What is Cyanobacteria?
- Cyanobacteria, also known as Cyanophyta or blue-green algae, are a phylum of photosynthetic bacteria that obtain energy through photosynthesis. The name “cyanobacteria” refers to their characteristic blue color. While they are often referred to as blue-green algae, they are not classified as true algae.
- Cyanobacteria are ancient organisms, with fossil records dating back 3.5 billion years. They played a crucial role in shaping the Earth’s atmosphere by being the first organisms to produce oxygen through photosynthesis. This process led to the Great Oxidation Event and significantly altered the composition of life on Earth.
- These bacteria use a variety of pigments, such as chlorophyll, carotenoids, and phycobilins, to capture light energy for photosynthesis. Unlike other bacteria, cyanobacteria have internal membranes called thylakoids, where photosynthesis takes place. They are also capable of fixing atmospheric nitrogen, which is essential for the growth of many organisms.
- Cyanobacteria exhibit a wide range of forms, from unicellular to filamentous and colonial. They are found in diverse environments, including freshwater, marine habitats, and terrestrial habitats like moist soils. Some species form symbiotic relationships with other organisms, such as lichen-forming fungi and haptophyte algae.
- In marine ecosystems, cyanobacteria are important primary producers and contribute significantly to global carbon and nitrogen cycles. They dominate phytoplankton assemblages and are particularly abundant in open oceans. The marine cyanobacteria Prochlorococcus and Synechococcus are among the smallest photosynthetic organisms and are responsible for a substantial amount of oxygen production on Earth.
- However, cyanobacteria can also have negative impacts. Certain species can form harmful algal blooms, leading to the disruption of aquatic ecosystems and the production of toxins known as cyanotoxins. These toxins pose a threat to human and animal health and can contaminate water supplies.
- In biotechnology, cyanobacteria have attracted interest due to their potential applications. They are studied as model organisms and have been explored for their ability to produce bioethanol, food colorings, dietary supplements, and other valuable products. Their metabolic capabilities and adaptation to extreme environments make them promising candidates for various biotechnological processes.
- Overall, cyanobacteria are globally distributed and play significant roles in the Earth’s ecosystems. They have shaped the planet’s atmosphere, contribute to biogeochemical cycles, and serve as a fundamental component of marine food webs. However, their proliferation as harmful algal blooms requires careful monitoring and management to safeguard aquatic environments and public health.
Definition of Cyanobacteria/Cyanobacteria definition
Cyanobacteria are photosynthetic bacteria that obtain energy through photosynthesis. They are often referred to as blue-green algae, although they are not classified as true algae. Cyanobacteria played a crucial role in the production of oxygen and the shaping of Earth’s atmosphere. They can be found in various aquatic and terrestrial environments and can form harmful algal blooms in certain conditions.
General Characteristics of Cyanobacteria
Cyanobacteria, a diverse group of photosynthetic bacteria, share several general characteristics that define their biology and behavior. These characteristics include:
- Prokaryotic Cells: Cyanobacteria are classified as prokaryotes, lacking a membrane-bound nucleus and other organelles typically found in eukaryotic cells.
- Photoautotrophs: Cyanobacteria are capable of photosynthesis, utilizing solar energy to synthesize their own food and generate organic compounds.
- Cell Wall: Cyanobacteria possess a cell wall consisting of peptidoglycan on the inner side and lipopolysaccharides on the outer side, often covered by a layer of fibrous material.
- Blue-Green Color: The pigments present in cyanobacteria, such as phycobilisomes and chlorophyll, give them a characteristic blue-green color, from which their name is derived.
- Photosynthetic Pigments: Cyanobacteria contain phycobilisomes, responsible for their blue-green pigmentation, as well as chlorophyll for photosynthesis. Some species lack phycobilisomes but have chlorophyll b.
- Photosynthetic Machinery: Phycobilisomes are embedded in the intracytoplasmic membranes called thylakoids, where they serve as light-harvesting antennae for cyanobacterial photosystems.
- Thylakoids: Cyanobacteria have separate compartments called thylakoids, involved in both photosynthesis and cellular respiration, playing a role in electron transport and energy conversion.
- Carboxysomes: Cyanobacteria possess carboxysomes, specialized microcompartments that concentrate CO2, enhancing the efficiency of the CO2-fixing enzyme RuBisCo.
- Cell Organization: Cyanobacteria can occur as single cells or form colonies, often arranged in filamentous structures. Examples of filamentous genera include Anabaena, Oscillatoria, and Spirulina.
- Specialized Cells: Filamentous cyanobacteria can differentiate into akinetes, spore-like structures resistant to harsh environmental conditions, and heterocysts, specialized cells capable of nitrogen fixation in anoxic environments.
- Nitrogen Fixation: Cyanobacteria, particularly those with heterocysts, are capable of fixing atmospheric nitrogen into a usable form, playing a crucial role in nitrogen cycling.
- Motility: While some cyanobacteria are nonmotile, others can move via gliding motility. Motile filaments, known as hormogonia, may break away to initiate new colonies.
- Gas Vesicles: Many planktonic cyanobacteria possess gas vesicles, buoyant structures that allow them to float toward light-rich surface waters or sink to avoid harmful UV radiation.
- Cyanobacterial Circadian Rhythm: Some cyanobacteria exhibit a circadian rhythm, an internal biological clock that influences their metabolic and behavioral patterns.
- Asexual Reproduction: Cyanobacteria primarily reproduce through binary fission, with some species capable of multiple fission to form specialized endospores called baeocytes.
These general characteristics contribute to the diverse ecological roles and adaptations exhibited by cyanobacteria in various environments, making them important contributors to global biogeochemical cycles and primary production.
Morphology of Cyanobacteria
Cyanobacteria display a wide range of morphological variations, encompassing unicellular, filamentous, and colonial forms. These variations are crucial in understanding their structure and functions within different environments. Here are some key aspects of cyanobacterial morphology:
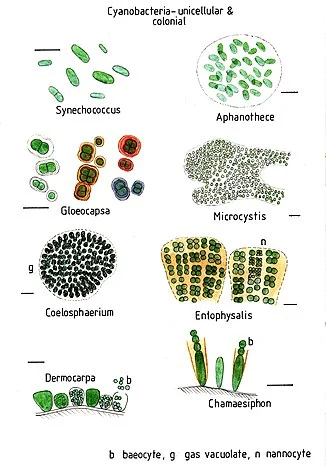
- Filamentous Forms: Many cyanobacteria exist as filamentous structures composed of chains of cells. These filaments can exhibit functional cell differentiation, which is considered an early form of multicellularity. Within the filaments, specialized cells such as heterocysts (for nitrogen fixation), akinetes (resting stage cells), and hormogonia (reproductive, motile filaments) can be observed. Heterocysts are involved in nitrogen fixation, akinetes serve as resistant spores in unfavorable conditions, and hormogonia are responsible for dispersal and colonization.
- Motile Filaments: Cyanobacteria often produce motile filaments called hormogonia, which can detach from the main biomass and form new colonies elsewhere. The cells within hormogonia are typically thinner than in the vegetative state, and the cells at each end of the motile chain may taper. To separate from the parent colony, a hormogonium may need to break apart a weaker cell in the filament known as a necridium.
- Morphological Diversity: Cyanobacteria exhibit a diverse range of morphologies. They can be unicellular or colonial, and their filamentous forms vary in branching patterns and cell differentiation. Some examples include:
- Unicellular: Species like Synechocystis and Synechococcus elongatus exist as individual cells.
- Non-heterocytous: Cyanobacteria such as Arthrospira maxima, Trichodesmium, and Phormidium do not possess heterocysts.
- False- or non-branching heterocytous: Nostoc and Brasilonema octagenarum exhibit heterocysts without branching.
- True-branching heterocytous: Stigonema species demonstrate true branching with heterocysts.
- Cell Types in Filamentous Species: Filamentous cyanobacteria can differentiate into distinct cell types:
- Vegetative cells: These are the typical photosynthetic cells that thrive under favorable conditions.
- Akinetes: When environmental conditions become harsh, cyanobacteria may form climate-resistant spores called akinetes.
- Thick-walled heterocysts: Heterocysts are specialized cells containing the enzyme nitrogenase, which is essential for nitrogen fixation. They have a thicker cell wall and function in an anaerobic environment due to their sensitivity to oxygen.
- Cell Structure: Individual cyanobacterial cells possess thick, gelatinous cell walls. Unlike many other microorganisms, cyanobacteria do not possess flagella for movement. However, some species can move by gliding along surfaces, facilitated by hormogonia. Certain multicellular filamentous forms, such as Oscillatoria, exhibit a waving motion, with the filament oscillating back and forth. Additionally, some cyanobacteria have the ability to float in water columns by forming gas vesicles, which are protein sheaths rather than lipid membranes.
Understanding the morphology of cyanobacteria provides insights into their adaptations, ecological functions, and interactions within their respective environments. It allows for a comprehensive exploration of their roles as primary producers, nitrogen fixers, and colonizers in various ecosystems.
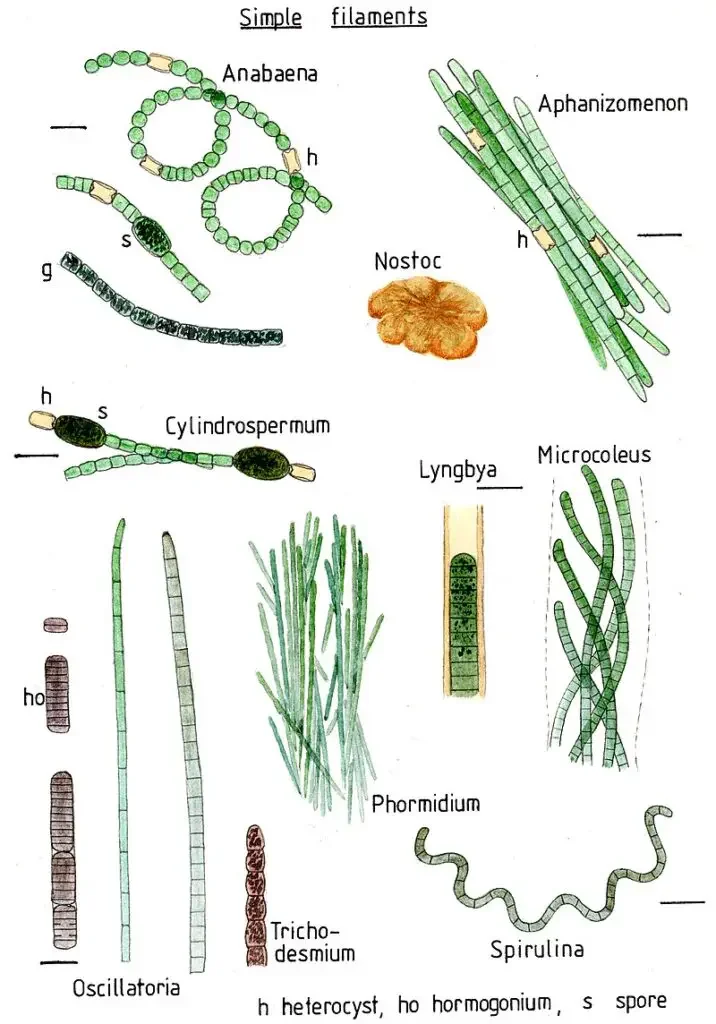
Types of cyanobacteria
There are numerous types of cyanobacteria, also known as blue-green algae, found in diverse environments worldwide. Cyanobacteria exhibit a wide range of morphological and physiological characteristics. Here are some examples of common cyanobacterial types:
- Oscillatoria: Oscillatoria is a filamentous cyanobacteria that forms long, unbranched chains of cells. These chains can oscillate or move in water, giving this genus its name. Oscillatoria is commonly found in freshwater habitats and can form slimy mats on the surface of ponds and lakes.
- Nostoc: Nostoc is a filamentous cyanobacteria that forms colonies composed of gelatinous masses. These colonies are often spherical or irregularly shaped and can be found in various aquatic and terrestrial environments, including soil, rocks, and symbiotic associations with plants.
- Anabaena: Anabaena is a filamentous cyanobacteria that forms heterocysts, specialized cells involved in nitrogen fixation. It can be found in freshwater habitats, such as ponds and rice fields. Anabaena can form visible blooms or floating mats on the water’s surface.
- Microcystis: Microcystis is a unicellular cyanobacteria that can form colonies or aggregates. It is known for its ability to produce toxins called microcystins, which can be harmful to aquatic organisms and pose risks to human health. Microcystis is commonly found in freshwater bodies, particularly in eutrophic environments.
- Synechococcus: Synechococcus is a unicellular cyanobacteria that is widespread in marine environments, ranging from coastal waters to open ocean regions. It is an important primary producer and plays a significant role in marine food webs. Synechococcus can exhibit various pigments, giving rise to different color variations.
- Prochlorococcus: Prochlorococcus is a small, unicellular cyanobacteria that is considered one of the most abundant photosynthetic organisms on Earth. It dominates in open ocean environments, particularly in nutrient-poor regions. Prochlorococcus is unique in that it contains chlorophyll b, similar to green algae, rather than the typical cyanobacterial pigment phycocyanin.
- Gloeocapsa: Gloeocapsa is a unicellular cyanobacteria that can form colonies surrounded by a gelatinous sheath. It is commonly found in soil, rocks, and other terrestrial habitats. Gloeocapsa colonies often appear as green or blackish patches on surfaces, such as roofs and stones.
These are just a few examples of the diverse types of cyanobacteria. There are many other genera and species with distinct characteristics and ecological niches.
Nitrogen fixation of Cyanobacteria
Nitrogen fixation is a crucial biological process performed by certain cyanobacteria, allowing them to convert atmospheric nitrogen gas (N2) into forms that are bioavailable to other organisms. Here are some key points about the nitrogen fixation of cyanobacteria:
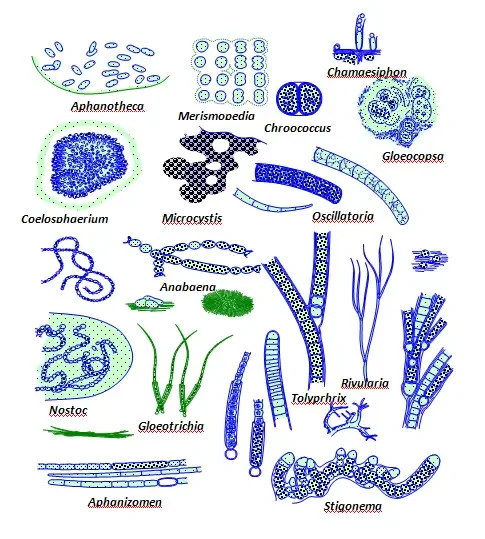
- Heterocysts: Cyanobacteria capable of nitrogen fixation have specialized cells called heterocysts. These cells are morphologically distinct from vegetative cells and are adapted to function in anaerobic conditions. Heterocysts have thick cell walls and lack oxygen-producing photosystems, creating an environment suitable for nitrogenase, the enzyme responsible for nitrogen fixation.
- Environmental Conditions: Heterocysts may form in cyanobacteria under appropriate environmental conditions, particularly when fixed nitrogen is scarce or when anaerobic conditions prevail. This adaptation allows cyanobacteria to respond to nitrogen limitations in their surroundings by initiating nitrogen fixation.
- Nitrogen Fixation Products: Heterocyst-forming cyanobacteria can convert atmospheric nitrogen gas into ammonia (NH3), nitrites (NO2−), or nitrates (NO3−). These nitrogen-containing compounds are then released into the surrounding environment, where they can be absorbed by plants. Plants can utilize these compounds to synthesize proteins and nucleic acids, essential for their growth and development. Atmospheric nitrogen is not directly usable by most plants, except those that have established symbiotic relationships with nitrogen-fixing bacteria, such as certain plants in the family Fabaceae.
- Free-Living Cyanobacteria: Free-living cyanobacteria are found in various environments, including the water of rice paddies. In these rice paddy ecosystems, cyanobacteria play a significant role in nitrogen fixation, enriching the soil with bioavailable nitrogen compounds. The presence of cyanobacteria helps provide a natural source of nitrogen to support the growth and productivity of rice plants.
- Symbiotic Relationships: Cyanobacteria can also form symbiotic relationships with other organisms to facilitate nitrogen fixation. An example is the association between certain cyanobacteria, such as Anabaena, and the aquatic fern Azolla. The cyanobacteria reside within specialized cavities in the Azolla plant, providing it with a source of fixed nitrogen. This mutualistic symbiosis benefits both the cyanobacteria and the host plant, as the cyanobacteria obtain a protected environment and a nutrient source, while the host plant receives a supply of bioavailable nitrogen.
The nitrogen fixation capability of cyanobacteria contributes to the global nitrogen cycle and is of significant ecological importance. By converting atmospheric nitrogen gas into usable forms, cyanobacteria play a vital role in providing bioavailable nitrogen to support the growth of other organisms, including plants, and contribute to the overall productivity and sustainability of ecosystems.
Cyanobionts
![Cyanobacteria - Definition, Characteristics, Structure, Functions, Examples 4 Symbiosis with land plants [96] Leaf and root colonization by cyanobacteria (1) Cyanobacteria enter the leaf tissue through the stomata and colonize the intercellular space, forming a cyanobacterial loop. (2) On the root surface, cyanobacteria exhibit two types of colonization pattern; in the root hair, filaments of Anabaena and Nostoc species form loose colonies, and in the restricted zone on the root surface, specific Nostoc species form cyanobacterial colonies. (3) Co-inoculation with 2,4-D and Nostoc spp. increases para-nodule formation and nitrogen fixation. A large number of Nostoc spp. isolates colonize the root endosphere and form para-nodules.](https://biologynotesonline.com/wp-content/uploads/2024/03/image-756.png)
Cyanobionts, also known as cyanobacterial symbionts, are cyanobacteria that engage in a symbiotic relationship with other organisms, including both unicellular and multicellular organisms.
One of the notable examples of cyanobionts is their interaction with land plants. Cyanobacteria can establish symbiosis with plants by entering through the stomata and colonizing the intercellular space. They form distinctive loops and intracellular coils within the plant tissue. For instance, Anabaena species can colonize the roots of wheat and cotton plants, while Calothrix species have been found on the root system of wheat. Nostoc species have been observed to colonize monocots like wheat and rice. Different types of association patterns have been identified, including loose colonization of root hairs by Anabaena and tight colonization of the root surface within a restricted zone by Nostoc.
Cyanobionts also exhibit symbiotic relationships with certain protists. This is particularly significant as some nitrogen-fixing cyanobacteria, called diazotrophs, play a crucial role in primary production, especially in nitrogen-limited oceans. Marine cyanobacteria, such as pico-sized Synechococcus and Prochlorococcus, are widely distributed and represent the most abundant photosynthetic organisms on Earth. They contribute significantly to carbon fixation in marine ecosystems. However, certain cyanobionts focus on nitrogen fixation rather than carbon fixation in their host organisms. The physiological functions of most cyanobionts are still not fully understood. Cyanobionts have been identified in various protist groups, including dinoflagellates, tintinnids, radiolarians, amoebae, diatoms, and haptophytes. The nature of the symbiosis, such as genetic diversity, host or cyanobiont specificity, and cyanobiont seasonality, remains largely unexplored, particularly in relation to dinoflagellate hosts.
The symbiotic relationship between cyanobionts and land plants involves leaf and root colonization by cyanobacteria. In leaves, cyanobacteria enter the tissue through stomata and colonize the intercellular space, forming characteristic cyanobacterial loops. On the root surface, cyanobacteria exhibit two colonization patterns: loose colonies of Anabaena and Nostoc species form in the root hairs, while specific Nostoc species form cyanobacterial colonies in a restricted zone on the root surface. Co-inoculation with certain substances can enhance para-nodule formation and nitrogen fixation in the root system. Nostoc species are frequently found colonizing the root endosphere and forming para-nodules.
Overall, cyanobionts play important roles in symbiotic associations with various organisms, contributing to nitrogen fixation, primary production, and the ecological dynamics of their hosts. Further research is needed to unravel the complexities of cyanobiont symbiosis and its implications for different ecosystems.
Cyanobacteria Photosynthesis
Photosynthesis is a vital process for cyanobacteria, enabling them to utilize sunlight as an energy source to synthesize organic compounds from carbon dioxide. Here are some key aspects of the photosynthesis of cyanobacteria:
- Carbon Fixation: Cyanobacteria employ various strategies, collectively known as a “CO2 concentrating mechanism,” to acquire inorganic carbon (CO2 or bicarbonate) from their aquatic environment. One prevalent strategy involves the use of carboxysomes, which are bacterial microcompartments. Carboxysomes work in conjunction with CO2 and bicarbonate transporters to accumulate bicarbonate within the cell’s cytoplasm. These icosahedral structures, composed of shell proteins, create an optimal environment for the CO2-fixing enzyme RuBisCO and carbonic anhydrase, enhancing local CO2 concentrations and improving the efficiency of RuBisCO.
- Electron Transport: Cyanobacteria have thylakoid membranes that are distinct compartments separate from the plasma membrane. The photosynthetic machinery is embedded in these thylakoid membranes, with phycobilisomes acting as light-harvesting antennae. Phycobilisomes capture light in the green spectrum (wavelengths from 450 nm to 660 nm), giving cyanobacteria their characteristic green pigmentation. High-energy electrons derived from water are primarily used by cyanobacterial cells for their own energy needs, but a fraction of these electrons may be released to the external environment through electrogenic activity.
- Respiration: Cyanobacteria can carry out respiration alongside photosynthesis in their thylakoid membranes. While photosynthesis builds carbohydrates from CO2, respiration reverses this process, breaking down carbohydrates into CO2 and releasing energy. Cyanobacteria separate these two processes spatially, with components of the respiratory chain located in the plasma membrane and an interconnected respiratory and photosynthetic electron transport chain present in the thylakoid membrane. Cyanobacteria utilize electrons from succinate dehydrogenase for respiration instead of NADPH.
- Day-Night Switch: Cyanobacteria only respire during the night or in the dark. This is because the facilities used for electron transport are utilized in reverse for photosynthesis when exposed to light. The plasma membrane is responsible for respiration, while the thylakoid membrane hosts both respiratory and photosynthetic electron transport.
- Electron Transport Chain: Cyanobacteria possess the ability to reduce nitrogen and carbon dioxide under aerobic conditions, contributing to their evolutionary and ecological success. Water-oxidizing photosynthesis occurs through the coupling of photosystem II and I (Z-scheme). Phycobilisomes, attached to the thylakoid membrane, serve as light-harvesting antennae for the photosystems. Phycobilisome components, such as phycobiliproteins, give cyanobacteria their blue-green pigmentation. Some cyanobacteria exhibit color variations based on the light they are exposed to, utilizing complementary chromatic adaptation to optimize photosynthesis.
- Metabolism: Cyanobacteria primarily utilize water as an electron donor for photosynthesis, resulting in the production of oxygen as a byproduct. They also reduce carbon dioxide to form carbohydrates through the Calvin cycle. Cyanobacteria played a significant role in increasing atmospheric oxygen levels through their oxygen-generating activities. Additionally, they form symbiotic relationships with various organisms such as fungi, corals, pteridophytes, and angiosperms. Some cyanobacteria can exhibit heterotrophic growth, while others can be parasitic, causing diseases in invertebrates or algae.
Photosynthesis in cyanobacteria is a fundamental process that sustains their growth and provides oxygen and organic compounds essential for the functioning of ecosystems. Their diverse strategies for carbon fixation, electron transport, and metabolic adaptations contribute to their ecological success and impact on the global carbon and nitrogen cycles.
Movement of Cyanobacteria
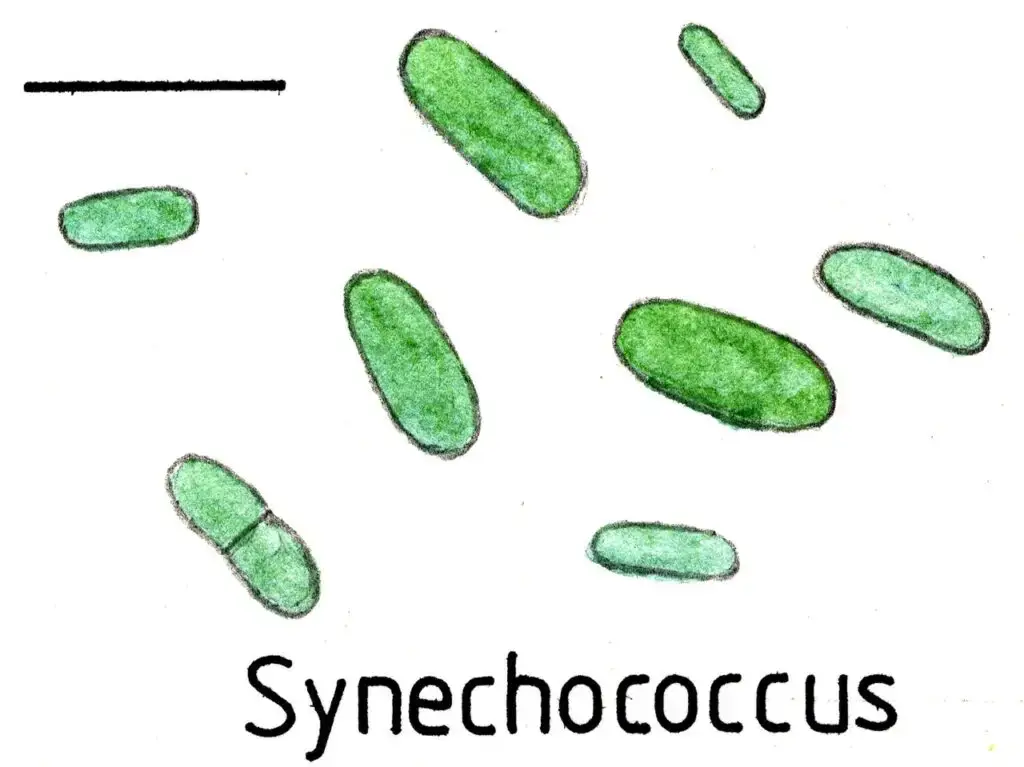
Cyanobacteria exhibit various types of movement that allow them to navigate their environment and optimize their exposure to light. Here are some key aspects of cyanobacterial movement:
- Type IV Pili and Surface Motions: Filamentous cyanobacteria utilize type IV pili to perform surface motions. These movements are characterized by the formation of waves on the surface of the cyanobacterial filaments, which generate backward water flow. This mechanism contributes to the repositioning of cyanobacteria in their habitat.
- Swimming Mechanism: Certain marine cyanobacteria, such as Synechococcus, are capable of swimming independently. They achieve this by employing a mechanism distinct from bacterial flagella. The exact details of this swimming mechanism are still under investigation.
- Gliding: Many species of cyanobacteria exhibit gliding motility, which allows them to move across surfaces. Gliding is different from crawling or swimming as it does not involve obvious external organs or changes in cell shape. Instead, it relies on the presence of a substrate. Filamentous cyanobacteria employ a “slime jet” mechanism for gliding. They extrude a gel-like substance that rapidly expands upon hydration, propelling the cells forward. Some unicellular cyanobacteria use type IV pili for gliding.
- Photomovement: Cyanobacteria possess strict light requirements and exhibit photomovement to optimize their exposure to light. Photomovement can be categorized into three types: photokinesis, phototaxis, and photophobic responses.
- Photokinetic movement involves modulating the gliding speed in response to changes in incident light intensity. Cyanobacteria like Phormidium autumnale increase their gliding speed linearly with higher light intensity.
- Phototactic movement refers to the directional movement of cyanobacteria in response to the light source. Positively phototactic species tend to move parallel to the light and towards the light source. Some cyanobacteria rely on random collisions to orient themselves towards the light, while others can steer by bending their trichomes.
- Photophobic responses occur when cyanobacteria respond to spatial and temporal light gradients. Step-up photophobic reactions involve reversing direction upon entering a brighter area from a darker one, avoiding intense light. Step-down reactions occur when cyanobacteria enter a dark area from a bright one and reverse direction to remain in the light.
The migration of filamentous cyanobacteria within microbial mats is driven by their need to balance light requirements for photosynthesis and sensitivity to photodamage. They vertically and horizontally move within the mat to find optimal niches that meet their light needs. Photomovement plays a significant role in these migrations, although other forms of taxis may also contribute.
Overall, cyanobacteria display diverse movement strategies that enable them to adapt to their environment, optimize their exposure to light, and ensure their survival and growth.
Genetics of Cyanobacteria
The genetics of cyanobacteria encompasses their ability for natural genetic transformation and the mechanisms they employ to repair DNA damage. Here are some key aspects of the genetics of cyanobacteria:
- Natural Genetic Transformation: Cyanobacteria are capable of natural genetic transformation, which refers to the uptake and incorporation of exogenous DNA from their surroundings, resulting in genetic alteration. For transformation to occur, the recipient bacteria must be in a state of competence. In nature, competence can be induced by conditions such as starvation, high cell density, or exposure to DNA damaging agents. This process allows cyanobacteria to acquire new genetic material and incorporate it into their genome.
- Chromosomal Transformation and Homologous Recombination: In chromosomal transformation, homologous transforming DNA can integrate into the recipient cyanobacterial genome through a process called homologous recombination. Homologous recombination involves the exchange of genetic material between homologous DNA sequences. This mechanism is thought to be an adaptation for repairing DNA damage in cyanobacteria. By integrating homologous DNA into their genome, cyanobacteria can repair damaged DNA and maintain genomic stability.
- DNA Repair: Cyanobacteria face various environmental stresses and reactive oxygen species that can cause DNA damage. To counteract these challenges, cyanobacteria possess a repertoire of DNA repair genes, similar to those found in Escherichia coli (E. coli). The presence of conserved DNA repair genes in cyanobacteria, even in small genomes, suggests that core DNA repair processes such as recombinational repair, nucleotide excision repair, and methyl-directed DNA mismatch repair are common among cyanobacteria. These repair mechanisms play a crucial role in maintaining the integrity of the cyanobacterial genome and ensuring their survival in the face of DNA damage.
The genetics of cyanobacteria not only involve their capability for natural genetic transformation but also encompass the mechanisms they employ to repair DNA damage. These genetic processes contribute to the adaptability and survival of cyanobacteria in diverse environments, enabling them to respond to changing conditions and maintain genetic stability.
Classification of Cyanobacteria
The classification of Cyanobacteria has undergone changes over time due to advancements in scientific understanding and the development of newer taxonomic systems. Initially, Cyanobacteria was classified as Cyanophyta within the five-kingdom scheme of classification, specifically as a phylum of Kingdom Protista. In this scheme, Cyanophyta was grouped together with other phyla such as Euglenophyta, Chrysophyta, Pyrrophyta, Chlorophyta, Phaeophyta, and Rhodophyta.
Cyanophyta was considered a plant-like protist due to its photosynthetic ability, a feature commonly associated with plants. However, unlike true plants (embryophytes), Cyanophyta lacked structures like roots, stems, and leaves.
In more recent years, taxonomic positions have been revised based on new studies and findings. As a result, Cyanophyta, or blue-green algae, is now more commonly referred to as Cyanobacteria, which is classified as a phylum of bacteria. Cyanobacteria are recognized as prokaryotic organisms, lacking a membrane-bound nucleus and other organelles typically found in eukaryotes.
In the field of phycology (the study of algae), Cyanophyta may still be considered an algal group and the phylum to which all blue-green algae belong. However, it is important to note that Cyanophyta is the only group of algae that consists of prokaryotic organisms. The remaining algal groups are eukaryotes.
These changes in classification reflect the evolving understanding of Cyanobacteria’s biology and their relationships with other organisms. The recognition of Cyanobacteria as bacteria highlights their unique characteristics and distinguishes them from eukaryotic algae.
Taxonomy and Sub-groups of Cyanobacteria
The taxonomy of Cyanobacteria (Cyanophyta) includes several major taxonomic orders that help classify and organize these organisms. The following are the main taxonomic orders within the phylum Cyanobacteria:
- Chroococcales: This is a polyphyletic group that consists of cyanobacteria with various shapes such as coccoid, cylindrical, or rod-shaped. They have the ability to form colonies as dense masses on moist rocks and surfaces. Examples of genera within this order include Microcystis, Pleurocapsa, Gloeocapsa, and Aphanocapsa.
- Pleurocapsales: This polyphyletic group comprises coccoid spore-forming cyanobacteria. They have been observed to divide by multiple fissions, producing endospores called baeocytes. Genera within this order include Pleurocapsa, Odorella, and Dermocarpella.
- Oscillatoriales: This polyphyletic group consists of uniseriate filamentous cyanobacteria that do not form akinetes, heterocysts, or true branching. Examples of genera in this order are Oscillatoria, Phormidium, and Lyngbya.
- Nostocales: This monophyletic group is capable of forming heterocysts, akinetes, heterogenous trichomes, and false branching. Genera within Nostocales include Anabaena, Nostoc, Richelia, and Calothrix.
- Stigonematales: Similar to Nostocales, this monophyletic group can form heterocysts, akinetes, and heterogenous trichomes. However, Stigonematales can also form true branched trichomes. Examples of genera in this order include Stigonema and Mastigocladus.
These taxonomic orders help categorize Cyanobacteria based on their general features, such as morphology, cellular characteristics, and reproductive structures. It is worth noting that the traditional classification of Cyanobacteria into five sections (Chroococcales, Pleurocapsales, Oscillatoriales, Nostocales, and Stigonematales) is not fully supported by recent phylogenetic studies. Nevertheless, Nostocales and Stigonematales are recognized as monophyletic groups, particularly because they encompass the heterocystous cyanobacteria.
The taxonomy and classification of Cyanobacteria have evolved over time, and the current accepted taxonomy is based on the List of Prokaryotic Names with Standing in Nomenclature (LPSN) and the National Center for Biotechnology Information (NCBI) databases. It is important to note that not all taxa within the phylum Cyanobacteria have been officially published under the International Code of Nomenclature of Prokaryotes (ICNP).
Overall, understanding the taxonomic orders helps scientists categorize and study Cyanobacteria more effectively, enabling further exploration of their ecological roles, physiology, and evolutionary relationships.
Evolution of Cyanobacteria
The evolution of cyanobacteria has played a significant role in shaping Earth’s history and the development of life on our planet. Cyanobacteria are ancient organisms that have existed for billions of years, and their evolutionary journey provides important insights into the origins of photosynthesis and the establishment of complex ecosystems.
Cyanobacteria are believed to have emerged during the Archean Eon, around 3.5 billion years ago. These single-celled organisms were among the earliest life forms on Earth and were responsible for the production of oxygen through photosynthesis. The rise of cyanobacteria and the subsequent increase in atmospheric oxygen had a profound impact on the planet, leading to the development of aerobic organisms and fundamentally altering the composition of the atmosphere.
Stromatolites, layered structures formed by cyanobacteria in shallow water, provide some of the earliest evidence of life on Earth. These structures, composed of sedimentary grains bound together by biofilms of cyanobacteria, date back as far as 3.5 billion years. Stromatolites grew in various environments, both marine and non-marine, and offer a glimpse into the diverse habitats where cyanobacteria thrived.
The evolution of cyanobacteria is closely tied to the evolution of photosynthesis. Oxygenic photosynthesis, the process by which cyanobacteria and later photosynthetic eukaryotes convert sunlight into energy while producing oxygen, is believed to have originated only once in cyanobacteria. All photosynthetic eukaryotes, including plants and algae, acquired this ability from cyanobacteria or their descendants. The oxygen produced by cyanobacteria paved the way for the development of aerobic organisms and the diversification of life on Earth.
During the Proterozoic Eon, cyanobacteria remained the primary producers in many ecosystems. They played a crucial role in nitrogen fixation, contributing to the redox structure of the oceans and the availability of nutrients for other organisms. While green algae joined cyanobacteria as major primary producers in marine environments, it was not until the Mesozoic Era that primary production in marine shelf waters took its modern form with the radiations of dinoflagellates, coccolithophorids, and diatoms.
The origin of chloroplasts, specialized organelles found in photosynthetic eukaryotes, is believed to have resulted from endosymbiotic events between ancestral cyanobacteria and early eukaryotic cells. Chloroplasts share many similarities with cyanobacteria, including a circular chromosome and prokaryotic-type ribosomes. The endosymbiotic theory suggests that photosynthetic bacteria were engulfed by early eukaryotic cells, eventually evolving into chloroplasts. This theory is supported by phylogenetic, genomic, biochemical, and structural evidence.
The marine environment has played a significant role in the evolution of cyanobacteria. These organisms have had a profound impact on global geochemistry, particularly in terms of oxygen levels and biogeochemical cycles. The Great Oxidation Event, which marked a significant increase in atmospheric oxygen, is believed to be the result of cyanobacteria activity. Other intervals of environmental change, such as the Neoproterozoic Oxygenation Event, also had connections to cyanobacteria evolution. Understanding the evolution of cyanobacteria in marine environments is crucial for unraveling the complex interactions between these organisms and the Earth’s ecosystems.
In conclusion, the evolution of cyanobacteria has been a key driver in Earth’s history. From their emergence in the ancient oceans to their role in oxygen production, nitrogen fixation, and the development of complex ecosystems, cyanobacteria have shaped the planet’s biology and geochemistry. Studying their evolutionary journey provides valuable insights into the origins of life and the interplay between organisms and their environment.
Cyanobacteria toxins
Cyanobacteria are capable of producing various toxins, which are commonly referred to as cyanotoxins. These toxins can pose a threat to human and animal health as well as the environment. Here are some examples of cyanobacterial toxins:
- Microcystins: Microcystins are one of the most well-known and widely studied cyanotoxins. They are hepatotoxins, meaning they can cause liver damage. Microcystins can be produced by several cyanobacterial genera, including Microcystis, Planktothrix, and Anabaena.
- Anatoxins: Anatoxins are neurotoxins produced by certain cyanobacterial species, such as Anabaena and Aphanizomenon. They can affect the nervous system and can be lethal to animals if ingested.
- Cylindrospermopsin: Cylindrospermopsin is a toxin produced by various cyanobacteria, including Cylindrospermopsis, Aphanizomenon, and Raphidiopsis. It can cause liver damage, kidney toxicity, and other health issues.
- Saxitoxins: Saxitoxins are potent neurotoxins produced by some cyanobacterial species, such as Anabaena, Aphanizomenon, and Cylindrospermopsis. They are also associated with harmful algal blooms (HABs) and can cause paralytic shellfish poisoning (PSP) in humans if contaminated shellfish are consumed.
- Lyngbyatoxins: Lyngbyatoxins are cytotoxic toxins produced by certain cyanobacterial species, including Lyngbya and Phormidium. They can have harmful effects on cells and can cause skin irritations and other health problems.
It is important to note that the production of cyanotoxins can be influenced by various factors such as environmental conditions, nutrient availability, and the specific cyanobacterial species present. Exposure to cyanobacterial toxins can occur through ingestion of contaminated water or food, inhalation of aerosols, or direct contact with blooms. Monitoring and managing cyanobacterial blooms are essential to mitigate the risks associated with cyanotoxins.
Ecological Importance of Cyanobacteria
Cyanobacteria, often referred to as blue-green algae, play a significant ecological role in various ecosystems. Their importance stems from their diverse abilities and contributions to the environment. Here are some key ecological aspects in which cyanobacteria have an impact:
- Phytoplankton and Primary Production: Cyanobacteria are part of the phytoplankton community, alongside microalgae, in aquatic ecosystems. Phytoplankton, including cyanobacteria, are vital primary producers. Through photosynthesis, they convert sunlight and nutrients into organic matter, serving as a fundamental food source for other organisms within the ecosystem. From zooplankton to large marine animals like blue whales, many rely on phytoplankton for sustenance.
- Cyanobacterial Blooms: Under favorable conditions, cyanobacteria can experience exponential growth, leading to the formation of algal blooms. These blooms are commonly observed in warm, stagnant, or slowly flowing aquatic environments that accumulate high concentrations of nutrients like phosphorus and nitrogen. Cyanobacterial blooms can occur in both freshwater and marine environments, often appearing as scum-like, blue-green accumulations. While some cyanobacteria species are harmless, others produce toxic secondary metabolites known as cyanotoxins. These toxins can be detrimental to fish, animals, and even humans, posing risks to their nervous system, liver, and skin when ingested.
- Nitrogen Fixation: Certain cyanobacteria, such as Anabaena species, possess specialized cells called heterocysts, which enable them to fix atmospheric nitrogen. Nitrogen-fixing cyanobacteria convert atmospheric nitrogen into forms (e.g., ammonia, nitrites, or nitrates) that are readily usable by other organisms, including plants and animals. This process establishes symbiotic relationships with various organisms, contributing to the nitrogen cycle and nutrient availability in ecosystems. In rice paddies, nitrogen-fixing cyanobacteria can be utilized as an alternative to chemical nitrogen fertilizers.
- Soil Stabilization: Cyanobacteria also play a crucial role in terrestrial habitats by stabilizing soils. Species like Microcoleus vaginatus produce polysaccharide sheaths that bind soil particles together, preventing erosion and aiding in soil moisture retention. Their growth helps maintain soil structure and stability, especially in damp soil environments.
- Oxygen Production: Cyanobacteria significantly contribute to the oxygen cycle through their oxygenic photosynthesis. Prochlorococcus species, for instance, are responsible for a substantial portion of oxygen production in the open ocean. These tiny cyanobacteria are adept at photosynthesis and have a notable impact on maintaining oxygen levels, supporting the survival of various organisms in marine ecosystems.
- Heterotrophy and Disease: While most cyanobacteria are photosynthetic, some have the ability to become heterotrophic. Heterotrophic cyanobacteria can obtain nutrients by consuming organic matter. However, certain parasitic cyanobacteria can cause diseases in their invertebrate hosts, such as black band disease, highlighting their role in the dynamics of coral reef ecosystems.
Overall, cyanobacteria’s ecological importance is evident in their contributions to primary production, nutrient cycling, soil stabilization, oxygen production, and their complex interactions within diverse ecosystems. Understanding their roles and dynamics is crucial for preserving the balance and health of these ecosystems and mitigating the potential risks associated with cyanobacterial blooms and toxins.
Cyanobacteria Examples
Here are some examples of cyanobacteria:
- Anabaena: Anabaena is a filamentous cyanobacterium that forms long chains of cells. It is known for its ability to perform nitrogen fixation and is commonly found in freshwater environments.
- Microcystis: Microcystis is a genus of cyanobacteria that can form harmful algal blooms (HABs). Some species of Microcystis produce toxins called microcystins, which can be harmful to aquatic organisms and humans.
- Nostoc: Nostoc is a genus of filamentous cyanobacteria that can form colonies or gelatinous masses called “cyanobacterial mats.” They are found in various terrestrial habitats such as soil, rocks, and tree trunks.
- Spirulina: Spirulina is a well-known cyanobacterium that is commercially cultivated for its high protein content. It is used as a nutritional supplement and has gained popularity as a health food due to its rich nutrient profile.
- Synechococcus: Synechococcus is a unicellular cyanobacterium that is commonly found in marine environments. It is an important primary producer in oceans and contributes to the marine food web.
- Prochlorococcus: Prochlorococcus is another marine cyanobacterium that is considered one of the most abundant photosynthetic organisms on Earth. It is extremely small and plays a significant role in global carbon and oxygen cycles.
- Gloeocapsa: Gloeocapsa is a genus of cyanobacteria that forms colonies embedded in a gelatinous matrix. It is often found as green or blackish patches on rocks, trees, and other surfaces.
- Oscillatoria: Oscillatoria is a filamentous cyanobacterium that forms long, unbranched chains of cells. It is commonly found in freshwater environments and can produce toxins under certain conditions.
- Lyngbya: Lyngbya is a filamentous cyanobacterium that forms dense mats in freshwater and marine environments. Some species of Lyngbya produce toxins that can harm aquatic organisms and impact ecosystem health.
- Aphanizomenon: Aphanizomenon is a genus of filamentous cyanobacteria that can form blooms in freshwater lakes and ponds. Some species produce toxins and contribute to the occurrence of HABs.
These are just a few examples of the wide diversity of cyanobacteria found in various habitats around the world.
What eats cyanobacteria?
Several organisms consume cyanobacteria as part of their diet. Here are some examples of organisms that commonly feed on cyanobacteria:
- Zooplankton: Small aquatic animals, such as rotifers, copepods, and cladocerans, are known to graze on cyanobacteria. These zooplankton organisms play an important role in controlling the population of cyanobacteria in freshwater ecosystems.
- Filter-Feeding Invertebrates: Certain filter-feeding invertebrates, such as freshwater mussels and some species of sponges, can consume cyanobacteria. They extract suspended particles, including cyanobacterial cells, from the water as they filter-feed.
- Protists: Various protists, including amoebas, ciliates, and flagellates, can consume cyanobacteria. These single-celled organisms feed on cyanobacterial cells as a source of nutrition.
- Insects and Larvae: Some insects, particularly aquatic insects, may feed on cyanobacteria. For example, certain species of mosquito larvae and midge larvae can consume cyanobacterial cells.
- Fish: Some fish species, such as tilapia, silver carp, and certain species of herbivorous fish, can consume cyanobacteria. They may feed on cyanobacterial mats or actively graze on suspended cyanobacterial cells.
It is worth noting that the consumption of cyanobacteria by organisms can vary depending on factors such as the specific cyanobacterial species, the availability of alternative food sources, and the ecological dynamics of the ecosystem. Additionally, some organisms may selectively consume certain types of cyanobacteria while avoiding those that produce toxins.
Cyanobacteria fossil
Cyanobacteria, also known as blue-green algae, have a long history dating back billions of years. Fossil evidence of cyanobacteria provides valuable insights into their evolutionary history and their role in shaping the Earth’s ecosystems. Here are some key points about cyanobacteria fossils:
- Stromatolites: Stromatolites are layered sedimentary structures formed by the growth of cyanobacteria in shallow aquatic environments. These structures are one of the earliest forms of life on Earth and can be found in fossil records dating back around 3.5 billion years. Stromatolite fossils provide evidence of cyanobacteria’s ancient existence and their role in creating oxygen-rich environments.
- Microfossils: Microscopic fossils of cyanobacteria have been discovered in various rock formations. These microfossils include filamentous structures and cell-like shapes preserved in ancient sediments. They provide evidence of the diverse forms of cyanobacteria that existed throughout geological time.
- Cyanobacterial Mats: Fossilized cyanobacterial mats, known as microbialites, have been found in different geological formations. These mats consist of layered structures created by the growth and trapping of cyanobacteria. They offer insights into the ecological interactions and environmental conditions of ancient cyanobacterial communities.
- Preservation: Cyanobacteria fossils are often preserved in sedimentary rocks such as limestone, shale, and chert. The fossilization process involves the entombment and mineralization of cyanobacterial remains, which helps in their long-term preservation and eventual discovery by paleontologists.
- Cyanobacteria and Oxygen Evolution: Fossil evidence of cyanobacteria is crucial in understanding the history of oxygen evolution on Earth. Cyanobacteria played a significant role in the Great Oxygenation Event, around 2.4 billion years ago, when their photosynthetic activity led to the oxygenation of Earth’s atmosphere, profoundly influencing the subsequent evolution of life.
Studying cyanobacteria fossils provides scientists with important information about early life on Earth, the development of ecosystems, and the impact of cyanobacteria on our planet’s geological and biological history.
When did cyanobacteria start producing pure oxygen?
Cyanobacteria, also known as blue-green algae, played a significant role in the history of Earth’s atmosphere by producing oxygen through photosynthesis. The process of oxygen production by cyanobacteria, known as oxygenic photosynthesis, began approximately 2.7 billion years ago during the Proterozoic Eon. This marked the first instance of significant oxygen accumulation in the Earth’s atmosphere, leading to the Great Oxygenation Event (GOE). The GOE, which occurred over a span of hundreds of millions of years, transformed the atmosphere from oxygen-poor to oxygen-rich, paving the way for the development of oxygen-dependent life forms.
Do cyanobacteria have chloroplasts?
No, cyanobacteria do not have chloroplasts. Chloroplasts are specialized organelles found in eukaryotic algae and plants that are responsible for carrying out photosynthesis. Cyanobacteria, on the other hand, are prokaryotic organisms and lack membrane-bound organelles like chloroplasts.
However, it is worth noting that cyanobacteria played a crucial role in the evolution of chloroplasts. It is believed that chloroplasts originated from an ancient endosymbiotic event where a free-living cyanobacterium was engulfed by a eukaryotic cell, establishing a symbiotic relationship. Over time, the cyanobacterium evolved into a chloroplast, retaining its ability to carry out photosynthesis. This endosymbiotic event is thought to be responsible for the presence of chloroplasts in algae and plants.
Algae vs Cyanobacteria
Algae and cyanobacteria are both photosynthetic organisms, but they differ in several important aspects. Here are some key differences between algae and cyanobacteria:
- Cellular Structure: Algae are eukaryotic organisms, meaning their cells have a nucleus and other membrane-bound organelles. In contrast, cyanobacteria are prokaryotic organisms, lacking a nucleus and membrane-bound organelles. Cyanobacteria have a simpler cellular structure compared to algae.
- Pigments: Algae contain various pigments, including chlorophylls a and b, as well as accessory pigments like carotenoids and phycobiliproteins. These pigments allow algae to exhibit a wide range of colors, from green to brown, red, and even blue. Cyanobacteria also contain chlorophyll a, but they lack chlorophyll b. Instead, cyanobacteria have unique pigments called phycobilins, which give them a bluish-green color.
- Habitat: Algae can be found in diverse habitats, including freshwater, marine environments, soil, and even symbiotic associations with other organisms. They are commonly found in both aquatic and terrestrial ecosystems. Cyanobacteria are also widespread and can inhabit a variety of environments, including freshwater, marine, and terrestrial habitats. They are particularly well-known for their ability to thrive in extreme conditions like hot springs and deserts.
- Cellular Organization: Algae can exist as single-celled organisms, such as Chlorella and diatoms, or as multicellular organisms, such as seaweeds and kelps. They may have specialized tissues and structures for different functions. Cyanobacteria, on the other hand, are mostly unicellular or filamentous, forming chains or colonies. While some cyanobacteria may form structures like heterocysts for nitrogen fixation, they do not have the same level of cellular differentiation as algae.
- Reproduction: Algae exhibit various modes of reproduction, including both asexual and sexual reproduction. They can reproduce through cell division, fragmentation, or the formation of spores or gametes. Cyanobacteria also reproduce asexually through cell division, forming identical daughter cells. Some cyanobacteria can also undergo specialized forms of reproduction, such as akinetes or hormogonia, which aid in survival and dispersal.
- Ecological Impact: Algae play crucial ecological roles as primary producers, oxygen producers, and as a food source for many organisms in aquatic ecosystems. They also contribute to carbon dioxide sequestration and nutrient cycling. Cyanobacteria, despite being prokaryotes, perform similar functions as primary producers and oxygen producers through photosynthesis. However, some cyanobacteria can form harmful algal blooms and produce toxins that can be harmful to the environment and human health.
Overall, while algae and cyanobacteria share some similarities in their photosynthetic nature, they differ in terms of cellular structure, pigments, habitat, cellular organization, reproduction, and ecological impact.
Aspect | Algae | Cyanobacteria |
---|---|---|
Cellular Structure | Eukaryotic | Prokaryotic |
Pigments | Chlorophylls a and b, carotenoids, phycobiliproteins | Chlorophyll a, phycobilins |
Habitat | Freshwater, marine, soil, symbiotic associations | Freshwater, marine, terrestrial |
Cellular Organization | Unicellular or multicellular | Mostly unicellular or filamentous |
Reproduction | Asexual and sexual reproduction | Asexual (cell division), specialized forms of reproduction in some species |
Ecological Impact | Primary producers, oxygen producers, carbon dioxide sequestration, nutrient cycling, food source | Primary producers, oxygen producers, potential for harmful algal blooms and toxin production |
Cyanobacteria Function
Cyanobacteria serve several important functions in various ecosystems. Here are some key functions of cyanobacteria:
- Photosynthesis: Cyanobacteria are primary producers that carry out photosynthesis, using sunlight to convert carbon dioxide and water into organic compounds. They play a crucial role in the production of oxygen and the cycling of carbon in aquatic and terrestrial environments.
- Nitrogen Fixation: Certain species of cyanobacteria have the ability to fix atmospheric nitrogen into a form usable by other organisms. They convert nitrogen gas into ammonia or other nitrogen compounds, enriching the soil and providing a vital nutrient for plants and other organisms.
- Food Source: Cyanobacteria are an essential food source for many aquatic organisms, including zooplankton, small fish, and invertebrates. They form the base of the food chain in freshwater and marine ecosystems, supporting higher trophic levels.
- Symbiotic Associations: Cyanobacteria form symbiotic relationships with various organisms. For example, they form mutualistic partnerships with lichens, where cyanobacteria provide a source of fixed carbon to the fungal partner. Cyanobacteria also establish symbiotic associations with some plants, such as cycads and hornworts, providing them with nitrogen.
- Oxygen Production: Through oxygenic photosynthesis, cyanobacteria are significant contributors to the production of oxygen in the Earth’s atmosphere. They played a critical role in the oxygenation of the planet billions of years ago, paving the way for the development of aerobic organisms.
- Carbon Cycling: Cyanobacteria participate in the global carbon cycle by assimilating carbon dioxide during photosynthesis and converting it into organic matter. They help regulate carbon dioxide levels in aquatic ecosystems and contribute to carbon sequestration.
- Ecological Engineering: Some cyanobacteria species form large colonies or mats that alter the physical and chemical properties of their environment. They can stabilize sediments, influence nutrient cycling, and create habitats for other organisms.
- Biotechnological Applications: Cyanobacteria are of great interest in biotechnology and bioengineering. They have potential applications in biofuel production, wastewater treatment, production of pharmaceuticals and bioactive compounds, and as model organisms for studying photosynthesis and microbial processes.
It’s important to note that while cyanobacteria provide numerous beneficial functions, certain species can also produce toxins under certain conditions, leading to harmful algal blooms and posing risks to human and ecosystem health.
Cyanobacteria Under Microscope
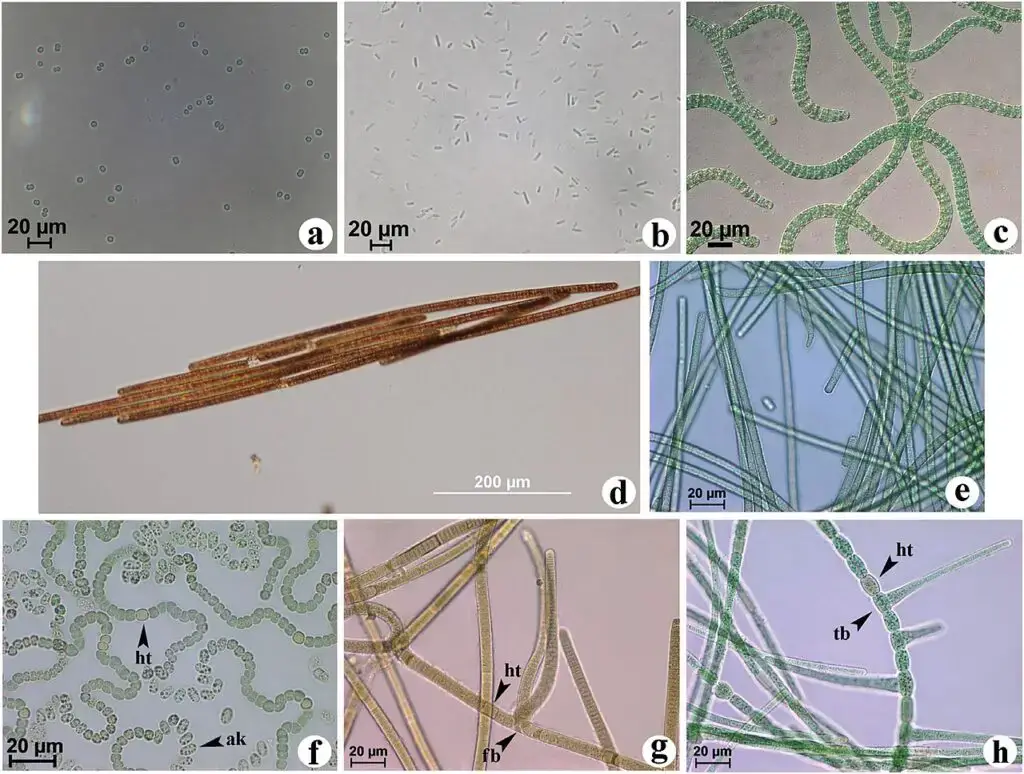
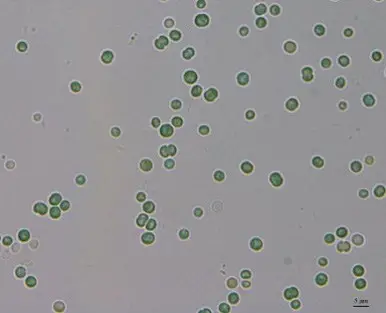
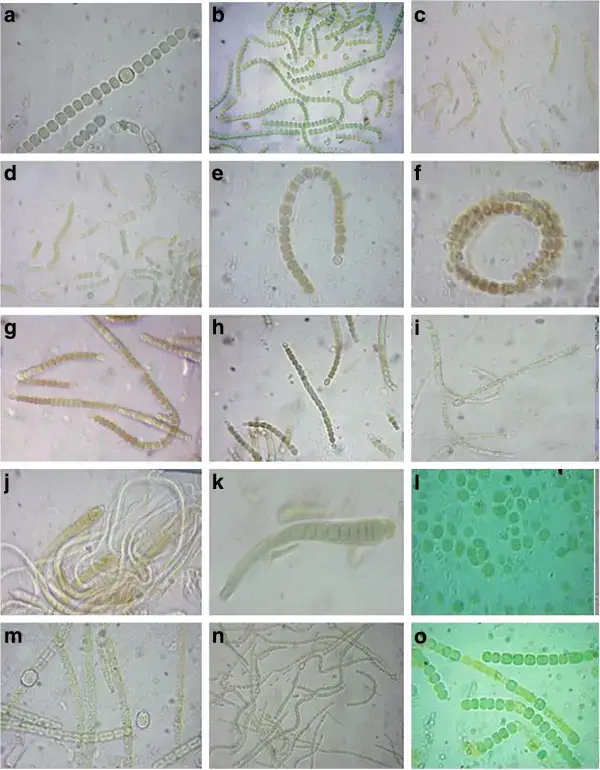
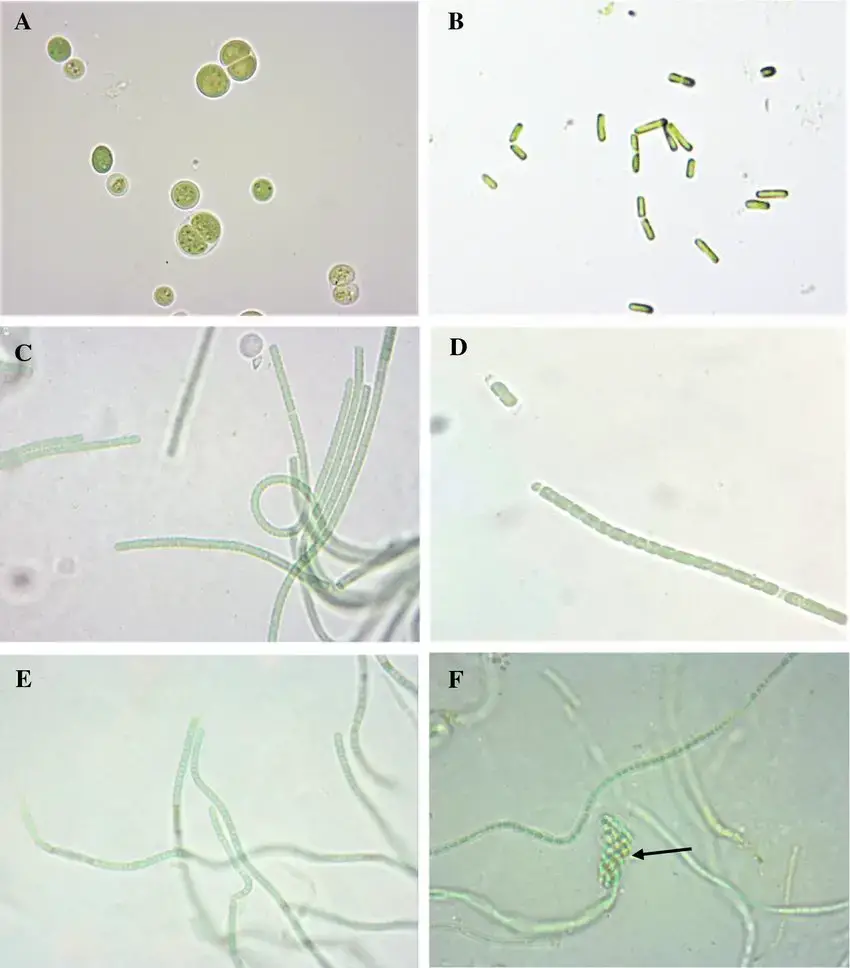
FAQ
What are cyanobacteria?
Cyanobacteria, also known as blue-green algae, are a group of photosynthetic bacteria that obtain energy through photosynthesis. They are found in diverse habitats, including freshwater, marine environments, and even terrestrial ecosystems.
What is the role of cyanobacteria in the environment?
Cyanobacteria play a crucial role in the environment as primary producers. Through photosynthesis, they convert sunlight and carbon dioxide into organic matter, releasing oxygen as a byproduct. They are also involved in nutrient cycling and can form symbiotic relationships with other organisms.
Can cyanobacteria be harmful?
Yes, some cyanobacteria can produce harmful toxins, known as cyanotoxins. When certain environmental conditions are favorable, cyanobacterial blooms can occur in bodies of water, leading to harmful algal blooms (HABs) that can have detrimental effects on aquatic ecosystems and pose risks to human and animal health.
How do cyanobacteria contribute to nitrogen fixation?
Certain cyanobacteria have the ability to fix atmospheric nitrogen into a form usable by plants and other organisms. They possess specialized cells called heterocysts that create an anaerobic environment for nitrogen fixation. This process plays a vital role in nitrogen cycling and enriches ecosystems with bioavailable nitrogen.
Can cyanobacteria move?
Yes, cyanobacteria can exhibit various forms of movement. Some species can glide on surfaces using specialized mechanisms, while others exhibit swimming motility. Movement in cyanobacteria helps them respond to environmental cues, find optimal light conditions, and explore nutrient-rich areas.
How do cyanobacteria cope with environmental stresses?
Cyanobacteria have developed several adaptive mechanisms to cope with environmental stresses. These include the production of protective pigments, such as phycobilins and carotenoids, that help absorb or dissipate excess light energy. They also possess DNA repair mechanisms to counteract DNA damage caused by environmental factors.
Can cyanobacteria be used as a source of biofuels?
Yes, cyanobacteria are being studied as potential sources of biofuels. Some species can accumulate high levels of lipids or starch, which can be converted into biofuels through processes like biodiesel production or anaerobic digestion.
Do cyanobacteria have any industrial or commercial applications?
Yes, cyanobacteria have various industrial and commercial applications. They are used in the production of food additives, biofertilizers, and high-value compounds like pigments and pharmaceuticals. They are also being explored for their potential in wastewater treatment and carbon capture technologies.
Are cyanobacteria related to algae?
Cyanobacteria are often referred to as blue-green algae, but they are not true algae. Algae are eukaryotic organisms, while cyanobacteria are prokaryotes. However, they share some similarities in their ecological roles and ability to perform photosynthesis.
How are cyanobacteria studied in the laboratory?
Cyanobacteria are studied in the laboratory using a range of techniques. These include culturing cyanobacterial strains in specific growth media, analyzing their growth and physiological parameters, studying their genetic makeup through molecular techniques, and characterizing their metabolic pathways and interactions with the environment.
is cyanobacteria prokaryotic or eukaryotic?
Cyanobacteria are prokaryotic organisms. Prokaryotes are characterized by the absence of a nucleus and other membrane-bound organelles. In the case of cyanobacteria, their genetic material is not enclosed within a nucleus, and they lack membrane-bound structures such as mitochondria and chloroplasts.
Prokaryotes, including cyanobacteria, belong to the domain Bacteria, whereas eukaryotes, which include plants, animals, fungi, and protists, have cells with a true nucleus and membrane-bound organelles.