- Salts and gases are dissolved in natural waters, especially sea and mineral waters.
- 70% of the earth’s surface is covered by water, making it the most vital habitat for life.
- It is estimated that the total volume of inland waters is 7,5 x 105 km3, that of seas and oceans is 1,4 x 109 km3, and that of glaciers and continental glaciers is 1,8 x 107 km.
- Water is the most essential component of living beings (70-90 percent of cell mass) and participates in several biological reactions and processes.
Types of Waters Inhabited by Microorganisms
Biotopes of water microorganisms may include subterranean and/or surface waters, as well as sediments on the ocean floor.
- Due to their oligotrophic (nutrient-deficient) nature, underground waters (mineral and thermal springs, ground waters) are often inhabited by a microflora consisting of a small number of species and essentially no higher plants or animals.
- The flora and fauna of surface waters such as streams, rivers, lakes, and the ocean are diverse. The microorganisms in these waters are quite diverse. In addition to the conventional aquatic organisms, additional bacteria from soil habitats and sewage resulting from human and industrial contamination are also present.
- Bottom sediments are a transient form of habitat, i.e. the soil-water habitat, which is nearly always oxygen-free and in which microorganisms’ anaerobic decomposition activities emit hydrogen sulphide and methane into the water. Anaerobic putrefying microflora, cellulolytic bacteria, and anaerobic chemoautotrophs develop in the bottom sediment.
Microorganisms of Aquatic Environment
Microorganisms inhabit surface waters in all zones; they may be suspended (plankton), cover fixed submerged objects, plants, etc. (periphyton), or reside in sediments at the bottom (benthos).
1. Plankton
Plankton or bioseston refers to the group of organisms that passively float in water without the ability to resist the movement and flow of water mass. These are classified as follows:
a. Phytoplankton
- The majority of phytoplankton are microscopic algae and blue-green algae. It is a diverse community in terms of systematics and is predominantly made up of organisms less than 50 m.
- Diatoms and dinophyta dominate sea phytoplankton, whereas cryptophytes, diatoms, green algae, and blue-green algae dominate fresh water phytoplankton.
b. Zooplankton
- Zooplankton are tiny aquatic organisms found in plankton.
- In fresh waters, there are three systematic groups: rotifers, branchiopods, and copepods.
- The plankton of sea water consists of copepods, ctenophores, urochordata, arrow-worms, and several species of snails. The majority are either filtrators (which concentrate suspended particles) or predators.
c. Protozoa plankton
- Protozoa plankton consists of protozoa such as flagellates and ciliates that inhabit open water zones.
- They are the primary bacteria consumers.
- In addition, the majority of ciliates consume flagellates, algae, and smaller ciliates.
- The protozoa feed the zooplankton directly.
d. The heterotrophic bacteria plankton
- Plankton comprised of heterotrophic bacteria inhabits waters rich in organic substances.
- The bacterial concentration in open water varies between 105 and 107 cells/ml.
e. Virus plankton
- Virus plankton is comprised of viruses, which constitute plankton’s tiniest component.
- In a variety of fresh and saltwater settings, their numbers can be quite high (up to 108 in 1ml).
- In addition to protozoa, viruses are a key component in bacterial death.
2. Periphyton
- Periphyton inhabits coastal zones. They are a collection of creatures that attach themselves to various objects and aquatic plants.
- Typically, they consist of microscopic algae, such as diatoms, green algae, and bacteria.
- In addition, several established or semi-settled protozoa, eelworm swarms, oligochaetes, insect larvae, and even crustaceans comprise the periphyton biocenosis.
- Periphyton’s biocenosis is characterised by its complexity, and numerous ecological interactions can be seen between its constituents.
3. Benthos
- The benthos are a collection of species that inhabit the bottom environment.
- The mucky bottom includes an abundance of organic chemicals produced by decomposition of dead materials (fallen parts of plants and animals).
- At deep depths, there are no plants because they cannot develop in the absence of light. However, the absence of oxygen promotes the growth of a putrid microbiota, among others.
- Most abundant among the benthos microflora are bacteria and fungus (decomposers) as well as a few mammals (detritophages).
- Both of the aforementioned groups are responsible for the breakdown of biological materials. The benthic layer of shallow reservoirs may also contain algae.
4. Bacteria
- Bacteria, some of the smallest and oldest organisms on the planet, are prevalent in all water systems and practically every environment. Many bacteria wash into rivers and streams from the surrounding land, and their numbers can grow substantially after a rainfall. Bacteria are frequently present in the millions per millilitre (mL), and in the hundreds of millions per millilitre in waterways that are particularly productive or contaminated.
- When conditions are favourable, bacteria reproduce exceedingly rapidly through simple division, resulting in a rapid increase in population.
- As part of the biofilm, bacteria can be found suspended in water, connected with decaying matter (such as dead wood or leaves), or covering the surface of rocks, stones, and sand grains (the slippery coating on hard surfaces in rivers). They can constitute a significant portion of the living matter in aquatic systems.
- The metabolic capabilities of bacteria are the most diverse of any group of species. Both heterotrophic and autotrophic microorganisms exist.
- In aquatic systems, heterotrophic bacteria play a significant role in the breakdown of organic materials and the cycling of nutrients.
- Autotrophic bacteria and real algae are both primary producers in aquatic systems. Consequently, autotrophic bacteria (mostly cyanobacteria) are sometimes classified as “algae,” despite the fact that the organisms are not closely related.
- Previously, cyanobacteria were incorrectly referred to as “blue-green algae.” Much of what applies ecologically to algae also applies to autotrophic bacteria.
5. Fungi
- Fungi consist of solitary cells and filaments known as hyphae. The majority of aquatic fungi are tiny, with hyphomycetes being the most abundant and significant.
- As with heterotrophic bacteria, fungus receive their nutrition by secreting exoenzymes into their immediate surroundings, which degrade complex chemicals into simpler substances that they can absorb.
- Fungi play a crucial role in the decomposition of plant matter in aquatic systems, as they are among the only creatures capable of decomposing plant structural components such as cellulose and lignin.
6. Protozoa
- Protozoa are small, unicellular organisms that occasionally form colonies.
- Protozoa come in both autotrophic and heterotrophic varieties. In contrast to bacteria and fungi, heterotrophic protozoa (such as amoebas and Paramecium) devour other species, including algae, bacteria, and other protists.
- Protozoa, together with other microbes, compose the biofilm that coats riverbed sediments and hard surfaces, but some protozoa are free-swimming. Certain parasitic protozoa cause diseases such as giardia (beaver fever).
7. Algae
- Several types of predominantly autotrophic protists are known as algae. As with the term “microorganisms,” it is a colloquial phrase used for convenience to refer to microorganisms that carry out photosynthesis; cyanobacteria are frequently categorised as algae.
- Algae range in size from microscopic to colonies so vast that they are classified as macrophytes. Multiple forms of algae, particularly phytoplankton, play a crucial role in giving energy to the base of many aquatic food webs.
Factors Affecting Growth of Microorganisms in Water
The development of microorganisms in water is governed by a multitude of chemical and physical elements that interact or oppose one another in diverse ways. They impact the size, species, and composition of the microbial biocenosis, as well as the appearance and life activities of microorganisms. Within water ecosystems, there are two kinds of elements that have a significant impact on the quantitative and qualitative connections among microorganisms:
- Abiotic factors: Light and thermal energy, water reaction, water flow, climate, and the substances dissolved and suspended in water are examples of abiotic variables (dead organic matter, non-organic compounds and gases such as oxygen, carbon dioxide, methane and others).
- Biotic factors: Biotic factors are all water-dwelling creatures, including plants, animals, and bacteria, and their relationships.
A. Abiotic factors
1. Light energy
- Light plays a crucial function in the photosynthetic process. The amount of light that penetrates different layers of water varies on the sun’s location, the water’s transparency, colour, and depth.
- The narrower the incidence angle, the less solar rays are lost owing to reflection.
- Depending on the level of insolation and water turbidity, the biologically active rays of the sun often penetrate water between 10 and 100 metres.
- Unquestionably, sea water is clearer and less polluted than inland water, therefore light can reach these waters far deeper. Sun rays penetrate seawater to a depth of approximately 150 metres, forming the so-called photic zone where photosynthesis occurs.
- Due to varying light conditions, photoautotroph growth is not uniform over the entire water body.
- The indicator of illumination level is frequently the lower limit (limit) of algae occurrence — their highest development occurs at a depth of 0.5-2 metres.
- The majority of algae have the ability to alter and adapt their colour to the light conditions. Without pigments, bacteria are susceptible to phototoxicity.
- Both the UV and the longer wavelength may be harmful. For example, blue light (wavelength 366-436 nm) suppresses Nitrobacter vinogradskyi’s nitrite oxidation pathway.
- Light also influences the development of water fungus. Red rays have a higher impact than blue and green rays.
2. Temperature
- Similar to light energy, the amount of heat energy depends on the incidence angle (the position of the sun in relation to the water surface).
- Consequently, it varies with the time of day, the seasons, and the latitude. Due to regular mixing by the water flow, the temperature of lotic waterways such as rivers is uniform throughout their mass.
- However, this ecosystem is characterised by daily temperature swings, particularly in rivers with modest depths. In lentic (stagnant) waters such as lakes, where the water movement is very weak or nonexistent, the annual cycle of temperature fluctuation is observed.
- Lakes, particularly deep ones, are distinguished by vertical stratification (the formation of layers that vary according to their composition and temperature).
- Warm, near-surface waters that are illuminated have a lower density than cold, deep waters.
- The difference in density prevents the layers from combining. The layer of warm water is known as the epilimnion.
- With increasing depth, the cooler layers from below form a thermocline or metalimnion and become cooler.
- The temperature decreases by 1°C per metre. The water in the hypolimnion has a temperature of 4°C and the maximum density.
- The thermocline functions as a dividing line between the epi- and hypolimnion. Different densities prevent the top waters from mixing throughout the year.
- Only wind may move water within the epilimnion layer. The biogenes found towards the bottom are inaccessible to the organisms dwelling in the upper layers; hence, the upper layer lacks trophic ingredients in late summer.
- In autumn, the surface waters begin to cool and gradually sink, pushing the warmer waters upwards, where they also cool.
- As the waters continue to interchange (autumn circulation) and are stirred by the wind, they oxygenate and release CO2 into the atmosphere, particularly from the bottom waters.
- In the winter, a little inversion of temperature occurs because water below 4°C has a lower density than water at 4°C and rises to the surface.
- As the surface waters warm in the spring, there is a shift in circulation. The entire body of water is then abundant in oxygen and biogens. The mixing of water also affects the movement of organisms.
3. Water movement
The mixing of water is crucial for both the temperature distribution and the chemical composition equilibrium (gasses, nutrients, substances that equalise the osmotic pressure, water pH etc.). Variations in density produced by varying temperatures and concentrations of soluble. suspended chemicals. winds. differences in the levels at the bottom of a body of water (lotic waters). specialised methods of hydraulic engineering.
4. Pressure
- Pressure is an important ecological component that greatly affects the lives of microorganisms by altering the activity of their enzyme systems, among other mechanisms.
- In water, the hydrostatic pressure increases at a rate of 1 atm every 10 metres of depth. Thus, the pressure is fairly high in vast oceans and some deep lakes; in most seas, it is approximately 100 atm, and in some Pacific trenches, it can even reach 1100 atm.
- The group of bacteria found at depths of 10,000 metres is known as barophilic. They grow and develop not only under great pressures, but also at extremely low temperatures (3 – 5°C), albeit at a very slow rate.
- When the pressure exceeds 200 atm, most freshwater and soil bacteria do not develop (barophobic microorganisms).
5. pH of water
- The ideal pH range for bacteria in water is between 6.5 and 8.5. Most lakes have a pH of 7.0, rivers – 7.5, while the surface layer of the oceans has a pH of 8.2.
- Due to the high carbonate content and buffering characteristics of carbonates, the pH of water does not typically change dramatically.
- But when photosynthesising organisms proliferate rapidly, the pH can climb significantly. Some mineral springs and inland waters with a high humus component concentration may be acidic.
- Under such conditions, acidophilic fungi proliferate. In eutrophic lakes, where the pH ranges from 7 to 10, it is possible to see relatively substantial pH fluctuations, which have a noticeable effect on the populations of bacteria and fungi.
6. Salinity
- Most microorganisms that inhabit clean rivers and lakes are halophobic and cannot survive in waters with a salinity greater than 10% under natural conditions.
- There are not many halophilic species that can grow in saltier water. Due to salinity, sea is regarded as a different (unique) biotope; the majority of halophilic bacteria and fungus inhabit oceans.
- Their life processes depend on a certain concentration of NaCl; hence, the majority of species that inhabit these environments cannot thrive in any other environment.
- The majority of salt is made up of the following elements: Cl, Na, S, Mg, Ca, and K (99%). The average proportion of salt in sea water is 35%.
- Between 25 and 40% is the ideal salinity range for the majority of halophilic bacteria and fungi. In the oceans, the range is between 32 and 38%, while in closed seas (salty lakes), it is much greater.
- For example, the Caspian Sea includes a modest concentration of salt (1.1% to 1.3%), whereas the Dead Sea contains up to 28% salt.
- An rise in salinity affects the production cycle and morphological and physiological characteristics of bacteria and fungi.
- Lakes with a high concentration of salts are extreme biotopes with little species diversity among their biotic categories (the main microorganisms are the bacteria, blue-green algae, flagellates).
7. Other non-organic substances
- In addition to NaCl, phosphorus and nitrogen molecules play a significant role in the life cycle of water microorganisms.
- In addition to free nitrogen, surface waters include several mineral complexes of this element, such as nitrates, nitrites, and ammonium salts. Most frequently, algae and heterotrophic bacteria utilise nitrates and ammonium salts.
- The maximum levels of nitrogen that various kinds of algae can tolerate vary. For example, diatoms (such as Asterionella) may proliferate at high concentrations – even at 100 g N/l – but the maximal level for Pediastrum algae is only 2 g N/l.
- It is the same for bacteria, however the maximum amount varies between species. Phosphorus is the most essential ingredient that inhibits the growth of algae. Its concentration in water is quite modest (0.01-0.1 mg P2O5 per litre).
- Mineral phosphorus is found in water in diluted forms (orthophosphate) and as insoluble salts (calcium phosphate, magnesium phosphate, etc.). Phosphorus may be stored in algae’s cells in excess of their requirements.
- Water blooming is caused by an increase in phosphate concentration due to the inflow of contaminants.
- In oligotrophic lakes and nutrient-deficient seas, it is difficult to identify the presence of ammonium ions, nitrites, nitrates, and phosphates because phytoplankton consumes these components quickly after their formation. In the photic zones of many tropical waters, shortages in nitrogen and phosphorus compounds persist year-round, whereas in temperate zones, these deficiencies fluctuate seasonally.
- In contrast, heterotrophic microorganisms are responsible for the accumulation of nitrates and phosphates in the deep waters of certain major lakes and seas.
- Ammonium ions and nitrites are the energy substrates for nitrification bacteria, but a variety of denitrifying bacteria can use oxygen mixed in nitrates to oxidise organic molecules under anaerobic circumstances.
- Other life-essential salts include S, Mg, Ca, K, Fe, and Si compounds. Microorganisms utilise them to construct cell structures and to activate enzymes.
8. Gases-In water reservoirs
- In addition to minerals and organic compounds, minor volumes of diluted gas can be discovered in water reservoirs.
- Water has the ability to dilute gases, but the solubility decreases with increasing temperature and salinity; it is lower in sea water basins than in fresh water basins. It focuses mostly on oxygen, carbon dioxide, and nitrogen.
- The atmosphere is the primary source of the aforementioned gases, which seep into the higher layers of water until saturation is reached.
- Moreover, biological processes may produce gases diluted in water and sediments.
- Green plants release oxygen as a result of photosynthesis, carbon dioxide during respiration, free nitrogen during denitrification, hydrogen sulphide during desulfurization, and hydrocarbons during fermentation.
9. Organic substances
- Either live cells or their autolysis produce organic compounds. However, sewage is the major contributor of organic molecules to water.
- In water, organic molecules exist as solutions or as suspended particles. They serve as food for heterotrophic bacteria and fungus initially.
- Beneficial feeding circumstances exist for microorganisms that frequently occur on the surface of suspensions, particularly on particles of detritus that absorb organic compounds from water.
- The number of readily available organic compounds (such as carbohydrates, organic acids, proteins, and lipids) has a greater influence on the development and metabolic alterations of microorganisms than the total amount of organic substances.
- Their water supply depletes pretty rapidly. Lack of organic molecules prevents bacteria from reaching their correct size and slows their cell division.
10. Trophicity of surface waters
- Trophicity is the amount of biogenic components and simple chemical molecules soluble in water.
- Trophicity determines the rate of primary production and biomass size. Important indications of water trophicity include phosphate and nitrogen concentration, chlorophyll concentration, water clarity, and oxygen conditions near the water’s bottom.
- Based on the trophicity of the water, the following types of water reservoirs can be identified: oligotrophic (low nutrient concentration), mesotrophic (mid nutrient concentration), eutrophic (rich in nutrients/fertile), and hypertrophic (very rich in nutrients). The nutritional content of water reservoirs fluctuates over time.
- This process of increasing water productivity from oligotrophic to mesotrophic to eutrophic is known as eutrophication.
- When the preceding process is mild and has favourable benefits, it is considered a fertilisation process.
- In contrast, biogenic substance pollution occurs when the process is excessive and its effects are not favourable.
- Nutrient-deficient, low-fertile waters are those with a low concentration of phosphorus and nitrogen — the two most essential nutrients.
- Therefore, the seas are free of phytoplankton and zooplankton and are clean and transparent. In waters with a low plankton concentration, virtually little dead stuff sinks to the bottom.
- Therefore, the degradation of this material does not deplete the oxygen stores near the ocean floor. Up to a certain point, the increase in water fertility results in an increase in the number of most aquatic organisms and a corresponding intensification of life expressions.
- When nutrient levels are excessive, the organic matter produced disrupts the equilibrium of an ecosystem. Certain enzymes secreted by bacteria promote the breakdown of other bacteria and algae.
- Due to the release of organic compounds, plankton microbes flourish. Oxygen depletion in deeper waters leads to the development of anaerobic microbes and the production of methane and hydrogen sulphide.
- Thus, a high vitality indicates an increase in production in water basins, the development of phytoplankton biomass, and a decrease in oxygen content in deeper layers.
- The reproduction of algae in the upper layers of water results in water blooms, which are a result of eutrophication.
- This process alters the colour and turbidity of water, degrades water quality, and generates hazardous chemicals. The natural eutrophication process is extremely slow, taking up to a few thousand years.
- The acceleration of eutrophication is, however, a result of human activity. Consequently, industrial and municipal wastes, as well as areas fed with phosphorus and nitrogen fertilisers, contribute excess levels of nitrogen and phosphorus to water pollution.
- These actions substantially enhance the concentration of biogenes, establishing favourable conditions for the reproduction of algae.
- In such polluted waterways, faeces and harmful bacteria may be able to survive longer. Rainfall in highly industrialised and polluted regions has also influenced eutrophication.
B. Biotic factors
Individual members of the biocenosis that inhabit surface waters interact with one another. As a result, the organisms may cooperate (synergy) or compete (antagonistic interactions) (antagonism).
1. Competition for food
- The species that find and consume food most effectively may have an edge over others. For a particular environment with a typical nutrient supply, the number of microorganisms increases rapidly.
- However, in many instances, the copious synthesis of metabolic products (inhibitors) reduces the number of competitors, sometimes to the point of eradication.
- When the pH is dramatically altered by acidification or alkalinization, or when antibiotic compounds are released, for instance, such conditions can arise.
2. Co-operation
- In feeding and development processes, microorganism cooperation is frequently observed.
- It expedites the growth of mixed microorganism cultures. Biodegradation is a multistage process in which different specialised bacteria conduct successive reactions.
- The procedure prevents the buildup of metabolic byproducts. This collaboration makes possible the biodegradation of persistent organic molecules (ligninocellulose).
3. Predation
- For lower animals, bacteria and fungi are food sources. This is why their numbers in some water reserves can vary greatly. The majority of protozoa eat bacteria.
- It has been established that their biomass increases in tandem with the bacterial population.
- Additionally, numerous multicellular species eat microorganisms. Primarily, these are animals that filter water, such as sponges.
- Numerous creatures in sediments feed on fungus. Benthic blue-green algae are frequently consumed by turbellarians, worms, crabs, and insect larvae.
- Blue-green algae are consumed by zooplankton; without zooplankton, the water would bloom and poisonous compounds would be released.
4. Parasitism
- Microorganisms in water are attacked and eliminated by viruses, bacteria, and fungi. Bacteriophages have been confirmed in both fresh and salt water.
- They are very abundant in sewage and are likely responsible for the rapid depletion of bacteria in sewage-polluted rivers, lakes, and coastal waterways.
- Parasitic Vibrio bacteria, which are members of the Bdellovibrio genus and contribute to the limiting of bacterial populations, are a further factor.
- They attach themselves to host cells, multiply using their energy, and then digest the contents of the host cells. After destroying the host’s cell wall, they escape and infect further germs.
Microorganisms in different Water Habit
1. Microorganisms in Unpolluted Water
- Typically, pristine mountain lake or stream water has few organic nutrients. The quantity of microorganisms per millilitre is only a few thousand.
- Actinomycetes are ubiquitous. Alongside free-living protozoans such as Euglena, Paramecium, and different amoebae, autotrophic bacteria are abundant.
2. Microorganisms in Polluted Water
- In polluted rivers, there is an abundance of organic materials originating from sewage, human waste, and industrial complexes. Typically, the bacteria are heterotrophic.
- Due to the insufficient digestion of organic stuff by these organisms, acids, bases, alcohols, and other gases build.
- Coliform bacteria, gram-negative, non-spore-forming bacilli typically found in the colon, are the most common type of bacteria. This group contains E. coli and Enterobacter species. Lactose is fermented into acid and gas. Also present are non-conform microorganisms such as Streptococcus, Proteus, and Pseudomonas.
- Under particular conditions, polluting organisms proliferate rapidly and use the majority of available oxygen. Nutrients reach the river from sources such as sewage treatment plants and urban/suburban runoff for convenience. Thus, the river develops a sudden high nutritional concentration. Under those conditions, algae may proliferate fast.
- This causes oxygen depletion in water. Protozoa, small animals, fish, and plants have access to very little oxygen. Due to the lack of oxygen, dead organisms, dirt, and silt will accumulate at the bottom, and anaerobic species such as Clostridium, Desulfovibrio, etc. will thrive.
- They release gases. One gas, H2S, interacts with lead or iron to form a precipitate that turns the mud black and poisons the water.
- Due to a total lack of oxygen, the bacteria in suspension perish in their own waste products. At this point, there is hardly any life in the water. Anaerobes in the mud release gas bubbles that break the surface. Such processes result in the river’s demise.
3. Microorganisms in Marine Water
- Only halophilic organisms can survive in the salty water of the ocean. Since the temperature is quite low, the most are psychrophilic.
- Diatoms and protozoa like dinoflagellates are essential food chain components. The oceanic zone offshore contains photosynthetic creatures. This consists of diatoms and dinoflagellates.
- The majority of marine microorganisms are located along the coast or in the littoral zone, where nutrients are abundant.
- On the ocean floor, in the benthic zone and even at the bottom of several-mile-deep tunnels, in the abyssal zone, exist uncommon species.
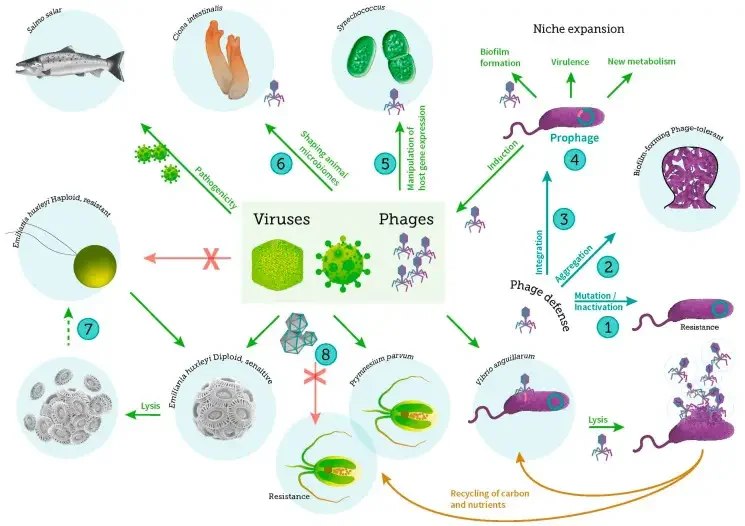
4. Microorganisms in Lakes
- In contrast to rivers and streams, lakes are lentic systems dominated by planktonic microorganisms and animals.
- The topology and hydrology of lakes play a crucial role in the formation of these communities. Lake hydrology encompasses all aspects of water movement, including the lake’s water as well as its input and outflow.
- Due to the fact that majority of a lake’s water originates from the surrounding land, the geology and use of the land that feeds the lake greatly influence the chemistry of the inflow water. For example, highland lakes receive water from very barren soil, resulting in oligotrophic conditions.
- This explains why mountain lakes are typically so clear: only microorganisms that can thrive in low nutrition environments are able to flourish.
- These microorganisms typically have lengthy generation times. Conversely, low-lying lakes typically collect water from fertile, cultivated soils and are therefore nutrient-rich.
- This promotes a high amount of planktonic growth, and lakes may appear murky as a result. The picoplankton often consists of freshwater species of Synechococcus, whereas the macroplankton (microbes larger than 200m) consists of filamentous cyanobacteria such as those in the genera Anabaena and Microcystis.
- Deep lakes can be separated into two major regions: the littoral zone, or shoreline, and the pelagic zone, located in the lake’s centre (these terms also apply to marine systems).
- The depth of the photic zone also defines the littoral zone. Each zone has a separate microbial community.
- Allochthonous DOC provides organic substrates and nutrients such as phosphate and nitrate into the littoral zone.
- Because the water is shallow, light can permeate the entire water column, allowing for the growth of larger plants. Frequently, biofilms grow on these plants and in the sediments. The lake’s depth influences the composition of the microbial population within the pelagic zone.
- Light permeates the whole water column in shallow lakes (less than around 10 metres), therefore benthic autotrophs, such as diatoms and cyanobacteria, may be the most important primary producers.
- In lakes with greater depth, the middle pelagic zone might develop thermal stratification. This is true for temperate regions.
- In the summer, the epilimnion, the uppermost layer of the atmosphere, is warmed and oxygen is exchanged. The thermocline traverses a zone known as the metalimnion; this region prevents the mixing of upper and lower water masses.
- The hypolimnion, the colder, lower zone, can become anoxic. Due to the fact that these two water masses do not interact (or mix very little), the upper layer supports an abundance of primary producers and consumers, which can deplete nutrients.
- In the hypolimnion, where circumstances are dark, anoxic, and chilly, growth is restricted. The microbial population predominantly comprises of benthic heterotrophs and nutrients accumulate.
- When autumn weather cools the top and storms physically mix the two levels, a brief bloom can result from the mixing of nutrients from the lake’s bottom with the photic zone. In the spring, when temperature stratification occurs but nutrients in the epilimnion are not exhausted, blooms may be much longer.
- There are no substantial annual temperature changes in tropical lakes, but there are seasonal differences in precipitation. Although there are many similarities between tropical and temperate lakes, there are significant distinctions.
- Plankton blooms may occur at any time of the year due to the warmer, year-round temperatures, which promote greater productivity.
- In tropical lakes, cyanobacteria capable of nitrogen fixation may predominate, as low N:P ratios are common. In temperate lakes, nutrient cycling is mostly driven by physical mixing, but in tropical lakes, biological activities are more essential.
- In this regard, the figure-depicted microbial loop can be applied to the epilimnion of larger tropical lakes that receive minimal allochthonous nutrients.
- Lakes differ in nutritional quality. Others are eutrophic while others are oligotrophic. Throughout the year, nutrient-poor lakes remain oxic, and seasonal temperature fluctuations rarely result in oxygen stratification.
- In contrast, the sediments at the bottom of eutrophic lakes are typically rich in organic matter. Cyanobacteria that are capable of nitrogen fixation may proliferate in oligotrophic lakes.
- Several taxa, including Anabaena, Nostoc, and Cylindrospermum, possess the ability to fix nitrogen under oxic environments.
- Those belonging to the genus Oscillatoria utilise hydrogen sulphide as an electron donor for photosynthesis, allowing nitrogen fixation in anoxic environments.
- In the presence of both nitrogen and phosphorus, cyanobacteria compete with algae. Cyanobacteria perform better in alkaline waters (pH 8.5 to 9.5) and at higher temperatures (30 to 35°C). By consuming carbon dioxide at a high rate, cyanobacteria raise the pH, making the environment less appropriate for protists. Cyanobacteria have additional benefits in competition.
- Many create hydroxamates, which bind iron, reducing the availability of this essential trace nutrient for protists. Some cyanobacteria are resistant to predation due to the production of toxins.
- Moreover, some produce odor-causing substances that degrade the quality of drinking water. When cyanobacteria or protists (algae) generate enormous blooms in highly eutrophied lakes, these issues are exacerbated.
- The management of lakes can improve the situation by removing or sealing sediments from the lakebed or by introducing coagulants to accelerate sedimentation.
5. Microorganisms in Streams and Rivers
Streams and rivers are significantly more active than glacial lakes. The table below lists some of the physical and biological elements that help manage the microbial populations of various freshwater environments.
- Lakes, rivers, and streams can be generically characterised as either lotic systems with free-flowing waters (streams, the majority of rivers, and canals) or lentic systems with still waters (e.g., ponds; lakes; marshes; very broad, slow-moving rivers).
- In vast, lentic rivers and lakes, phytoplankton flourish and account for the majority of the fixed carbon. Autochthonous refers to the biologically accessible carbon produced inside the system.
- In contrast, the steady flow of water in streams and all rivers but the largest hinders the growth of considerable planktonic ecosystems.
- In addition, the amount of available light due to overhanging vegetation, turbidity, and rapid water mixing can further inhibit phytoplankton growth. Therefore, the source of nutrients in the majority of streams and rivers is the surrounding land.
- These nutrients are known as allochthonous. This contrast is significant because lentic systems often have a net autotrophic metabolism, whereas lotic systems are typically heterotrophic.
- Both dissolved and particulate organic carbon are included in the allochthonous carbon that enters rivers and streams (DOC and POC, respectively).
- The amount of carbon that enters freshwater systems is dependent on the surrounding flora, the proximity of human activities, and the geology of the area.
- In addition, hydrological conditions, such as the length of time water remains in the soil before joining a river or stream, impact the form of organic carbon.
- Therefore, DOC ranges from readily assimilable material to carbon that only a few microbial groups can slowly decay.
- Much of the particulate organic carbon consists of big things such as leaf litter, which serve as a substrate for the colonisation of fungus and bacteria.
- The activity of these bacteria initially releases a burst of water-soluble DOC, followed by the steady leaching of nutrients that may not be as readily assimilable.
- Most rivers and streams feature thriving benthic communities of connected bacteria that produce biofilms that are devoured by grazing species. Some biofilms in shallow rivers and streams are dominated by diatoms and other photosynthetic protists, which contribute to the system’s overall primary output. Biofilms composed mostly of heterotrophic microorganisms are significant sources of decomposition and mineralization.
- The microbial loop can be used to characterise the involvement of benthic microorganisms in rivers and streams.
- When river and stream chemoorganotrophic bacteria digest the available organic material, they recycle nutrients within the environment, as they do in all microbial communities.
- Autotrophic microorganisms utilise the minerals generated by decaying organic materials for growth. Consequently, during daylight hours, when the rates of photosynthesis and respiration are in dynamic equilibrium, O2 is produced.
- Streams and rivers are kept productive when the amount of organic matter introduced does not exceed their oxidative capacity.
- However, like with ocean dead zones, the ability of aquatic bacteria to metabolise extra organic matter is restricted, and the water may become anaerobic. This occurs occasionally in streams and rivers bordering urban and rural regions.
- Point source pollution is the release of untreated municipal wastes and other contaminants from a specific spot along a river or stream.
- Such additions of organic matter can cause unique and predictable changes in the microbial community and oxygen content, resulting in an oxygen sag curve where oxygen is depleted directly upstream of the pollution source.
- Non-point source pollution is represented by runoff from agriculturally active fields and feedlots. This can also lead to microbial community imbalance and algal or cyanobacterial blooms.
6. Microorganisms in Glaciers and Permanently Frozen Lakes
- The majority of the Earth’s ice has stayed frozen for millions of years. It is vital to note that the majority of Earth’s surface temperature never reaches 5°C.
- This covers arctic regions, the depths of the ocean, and high altitude locations on land throughout the earth.
- Microbes within glaciers are surprisingly not dormant. Rather, over the past decade, evidence suggesting active microbial communities occur in these conditions has increased.
- In reality, this is an exciting time for glacial microbiology; identifying the variety in these systems and evaluating the importance of these microorganisms in biogeochemical cycling might include innovative and creative methods.
- Moreover, with the finding of ice on Mars and Jupiter’s moon Europa, astrobiologists have taken a keen interest in ice-dwelling psychrophilic microorganisms.
- As it has become evident that these ecosystems serve as sentinels of climate change, the biology of lakes connected with glaciers has acquired significance.
- Low temperature and limited solar exposure constrain glacial lakes, and in the Antarctic, lakes are also separated from macrofauna. Here, phytoplankton, bacteria, and protists compose basic food webs nearly devoid of multicellular creatures.
- It is not surprising that the primary production of these lakes must be augmented by the deposition of organic carbon from the surrounding environment, as microbial growth rates are modest.
- In fact, phototrophic flagellated and ciliated protists can consume both bacteria and dissolved organic carbon. These mixotrophs are not the only bacteria-consuming organisms; the majority of viruses are bacteriophages.
- In Antarctica’s lakes, the rate of viral lysis of bacteria is nearly tenfold that of comparable temperate and tropical lakes.
References
- Aryal, Sagar & Karki, Gaurab & Pandey, Sunil. (2015). Microbial Diversity in Freshwater and Marine Environment. Nepal Journal of Biotechnology. 3. 68. 10.3126/njb.v3i1.14236.
- Pernthaler, J. (2013). Freshwater Microbial Communities. In: Rosenberg, E., DeLong, E.F., Lory, S., Stackebrandt, E., Thompson, F. (eds) The Prokaryotes. Springer, Berlin, Heidelberg. https://doi.org/10.1007/978-3-642-30123-0_40
- https://en.wikipedia.org/wiki/Marine_microorganisms
- https://microbiologynotes.org/microorganisms-in-freshwater-ecosystems/
- http://archives.esf.org/fileadmin/Public_documents/Publications/MarineBoard_PP17_microcean.pdf
- https://www.suezwaterhandbook.com/water-and-generalities/aquatic-organisms/importance-of-aquatic-micro-organisms-for-the-water-treatment-specialist/micro-organisms-for-which-freshwater-is-their-natural-habitat
- http://www.ramp-alberta.org/river/ecology/life/microorganisms.aspx
- https://ccelms.ap.gov.in/adminassets/docs/21112020171644-microflora_of_fresh_waterr_and_marine_water_final.pdf
- https://plantlet.org/microbiology-in-freshwater-and-marine-habitats/
- https://www.slideshare.net/FatimahTahir/microbial-flora-of-the-aquatic-env