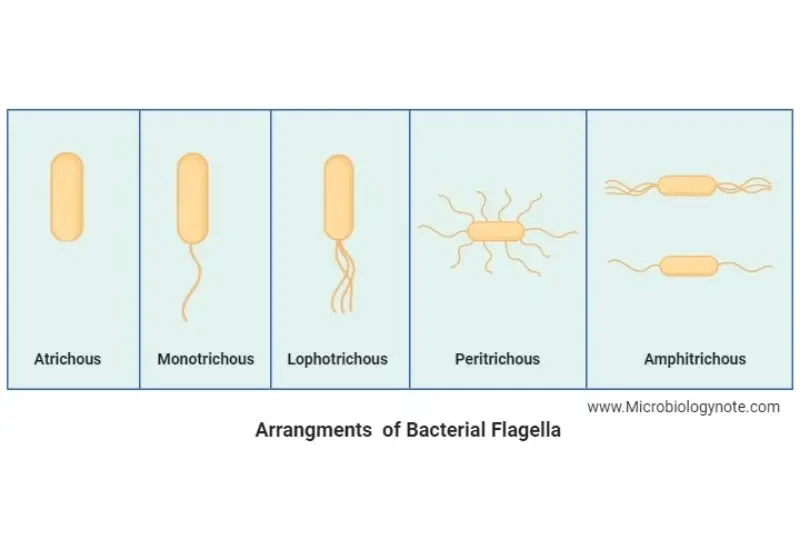
4. Amphitrichous
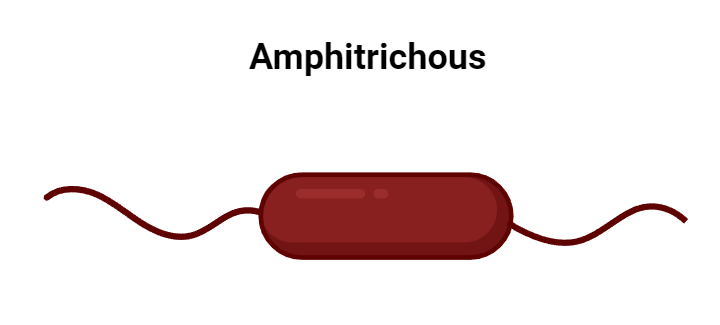
Amphitrichous flagellation represents a unique configuration in bacterial motility, characterized by the presence of flagella at both poles of the bacterial cell. This dual-polar arrangement provides bacteria with a distinct advantage in movement and navigation, allowing for versatile responses to environmental stimuli.
Structural Characteristics: Amphitrichous bacteria possess either a singular flagellum or a tuft of flagella at each of their polar ends. When multiple flagella are present, they are anchored within a specialized region of the cell membrane known as the “polar organelle.” This organelle ensures the structural integrity and coordinated movement of the flagella.
Mechanism of Movement: The motility mechanism of amphitrichous bacteria is multifaceted and not entirely understood. However, several observations provide insights:
- Differential Operation: Studies suggest that the flagella at each pole may operate independently, with each capable of moving in a specific direction, akin to the monotrichous or lophotrichous configurations.
- Simultaneous Rotation: Another perspective posits that both sets of flagella work in tandem but rotate in opposing directions. This synchronized yet opposite rotation could facilitate intricate maneuvers, allowing the bacterium to navigate complex environments with precision.
- Common Motility Mechanism: Despite the unique arrangement, the fundamental principles governing the movement of amphitrichous flagella are consistent with other flagellar configurations. They respond to environmental cues, undergo rotation, and propel the bacterium in a desired direction.
Representative Bacteria with Amphitrichous Flagellation:
- Campylobacter jejuni: A well-known pathogen, C. jejuni utilizes its amphitrichous flagella for effective movement within the host’s gastrointestinal tract.
- Rhodospirillum rubrum: This photosynthetic bacterium employs its dual-polar flagella to navigate aquatic habitats efficiently.
- Magnetospirillum: Recognized for its magnetotactic behavior, Magnetospirillum uses its amphitrichous flagella to move in response to magnetic fields.
In conclusion, the amphitrichous arrangement offers a unique motility advantage, allowing bacteria to harness the power of flagella at both poles. This dual-polar propulsion system underscores the adaptability of bacteria, enabling them to thrive in diverse environments.
5. Peritrichous
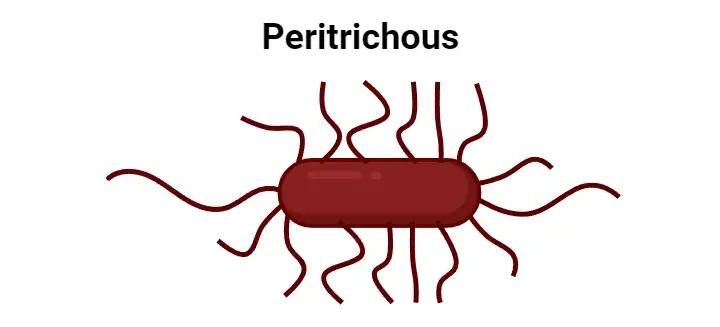
Peritrichous flagellation represents a distinctive configuration in bacterial motility, characterized by the presence of flagella uniformly distributed over the entire bacterial cell surface. This widespread arrangement provides bacteria with enhanced maneuverability, enabling them to navigate through diverse environments with agility and precision.
Structural Overview: In the peritrichous configuration, flagella emanate from multiple points on the bacterial cell, extending in various directions. This scattered distribution ensures that the bacterium can propel itself in any direction, offering a strategic advantage in response to environmental cues.
Mechanism of Movement: The movement dynamics of peritrichous bacteria are intricate:
- Bundled Movement: When moving towards an attractant, the flagella bundle together, propelling the bacterium forward in a straight line, a motion termed as the ‘run.’
- Phosphorylation Cascade: In the presence of a repellent, a series of phosphorylation events are initiated, altering the phosphorylation status of the regulatory protein, CheY.
- Motor Interaction and Flagellar Rotation: The activated CheY regulator interacts directly with the flagellar motor switch proteins. This interaction induces the flagella to rotate clockwise, disrupting the bundled arrangement.
- Tumbling and Reorientation: The disintegration of the flagellar bundle causes the bacterium to tumble, altering its direction. Following this, the bacterium resumes its run in a new direction. This tumbling and reorientation continue until the bacterium senses another stimulus, allowing it to navigate efficiently through its environment.
Exemplary Bacteria with Peritrichous Flagellation:
- Escherichia coli: A model organism for studying bacterial motility, E. coli employs its peritrichous flagella to move adeptly in response to environmental gradients.
- Bacillus subtilis: This gram-positive bacterium utilizes its peritrichous flagella for effective movement, especially during the vegetative phase.
- Salmonella: A pathogenic bacterium, Salmonella uses its peritrichous flagella to navigate through the host’s gastrointestinal tract.
- Klebsiella: Another pathogenic bacterium, Klebsiella relies on its peritrichous flagella for movement, especially during infections.
In summary, the peritrichous arrangement offers bacteria a comprehensive motility system, allowing them to move with versatility and adaptability, responding promptly to environmental changes.
Type of Flagella | Description | Examples |
---|---|---|
Atrichous | Bacteria that do not possess flagella or any similar structure for motility. | Streptococcus, Lactobacillus |
Monotrichous | Presence of a single flagellum in each cell, often at the polar end. | Vibrio cholerae, Campylobacter spp., Caulobacter crescentus |
Lophotrichous | Multiple flagella present at the same point in the cell, usually at the polar end. | Spirillium, Pseudomonas fluorescens |
Amphitrichous | Either a single flagellum or multiple flagella at both polar ends of the cell. | Campylobacter jejuni, Rhodospirillum rubrum, Magnetospirillum |
Peritrichous | Flagella are uniformly distributed over the entire surface of the cell. | Escherichia coli, Bacillus subtilis, Salmonella, Klebsiella |
Bacterial Flagella Structure
Bacterial flagella are composed of flagellin protein. These are 20-30 nm in diameter and about 15µm long. Flagellum is made of three important part such as;
- Basal Body
- Hook
- Filament
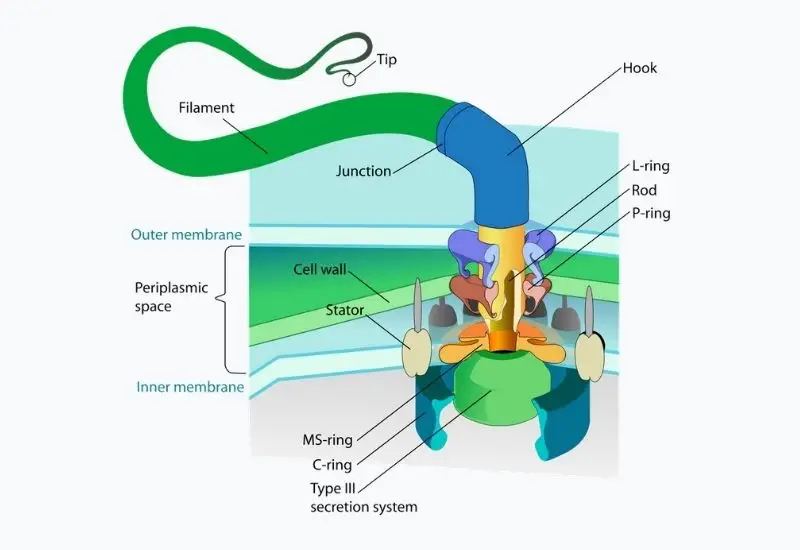
Bacterial flagella, distinct from their eukaryotic and archaeal counterparts, are intricate locomotive appendages that extend from the bacterial cell surface. These structures are pivotal for bacterial motility, allowing them to navigate through various environments. The bacterial flagellum is a marvel of biological engineering, comprising several components, each with its unique function and structure.
1. Filament
- The filament, a crucial component of the flagellum, constitutes approximately 98% of the flagellum’s entire structure, making it the most conspicuous segment.
- Extending outward from a hook-like structure situated within the cell’s cytoplasm, the filament boasts an average length of 18 nm. However, this length is not consistent across all organisms. For instance, bacterial flagella have filaments measuring around 20 nm, while those in archaea typically range between 10-14 nm.
- Visible outside the cell membrane, the filament’s presence can be accentuated using specific flagellar staining techniques. Intriguingly, the movement of this filament is orchestrated by a motor located within the cytoplasm, ensuring precise and coordinated motion.
- At its core, the filament is a sophisticated assembly of macromolecular structures. It is primarily composed of flagellin proteins and hook proteins. The exact number of these subunits can vary among different cells.
- The flagellin proteins, which are arranged in a helical configuration, bestow the filament with its structural robustness and the flexibility vital for its function.
- As the primary driver of propulsion, the filament creates undulating waves or engages in rotational actions, enabling the organism to navigate through fluidic environments with ease.
2. Hook
- The flagellar hook serves as a pivotal junction in the flagellar apparatus, acting as a bridge between the basal body (or flagellar motor) and the elongated filament.
- This curved, tubular structure is not merely a connector but plays a vital role in translating the torque generated by the motor to the helical filament, enabling it to execute diverse movements and functions. Moreover, the hook is instrumental during the assembly process of the flagellum.
- Constructed from a multitude of hook protein subunits, the hook manifests as polymorphic supercoil structures. While this component is universally present adjacent to the cell membrane across various cell types, its precise shape and composition can exhibit variations.
- Delving deeper into its architecture, the hook is demarcated into two distinct regions: the Connector Region and the Bend Region. The former, as the name suggests, latches onto the filament, establishing a flexible linkage that facilitates rotational dynamics.
- The latter, the bend region, is characterized by a pronounced curve or bend in the hook. This unique configuration imparts added flexibility to the flagellum, equipping it with the agility to alter its direction and adeptly maneuver through diverse environments.
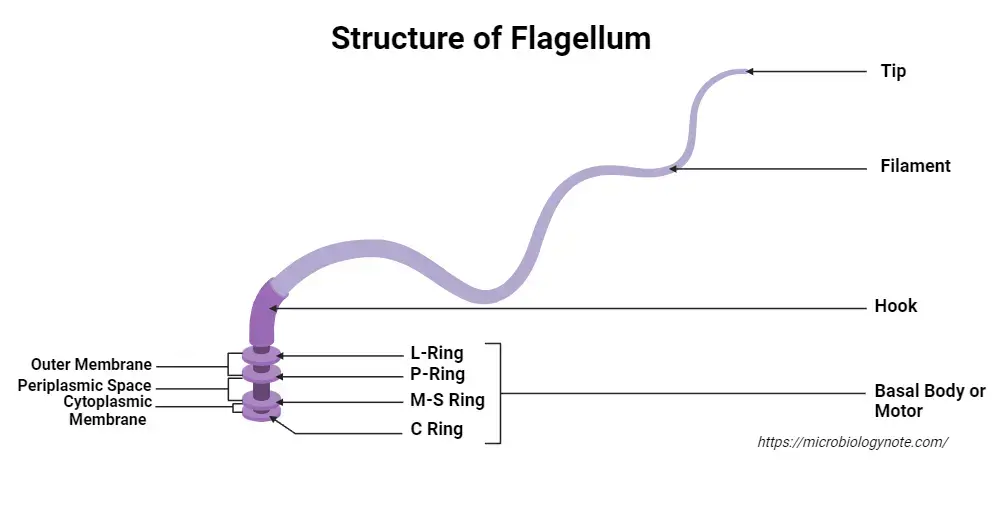
3. Basal Body
The basal body, an intricate structure situated at the base of the flagellum, plays a pivotal role in anchoring the flagellum to the cell membrane and orchestrating its movement. This foundational component not only offers structural reinforcement to the flagellum but also houses the motor proteins responsible for generating the torque essential for the flagellum’s rotational dynamics.
Delving into its architecture, the basal body comprises several integral components:
- Rod: Serving as the structural backbone of the flagellum, the rod is a robust, cylindrical entity that extends from the hook deep into the cell. Composed of specific proteins, the rod ensures the flagellum’s structural integrity and stability. Furthermore, it plays a crucial role in transmitting the rotational forces, produced by the motor, to the filament, culminating in the flagellum’s movement.
- Rings:
- L-ring: Exclusively found in gram-negative bacteria, the L-ring is an outer membrane protein ring. It functions as a bridge, connecting the basal body to the cell envelope.
- P-ring: Situated in the periplasmic space of gram-negative bacteria, the P-ring, or peptidoglycan ring, offers structural support by linking the rod to the cell wall.
- MS-ring: Nestled within the cytoplasmic membrane, the MS-ring serves as a foundation for the assembly of motor proteins, ensuring a stable base for the motor’s operations.
- C-ring: Also referred to as the rotor, the C-ring is positioned at the flagellum’s base and is an integral part of the motor complex. It facilitates rotational movement by interacting with the motor proteins.
- Motor: Residing within the basal body, the motor is a sophisticated protein assembly encompassing a stator and a rotor.
- Stator: Embedded within the cell membranes, the stator acts as a fixed anchor. It interacts with the rotor to produce torque. The proteins constituting the stator are instrumental in transmuting electrochemical energy into the mechanical energy vital for the movement of the flagellum.
- Rotor: Comprising the C-ring and its associated proteins, the rotor is tasked with translating the torque, generated by the stator, into rotational motion. This rotation propels the flagellum, enabling the cell to navigate its surroundings.
In essence, the basal body is a marvel of cellular engineering, ensuring the efficient and coordinated movement of the flagellum, thereby facilitating the cell’s locomotion and interaction with its environment.
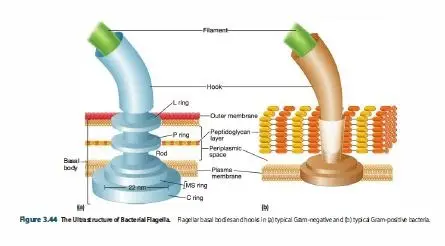
Motor
- The bacterial flagellum contains a rotary engine (Mot complex) at the flagellum’s anchor point on the inner cell membrane. It controls the rotation of flagella.
- It is made up of protein which is known as Mot-protein.
- The proton motive force powered this rotary engine. In proton motive force, the hydrogen ions or protons move across the bacterial cell membrane due to a concentration gradient which is set up by the cell’s metabolism.
- The rotor carries protons crossed the membrane, and is used in the process. The rotor solely can spin at 6,000 to 17,000 rpm, but besides the flagellar filament attached normally only gives 200 to 1000 rpm.
- The direction of rotation can be altered by the flagellar motor switch almost immediately, affected by a slight alteration in the state of a protein, FliG, in the rotor.
- The flagellum uses very little energy, which means it is highly energy-efficient.
- The definite mechanism for torque formation is yet badly understood. Because there are no on-off switch for the flagellar motor, the protein epsE is utilized as a mechanical link to release the motor from the rotor, therefore stopping the flagellum and subtracting the bacterium to settle in one place.
- The rotational velocity of flagella changes in response to the strength of the proton motive force, some bacteria achieve roughly 60 cell lengths per second. At this speed, a bacterium would take about 245 days to cover 1 km.
Bacterial Flagella Functions
Flagella, slender, hair-like structures present in many organisms, play a pivotal role in a myriad of biological processes. Their functions span from basic motility to intricate cellular communication. Here’s a comprehensive overview of the functions of flagella:
- Motility: Flagella primarily serve as locomotive appendages. They enable organisms, especially bacteria, to swim and navigate through their environment. This movement is achieved through a specialized mechanism known as flagellar propulsion.
- Steering and Directional Movement: Organisms with multiple flagella can manipulate their direction by coordinating the rotation of these structures. This allows for intricate movements and the ability to navigate complex environments.
- Sensory Organelles: Beyond movement, flagella have evolved to detect environmental changes. They can sense a range of external factors, including temperature, light intensity, and chemical gradients. This sensory capability allows organisms to adapt and respond to their surroundings effectively.
- Attachment and Colonization: Some bacteria utilize their flagella to adhere to surfaces or host tissues. This adhesive capability can be crucial for bacteria to establish infections or form protective communities known as biofilms.
- Nutrient Acquisition: In specific contexts, flagella aid in nutrient uptake. For photosynthetic organisms, flagella can position the cell optimally to capture sunlight. In bacteria, they can guide the organism towards nutrient-rich zones.
- Gravitaxis: Some microorganisms use flagella to orient themselves in relation to gravitational forces, ensuring optimal positioning in their aquatic habitats.
- Cellular Communication: Flagella can act as communication conduits between cells. Through coordinated movements or the release of signaling molecules, cells can synchronize behaviors or processes.
- Fluid Dynamics: The collective movement of flagella in certain environments can induce fluid flows, facilitating the mixing or transport of substances.
- Cell Locomotion: Beyond simple movement, flagella drive the overall locomotion of many cells, allowing them to traverse diverse environments, from aquatic habitats to viscous mediums like mucus.
- Reproductive Assistance: Flagella are instrumental in the reproductive processes of some organisms. In animals and certain algae, they propel sperm cells, facilitating fertilization.
- Biofilm Formation: Flagella play a role in the early stages of biofilm formation, a protective mode of growth adopted by many bacteria. They help in colonization and distribution within these structures.
- Host Interaction: In the context of pathogenic bacteria, flagella can influence the organism’s virulence, aiding in tissue colonization, cellular invasion, and immune evasion.
- Research and Biotechnological Implications: The intricate workings of flagella have spurred extensive research, enriching our understanding of cellular physiology, microbial ecology, and evolutionary biology. Moreover, insights into flagellar function hold promise for innovative antimicrobial strategies and biotechnological applications.
In summary, flagella are multifunctional organelles, integral to many cellular processes. Their roles extend beyond mere movement, influencing how organisms interact with their environment, each other, and their hosts.
Flagellar Assembly: biosynthesis and regulation
Flagellar biosynthesis
Flagellar biosynthesis is a multifaceted cellular process that orchestrates the assembly and construction of the flagellum, a vital appendage for cellular motility. This intricate procedure is underpinned by a series of meticulously coordinated steps, ensuring the precise formation of the flagellum’s various components.
- Transcription: The initial phase of flagellar biosynthesis commences at the genetic level. Genes responsible for encoding the myriad of flagellar components are transcribed, resulting in the formation of corresponding mRNA molecules.
- Translation: Subsequent to transcription, these mRNA molecules serve as templates for the synthesis of protein subunits. Through the process of translation, flagellin proteins, alongside other structural proteins pivotal for flagellar formation, are produced.
- Filament Assembly: The synthesized flagellin proteins undergo polymerization, adopting a helical configuration. This results in the formation of the filament, the primary structure responsible for the flagellum’s propulsive capabilities.
- Hook Formation: Simultaneously, hook protein subunits are synthesized and coalesce to form the hook. This curved tubular structure serves as a bridge, connecting the filament to the basal body, ensuring seamless transmission of torque.
- Basal Body Assembly: The basal body, a cornerstone of the flagellum, is assembled next. This involves the synthesis and integration of its various components, including the rings, rod, and motor proteins. Once assembled, the basal body firmly anchors itself to the cell membrane, providing stability to the flagellum.
- Export and Secretion: The cell employs specialized protein export mechanisms, such as the type III secretion system, to ensure the efficient transport and secretion of flagellar proteins. This ensures that these proteins are directed to their designated locations, facilitating the assembly process.
- Integration and Maturation: The culmination of flagellar biosynthesis involves the integration of the various components. The filament, hook, and basal bodies are meticulously integrated, undergoing a series of maturation processes. This ensures the formation of a fully functional flagellum, primed for its role in cellular motility.
In conclusion, flagellar biosynthesis is a testament to the precision and complexity of cellular processes. Through a series of well-coordinated steps, cells are equipped with a functional flagellum, underscoring the marvels of biological engineering.
Filament Polymerization
- Filament polymerization is a pivotal cellular mechanism responsible for the construction of the flagellar filament, a primary component essential for bacterial locomotion. This process entails the systematic assembly of protein subunits, culminating in the formation of a helical structure characteristic of the flagellar filament.
- The intricacies of filament polymerization commence at the nascent tip of the flagellum. Here, protein subunits are methodically integrated in a sequential manner. As each subunit is incorporated, the filament undergoes progressive elongation, extending its length and enhancing its structural integrity. This orchestrated assembly not only ensures the formation of a robust helical structure but also underpins the flagellum’s functional capabilities, facilitating efficient bacterial movement.
- In essence, filament polymerization is a testament to the precision inherent in cellular processes, underscoring the meticulous nature of biological assembly and the significance of the flagellar filament in bacterial motility.
Regulatory Mechanisms
The intricate process of flagellar assembly and its subsequent function is underpinned by a series of regulatory mechanisms. These mechanisms are paramount in ensuring the precise formation, operation, and adaptation of flagella in response to environmental stimuli and intracellular conditions.
- Transcriptional Regulation: Central to the genesis of flagellar components is the transcriptional regulation. Specific transcriptional regulators govern the expression of genes associated with flagellar biosynthesis. Depending on the cellular context or external signals, these regulators can either augment or inhibit the transcription of pertinent flagellar genes.
- Post-transcriptional Regulation: Beyond transcription, the post-transcriptional landscape plays a pivotal role in flagellar assembly. This involves RNA-binding proteins and small non-coding RNAs that modulate various facets of flagellar mRNA molecules, from their stability and translation to their processing. Such regulation fine-tunes the synthesis and subsequent assembly of flagellar constituents.
- Chemotaxis Signaling: Chemotaxis, the directed movement of cells in response to chemical gradients, is integral to flagellar function. This mechanism encompasses specific chemotaxis genes and signaling cascades that dictate flagellar motility. By sensing environmental cues, these pathways adeptly modulate the flagellum’s rotation and trajectory, ensuring optimal navigation.
- Protein Secretion and Export Systems: The assembly of a functional flagellum necessitates the transport of its components to their designated locations within the cell. This is facilitated by specialized protein secretion and export systems, such as the type III secretion apparatus in bacteria. These systems are instrumental in ensuring the correct localization and integration of flagellar components.
- Protein-Protein Interactions: The assembly process is further characterized by a myriad of protein-protein interactions. These interactions, which occur at various stages of flagellar assembly, involve specific proteins that collaborate to ensure the proper assembly, stabilization, and anchoring of flagellar components.
In summation, the regulatory mechanisms governing flagellar assembly and function epitomize the complexity and precision of cellular processes. They not only orchestrate the meticulous assembly of the flagellum but also ensure its adaptive and responsive functionality, enabling organisms to adeptly navigate their environment.
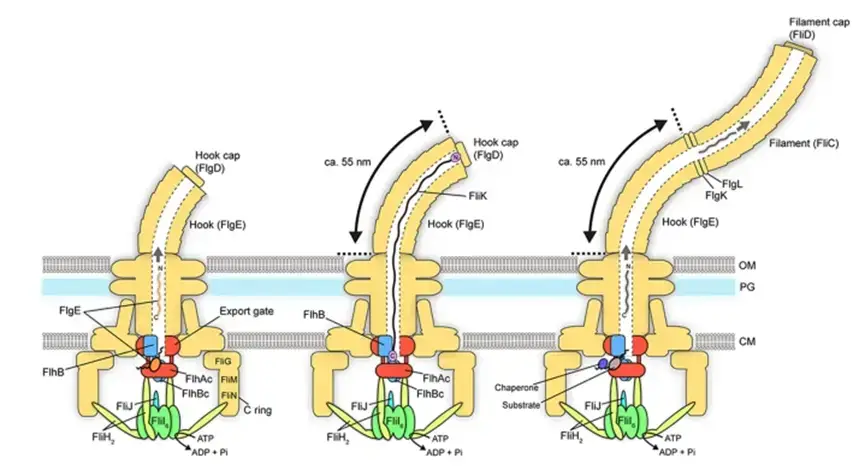
Mechanism of Flagella Formation and Assembly – Overview
Flagella, essential appendages for cellular motility, undergo a meticulously orchestrated formation and assembly process. This process, predominantly studied in bacteria, involves a series of sequential steps, each crucial for the proper construction and functionality of the flagellum.
1. Basal Body Formation: The genesis of flagella assembly is marked by the formation of the basal body, beginning with the integration of the FliF protein into the cytoplasmic membrane. This protein constitutes the MS-ring, a foundational structure that sets the stage for subsequent flagellar assembly.
- MS-ring Assembly: The FliF proteins coalesce to form a singular ring, creating two loops that span the cytoplasmic membrane. This ring acts as a scaffold for the subsequent incorporation of proteins.
- Incorporation of Additional Proteins: Post FliF integration, other pivotal proteins, namely FliG, FliM, and FliN, are embedded into the cytoplasmic face of the MS-ring. These proteins play instrumental roles in flagellar motility and the export of flagellar components.
- C-ring Formation: Progressing inwards, the C-ring emerges in the cytoplasmic space. This structure houses the flagellar export apparatus, designed to transport flagellar proteins through the flagellum’s central channel.
- Rod Assembly: Subsequent to the C-ring’s formation, the rod, a core component of the basal body, is constructed. Comprising five distinct proteins, the rod bridges the FliF ring at its proximal end and the hook at its distal end.
2. Hook Formation: The hook’s formation is a pivotal step in flagellar assembly. This process commences with the translocation of proteins through the rod, culminating in the hook’s growth.
- Initiation by FlgD: The protein FlgD acts as a catalyst for hook formation. Absent in mature flagella, FlgD facilitates the polymerization of subunits into an α-helical configuration.
- Hook Capping: As the hook’s assembly progresses, the rod’s cap protein is supplanted by hook capping proteins. These proteins, specifically FlgE, are transported from the cytoplasm through the flagellum’s central channel, from its base to its tip.
3. Filament Assembly: The final phase of flagellar assembly involves the construction of the filament. This process is orchestrated by the HAP2 pentamer complex, which caps the filament’s distal end.
- Role of the HAP2 Pentamer: This complex is indispensable for filament assembly. It not only prevents the diffusion of subunits from the filament but also induces a conformational shift, facilitating polymerization.
In summation, the formation and assembly of flagella are intricate processes, characterized by a series of well-coordinated steps. Each phase, from the basal body’s formation to the filament’s assembly, underscores the precision inherent in cellular mechanisms, ensuring the proper functionality of the flagellum.
Mechanisms of Flagellum Motility
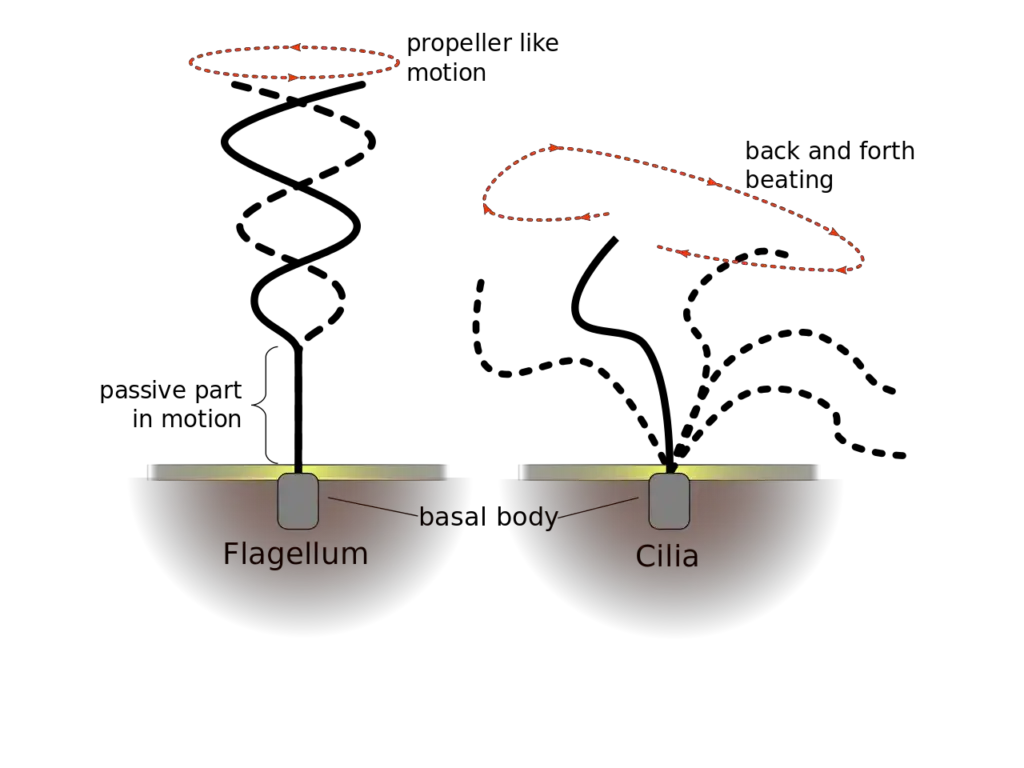
The motility of the flagellum, a whip-like appendage extending from the surface of many cells, is governed by intricate mechanisms that vary based on the organism and flagellar type. These mechanisms are instrumental in propelling cells through their environments, enabling them to navigate and respond to external stimuli.
- Rotational Motion:
- Nature of Movement: Predominantly observed in bacterial and archaeal flagella, rotational motion involves the flagellum acting akin to a propeller, generating thrust that propels the cell. The directionality of this rotation—whether clockwise or counterclockwise—dictates the cell’s movement trajectory, with the former causing a tumbling motion and the latter facilitating linear swimming.
- Regulatory Mechanisms:
- Chemotaxis: This is a phenomenon where bacteria adjust their movement based on chemical gradients in their environment. By detecting specific molecules through sensory receptors, bacteria can modulate the direction of flagellar rotation, enabling them to gravitate towards beneficial conditions or evade detrimental ones.
- Phototaxis: Certain microorganisms, especially those capable of photosynthesis, exhibit phototaxis. Here, light serves as a directive for flagellar rotation. Photoreceptors gauge the intensity and wavelength of light, adjusting the flagellar rotation to optimize light absorption or evade excessive illumination.
- Signal Transduction: Intracellular signaling pathways and interactions between proteins play a pivotal role in steering flagellar rotation. Proteins involved in signaling, such as response regulators, phosphorylate flagellar motor proteins, influencing the speed and direction of rotation.
- Role of Capping Proteins: These proteins are paramount in the regulation and assembly of flagella. They cap the ends of the flagellar filament, halting further growth or disassembly. By modulating filament length, capping proteins ensure the flagellum’s structural and functional integrity.
- Beating Motion:
- Nature of Movement: Eukaryotic cells, particularly their flagella and cilia, exhibit a beating motion. This movement is orchestrated by axonemes, microtubule-based structures within the flagella, which produce a bending motion. This motion either propels the cell or aids in fluid movement around it.
- Mechanism: The beating motion is a result of dynein motor proteins generating sliding forces between adjacent microtubule doublets. This sliding action induces a bending motion, culminating in a synchronized beating pattern.
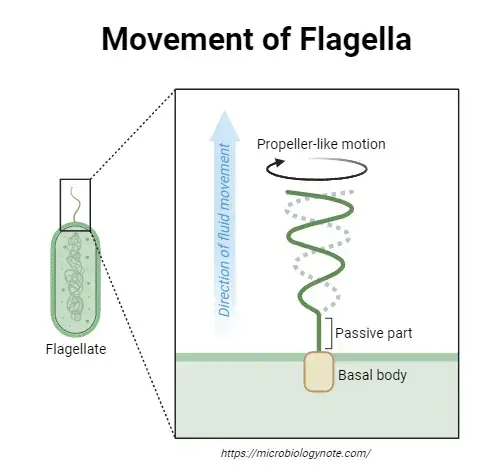
In summary, the flagellum’s motility mechanisms, whether rotational or beating, are intricate processes governed by a myriad of cellular components and regulatory pathways. These mechanisms enable cells to adeptly navigate their surroundings, responding efficiently to environmental cues.
Endoflagella
- The flagella-like organelles which are found in periplasmic space and encased by the outer membrane are known as Endoflagella.
- The endoflagella start at each edge of the organism and wind throughout it, spreading to and overlapping at the midpoint.
- Inside the endoflagella is the interior membrane (cytoplasmic membrane) that provides osmotic balance and includes the protoplasmic cylinder.
Flagella Example
Flagella, intricate cellular appendages, are paramount for the movement and functionality of various organisms. Here are detailed insights into the flagella of specific entities:
1. Helicobacter pylori Flagella
- Structure: Helicobacter pylori possesses approximately 4-8 unipolar flagella.
- Function: These flagella are not merely locomotive structures but are also pivotal virulence factors, instrumental in the onset of diseases instigated by this bacterium.
- Motility: The flagella confer two types of motility to the bacterium: swimming motility, allowing it to traverse liquid mediums, and swarming motility, facilitating movement through semisolid media.
- Physiological Impact: The flagella play a multifaceted role in the bacterium’s physiology, influencing inflammation, immune evasion, and colonization capabilities of H. pylori.
2. Human Sperm Cell Flagellum
- Structure: The flagellum of a human sperm cell is characterized by its core, which is constituted of microtubules arranged in a distinct 9+2 configuration. This core is further adorned with structures such as the dynein regulatory complex, radial spokes, and dynein arms.
- Function: This flagellum is not just a propelling apparatus but is also crucial for successful in vivo fertilization in humans.
- Motility: Any impediment in the flagellum’s movement or its inability to propel the sperm cell can culminate in fertilization failure during human sexual reproduction.
- Reproductive Role: The flagellum is not only responsible for the sperm’s motility but also plays a decisive role during fertilization. It ensures the sperm’s correct orientation, facilitating its penetration into the egg.
In essence, flagella, while serving as primary locomotive structures, also influence various physiological and reproductive processes in different organisms, underscoring their multifunctional nature.
Quiz
What is the primary function of flagella in most bacteria?
a) Reproduction
b) DNA replication
c) Motility
d) Photosynthesis
Which of the following is NOT a part of the bacterial flagellum?
a) Filament
b) Hook
c) Basal body
d) Nucleoid
Which type of flagellar arrangement is characterized by flagella distributed over the entire surface of the bacterial cell?
a) Monotrichous
b) Lophotrichous
c) Amphitrichous
d) Peritrichous
In which organism would you find a flagellum with a 9+2 arrangement of microtubules?
a) Escherichia coli
b) Human sperm cell
c) Helicobacter pylori
d) Archaea
Which protein primarily makes up the bacterial flagellar filament?
a) Tubulin
b) Actin
c) Flagellin
d) Keratin
Which bacterial flagellar component is responsible for generating torque for rotation?
a) Filament
b) Hook
c) Basal body
d) C-ring
In which type of flagellar arrangement is a single flagellum present at both ends of the bacterial cell?
a) Monotrichous
b) Lophotrichous
c) Amphitrichous
d) Peritrichous
Which of the following is NOT a function of flagella?
a) Cell division
b) Sensory perception
c) Attachment to surfaces
d) Nutrient acquisition
Which type of flagellar motion is characterized by a back-and-forth beating pattern?
a) Rotational motion
b) Linear motion
c) Beating motion
d) Vibrational motion
Which of the following bacteria is known for its helical shape and multiple flagella that wrap around its body?
a) Escherichia coli
b) Staphylococcus aureus
c) Helicobacter pylori
d) Bacillus subtilis
FAQ
What are flagella?
Flagella are long, whip-like structures that protrude from the surface of certain types of cells. They are used for locomotion, allowing the cell to move through fluid environments.
What are the different types of flagella?
There are two main types of flagella: bacterial flagella and eukaryotic flagella. Bacterial flagella are simple, helical structures that rotate like a propeller. Eukaryotic flagella are more complex, consisting of a bundle of microtubules surrounded by a plasma membrane.
How do flagella work?
Flagella work by propelling the cell through fluid environments. Bacterial flagella rotate like a propeller, while eukaryotic flagella use a whipping motion to move the cell forward.
What is the structure of flagella?
Flagella consist of several different proteins, including a filament that extends from the surface of the cell, a hook that connects the filament to the cell body, and a basal body that anchors the flagellum to the cell membrane.
What is the function of flagella?
The main function of flagella is locomotion, allowing cells to move through fluid environments. However, flagella may also be involved in other functions, such as sensing the environment and communicating with other cells.
Where are flagella found?
Flagella are found in a variety of organisms, including bacteria, archaea, and eukaryotic cells. In bacteria, flagella are often located at one or both ends of the cell, while in eukaryotic cells they are typically found on the surface of the cell.
How are flagella different from cilia?
Flagella and cilia are both organelles found in cells that are involved in movement, but they have some differences in their structure and function.
Flagella are longer and fewer in number than cilia. They are whip-like structures that extend from the surface of the cell and move in a wave-like pattern, propelling the cell through its environment. Flagella are found in many single-celled organisms, such as bacteria and protozoa, as well as in sperm cells in animals.
Cilia, on the other hand, are shorter and more numerous than flagella. They are hair-like structures that extend from the surface of the cell and move in a coordinated fashion, creating a current that moves substances along the surface of the cell. Cilia are found in many types of cells in animals, such as the cells that line the respiratory tract and the cells that make up the reproductive system.
In summary, flagella are longer and fewer in number, and they move in a wave-like pattern to propel the cell, while cilia are shorter and more numerous, and they move in a coordinated fashion to create a current that moves substances along the surface of the cell.
References
- Aizawa, S.-I. (2015). Flagella. Molecular Medical Microbiology, 125–146. doi:10.1016/b978-0-12-397169-2.00007-x
- Aizawa, S.-I. (2017). Flagella ☆. Reference Module in Life Sciences. doi:10.1016/b978-0-12-809633-8.06434-7
- Nazir, R., Rehman, S., Nisa, M., & Baba, U. ali. (2019). Exploring bacterial diversity. Freshwater Microbiology, 263–306. doi:10.1016/b978-0-12-817495-1.00007-4
- Berry, R. M., & Armitage, J. P. (1999). The Bacterial Flagella Motor. Advances in Microbial Physiology, 291–337. doi:10.1016/s0065-2911(08)60169-1
- Aizawa, S.-I. (2015). Flagella. Molecular Medical Microbiology, 125–146. doi:10.1016/b978-0-12-397169-2.00007-x
- Haiko J, Westerlund-Wikström B. The role of the bacterial flagellum in adhesion and virulence. Biology (Basel). 2013 Oct 25;2(4):1242-67. doi: 10.3390/biology2041242. PMID: 24833223; PMCID: PMC4009794.
- Vonderviszt F, Namba K. Structure, Function and Assembly of Flagellar Axial Proteins. In: Madame Curie Bioscience Database [Internet]. Austin (TX): Landes Bioscience; 2000-2013. Available from: https://www.ncbi.nlm.nih.gov/books/NBK6250/
- Lodish H, Berk A, Zipursky SL, et al. Molecular Cell Biology. 4th edition. New York: W. H. Freeman; 2000. Section 19.4, Cilia and Flagella: Structure and Movement. Available from: https://www.ncbi.nlm.nih.gov/books/NBK21698/
- Nikhil A. Thomas, Sonia L. Bardy, Ken F. Jarrell, The archaeal flagellum: a different kind of prokaryotic motility structure, FEMS Microbiology Reviews, Volume 25, Issue 2, April 2001, Pages 147–174, https://doi.org/10.1111/j.1574-6976.2001.tb00575.x
- Vonderviszt F, Namba K. Structure, Function and Assembly of Flagellar Axial Proteins. In: Madame Curie Bioscience Database [Internet]. Austin (TX): Landes Bioscience; 2000-2013. Available from: https://www.ncbi.nlm.nih.gov/books/NBK6250/
- Samatey, F., Matsunami, H., Imada, K. et al. Structure of the bacterial flagellar hook and implication for the molecular universal joint mechanism. Nature 431, 1062–1068 (2004). https://doi.org/10.1038/nature02997
- Ng SY, Chaban B, Jarrell KF. Archaeal flagella, bacterial flagella and type IV pili: a comparison of genes and posttranslational modifications. J Mol Microbiol Biotechnol. 2006;11(3-5):167-91. doi: 10.1159/000094053. PMID: 16983194.
- Dmitry Apel, Michael G. Surette. Bringing order to a complex molecular machine: The assembly of the bacterial flagella. Biochimica et Biophysica Acta (BBA) – Biomembranes. Volume 1778, Issue 9. 2008. Pages 1851-1858. https://doi.org/10.1016/j.bbamem.2007.07.005.
- Nakamura, Shuichi, and Tohru Minamino. “Flagella-Driven Motility of Bacteria.” Biomolecules vol. 9,7 279. 14 Jul. 2019, doi:10.3390/biom9070279
- Tohru Minamino, Katsumi Imada. The bacterial flagellar motor and its structural diversity. Trends in Microbiology. Volume 23, Issue 5. 2015. Pages 267-274. https://doi.org/10.1016/j.tim.2014.12.011.
- https://www.slideshare.net/amjadkhanafridi4all/flagella-54587839
- https://biologydictionary.net/flagellum/