What are Pesticides?
any substance or mixture of substances intended for preventing, destroying, or controlling any pest, including vectors of human or animal disease, unwanted species of plants or animals, causing harm during or otherwise interfering with the production, processing, storage, transport, or marketing of food, agricultural commodities, wood and wood products or animal feedstuffs; or substances that may be administered to animals for the control of insects, arachnids, or other pheromone-producing arthropods.
- Pesticides are chemical chemicals designed to eliminate pests. Pesticides are typically chemical or biological agents, such as a virus, bacteria, antibiotic, or disinfectant, that discourage, incapacitate, or kill pests.
- This pesticide application is so prevalent that the term pesticide is frequently used interchangeably with plant protection product.
- It is often used to eradicate or control a wide range of agricultural pests that can cause crop and livestock damage and diminish farm output.
- The most often used pesticides include insecticides, herbicides, rodenticides, and fungicides to control fungi, mould, and mildew.
- Pesticides are not recent innovations. Numerous ancient civilizations protected their crops from insects and other pests with insecticides.
- Ancient Sumerians employed elemental sulphur to repel insects from their crops. In contrast, mediaeval farmers experimented with arsenic and lead-based poisons on popular crops.
- To combat body lice and other pests, the Chinese utilised arsenic and mercury compounds. While the ancient Greeks and Romans employed oil, ash, sulphur, and other substances to protect themselves, their cattle, and their crops from a variety of pests, modern farmers utilise pesticides.
- In the meantime, researchers in the nineteenth century focused more on natural procedures employing chemicals derived from the roots of tropical crops and chrysanthemums.
- In 1939, Dichloro-Diphenyl-Trichloroethane (DDT) was discovered; it has since become the world’s most effective and widely used insecticide. However, twenty years later, nearly 86 nations have banned DDT due to its biological impacts and human safety.
Types of Pesticides
These are categorised by the sorts of pests they eliminate:
Grouped by Types of Pests They Kill
- Insecticides – insects
- Herbicides – plants
- Rodenticides – rodents (rats & mice)
- Bactericides – bacteria
- Fungicides – fungi
- Larvicides – larvae
Based on how biodegradable they are
Additionally, pesticides can be considered:
- Biodegradable: Biodegradable materials are those that can be degraded by microorganisms and other living organisms into harmless chemicals.
- Persistent: The persistent ones, on the other hand, may take months or even years to break down.
Consider those that are chemical forms or are obtained from a common source or production process as a second classification method.
Chemically-related pesticides
- Organophosphate: The majority of organophosphates are insecticides; they damage the neurological system by interfering with the enzyme that controls a neurotransmitter.
- Carbamate: Similar to organophosphorus pesticides, carbamate pesticides harm the neurological system by interfering with an enzyme that controls the neurotransmitter. However, the effects of enzymes are typically reversible.
- Organochlorine insecticides: Organochlorine pesticides were once widely employed, but many nations have withdrawn them from the market due to their adverse health and environmental consequences, as well as their tenacity (e.g., DDT, chlordane, and toxaphene).
- Pyrethroid: These are a synthetic form of the naturally occurring pesticide pyrethrin, which is found in chrysanthemums (Flower). They were designed to have the greatest environmental stability possible.
- Sulfonylurea herbicides: For weed management, sulfonylurea herbicides such as pyrithiobac-sodium, cyclosulfamuron, bispyribac-sodium, terbacil, and sulfometuron-methyl have been sold. Sulfosulfuron, rimsulfuron, pyrazosulfuron-ethyl, imazosulfuron, nicosulfuron, oxasulfuron, nicosulfuron, flazasulfuron, primisulfuron-methyl, halosulfuron-methyl, flupyrsulfuron-methyl-sodium, ethoxysulfuron, chlorimuron-ethyl, bensulfuron-methyl, azimsulfuron, and amidosulfuron.
- Biopesticides: Biopesticides are pesticides derived from natural substances such as animals, plants, microbes, and certain minerals.
Examples of pesticides
Examples of pesticides are fungicides, herbicides, and insecticides. Specific examples of synthetic chemical pesticides include glyphosate, aciphate, deet, propoxur, metaldehyde, boric acid, diazinon, dursban, ddt, and malathion, among others.
Benefits of Pesticides
The greatest benefit of pesticides is their ability to save farmers. By preventing insects and other pests from damaging crops. However, the following are other major benefits.
- Controlling pests and vectors of plant diseases.
- Controlling human/animal disease vectors and pest species.
- Controlling organisms that threaten human buildings and activities.
Effects of Pesticides
- Changes in the ecological equilibrium of the soil microflora.
- Large-scale application of pesticides may result in permanent alterations to the soil microbiota.
- negative impact on soil fertility and agricultural yield
- Inhibition of soil bacteria that fix nitrogen, such as Rhizobium, Azotobacter, and Azospirillum, as well as cellulolytic and phosphate solubilizing microorganisms.
- Nitrosomonas and Nitrobacter have been observed to be inhibited by the soil fumigants ethylene bromide, Telone, and vapam.
- modifications in the soil’s nitrogen equilibrium.
- interfering with soil ammonification.
- Negative effect on plant mycorrhizal symbioses and legume nodulation.
- modifications in the microbiota of the rhizosphere, both numerically and qualitatively.
What is Biodegradation?
- Biodegradation is the process through which microorganisms, such as bacteria and fungi, decompose organic matter.
- It is commonly believed to be a natural process, distinguishing it from composting. Composting is a human-driven process in which biodegradation takes place under particular conditions.
- Objects undergo biodeterioration, which is the mechanical weakening of their structure, biofragmentation, which is the breakdown of materials by microbes, and assimilation, which is the integration of old material into new cells.
- In practise, virtually all chemical compounds and materials are susceptible to biodegradation, with time being the determining factor.
- Vegetables can decay in a matter of days, whereas glass and other polymers require several millennia.
- Within six months, greater than 90 percent of the original material must be transformed into CO2, water, and minerals by biological processes, according to a European Union criterion for biodegradability.
Factors that affect the degradation of pesticides by Microorganisms
1. The impact of microbes themselves
- The microbial species, metabolic activity, environmental adaptation, and other factors have a direct effect on the breakdown of pesticides, and the spatial distribution, population density, and interactions with other microorganisms have affected the degradation efficiency of microorganisms.
- Numerous tests have demonstrated that the organic substrate responses of different microbial species or the same species with different strains are vastly distinct.
- Microorganisms acquire the ability to degrade by a specific adaption process, the introduction of new chemicals, or the production of related enzymes through gene mutations, as a result of their robust adaptability and domestication.
- The functional properties and variations of microbial degradation are also major determinants of degradation capability and mechanism.
- With a profound understanding of microorganisms, pesticide degradation research will advance by leaps and bounds. Varied microbes have different effects on the degradation of different pesticides, which is why scientists are typically concerned about the issue.
2. Effects of Pesticide Structures
- The difficulty of microorganism degradation is affected by the molecular weight of pesticide compounds, the structure of the space, the type and quantity of substituents, and the number of substituents.
- Under normal conditions, polymer compounds are more difficult to disintegrate than low molecular weight compounds; polymers, complexes are more resistant to biodegradation; simple structure is more resistant to degradation than complex structure.
- Compounds containing -OH or -NH2 on the benzene ring were more prone to breakdown by Pseudomonas sp. WBC-3, which was typically pre-hydroxylated and re-opened, consistent with the Principle.
- Degradation of PAHs in settings of tiny compost. It was shown that aromatics with 2 to 4 rings were more susceptible to degradation than aromatics with 5 to 6 rings.
- The majority of current environmental contaminants are synthetic biologically heterologous organic compounds that do not occur in nature and tend to display high microbe resistance.
- Because these molecules are very short, microorganisms have not evolved the metabolic pathways necessary to breakdown them.
- Despite the fact that some harmful substances may be destroyed slowly in nature by the synergistic effect of naturally occurring microbial populations, this remains a new problem for the microbial world.
- The process by which microorganisms can degrade the capability of a chemical by altering its own information is sluggish, and the natural evolutionary process of bacteria is plainly inadequate to meet the requirements when compared to the synthetic heterologous substances that are extensively utilised today.
- Consequently, Will cause a disruption to the entire ecosystem. Therefore, it is crucial and necessary to investigate techniques that enable microbial populations to achieve maximum isoform degradation in a relatively brief amount of time.
3. Impact of environmental factors
- Pesticide pollution and its degradation by microorganisms are influenced by environmental temperature, humidity, pH, the presence of carbon and nitrogen, and other organic matter, salinity, matrix adsorption, viscosity, and ventilation.
- Most microorganisms have the optimal temperature for development, and the degrading enzyme has the optimal temperature for reaction. Changes in temperature can impact the metabolism of microorganisms, the enzymatic activity of degrading enzymes, and even the physical state of pesticide pollutants, so influencing the pace of disintegration.
- In general, alkaline or neutral pH is conducive to bacterial multiplication, while acidic pH is conducive to fungus reproduction.
- Alkaline ph, organic phosphorus, and organochlorine insecticides are vulnerable to breakdown and have low residual levels. In the moist, organic matter-rich, well-ventilated environment, aerobic or facultative anaerobic microbial growth can flourish.
- The activity of microorganisms degrading polycyclic aromatic hydrocarbons in compost is directly proportional to oxygen concentration and moisture content.
- Compost is transformed from aerobic to anaerobic conditions when its oxygen level is less than 18% and its moisture content is greater than 75%, consequently impacting the breakdown of PAHs.
Criteria for Biodegradation
For effective biodegradation of pesticides in soil, the following factors must be considered:
- To decrease the quantity of pollutants, organisms must possess the catabolic activity necessary for their rapid breakdown.
- The bioavailability of the pollutant must be the aim.
- Therefore, favourable circumstances must exist for microbial/plant development and enzyme activity.
- The cost of bioremediation must be cheaper than alternative methods of pollutant removal.
Microorganisms involved in the biodegradation of pesticides
- Various biological systems, including microbes, have been employed to biotransform insecticides.
- It has been observed that a portion of the soil biota can rapidly acquire the ability to breakdown some pesticides when they are sprayed consistently to the soil. These substances provide a suitable carbon supply and electron donors for specific soil microorganisms, thereby establishing a treatment method for pesticide-contaminated locations.
- The transformation of such substances depends not only on the existence of microorganisms with suitable degrading enzymes, but also on a vast array of environmental factors.
- Fungi and bacteria are regarded as the most effective extracellular enzyme-producing microorganisms. White rot fungi have been suggested as promising bioremediation agents, particularly for chemicals that are difficult for bacteria to breakdown. This capability is a result of the creation of extracellular enzymes that act on a wide variety of chemical molecules.
- The following bacteria are commonly involved in the breakdown of pesticides. Aspergillus fumigatus, A. niger, A. terreus, Absidia, Rhizopus microsporus var corymberifera, microsporis, Pseudomonas, Rhodococcus sp Absidia , Fusarium, Botrytis cinerea Trichoderma viridae, etc.
Strategies for Bioremediation
Following strategies are required for the successful biodegradation / bioremediation of a given pollutant.
- Passive/ intrinsic Bioremediation: Passive/ intrinsic Bioremediation is the natural bioremediation of contaminants by indigenous microorganisms, with a relatively sluggish rate of disintegration.
- Biostimulation: The practise of adding nitrogen and phosphate to soil to stimulate native microorganisms.
- Bioventing: Process/method of Biostimulation in which gases stimulating microbial activity, such as oxygen and methane, are introduced to or pushed into soil.
- Bioaugmentation: Bioaugmentation is the inoculation or introduction of microorganisms to a contaminated site or soil in order to promote biodegradation.
- Composting: To breakdown toxins, contaminated soils are piled and treated with aerobic thermophilic bacteria by composting. To encourage microbial activity, periodic physical mixing and wetting of piles is performed.
- Phytoremediation: Phytoremediation can be accomplished either directly or indirectly by growing plants that hyperaccumulate heavy metals or by plants stimulating microorganisms in the rhizosphere.
- Bioremediation: The use of microorganisms to detoxify toxic/unwanted chemicals/contaminants in soil and other environments.
- Mineralization: Mineralization is the complete transformation of an organic pollutant into its inorganic component by a microbe or microbial community.
The mechanism of microbial degradation of pesticides
- Microorganisms on the role of pesticides can be divided into two classes: a class of microorganisms directly on the role of pesticides, pesticide degradation by enzymatic reactions; it is often said that pesticide microbial degradation belongs to this class; and a class of microorganisms that change the chemical and physical environment and indirectly apply to pesticides.
- On pesticides, common modes of action include mineralization, co-metabolism, bioconcentration or cumulative effects, as well as microbial effects.
- The enzymatic reactions involved in the microbial breakdown of pesticides are primarily oxidation, dehydrogenation, reduction, hydrolysis, and synthesis.
- When intracellular enzymes are responsible for the destruction of microbial pesticides, the entire degradation process consists of three steps:
- The adsorption of pesticides on the surface of microbial cells is a dynamic equilibrium that leads to the rapid breakdown of the pesticides. Phase crucial of the lag phase.
- Pesticides permeate the cell membrane, the permeability of the cell membrane dictates the amount of pesticide that can permeate the cell membrane, and pesticide penetration of the cell membrane is the degradation rate limiting step. This pesticide penetration rate is highly related to their molecular structural factors (mainly lipophilic parameters and steric hindrance parameters).
- Pesticides in the cell membrane are rapidly degraded through an enzymatic reaction involving the degrading enzyme.
1. Mineralization
- Numerous chemical pesticides are mimics of natural substances and can be employed as a source of microbial nutrition through microbial breakdown, the generation of inorganic compounds, carbon dioxide, and water.
- Mineralization is the optimal method of degradation. This is due to the complete degradation of the pesticide into non-toxic inorganic substances.
2. Co-metabolism
- Some synthetic chemicals cannot be destroyed by microorganisms, but in the presence of another carbon matrix and energy, they can be partially decomposed; this phenomenon is known as co-metabolism.
- The degradation of the monomethylamine product of Pseudomonas dactylus DR-8 was 2,4-dimethylaniline and NH3, and the DR-8 strain could not grow with monomethylamine as a carbon source and energy, Other organic nutrient matrix as a carbon source under the conditions of methamidine degradation, and the methamidine degradation products are not fully mineralized, belonging to the common metabolic type.
- Utilizing organic pesticides as a carbon source, nitrogen source, or decomposing complex pesticide molecules into simple compounds like CO2, H2O, and NH3 reduces pesticide residues and toxicity in the environment.
3. Other microbial degradation pathways
- Hydrolysis: Under the influence of microorganisms, the ester bond and the dialkylamine bond hydrolyze, resulting in the detoxification of pesticides such as malathion, propanil, and others.
- Dehalogenation: Fecal hydrocarbon pesticides, in the role of the enzyme, the halogen substituents by the H atom or carboxyl, to replace the loss of toxicity, such as DDT degradation into DDE.
- Oxidation: Microorganisms, through the creation of oxidase, insert a hydroxyl or produce an epoxide, such as carbendazim and 2,4- D degradation, into organic compounds, especially those with an aromatic ring.
- Nitro reduction: N2O in the pesticide to NH2, such as 2,4-dinitrophenol, breakdown products of 2-amino-4-nitrophenol and 4-amino-2-nitrophenol; parathion to amino-phosphorus.
- Methylation: To passivate methyl groups, add hazardous phenols such as pentachlorophenol, tetrachlorophenol, and others.
- Demethylation: Containing methyl or other hydrocarbon groups, related to N, O, and S, to remove these groups into non-toxic chemicals, such as the removal of two N-methyl from dexamethasone degradation.
Major enzymes applied for pesticide biodegradation
1. Hydrolases
- Hydrolases are a large set of enzymes involved in the biodegradation of pesticides.
- Hydrolases accelerate the hydrolysis of several key biochemical groups of pesticide (esters, peptide bonds, carbon-halide bonds, ureas, thioesters, etc.) and often work without redox cofactors, making them good candidates for all of the current bioremediation techniques.
- As an illustration of the catalytic activity of enzyme hydrolases, the degradation process of the pesticide carbofuran is provided as an example.
- This herbicide is susceptible to transformation in the environment, and several metabolites are produced and deposited in potentially polluted areas (soil, water and sediments, mainly).
- Diverse species isolated from contaminated areas have been identified and classified as carbofuran-transforming microorganisms, resulting in distinct metabolites.
2. Phosphodiesterases
- PTEs are one of the most important classes of pesticide-degrading enzymes that have been extensively explored.
- These enzymes, which hydrolyze and detoxify organophosphate insecticides, have been extracted from several microorganisms (OPs). This decreases the toxicity of OPs by reducing their capacity to inactivate AchE.
- The first phosphotriesterase to be isolated is a member of the Pseudomonas diminuta MG species; this enzyme is highly catalytic towards organophosphate insecticides. The phosphotriesterases are coded by the opd gene (organophosphate-degrading). Flavobacterium ATCC 27551 contains the PTE-encoding opd gene. This individual cloned and sequenced the gene. These enzymes specifically hydrolyze phosphoester bonds, such as P–O, P–F, P–NC, and P–S, with a water molecule at the phosphorus core serving as the hydrolysis mechanism.
- Various microbial enzymes capable of hydrolyzing MP have been identified, including organophosphorus hydrolase (OPH; encoded by the opd gene), methyl-parathion hydrolase (MPH; encoded by the mpd gene), and hydrolysis of coroxon (HOCA; encoded by the hocA gene), which were isolated from Flavobacterium sp., Plesimonas sp. strain M6, and Pseudomonas
- The phosphotriesterase enzyme is a homodimeric protein with a molecular weight of 36 kilodaltons per monomer.
- As a first stage in the PTE organophosphorous pesticide hydrolysis mechanism, the enzyme active site takes a proton from water, activating this molecule. The activated water then directly attacks the core phosphorus of the pesticide molecule, causing a configuration inversion. A zinc atom is responsible for polarising the oxygen at the active site.
- This enzyme may be used to clean settings contaminated with organophosphorus insecticides.

![Degradation of Pesticides - Types, Mechanisms 2 Proposed mechanism for PTE activity. Zinc’s active site functions in phosphate polarization, making phosphor more susceptible to the attack. 1) A base subtracts a proton from a water molecule with the subsequent attack of the hydroxyl to the central phosphorous. 2) The intermediary complex originates the products 3) p-nitrophenol and diethyl thiophosphate [6].](https://biologynotesonline.com/wp-content/uploads/2022/10/image4-1024x309.webp)
3. Esterase
- Esterases are enzymes that catalyse hydrolysis reactions involving carboxylic esters, amides, phosphate esters, etc.
- In the reaction catalysed by esterases, a wide variety of ester substrates are hydrolyzed into their alcohol and acid components. R = O-OCH3+ H2O↔R = O-OH + CH3OH
- Numerous insecticides (organophosphates, carbamates, and pyrethroids) include a carboxylic ester, and carboxylesterases are the enzymes capable of hydrolyzing this ester link.
- Multiple classification nomenclature methods are now employed for these enzymes. According to the nomenclature of the International Union of Biochemistry and Molecular Biology (IUBMB), carboxylesterases belong to the subgroup 1 and subtype 1 of hydrolases (Enzyme Commission 3.1.1.1, EC 3.1.1.1).
- The nomenclature splits the esterases into three groups based on the nature of their interactions with organophosphorus insecticides, which is another frequent classification. According to this classification, carboxylesterases belong to the category of ali-esterases and B-esterases. Esterases are a broad class of arthropod enzymes.
- The esterases are a very varied set of enzymes that has been recognised as one of the most significant in the metabolism of xenobiotics, and whose mechanism is related with the creation of large quantities of multifunctional hydrolytic enzymes. Enzymes capable of hydrolyzing organophosphate insecticides.
- There are various types of esterases, and their distribution in tissues and organisms varies greatly. In addition to indigenous chemicals, the Carboxiesterases (type B esterases) hydrolyze xenobiotics with ester, amide, thioester, phosphate esters (parathion, paraoxon), and acid anhydrides (DIPFP=DFP) in mammals.
- The active core of esterases A has a Cys residue, whereas esterases B have a Ser residue.
- In esterases A, organophosphates engage with the SH functional group to generate a P=S bond that is readily hydrolyzed by H2O. Organophosphates interact with the SER-OH of esterase B, generating a P=O bond that is not dissolved by H2O. Organophosphates that bind to esterase B limit its enzymatic activity in a stoichiometric manner.
- Esterases are a heterogeneous group that protects the target site (acetylcholinesterase) by accelerating the hydrolysis of insecticides or by functioning as a substitute blank. Esterases have a broad spectrum of substrate specificities; they can bind to phosphate triesters, esters, thioesters, amides, and peptides, among others.
4. Oxidoreductases
- Oxidoreductases are an enzyme family that catalyses the transfer of electrons from one molecule (the reductant or electron donor) to another (the oxidant, or electron acceptor).
- Microorganisms play a crucial role in the removal of xenobiotics such as endosulfan from contaminated locations due to their dynamic, complex, and intricate enzyme systems that breakdown these compounds by removing their functional groups of the parent substance.
5. Mixed Function Oxidases (MFO)
- In the MFO-catalyzed reaction (EC 1.14.14.1), an oxygen atom is integrated into the substrate while the other oxygen molecule is reduced to water.
- In order to function, the MFO requires Nicotiamide-adenine dinucleotide phosphate (NADPH) and oxygen.
- It is an enzyme system consisting of two membrane proteins, cytochrome P450 and NADPH-cytochrome P450 reductase.
- They are also known as P450 system or dependent cytochrome P-450 monooxygenases.
- Based on their sequence similarity, the genes encoding the different isozymes form a superfamily of over 200 genes organised into 36 families.
- Enzymes of cytochrome P450 are involved in the metabolism of a wide variety of xenobiotics.
- The cytochrome P450 family is a large, well-characterized set of monooxygenase enzymes that have long been recognised for their potential in numerous industrial processes, particularly due to their capacity to enantiospecifically oxidise or hydroxylate substrates using molecular oxygen.
- Numerous cytochrome P450 enzymes have been demonstrated to catalyse biochemically refractory processes, such as the oxidation or hydroxylation of non-activated carbon atoms, with a broad substrate range. These characteristics are optimal for the cleanup of persistent pesticide residues.
- In diverse prokaryotes and eukaryotes, over 200 subfamilies of P450 enzymes have been identified. All of them have a catalytic iron-containing porphyrin group that absorbs carbon monoxide at 450 nm.
- P450 enzymes, like many of the other oxidoreductases discussed previously, require a non-covalently bound cofactor (NAD(P)H) to recycle their redox centre, limiting their potential for pesticide bioremediation to strategies involving living creatures.
- MFOs, which are located in the endoplasmic reticulum and mitochondria of insects, are involved in numerous processes, including growth, development, reproduction, detoxification, etc.
- MFO are engaged in the metabolism of both endogenous and exogenous drugs; hence, these molecules facilitate their induction. Due to their strong nonselectivity, MFOs metabolise a vast array of chemicals, including organophosphates, carbamates, pyrethroids, DDT, inhibitors of chitin production, juvenile hormone mimics, etc.
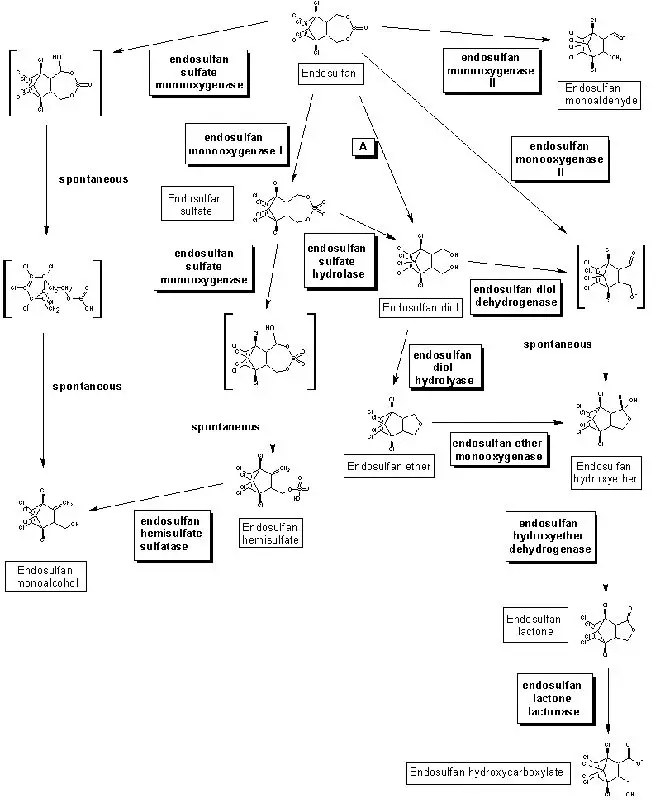
7. Glutathione S-Transferase (GST)
- The GSTs (EC 2.5.1.18) catalyse the conjugation of hydrophobic components with glutathione tripeptide.
- In this reaction, the thiol group of glutathione reacts with an electrophilic site on the target compound to form a conjugate that can be metabolised or excreted. These conjugates are involved in numerous cellular physiological processes, including detoxification of endogenous and exogenous compounds, intracellular transport, biosynthesis of hormones, and protection from oxidative stress.
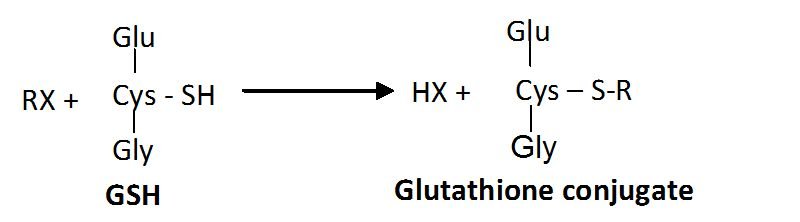
Chemical Reactions Leading to Biodegradation
Pesticide biodegradation is frequently complicated and requires a variety of biological reactions:
1. Detoxification
- Transformation of the molecule of the pesticide into a non-toxic chemical.
- A single mutation in the side chain of a complex molecule may render the substance non-toxic.
2. Degradation
- The decomposition or breakdown of a complex substrate into simpler compounds, which ultimately leads to mineralization. Thirum (fungicide) is degraded by a strain of Pseudomonas to dimethlamine, proteins, sulpholipaids, etc.
3. Conjugation
- The process through which an organism modifies the substrate or mixes the pesticide with cell metabolites.
- Conjugation is achieved by organisms catalysing the addition of an amino acid, organic acid, or methyl crown to the substrate; for example, in the microbial metabolism of sodium diethyldithiocarbamate, the organism combines the fungicide with an amino acid molecule that is normally present in the cell, rendering the pesticides/chemicals inactive.
4. Activation
- It is the transformation of a non-toxic substrate into a toxic molecule; for example, 4-butyric acid (2, 4-D B) and phorate are converted and activated microbiologically in soil to produce metabolites that are poisonous to weeds and insects.
5. Changing the spectrum of toxicity
- Some fungicides/pesticides are meant to control a specific group of organisms/pests, but they are metabolised to produce compounds that are inhibitory to wholly dissimilar groups of organisms; for example, the fungicide PCNB is transformed in soil to pesticidal chlorinated benzoic acids.
6. Leaching
- Since many pesticides are soluble, they are eliminated via leaching.
Example of Biodegradation of Pesticides
1. Dichloro Diphenyl Tricholroethene (DDT)
- Probably the most widely recognised instance of a chlorinated insecticide.
- This chemical has been used extensively as an insecticide to manage a variety of insect pests.
- However, its usage has been prohibited or restricted in numerous nations due to its harmful effects. Others have discovered that the process can take fifteen to twenty years or more. DDT can be destroyed in the soil in two years.
- The major breakdown products of DDT are DDE and DDD, which are dechlorination products. The path can be either DDTDDE> DDD or DDT> DDD. DDE is quickly dechlorinated anaerobically to DDNU and then to further compounds.
- The majority of microorganisms involved in DDT breakdown are bacteria, particularly soil inhabitants belonging to the genera Bacillus, Pseudomonas, Arthrobacter, and Micrococcus.
2. Carbamates
- Carbamates were introduced as pesticides in the early 1950s and continue to be widely utilised as a result of their efficacy and broad spectrum of biological action.
- They are substitutes for highly stable organochlorines like DDT. Carbofuran hydrolyase is primarily responsible for the hydrolysis of the methylcarbamate bond during pesticide degradation. Pseudomonas, Flavobacterium, Achromobacterium, Sphingomonas, and Arthrobacter are the genera containing strains capable of digesting carbamate.
Advantages
- Typically less expensive and minimally disruptive to the site.
- It reduces waste irreversibly. averts long-term responsibility.
- It can be combined with other physical or chemical ways of treatment.
Disadvantages
- Treatment duration is often lengthier.
- Biodegradable substances represent the only pollutants that can be properly addressed.
- The method is sensitive to the ground’s toxicity level and environmental conditions.
- If the process is not regulated, it is likely that the organic contaminants will not be completely degraded, resulting in harmful byproducts that are more mobile than the original pollution.
- Certain substances cannot be decomposed.
- Producing unknown byproducts is possible.
- Contaminants’ toxicity can be an issue.
References
- Ortiz-Hernández, M. L. Sánchez-Salinas, E., EdgarDantán-González, E., & Castrejón-Godínez, M. L. (2013). Pesticide Biodegradation: Mechanisms, Genetics and Strategies to Enhance the Process. In R. Chamy, & F. Rosenkranz (Eds.), Biodegradation – Life of Science. IntechOpen. https://doi.org/10.5772/56098
- Huang Y, Xiao L, Li F, Xiao M, Lin D, Long X, Wu Z. Microbial Degradation of Pesticide Residues and an Emphasis on the Degradation of Cypermethrin and 3-phenoxy Benzoic Acid: A Review. Molecules. 2018 Sep 11;23(9):2313. doi: 10.3390/molecules23092313. PMID: 30208572; PMCID: PMC6225238.
- Porto, André & Melgar, Gliseida & Kasemodel, Mariana & Nitschke, Marcia. (2011). Biodegradation of Pesticides. 10.5772/17686.
- https://www.slideshare.net/aachaljain2/biodegradation-of-pesticides
- https://extension.missouri.edu/publications/g7520
- https://www.sciencedirect.com/topics/agricultural-and-biological-sciences/pesticide-degradation
- https://www.slideshare.net/NEHAMISHRA150/miampco
- https://www.slideshare.net/AsheeshMishra8/bioremediation-of-pesticides
- https://www.slideshare.net/AmanChauhan8/biodegradation-of-pesticides-237399110
- https://www.ijcmas.com/vol-3-10/Kavita%20Rani%20and%20Geeta%20Dhania.pdf
- https://clu-in.org/download/techdrct/tdfrazar.pdf
- https://agriinfo.in/soil-microorganisms-in-biodegradation-of-pesticides-and-herbicides-168/
- https://citeseerx.ist.psu.edu/viewdoc/download?doi=10.1.1.716.3275&rep=rep1&type=pdf
- http://www2.bio.ku.dk/bibliotek/phd/Lea%20Ellegaard-Jensen.pdf
- https://www.biologydiscussion.com/biotechnology/biodegradation/biodegradation-and-bioremediation-with-diagram/11043
- https://www.intechopen.com/chapters/45111