What is Ligase?
In biochemistry, ligases are a class of enzymes that catalyze the joining of two molecules through the formation of new chemical bonds. This process, known as ligation, typically involves the hydrolysis of a small pendant chemical group from one of the substrates, which facilitates the creation of new bonds, such as C-O, C-S, or C-N linkages. For instance, DNA ligase is a specific type of ligase that joins complementary nucleic acid fragments by forming phosphodiester bonds, effectively repairing single-strand breaks that can occur in double-stranded DNA during replication.
The basic reaction catalyzed by ligases can be represented by the following dehydration reaction:
A-OH+B-H→A-B+H2O
This equation illustrates how the hydroxyl group (–OH) from molecule A and the hydrogen (–H) from molecule B combine to form a covalent bond while releasing water as a byproduct. Therefore, ligases play a crucial role in various biochemical pathways by facilitating the joining of different molecular entities.
The nomenclature for ligases can be inconsistent, as these enzymes are referred to by various names depending on their specific functions. While the term “ligase” is commonly used in names like DNA ligase, other terms such as “synthetase” or “synthase” may also be employed, particularly when the enzyme is involved in synthesizing new molecules. Furthermore, some ligases are designated as “carboxylases,” indicating their role in catalyzing carboxylation reactions. This variability in naming can lead to confusion, but generally, the terms synthetase and synthase are used interchangeably to describe ligases.
Ligases are classified under the Enzyme Commission (EC) number system as EC 6. This classification is divided into several subclasses based on the type of bond they form. Specifically, these subclasses include:
- EC 6.1: Ligases that form carbon-oxygen bonds.
- EC 6.2: Ligases that facilitate the formation of carbon-sulfur bonds.
- EC 6.3: Ligases involved in forming carbon-nitrogen bonds, such as argininosuccinate synthetase.
- EC 6.4: Ligases that create carbon-carbon bonds, exemplified by acetyl-CoA carboxylase.
- EC 6.5: Ligases that form phosphoric ester bonds, including DNA ligase.
- EC 6.6: Ligases that establish nitrogen-metal bonds, as seen in chelatases.
What is DNA Ligase?
- DNA ligase is an essential enzyme that plays a critical role in the integrity and maintenance of DNA within living organisms. Its primary function is to facilitate the joining of DNA strands by catalyzing the formation of phosphodiester bonds, a crucial process in DNA repair, replication, and recombination.
- In the context of DNA repair, DNA ligase addresses single-strand breaks in duplex DNA by utilizing the complementary strand as a template. When a single-strand break occurs, DNA ligase creates the final phosphodiester bond to complete the repair process. This mechanism ensures that the genetic information remains intact and functional. Some specialized forms of DNA ligase, such as DNA ligase IV, are particularly adept at repairing double-strand breaks, where both strands of the DNA helix are disrupted.
- Moreover, DNA ligase is integral to DNA replication. It joins newly synthesized DNA fragments, which are generated by DNA polymerases, ensuring that the newly formed strands are continuous. Specifically, DNA ligase catalyzes the final phosphodiester linkage between the 5′-phosphate group from DNA polymerase III and the 3′-hydroxyl group from DNA polymerase I. This action is vital for maintaining the structural integrity of the replicated DNA.
- The mechanism of action of DNA ligase is energy-dependent. In most organisms, the enzyme requires ATP or NAD+ to facilitate the joining of DNA fragments. ATP-dependent ligases are prevalent in eukaryotic cells, while bacterial, archaeal, and viral ligases may utilize either ATP or NAD+. The energy derived from these molecules is necessary to cleave the phosphate bonds, allowing for the joining of DNA nicks—locations where the phosphodiester linkage between adjacent nucleotides is absent.
- In addition to its natural biological functions, DNA ligase has significant applications in molecular biology. It is widely utilized in laboratory settings for recombinant DNA experiments, such as gene cloning. By purifying DNA ligase, researchers can effectively join DNA molecules, creating recombinant DNA that can be used for various biotechnological and therapeutic purposes.
- The discovery of DNA ligase traces back to 1967 when it was first isolated from Escherichia coli. Subsequent research in the late 1960s led to the identification of various other forms of this enzyme. DNA ligases belong to the nucleotide transferase superfamily, which includes RNA ligases and mRNA capping enzymes. This classification underscores the evolutionary significance of these enzymes in nucleic acid metabolism.
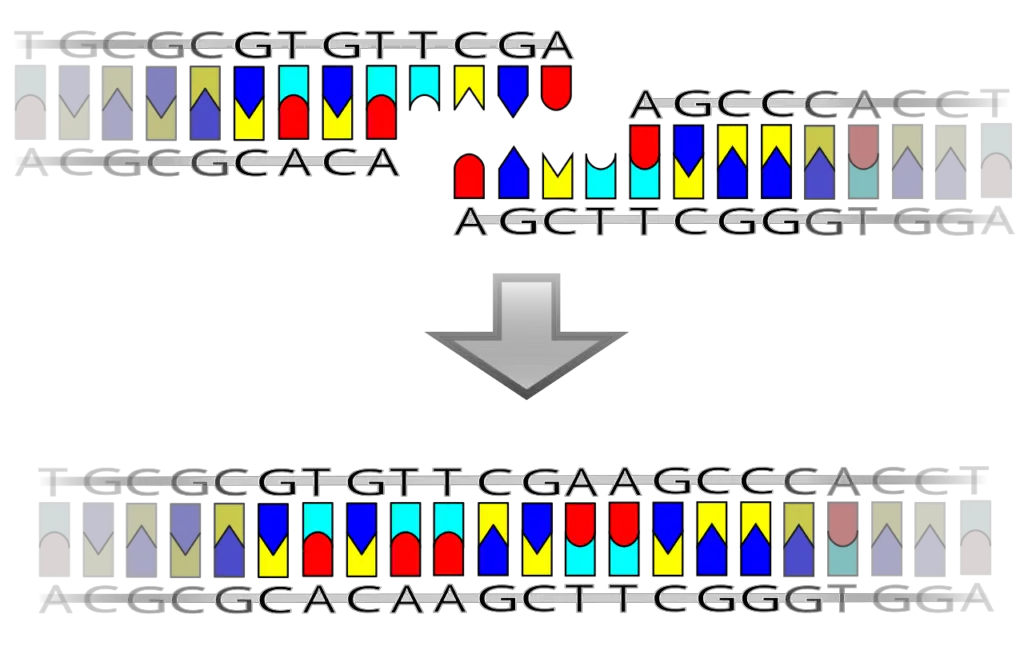
Structure of DNA Ligase
The structure of DNA ligase is essential for its function in catalyzing the joining of DNA strands. Variations in size and composition exist across different organisms, reflecting adaptations to their specific biological contexts. Understanding the structural components of DNA ligase provides insight into its mechanisms of action and overall role in DNA repair and replication.
- Size Variability: DNA ligases vary in size depending on the organism. For instance, viral ATP-dependent DNA ligases tend to be smaller compared to those from other organisms. This size difference may correlate with the specific requirements and efficiencies needed for DNA processing in different biological systems.
- Catalytic Core: Both ATP-dependent and NAD+-dependent DNA ligases possess a conserved catalytic core, which is crucial for their enzymatic activities. This core includes six conserved motifs—designated I, II, III, IV, V, and VI—that are integral for binding cofactors such as ATP or NAD+, coordinating metal ions, and facilitating ligation chemistry.
- Non-Catalytic Domain: Many DNA ligases also feature a conserved non-catalytic domain, the exact function of which remains unclear. This domain may play a role in the stability or regulation of the ligase, although further research is needed to elucidate its specific contributions.
- Structure of ATP-Dependent Ligases:
- Domain Composition: ATP-dependent ligases, exemplified by those derived from bacteriophage T7, are typically organized into two distinct domains. The first is the adenylation or nucleotide-binding domain, which includes a pocket beneath one of the β-sheets, serving as the binding site for ATP.
- Domain 1 Structure: This domain comprises three antiparallel β-sheets flanked by six α-helices, forming a stable framework for ATP interaction.
- Oligonucleotide Binding Fold Domain: The second domain consists of an oligonucleotide binding fold, characterized by a highly twisted antiparallel β-sheet accompanied by a single α-helix running along one edge.
- Linker Region: A flexible linker connects these two domains, allowing for dynamic interactions during the ligation process.
- Structure of NAD+-Dependent Ligases:
- Catalytic Core Composition: NAD+-dependent ligases, such as E. coli LigA, contain a central ligase catalytic core that integrates the oligonucleotide binding fold domain with a nucleotidyltransferase domain.
- Domain Organization: These domains are positioned at the edge of the N-terminal domain (referred to as domain la) and are associated with three C-terminal domains, which include a helix-hairpin-helix (HhH) domain, a zinc finger domain, and a BRTC domain.
- Role of Domain la: This domain is crucial for the reaction of the ligase with NAD+, leading to the formation of a ligase-AMP intermediate, a key step in the ligation process.
Types of DNA Ligase
DNA ligases are enzymes critical for DNA replication and repair, classified primarily based on their cofactor requirements: ATP-dependent and NAD+-dependent DNA ligases. Understanding these types is essential for appreciating their diverse roles in different organisms, including bacteria, archaea, and eukaryotes.
- ATP-Dependent DNA Ligases: Most eukaryotic DNA ligases utilize ATP as a cofactor. These ligases exhibit variability in size and structure across different organisms. For example:
- Size Variation: The DNA ligase from Haemophilus influenzae comprises 268 amino acids, whereas larger cellular ligases, such as human DNA ligase I, consist of 912 amino acids, and ligase IV contains 844 amino acids.
- Bacteriophage T7: This ligase is the most widely used variant, characterized by its monomeric structure with a molecular weight of 41 kDa. It features two distinct domains. Domain 1 is responsible for ATP binding, composed of six α-helices surrounding three antiparallel β-sheets. Domain 2 consists of antiparallel β-sheets with an accompanying α-helix.
- Bacteriophage T4: This ligase can ligate cohesive ends or blunt ends of DNA, RNA-DNA hybrids, and RNA. It exists as a monomeric form with a molecular weight of 68 kDa.
- Mammalian DNA Ligases:
- DNA Ligase I: Functions primarily to ligate Okazaki fragments during lagging strand replication and assists in joining some recombinant fragments.
- DNA Ligase II: Involved in the purification or excision repair of alternatively spliced forms of DNA ligase III, typically found in non-dividing cells.
- DNA Ligase III: The only mammalian ligase located in mitochondria, it plays a role in nucleotide excision repair and the ligation of recombinant fragments.
- DNA Ligase IV: Crucial for homologous recombination repair, this ligase is important for immune cell development and other vital cellular activities.
- NAD+-Dependent DNA Ligases: These ligases are predominantly found in bacterial species but also exist in some mimivirus and entomovirus. Additionally, some bacteria, archaea, and viruses may utilize both NAD+ and ATP for their ligases. For example, Mycobacterium tuberculosis codes for NAD+-dependent ligases and has at least three types of ATP-dependent ligases. The size of these ligases tends to be uniform, ranging from 656 to 837 amino acids. Notable examples include:
- E. coli DNA Ligases: This monomeric enzyme has a molecular weight of 74 kDa and requires NAD+ as a cofactor. It catalyzes the formation of phosphodiester bonds in double-stranded DNA containing cohesive ends. The ligases LigA and LigB, products of the lig gene, are predominantly utilized in E. coli.
- Taq DNA Ligases: Derived from thermophilic bacteria, Taq ligases are thermostable and require NAD+ as a cofactor. They demonstrate higher stability and activity at extreme temperatures compared to conventional DNA ligases, making them valuable in DNA amplification reactions for detecting mutations in mammalian DNA.
Mechanism of DNA ligase
The mechanism of DNA ligase is a critical process in DNA metabolism, enabling the joining of DNA fragments through the formation of phosphodiester bonds. This enzyme catalyzes the repair of single-strand breaks and plays a pivotal role in DNA replication. The mechanism generally unfolds in three main steps, each essential for the successful ligation of DNA.
- Adenylation of DNA Ligase/Activation of DNA Ligase: The initial step involves the activation of DNA ligase by ATP. In this process, ATP is hydrolyzed to adenosine monophosphate (AMP), releasing energy. This AMP is subsequently attached to the amino group of a lysine residue located at the enzyme’s conserved active site. This attachment occurs via a phosphoramidite bond, resulting in the formation of an enzyme-AMP complex, which is a crucial intermediate in the ligation process.
- Activation of 5’ Phosphate in Nicks: Following the formation of the enzyme-AMP complex, the activated ligase is transferred to the 5’ phosphate group at the site of the DNA break, commonly referred to as a “nick.” During this interaction, the AMP moiety engages with the phosphate group, facilitating the release of the ligase enzyme from the complex. This interaction effectively activates the 5’ end of the single-stranded DNA, setting the stage for the ligation reaction.
- DNA Ligation: In the final step, the activated 5’ phosphate group is subjected to nucleophilic attack by the 3’ hydroxyl group of an adjacent DNA strand. This attack leads to the formation of a covalent phosphodiester bond between the two nucleotides, effectively sealing the break in the DNA strand. The reaction results in the release of free AMP and hydrogen ions, completing the ligation process.
It is noteworthy that in bacterial systems, the ligase may be adenylated by NADH2, which is cleaved to produce nicotinamide mononucleotide. This variation highlights the diversity in ligation mechanisms across different organisms, although the core principles remain consistent.
DNA Ligation Reactions
DNA ligation reactions are fundamental processes in molecular biology, enabling the joining of DNA fragments to form recombinant DNA molecules. These reactions utilize specific ends created by restriction enzymes, leading to two primary types of ligation: blunt-end ligation and sticky-end ligation. Each method has distinct mechanisms and efficiencies, which are crucial for various genetic engineering applications.
- Blunt-End Ligation:
In this reaction, both the plasmid vector and the DNA insert are cut by restriction enzymes to create blunt ends. Blunt ends are flat, with no overhanging nucleotides, allowing for the direct joining of the fragments. DNA ligase facilitates the formation of a phosphodiester bond between these blunt ends. Although this method can successfully ligate fragments, it is generally less efficient than sticky-end ligation due to the lack of complementary overhangs that help stabilize the binding of the fragments prior to ligation. - Sticky-End Ligation:
This method involves a more complex interaction. Restriction enzymes cleave the plasmid vector and the DNA insert at two different positions, resulting in short, single-stranded overhangs at the 3’ and 5’ ends of the nucleic acid fragments. These overhangs are known as sticky ends and must be complementary for successful ligation. The presence of complementary sticky ends enhances the efficiency of the reaction because the overhangs can base-pair with one another, providing a stable initial interaction before the DNA ligase catalyzes the formation of the phosphodiester bond. This increased stability contributes to a higher likelihood of successful ligation, making sticky-end ligation a preferred choice in many cloning procedures.
Functions of DNA Ligase
Below are the primary functions of DNA ligase, highlighting its importance in cellular processes and biotechnology.
- DNA Replication: DNA ligase is integral to the DNA replication process, particularly during the synthesis of the lagging strand. It joins Okazaki fragments by forming phosphodiester bonds between the nicks left by DNA polymerase. This action ensures the continuity of the DNA strand, allowing for complete and accurate duplication of genetic material.
- DNA Repair: The enzyme is vital for repairing single-strand breaks in DNA. DNA ligase facilitates the rejoining of fragmented DNA strands that may arise from environmental damage, replication errors, or other cellular stresses. By sealing these nicks, the ligase helps maintain genomic stability and prevents the accumulation of mutations.
- Recombinant DNA Technology: DNA ligases are indispensable in the field of genetic engineering, particularly in the construction of recombinant DNA molecules. They are utilized alongside restriction endonucleases to insert foreign DNA fragments, such as genes, into plasmids. This process is critical for gene cloning, allowing researchers to create genetically modified organisms or produce specific proteins for research and therapeutic purposes.
- High-Efficiency Cloning: In molecular biology labs, E. coli DNA ligases are often employed for high-efficiency cloning of full-length complementary DNA (cDNA). Their ability to effectively ligate DNA fragments makes them valuable tools for constructing cDNA libraries and expressing eukaryotic genes in prokaryotic systems.
- Thermostable Applications: Commercially available thermostable Taq ligases are utilized in polymerase chain reactions (PCR) due to their ability to withstand high temperatures. This stability makes them suitable for amplification reactions, enhancing the efficiency and specificity of DNA amplification processes.
- V(D)J Recombination: In human cells, DNA ligase IV is crucial for V(D)J recombination, a process that generates diversity in immunoglobulin and T-cell receptor loci during the development of the immune system. This function is essential for producing a wide array of antibodies and T-cell receptors, allowing for a robust adaptive immune response.
How does DNA ligase work?
Understanding how DNA ligase functions is crucial for grasping the mechanisms of DNA replication, repair, and recombinant DNA technology. DNA ligase facilitates the joining of DNA fragments by forming covalent phosphodiester bonds. The process can be divided into three distinct steps, illustrating the enzyme’s action in catalyzing the ligation reaction.
- Self-Adenylation of DNA Ligase:
The process begins with the activation of DNA ligase. The enzyme reacts with either ATP or NAD+, resulting in the formation of a phosphamide bond. This bond is established between a lysine residue within the enzyme and an adenosine monophosphate (AMP) molecule derived from the cofactor. This step is crucial as it prepares the ligase for subsequent interactions with the DNA strands. - Transfer of the Adenyl Group:
Once the DNA ligase is activated and the AMP is attached, the next step involves the transfer of the adenyl group to the 5’ phosphorylated end of the donor DNA strand. This modification is essential because it activates the 5’ end, making it more reactive and primed for ligation. - Formation of the Phosphodiester Bond:
The final step in the mechanism occurs when the hydroxyl group of the acceptor DNA strand attacks the adenylated 5’ end of the donor DNA. This reaction results in the formation of a covalent phosphodiester bond, effectively sealing the nick in the DNA strand. The completion of this reaction releases AMP, allowing the ligase to continue functioning and participate in further ligation events.
Therefore, the action of DNA ligase is integral to maintaining the integrity of the DNA molecule by enabling the repair of nicks and gaps. The enzyme’s ability to facilitate the formation of phosphodiester bonds is essential for various biological processes, including DNA replication and repair, making it a vital component in molecular biology and genetic engineering.
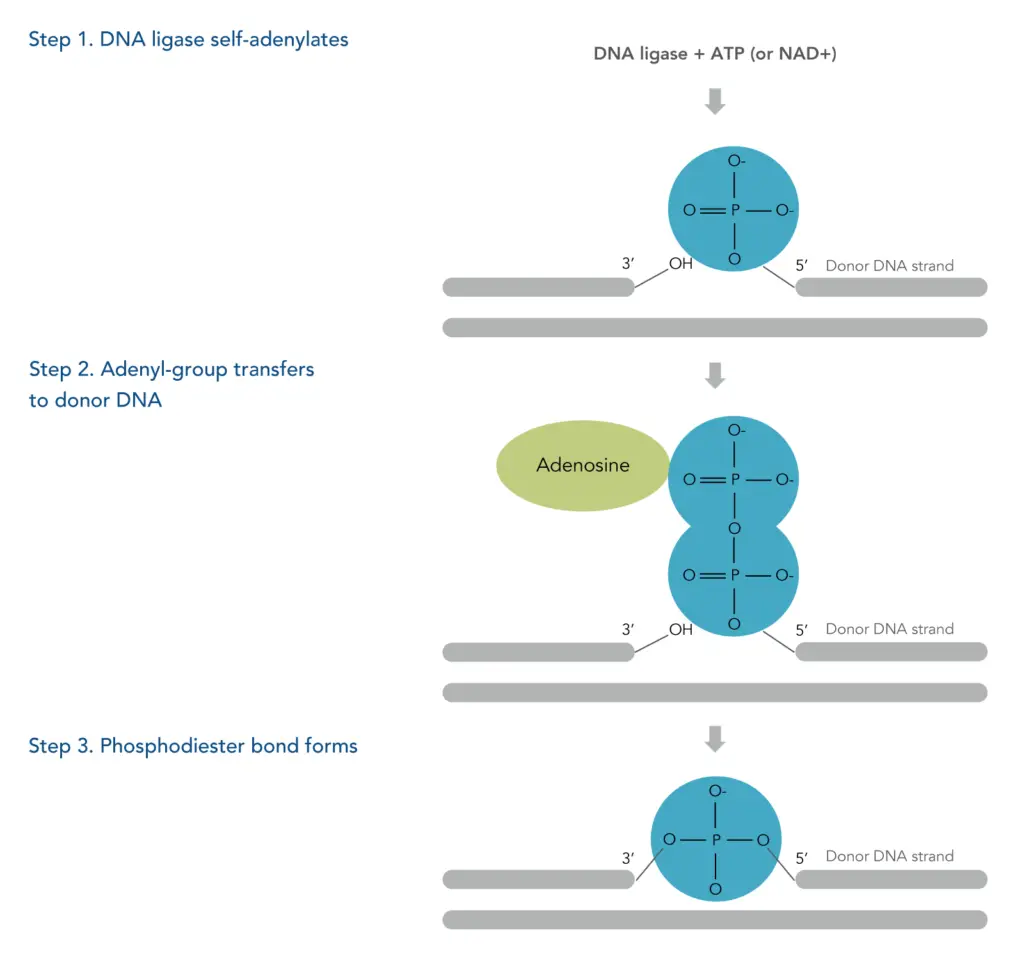
Disorders of DNA ligase
The following points outline some notable disorders linked to dysfunctional DNA ligases:
- LIG4 Syndrome:
LIG4 syndrome, also known as Ligase IV syndrome, arises from mutations in the DNA ligase 4 gene. This condition disrupts the repair of double-stranded DNA breaks, resulting in significant immunodeficiency in affected individuals. Patients with this syndrome often exhibit developmental abnormalities, including microcephaly and bone marrow hypoplasia, which can severely impact overall health and growth. - Xeroderma Pigmentosum (XP):
Xeroderma pigmentosum is an inherited disorder characterized by extreme sensitivity to ultraviolet (UV) radiation. Individuals with XP are particularly vulnerable to skin damage and the development of skin cancers due to impaired DNA repair mechanisms following UV exposure. This condition predominantly affects the skin and eyes, with some patients experiencing neurological complications as well. - Ataxia-Telangiectasia:
Ataxia-telangiectasia is caused by mutations in the ATM gene, which plays a crucial role in controlling cell division and facilitating DNA repair. This disorder is characterized by progressive difficulties with movement, balance issues, and neurological symptoms, including slurred speech and oculomotor apraxia. Individuals often require wheelchair assistance by adolescence due to the severity of their motor difficulties. - Fanconi Anemia (FA):
Fanconi anemia is a rare genetic blood disorder leading to bone marrow failure. This condition results in insufficient production of healthy blood cells, ultimately causing severe health issues, including an increased risk of leukemia. The disorder reflects a failure in the DNA repair pathways that are essential for maintaining genetic stability in hematopoietic cells. - Bloom Syndrome:
Bloom syndrome is characterized by a heightened sensitivity to sunlight, resulting in a distinctive butterfly-shaped rash across the nose and cheeks, as well as other skin manifestations. Patients may also develop telangiectases and experience pigmentation changes on non-exposed skin areas. The disorder is linked to defects in DNA repair mechanisms, which can lead to an increased risk of cancer.
- Eun, H.-M. (1996). Ligases. Enzymology Primer for Recombinant DNA Technology, 109–144. doi:10.1016/b978-012243740-3/50005-3
- Shuman S. DNA ligases: progress and prospects. J Biol Chem. 2009 Jun 26;284(26):17365-9. doi: 10.1074/jbc.R900017200. Epub 2009 Mar 27. PMID: 19329793; PMCID: PMC2719376.
- Doherty AJ, Suh SW. Structural and mechanistic conservation in DNA ligases. Nucleic Acids Res. 2000 Nov 1;28(21):4051-8. doi: 10.1093/nar/28.21.4051. PMID: 11058099; PMCID: PMC113121.
- https://www.khanacademy.org/science/biology/biotech-dna-technology/dna-cloning-tutorial/a/restriction-enzymes-dna-ligase
- https://byjus.com/neet/dna-ligase/
- https://www.cell.com/molecular-cell/fulltext/S1097-2765(07)00144-X
- https://www.eurekaselect.com/article/9441
- https://lifesciences.danaher.com/us/en/library/unveiling-dna-ligation.html
- https://study.com/academy/lesson/dna-ligase-definition-role-quiz.html
- https://www.idtdna.com/pages/education/decoded/article/how-dna-ligation-works
- https://www.sciencedirect.com/topics/biochemistry-genetics-and-molecular-biology/dna-ligase