What is Chlorophyta?
- Chlorophyta, commonly known as green algae, is a diverse group of photosynthetic organisms classified within the division Chlorophyta. The name originates from the Greek words “chloros” meaning yellow-green, and “phyton” meaning plant, which reflects their characteristic green color derived from the presence of chlorophylls a and b. This green pigment plays a crucial role in photosynthesis, the process by which these organisms convert sunlight into energy.
- Structurally, Chlorophyta is highly diverse, encompassing both unicellular and multicellular forms. The simplest members of this division, such as prasinophytes, are unicellular. However, within the major classes such as Chlorophyceae and Ulvophyceae, there has been evolutionary development toward more complex forms, including colonies and multicellular structures. This variety in structure allows Chlorophyta to thrive in different environmental conditions, from simple single cells to large, complex algae like Ulva (sea lettuce).
- A common feature of Chlorophyta species is the presence of a single green chloroplast within each cell. These chloroplasts are essential for the algae’s ability to capture light for photosynthesis. In addition to chlorophylls, Chlorophyta stores its food as starch within plastids, a key adaptation that supports energy storage and usage within the cells.
- Chlorophyta can be found in a wide range of habitats, from freshwater to marine and even terrestrial environments. Many species thrive in aquatic ecosystems, where light, carbon, nutrients, and water quality are optimal for their survival. Some, like Ulva, can tolerate more extreme conditions, including varying temperatures and exposure to air during low tides, making them highly adaptable to intertidal zones.
- Although the genome structure across different species of Chlorophyta varies significantly, there are common characteristics such as their ability to perform photosynthesis, store starch, and adapt to a wide range of environmental conditions. These organisms play an essential role in many ecosystems, providing oxygen through photosynthesis and serving as a food source for various marine life.
Important features of Chlorophyta
Below is a detailed breakdown of the key features of Chlorophyta:
- Eukaryotic Cells: The cells of Chlorophyta are eukaryotic, meaning they contain a well-defined nucleus. These cells also have various organelles, including mitochondria, Golgi bodies, plastids, endoplasmic reticulum, and ribosomes, which are all vital for cellular function and energy production.
- Cell Wall Composition: The cell wall in Chlorophyta consists of two layers. The inner layer is primarily composed of cellulose, providing structural strength, while the outer layer contains pectic substances, which aid in maintaining cell wall integrity and flexibility.
- Chloroplasts and Pigments: Chlorophyta has well-organized chloroplasts that contain the primary pigments for photosynthesis: chlorophyll a and chlorophyll b. Additionally, accessory pigments like carotenes (α and β carotene) and xanthophylls are present, though in smaller quantities. These pigments assist in capturing light for photosynthesis.
- Variable Chloroplast Shapes: The shape of chloroplasts varies significantly among species. Examples include cup-shaped chloroplasts in Chlamydomonas, girdle-shaped in Ulothrix, reticulate (net-like) in Cladophora, stellate (star-shaped) in Zygnema, spiral in Spirogyra, and discoid in Chara. This variation is a unique characteristic of different chlorophyte species.
- Storage of Reserve Food: The storage of reserve food in Chlorophyta occurs in the form of starch. The synthesis and storage of starch are closely associated with pyrenoids, specialized structures within the chloroplasts.
- Motile Reproductive Structures: The motile reproductive structures in Chlorophyta, such as zoospores and gametes, typically have 2 or 4 flagella. These flagella are either apical or subapical and are of the acronematic (whiplash) type, which assists in movement and locomotion.
- Sexual Reproduction: Sexual reproduction in Chlorophyta can occur in three forms:
- Isogamy: Involves the fusion of two morphologically identical gametes.
- Anisogamy: Involves the fusion of gametes that are different in size or form but similar in motility.
- Oogamy: Involves the fusion of a small, motile male gamete with a large, non-motile female gamete.
- Asexual Reproduction: Asexual reproduction in Chlorophyta takes place through multiple mechanisms such as binary fission, fragmentation, or the formation of motile zoospores. These methods enable rapid population growth and distribution.
- Alternation of Generations: Chlorophyta exhibits an alternation of generations, alternating between a haploid gametophyte phase and a diploid sporophyte phase. When both generations are multicellular, this is referred to as a diplobiontic life cycle. When only the gametophyte is multicellular, it is termed a haplobiontic life cycle.
- Flagella and Locomotion: Chlorophyta species that are motile possess apical flagella that help in locomotion. These flagella enable the organisms to move toward favorable conditions, such as areas with optimal light for photosynthesis.
- Diverse Forms: Chlorophyta includes a wide range of species, from unicellular organisms to multicellular forms. Some, like Ulva (sea lettuce), are macroscopic and visible to the naked eye. Others, like Volvox, form colonies where individual cells aggregate to form a larger structure.
- Paraphyletic Group: Chlorophyta is considered a paraphyletic group, meaning that it consists of organisms that share a common ancestor but do not include all descendant groups, making them an incomplete evolutionary grouping.
Cell structure of Chlorophyta
Chlorophyta, or green algae, exhibit a wide range of structural features at the cellular level, which contribute to their adaptability and function in diverse environments. Below are key structural elements that define their cellular organization:
- Flagella and Scales: In many Chlorophyta, the flagella do not possess microtubular hairs, though fibrillar hairs and Golgi-produced scales are present in some genera, such as Chlamydomonas and Pyramimonas. These features support movement and contribute to cell integrity in certain species.
- Cell Wall Composition: The composition of the cell wall in Chlorophyta is variable. In most species, cellulose acts as the main structural polysaccharide, providing rigidity and strength. However, in specific groups such as the Caulerpales, cellulose is replaced by xylans or mannans. Primitive algae within the Prasinophyceae class have walls or extracellular scales composed of acidic polysaccharides. In contrast, members of the Volvocales order have walls made of glycoproteins, adding flexibility and allowing for specialized interactions with their environment.
- Chloroplasts and Pigments: Chlorophyta contains chloroplasts surrounded by a double membrane, without the presence of a chloroplast endoplasmic reticulum. These chloroplasts house thylakoids grouped in bands of three to five, lacking grana, a structure seen in higher plants. The primary pigments within these chloroplasts are chlorophyll a and b, which are critical for photosynthesis. Additionally, accessory pigments like lutein and carotenoids are present. In species like Dunaliella and Haematococcus, carotenoids such as β-carotene and astaxanthin accumulate under stress conditions, like nitrogen deficiency or high salinity, leading to the cells turning orange or red. These pigments, referred to as hematochromes, can account for 8-12% of the cellular contents, contributing to the vibrant colors seen in various animals, including fish and birds, through the food chain.
- Special Chloroplast Pigments: Certain siphonous green algae and unicellular species, such as Tetraselmis and Mesostigma, possess unique pigments like siphonoxanthin and its ester siphonein. These pigments are distinct to these algae and contribute to their photosynthetic efficiency under specific environmental conditions.
- Starch Storage: Starch, the primary storage carbohydrate in Chlorophyta, is formed within the chloroplast. This process is closely linked to the presence of a pyrenoid, if present, a structure that helps in starch formation and storage. The starch stored in Chlorophyta is similar in composition to that of higher plants, made of amylose and amylopectin, which support cellular energy reserves.
- Contractile Vacuoles: Most members of the Volvocales, a group within Chlorophyta, have contractile vacuoles in their vegetative cells. These vacuoles, typically found near the base of flagella, function by contracting and distending in a cyclical manner. Their primary role is to regulate water content in the cell by expelling excess water, which is crucial in environments where the internal solute concentration of the cell is higher than the surrounding medium. Additionally, contractile vacuoles may play a role in waste removal, ensuring cellular homeostasis. Due to their rhythmic activity, these vacuoles are sometimes referred to as pulsating vacuoles.
- Amyloplasts and Thylakoids: In some siphonous genera like Caulerpa, amyloplasts containing starch grains are found within the chloroplasts. These amyloplasts, alongside a few thylakoids, participate in starch storage, further supporting the energy needs of these algae.
- Photosynthetic Pathways: The photosynthetic mechanisms in Chlorophyta are comparable to those seen in higher plants. Many key pathways, such as carbon fixation and energy conversion, were first studied in green algae like Chlorella, demonstrating the foundational role Chlorophyta has played in understanding plant biology.
Phototaxis and eyespots of Chlorophyta
Phototaxis, or the movement of organisms in response to light, plays a crucial role in the survival of Chlorophyta, allowing them to orient themselves optimally for photosynthesis. Two main mechanisms of phototactic movement exist in Chlorophyta: movement by flagella and movement by mucilage secretion. Both of these phototactic mechanisms are closely related to the function of the eyespot or stigma, a photoreceptive organelle located within the chloroplast.
- Flagellated Phototactic Movement: In most flagellated Chlorophyta cells, phototaxis occurs with the help of an eyespot, a crucial feature for detecting light. The eyespot is always located within the chloroplast, typically in the anterior portion near the flagella bases. It consists of layers of lipid droplets, usually found between the chloroplast envelope and the outermost band of thylakoids. These droplets are orange-red in color due to carotenoids, which enhance the cell’s ability to perceive light.
- Phototactic Response to Light Intensity: Chlorophyta’s phototactic behavior varies with light intensity. For instance, at moderate light levels, organisms may exhibit positive phototaxis (movement toward light). However, at high light intensities, they often display negative phototaxis (movement away from light). External factors such as temperature also influence this response. For example, Haematococcus zoospores show negative phototaxis at 4°C, positive phototaxis at 16–18°C, and a very strong phototactic response at 35°C. Similar behavior is observed in other genera like Ulothrix and Ulva.
- Light Perception Mechanism: Chlorophyta utilizes a two-instant mechanism for light perception. This system measures light at two different time intervals as the cell changes position relative to the light source. In Chlamydomonas, the photoreceptor is located in the plasma membrane above the eyespot, consisting of a chromophore (11-cis-retinal, derived from vitamin A) attached to a protein (opsin or apoprotein). The photoreception process begins when light triggers the isomerization of 11-cis-retinal into all-trans-retinal, initiating a signaling cascade. This 11-cis-retinal is then restored enzymatically.
- Rhodopsins and Photoreceptor Currents: Different proteins and rhodopsins are involved in phototaxis. In Chlamydomonas reinhardtii, the chromophore-protein complex is known as chlamyopsin, while in Volvox carteri it is called volvoxopsin. Both Chlamydomonas sensory rhodopsin A and B play roles in photoreception. Rhodopsin A, which absorbs light at 510 nm, mediates a fast photoreceptor current, facilitating the photophobic response, preventing the organism from crossing light-dark borders. Rhodopsin B, absorbing light at 470 nm, generates a slower current responsible for phototaxis, helping the organism move towards or away from the light source based on intensity.
- Flagellar Movement and Calcium Regulation: The eyespot reflects light onto the photoreceptor in the plasma membrane, causing changes in membrane potential and influencing calcium ion entry into the cell. Calcium ion concentration in the cytoplasm regulates the beating of the flagella. At different calcium concentrations, each flagellum beats differently, altering the swimming direction of the cell. The variation in flagellar movement due to calcium ion flux enables the cell to respond efficiently to light stimuli.
- Eyespot Structure and Function: Studies show that the eyespot region of the plasma membrane contains a higher density of protein particles compared to other areas. For instance, in Chlorosarcinopsis gelatinosa, the plasma membrane over the eyespot has 8200 protein particles per µm², whereas other membrane regions have only 2100 particles. These particles are likely involved in the photoreceptor system, disappearing during flagellar retraction, which suggests that the photoreceptor is no longer needed during certain life cycle stages, such as prior to cell wall secretion.
- Mucilage-Secretion-Based Phototactic Movement: Besides flagellated movement, some Chlorophyta move toward light using mucilage secretion. In 1848, Ralfs first noted this movement in desmids, where light acted as a stimulus. Braun further observed in 1851 that young cells of Penium curtum aligned their long axes and moved toward light. This movement is driven by the extrusion of slime through pores in the cell wall, particularly in the apical region. This method of movement, though less common than flagellar movement, demonstrates the diversity in Chlorophyta’s phototactic behaviors.
- Geotaxis: In addition to phototaxis, Chlorophyta exhibit geotactic responses, moving in relation to gravity. For example, Chlamydomonas displays negative geotaxis, swimming against gravity to move toward light, which would be beneficial in low-light conditions. This energy-dependent movement helps the organism remain near the water’s surface, ensuring access to sufficient light for growth and reproduction. Geotaxis is a slow process, requiring a minimum horizontal swimming path of 200 µm to maintain its course.
Reproduction of Chlorophyta
Reproduction in Chlorophyta occurs through both asexual and sexual mechanisms, offering adaptability and survival advantages in changing environments. These processes vary depending on the organism’s structure and environmental conditions. Understanding the mechanisms behind both forms of reproduction sheds light on the evolutionary strategies of these green algae.
- Asexual Reproduction:
- Chlorophyta employ various forms of asexual reproduction, with fragmentation being the simplest. In fragmentation, colonies divide into two or more parts, each of which becomes a new colony.
- Zoosporogenesis is another common method, often triggered by environmental changes. Zoospores, typically flagellated reproductive cells, are produced in vegetative cells, as seen in Ulothrix. However, in some cases, they are formed in specialized sporangia, as in Derbesia.
- Zoospores are primarily produced in younger filament parts, and their number often follows a power of two in uninucleate species.
- Aplanospores are non-flagellated spores with a distinct wall from the parent cell. These are considered abortive zoospores but can germinate into new plants, as seen in Trebouxia.
- Autospores are a specialized type of aplanospores that share the shape of the parent cell and are common in genera like Chlorella. They are produced in multiples of two within the parent cell.
- In colonies like those of Volvox, coenobia (colonies with a definite number of cells arranged in a specific manner) form. These colonies produce daughter coenobia with a fixed number of cells, and as these daughter colonies mature, the cells enlarge without further division of vegetative cells.
- Sexual Reproduction:
- Sexual reproduction in Chlorophyta follows three main forms: isogamy, anisogamy, and oogamy, representing evolutionary steps in reproductive complexity.
- In isogamous species, gametes are morphologically similar, while in anisogamous species, gametes differ in size.
- Oogamous species produce highly specialized gametes, typically within specialized reproductive organs known as gametangia, as seen in Coleochaete. In oogamy, a large non-motile egg is fertilized by a smaller motile sperm.
- The majority of Chlorophyta species form motile, flagellated gametes, known as zoogametes. However, some groups, such as the Zygnematales, produce non-flagellated aplanogametes or amoeboid gametes.
- Environmental changes often induce gametogenesis, although in some species, the presence of two sexually different strains is necessary. In these species, the vegetative cells of one strain secrete a substance that triggers sexual differentiation in the other strain. This process is commonly observed in the Volvocales.
- In species such as Oedogonium, sex organs form without the presence of the opposite strain, though fertilization is under complex hormonal regulation.
- In certain cases, eggs secrete chemotactic substances that attract spermatozoids, a phenomenon that is not typically observed in isogamous species.
- Mating-Type Reactions:
- In isogamous species, gametes randomly encounter one another and adhere through a process called agglutination. This is facilitated by flagellar adhesion, in which gametes of different mating types cluster and adhere by their flagella tips. After flagella adherence, the anterior ends of the complementary gametes fuse, freeing the flagella. The resulting motile zygote swims for some time before settling and secreting a thick protective wall.
- Mating-type substances, which mediate flagellar agglutination, are localized at the flagella tips. These substances can be isolated and retain their ability to induce agglutination in gametes of the opposite sex. For instance, adding a female mating-type substance to a culture of male gametes will cause them to clump together in a process called isoagglutination.
- Chlamydomonas has been the most thoroughly studied in terms of mating-type reactions, and its flagellar agglutination mechanisms provide valuable insight into this process.
- Syngamy and Meiosis:
- After gametes fuse in the process known as syngamy, meiosis occurs within the thick-walled zygotes. This is a common phenomenon in various orders of Chlorophyta, including the Volvocales, Ulotrichales, Oedogoniales, Chlorellales, and Zygnematales.
- Sexual reproduction in Chlorophyta follows three main forms: isogamy, anisogamy, and oogamy, representing evolutionary steps in reproductive complexity.
Classification of Chlorophyta
The classification of Chlorophyta, a group of green algae, is based on both ultrastructural characteristics and molecular genetics. The four primary classes within Chlorophyta are Prasinophyceae, Charophyceae, Ulvophyceae, and Chlorophyceae. The classification system was first introduced in the 1970s by researchers Karl Mattox and Kenneth Stewart, and it has since been confirmed by genetic studies. Each class exhibits distinct morphological and reproductive features, contributing to a better understanding of their evolutionary significance.
- Class 1: Prasinophyceae:
- Members of this class are either scaly or naked flagellates, meaning they possess either a scale-covered or bare surface.
- During cytokinesis, the interzonal spindles remain persistent.
- Considered primitive green algae, Prasinophyceae represent some of the earliest green algae, with some species potentially giving rise to the other classes within Chlorophyta.
- Class 2: Charophyceae:
- Motile cells in this class are asymmetrical, with two laterally attached flagella.
- The flagellar root is composed of a broad band of microtubules, alongside a smaller microtubular root.
- Some species possess a multilayered structure (MLS), but a rhizoplast is not present.
- Scales are often found outside of motile cells.
- During telophase, the mitotic spindle remains persistent, and a phragmoplast forms new cross walls after cell division.
- Unlike other classes, eyespots are generally absent.
- Charophyceae break down glycolate via glycolate oxidase and break down urea using urease.
- This class is predominantly freshwater and engages in sexual reproduction that results in a dormant zygote. Meiosis occurs upon the zygote’s germination.
- Charophyceae are evolutionarily significant because they are in the lineage that led to land plants (embryophytes). As such, there have been proposals to group Charophyceae with land plants into a supergroup known as Viridiplantae, or in some cases, Steptophyta.
- Class 3: Ulvophyceae:
- In Ulvophyceae, flagella are attached at the anterior end of motile cells, which exhibit near-radial symmetry externally.
- Their flagellar roots are composed of four cruciately arranged microtubular roots, and in some cases, a rhizoplast is present.
- Unlike Charophyceae, the multilayered structure (MLS) is absent in this class.
- Motile cells may be covered in scales.
- The interzonal spindle persists during telophase, and cell division occurs through the formation of a cleavage furrow that produces a new cross wall.
- Eyespots are common in this group, aiding in phototaxis.
- Ulvophyceae break down glycolate via glycolate dehydrogenase and use urease for the breakdown of urea.
- Predominantly marine, Ulvophyceae do not form dormant zygotes.
- In this class, alternation of generations is common, with species alternating between haploid and diploid stages in their life cycle.
- Class 4: Chlorophyceae:
- The motile cells in Chlorophyceae exhibit radial or near-radial symmetry and, similar to Ulvophyceae, have their flagella attached at the anterior end.
- The flagellar roots consist of four cruciately arranged microtubular roots, and a rhizoplast may also be present in some species.
- Motile cells often possess a theca, a rigid outer covering.
- In telophase, the interzonal spindle collapses, and a phycoplast forms the new cross wall during cell division.
- Eyespots are common in motile cells, contributing to their ability to detect light.
- Glycolate is broken down by glycolate dehydrogenase, and urea amidolyase is used to break down urea.
- Chlorophyceae are predominantly freshwater organisms.
- Their reproductive cycle involves a dormant period for the zygote, and meiosis takes place when the zygote germinates.
Biological Importance of Chlorophyta
- Primary Producers:
- Chlorophytes are essential producers in ecosystems, primarily because of their role in photosynthesis.
- Through this process, they convert light energy into starch, serving as a major energy source for other organisms.
- Besides starch production, Chlorophyta release oxygen as a byproduct of photosynthesis, contributing significantly to the Earth’s oxygen supply.
- Source of Food for Heterotrophs:
- Many heterotrophic organisms, particularly within aquatic environments, rely on Chlorophyta as a primary food source.
- The starch and organic matter produced by chlorophytes through photosynthesis are consumed by various organisms, sustaining different levels of the food web.
- Symbiotic Relationships:
- Chlorophytes are known to form symbiotic relationships with a range of other organisms.
- One of the most notable examples is their association with fungi to form lichens. In this relationship, the green algae provide photosynthates to the fungal partner, while the fungus offers a protected environment for the algae.
- Other examples of symbiosis include their interaction with ciliates, cnidarians, and mollusks, where these animals house chlorophytes within their tissues, benefiting from the oxygen and nutrients the algae produce.
- Heterotrophic Chlorophytes:
- While Chlorophyta are predominantly photosynthetic, not all species rely solely on this method for energy production.
- Certain chlorophytes, such as Prototheca sp., exhibit heterotrophic behavior, meaning they can obtain nutrients by consuming organic matter rather than through photosynthesis.
- Prototheca sp. is particularly notable as it can become pathogenic, causing protothecosis in humans and animals. This disease manifests when the algae infect tissues, typically in immunocompromised individuals.
Chlamydomonas
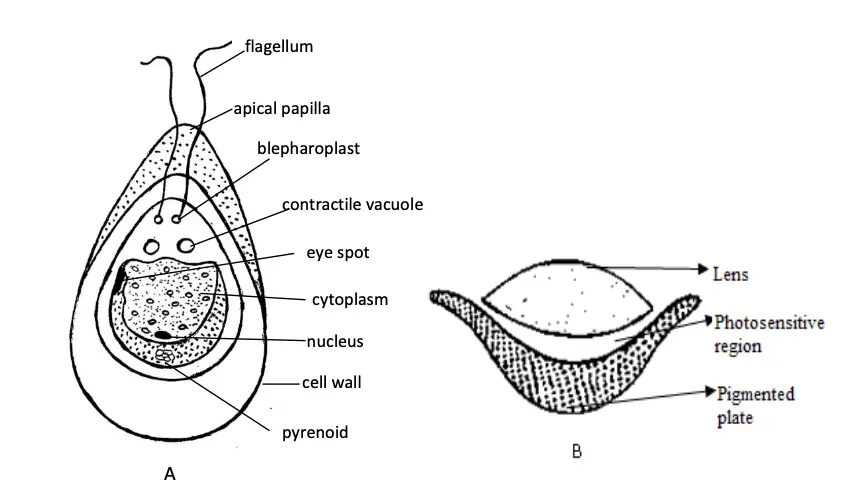
Occurrence of Chlamydomonas
Chlamydomonas is a highly diverse genus of green algae, represented by approximately 400 species. This unicellular, motile organism is primarily found in freshwater environments, though it can also thrive in a variety of other habitats. Its widespread presence reflects its adaptability to different environmental conditions, from nutrient-rich waters to extreme cold. The following points outline its typical habitats and occurrences:
- Freshwater Habitats:
- Chlamydomonas is most commonly found in freshwater environments, especially those rich in nitrogen salts and organic matter. These conditions support the growth of the alga, enabling it to thrive.
- It can be found in stagnant waters such as ponds, pools, ditches, water tanks, and sewage tanks. In these environments, its motile nature allows it to move towards favorable light and nutrient conditions.
- Additionally, Chlamydomonas is found in slow-running waters, where it contributes to the planktonic population. Its presence often gives the water a green appearance due to its abundance near the surface.
- Terrestrial Occurrence:
- Besides aquatic environments, some species of Chlamydomonas are terrestrial, growing on moist soil surfaces. These species are commonly found in rice fields and along the banks of rivers and lakes.
- The alga forms palmella stages, which create a scum layer on soil surfaces during moist conditions. This stage represents a protective strategy for survival under harsh environmental conditions.
- Saline and Brackish Water:
- Some species of Chlamydomonas are capable of living in saline or brackish water, where salt concentrations are higher than in freshwater environments. Their ability to adapt to these conditions demonstrates the genus’s wide ecological range.
- Cryophytic Habitats:
- Chlamydomonas species can also be found in extreme cold environments, growing on snow surfaces. These cryophytic species contribute to the coloration of snow in some regions.
- For example, Chlamydomonas nivalis causes red snow due to the presence of a red pigment called haematochrome, while Chlamydomonas yellowstonenris imparts a green color to snow. These pigments offer protection from intense light and ultraviolet radiation, allowing the algae to photosynthesize in these harsh conditions.
Structure of Thallus of Chlamydomonas
Chlamydomonas is a motile unicellular green alga, where the thallus is represented by a single cell. Its structure is specialized for photosynthesis, movement, and environmental sensing, with distinct features that enable these functions. Below are the key structural components of the thallus of Chlamydomonas:
- Shape and Size:
- The cell is biflagellate, meaning it has two flagella, and is typically spherical, ellipsoidal, or pear-shaped.
- The thallus is commonly pyriform (pear-shaped), with a narrow anterior end where the flagella are attached and a broad posterior end.
- In certain species like Chlamydomonas caudata, the posterior end is pointed.
- The cell is about 30 µm in length and 20 µm in diameter, reflecting its relatively small size as a unicellular organism.
- Flagella:
- Two whiplash-type flagella are located at the anterior end of the cell. These flagella are usually longer than the cell body but in some species, they may be equal to or shorter than the thallus.
- Each flagellum originates from a basal granule or blepharoplast and extends through a fine canal in the cell wall.
- These flagella provide motility, allowing the cell to swim through its aquatic environment.
- Contractile Vacuoles:
- Near the bases of the flagella, there are typically two contractile vacuoles, although occasionally more may be present.
- These vacuoles are involved in osmoregulation and excretion, helping the cell maintain proper water balance.
- Cell Wall:
- The cell wall is composed primarily of cellulose, which provides structural integrity.
- In some species, this cellulose wall is surrounded by a gelatinous sheath, offering additional protection and potentially aiding in adhesion to surfaces.
- Chloroplasts:
- A prominent cup-shaped chloroplast is present in each cell, essential for photosynthesis. This cup-shaped structure surrounds the cell’s nucleus.
- Different species of Chlamydomonas may have other types of chloroplasts, including:
- H-shaped chloroplast (e.g., Chlamydomonas biciliata)
- Discoid chloroplast (e.g., Chlamydomonas alpina)
- Reticulate chloroplast (e.g., Chlamydomonas reticulacta)
- Ridged chloroplast (e.g., Chlamydomonas stenii)
- Axile chloroplast (e.g., Chlamydomonas eradians)
- Pyrenoids:
- Embedded within the chloroplasts are pyrenoids, which are proteinaceous bodies responsible for the synthesis and storage of starch. These are key to the cell’s energy storage mechanisms.
- Nucleus:
- The cell contains a single large, dark nucleus located within the cavity of the cup-shaped chloroplast. This central position reflects its importance in controlling cellular functions.
- Eyespot (Stigma):
- The eyespot or stigma is a pigmented structure located in the anterior part of the cell. It plays a crucial role in photoreception, allowing Chlamydomonas to detect and move towards light (phototaxis).
- The eyespot consists of a colorless, biconvex photosensitive lens and a curved pigmented plate. This structure acts as a primitive eye, aiding the cell in finding optimal light conditions for photosynthesis.
Mode of Reproduction in Chlamydomonas
Reproduction in Chlamydomonas occurs through both asexual and sexual means. Each mode is adapted to the organism’s environmental conditions, allowing it to survive and propagate under various circumstances. Below is a detailed breakdown of the reproductive strategies:
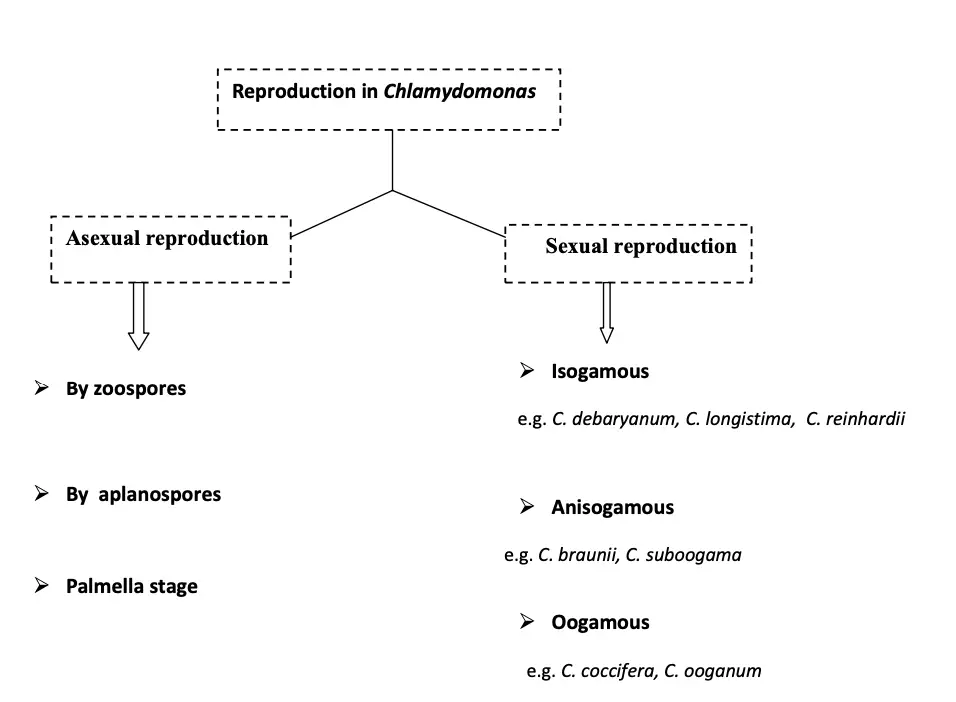
Asexual Reproduction
Asexual reproduction in Chlamydomonas involves the production of spores and occurs through the following methods:
- By Zoospores:
- Zoospore formation is the most common form of asexual reproduction in Chlamydomonas. It occurs under favorable conditions.
- The protoplast of the parent cell contracts, detaching from the cell wall, and the parent cell loses its flagella.
- The contractile vacuoles and neuromotor apparatus disappear.
- The protoplast undergoes a series of mitotic divisions, starting with a longitudinal division into two daughter cells, followed by another division at a right angle, resulting in four daughter chloroplasts.
- Each daughter protoplast develops a cell wall and flagella, transforming into a zoospore.
- The parent cell wall gelatinizes or ruptures, releasing the motile zoospores. These zoospores are smaller in size but structurally similar to the parent cell and grow to become mature Chlamydomonas.
- By Aplanospores:
- Aplanospore formation occurs under unfavorable conditions.
- The parent cell loses its flagella, and the protoplast divides into daughter cells. However, these daughter cells do not develop flagella.
- The protoplasts round off and secrete a thin wall, forming non-motile aplanospores.
- Under favorable conditions, the aplanospores either germinate directly or divide to produce motile zoospores.
- Palmella Stage:
- The palmella stage occurs during extreme environmental conditions such as water shortage or excess salts.
- The protoplast divides into many daughter cells, but unlike in zoospore formation, these cells do not form flagella.
- The parent cell wall gelatinizes, forming a mucilaginous sheath around the daughter cells, which are now enclosed in gelatinous walls.
- This forms an amorphous colony, termed the palmella stage. Once favorable conditions return, the gelatinous sheath dissolves, and the cells develop flagella and become motile.
Sexual Reproduction
Sexual reproduction in Chlamydomonas is carried out through three distinct methods, depending on the species:
- Isogamous Reproduction:
- This is the most common method in Chlamydomonas species, where similar-sized gametes fuse.
- These isogametes are morphologically identical but physiologically dissimilar.
- The gametes approach each other by their anterior ends, and fusion begins on the lateral sides, forming a quadriflagellate and binucleate zygote.
- The quadriflagellate zygote remains motile for several hours or even days before the two nuclei fuse, forming a diploid zygote.
- Anisogamous Reproduction:
- In anisogamy, the gametes are unequal in size. The male gamete (microgamete) is smaller and more motile, while the female gamete (macrogamete) is larger.
- The macrogametes are produced in the female gametangium, where the protoplast divides to form 2–4 gametes. In contrast, the microgametes are formed in the male gametangium, where the protoplast divides to form 8–16 gametes.
- The microgamete actively moves towards the macrogamete, and after fusion, a diploid zygote is formed.
- Oogamous Reproduction:
- This method is observed in certain species like Chlamydomonas coccifera and Chlamydomonas ooganum.
- In oogamy, the female gamete is a large, non-motile macrogamete, while the male gametes are motile microgametes.
- The female thallus withdraws its flagella and directly functions as the macrogamete. The microgametes reach the macrogamete, and their anterior ends fuse, forming a diploid zygote.
Zygote Development
- The zygote formed from sexual reproduction is diploid and can survive unfavorable conditions for extended periods.
- When favorable conditions return, the zygote undergoes reduction division, producing four daughter protoplasts.
- Each protoplast develops into a biflagellate zoospore, which eventually grows into a mature thallus of Chlamydomonas.
Volvox
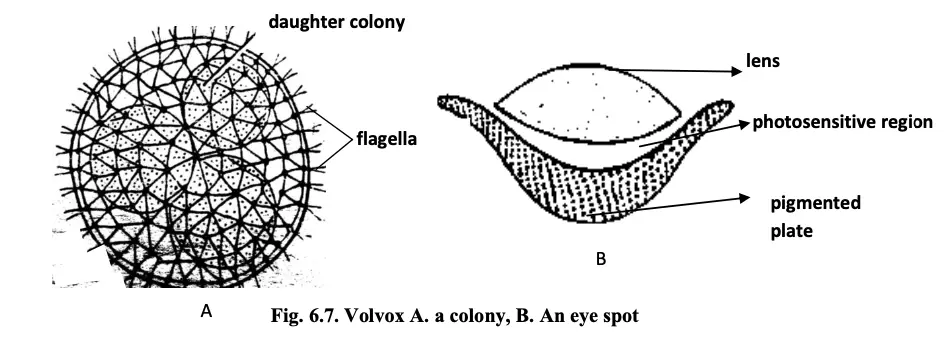
Occurrence of Volvox
Volvox is a fascinating free-floating freshwater green alga that exists predominantly in colonial forms. This genus comprises approximately 20 species, which thrive as plankton in various aquatic environments. The occurrence of Volvox can be categorized based on its habitat and growth patterns.
- Habitat:
- Volvox colonies are primarily found on the surface of freshwater bodies, including both temporary and permanent ponds and water tanks.
- These algae prefer water bodies rich in nutrients, particularly during periods of high availability.
- Growth Patterns:
- During the rainy season, Volvox exhibits rapid growth due to the influx of nutrients and favorable environmental conditions.
- This rapid proliferation can lead to the formation of dense colonies that turn the surface of water bodies a striking green hue.
- Appearance:
- The colonies of Volvox are visually distinctive, often resembling green rolling balls floating on the water’s surface. This characteristic appearance is a result of their colonial structure, where numerous individual cells work together in a coordinated manner.
- Species in India:
- Several species of Volvox are noted for their occurrence in India. Some of the prominent species include:
- Volvox globator
- Volvox aureus
- Volvox prolificus
- Volvox africanus
- Volvox rousselettii
- Several species of Volvox are noted for their occurrence in India. Some of the prominent species include:
Structure of Thallus
The thallus of Volvox is a well-organized, motile colonial structure characterized by a defined shape and a specific number of cells, collectively referred to as a coenobium. This structural arrangement allows Volvox to thrive as a colonial alga in freshwater environments.
- Shape and Size:
- The colonies of Volvox are generally oval or spherical in shape.
- Each colony can comprise between 500 to 60,000 cells, indicating a substantial degree of complexity and organization within the colony.
- Cell Arrangement:
- The cells are biflagellate, meaning each cell has two flagella, which are crucial for movement.
- Cells are arranged in a single layer along the periphery of a gelatinous colonial envelope, ensuring effective coordination and movement.
- Movement:
- Movement of the colony is facilitated by the joint action of the flagella of individual cells. This synchronized beating of flagella allows the entire colony to navigate through the water.
- Cellular Connectivity:
- All cells within the colony are interconnected by cytoplasmic strands, which enable communication and nutrient exchange between cells.
- Cell Characteristics:
- The cells of the anterior end possess larger eye spots compared to those at the posterior end. The larger eye spots enhance light detection for photosynthesis.
- Cells at the posterior end are primarily involved in reproduction when they reach maturity.
- Cells typically have a pyriform shape, characterized by a narrow anterior end and a broader posterior end.
- Cell Structure:
- Each cell contains:
- One nucleus
- A cup-shaped chloroplast, responsible for photosynthesis.
- Pyrenoids, which are proteinaceous bodies involved in starch synthesis and storage.
- An eye spot, which is located toward the external face of the cell. This structure consists of a colorless biconvex photosensitive lens and a curved pigmented plate.
- Two contractile vacuoles that help in osmoregulation and excretion.
- Notably, reproductive cells do not possess flagella, contrasting with the vegetative cells, which are motile.
- Each cell contains:
- Cell Independence:
- Each cell within the Volvox colony operates independently regarding various functions, contributing to the colony’s overall health and productivity.
Mode of Reproduction in Volvox
The mode of reproduction in Volvox is crucial for its life cycle and involves both asexual and sexual reproduction. The posterior cells of the colony primarily participate in reproduction, exhibiting distinct characteristics that facilitate these processes.
I. Asexual Reproduction
Asexual reproduction occurs under favorable conditions, typically during spring and early summer. The following steps outline this process:
- Gonidia Formation:
- Certain cells at the posterior end of the colony differentiate into reproductive cells known as gonidia or parthenogonidia.
- Cell Size and Structure:
- Gonidia are notably larger than vegetative cells. They lack flagella and eye spots, and their pyrenoids increase in number.
- Internal Positioning:
- The gonidia are pushed toward the interior of the colony, preparing for division.
- Initial Division:
- The first division of the gonidium is longitudinal, producing two cells.
- Subsequent Divisions:
- The second division occurs longitudinally at a right angle to the first division, resulting in four cells. A third longitudinal division occurs, yielding eight cells arranged in a plakea stage, characterized by a curved plate-like structure.
- Hollow Sphere Formation:
- Each of these eight cells undergoes longitudinal division, forming a hollow sphere comprised of 16 cells. This sphere features a small external aperture called the phialopore.
- Continued Division:
- The cells continue dividing until reaching the characteristic number for their species. At this stage, the cells are naked and in close contact, with their pointed anterior ends directed inward.
- Inversion of the Colony:
- The inversion of the colony begins with the formation of a constriction opposite the phialopore. The posterior cells, along with the constriction, are pushed inside the sphere, eventually emerging from the phialopore.
- Development of Daughter Colonies:
- After inversion, the cells develop cell walls, flagella, and eye spots. A gelatinous sheath forms around each cell, leading to the establishment of what is known as a daughter colony.
- Detachment:
- Initially, the daughter colonies remain attached to the gelatinous wall of the parent colony before eventually becoming free within the gelatinous matrix of the parent.
II. Sexual Reproduction
Volvox engages in oogamous sexual reproduction, which can be classified as monoecious or dioecious. Monoecious species, such as V. globator, typically display protandry, where male reproductive organs mature before female organs. It is important to note that colonies undergoing sexual reproduction typically do not form asexual daughter colonies. The steps in sexual reproduction are as follows:
- Gameteangia Formation:
- Reproductive cells primarily differentiate at the posterior part of the colony, enlarging, losing their flagella, and becoming known as gameteangia.
- Male and Female Gametes:
- The male reproductive cells are referred to as antheridia or androgonidia, while the female reproductive cells are called oogonia or gynogonidia.
- Development of Antheridia:
- The development of the antheridium begins with the formation of an initial androgonidial cell in the colony’s posterior end.
- Cell Enlargement:
- These initial cells enlarge, lose their flagella, and undergo multiple simple divisions, resulting in numerous daughter cells.
- Formation of Antherozoids:
- Each daughter cell develops into a naked, biflagellate, fusiform male reproductive cell known as an antherozoid. This structure contains two contractile vacuoles, a nucleus, a cup-shaped chloroplast, a pyrenoid, and an eye spot, typically exhibiting pale yellow or green coloration.
- Release of Antherozoids:
- The antherozoids are released either individually or in groups into the surrounding water.
- Development of Oogonia:
- The oogonium is larger than other colony cells, with the mature oosphere or ovum being a round or flask-shaped structure.
- Receptive Spot Functionality:
- The flask-shaped beak of the oogonium serves as a receptive spot for the male gametes.
- Swimming of Antherozoids:
- After being liberated from the antheridia, the antherozoids swim freely on the water’s surface.
- Chemotactic Response:
- Antherozoids exhibit chemotactic behavior, allowing them to locate and reach the oogonia. Upon reaching the oogonium, multiple antherozoids may enter, but only one successfully penetrates through the receptive spot.
- Fusion of Gametes:
- Following the entry of the antherozoid, plasmogamy (fusion of male and female cytoplasm) and karyogamy (fusion of male and female nuclei) occur, resulting in the formation of a diploid zygote.
- Zygote Dormancy and Germination:
- The diploid zygote secretes a thick wall and may remain dormant until favorable conditions return. Upon the arrival of suitable climatic conditions, the zygote germinates through a reduction division, wherein three out of four daughter cells degenerate. Only one survives, maturing into a zoospore.
- Development into a Colony:
- The zoospore undergoes mitotic divisions, leading to the establishment of a new Volvox colony.
Oedogonium
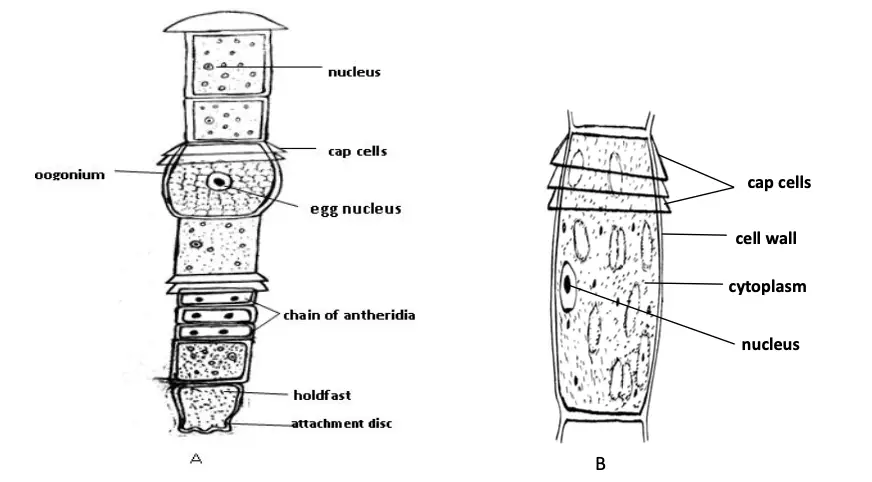
Occurrence of Oedogonium
Oedogonium is a freshwater, unbranched filamentous alga known for its ecological significance and distribution. This genus thrives primarily in various aquatic environments, demonstrating versatility in habitat preference. Below are key aspects regarding the occurrence of Oedogonium:
- Habitat:
- Oedogonium predominantly inhabits permanent freshwater bodies, including lakes, tanks, and ponds. These environments provide the necessary conditions for growth, including adequate light and nutrient availability.
- Terrestrial Species:
- Besides its aquatic presence, some species of Oedogonium, such as O. terrestris and O. randhawe, can be found in terrestrial habitats. These species thrive in moist soil conditions, showcasing the adaptability of the genus to various environmental settings.
- Diversity:
- There are approximately 200 species of Oedogonium reported in India. This rich diversity indicates the genus’s widespread occurrence and ecological importance in different regions.
- Ecological Role:
- The presence of Oedogonium in aquatic ecosystems contributes to primary production, serving as a food source for various aquatic organisms. Its filamentous structure allows it to capture light efficiently, thereby playing a vital role in the food web.
Structure of Thallus of Oedogonium
The thallus of Oedogonium exhibits a distinctive structure characterized by its filamentous and multicellular form. This organization contributes to the alga’s functionality and ecological role within its habitat. Below are the key structural features of the Oedogonium thallus:
- Filamentous Structure:
- Oedogonium consists of unbranched filaments made up of cylindrical cells. This linear arrangement allows for efficient nutrient absorption and light capture, which are essential for photosynthesis.
- Basal Cell:
- The thallus has a modified basal cell that functions as a holdfast. This basal cell anchors the alga to substrates in its aquatic environment, ensuring stability. Notably, the basal cell lacks chloroplasts, distinguishing it from other cells in the filament.
- Terminal Cells:
- The terminal cells of the filaments exhibit variability in shape. They can be rounded, elongated, or acuminate, contributing to the overall morphology of the alga. These shapes can aid in the buoyancy and positioning of the filament within the water column.
- Transverse Bands:
- A characteristic feature of Oedogonium is the presence of distinct transverse bands at the distal ends of some cells. These bands are formed during cell division and are referred to as apical caps. The cells that possess these apical caps are known as cap cells. This structural feature may play a role in the regulation of growth and cell division.
Mode of Reproduction in Oedogonium
Oedogonium exhibits a variety of reproductive strategies that contribute to its survival and propagation. The modes of reproduction can be classified into three main categories: vegetative reproduction, asexual reproduction, and sexual reproduction. Each of these methods has distinct mechanisms and implications for the growth and adaptation of the organism.
- Vegetative Reproduction:
- Vegetative reproduction occurs through two primary mechanisms:
- Fragmentation:
- Small fragments of the Oedogonium filament can grow independently to form new thalli. This fragmentation often results from mechanical pressures or the dissolution of transverse walls within the filament, allowing parts of the alga to establish themselves as independent entities.
- Akinetes:
- Under unfavorable environmental conditions, Oedogonium forms thick-walled structures known as akinetes. These reddish or brownish cells appear in small chains and serve as a means of survival. When conditions become favorable again, akinetes can germinate, each leading to the formation of new filaments.
- Fragmentation:
- Vegetative reproduction occurs through two primary mechanisms:
- Asexual Reproduction:
- Asexual reproduction in Oedogonium primarily involves the formation of zoospores:
- Zoospores are multiflagellate cells that develop singularly within the intercalary cap cell of the filament. The newly formed cap cell acts as the zoosporangium, where the zoospore matures.
- Once mature, the zoosporangium splits near its apex, releasing the zoospore. The liberated zoospores can be ovoid, spherical, or pyriform in shape and are characterized as uninucleate cells containing a chloroplast.
- After their release, the zoospores swim freely until they settle on a substrate, positioning their anterior end downward. Subsequently, the apical cell of the newly settled zoospore divides repeatedly, leading to the formation of a new filament.
- Asexual reproduction in Oedogonium primarily involves the formation of zoospores:
- Sexual Reproduction:
- The sexual reproduction in Oedogonium is characterized as an advanced oogamous process involving male and female gametes:
- The male gametes, known as antherozoids, are produced in structures called antheridia, while the female gametes, or eggs, are produced in oogonia.
- This genus exhibits sexual dimorphism; the male and female gametes differ both morphologically and physiologically.
- The reproductive strategy is further categorized into two types of species based on the distribution of sex organs:
- Macrandrous Species:
- In these species, antheridia are located on filaments of normal size. Some macrandrous species are monocious, having both antheridia and oogonia on the same filament (e.g., O. nodulosum and O. fragile), while others are dioecious, with antheridia and oogonia located on separate filaments (e.g., O. crassum and O. aquaticum).
- Nannandrous Species:
- In these species, the filaments bearing antheridia are morphologically distinct and much smaller than those bearing oogonia, referred to as dwarf males or nannandria. These dwarf males arise from androspores formed in androsporangia.
- Macrandrous Species:
- The antherozoids are unicellular, uninucleate, and multiflagellate, allowing them to swim towards the oogonium.
- Fertilization typically involves a single antherozoid entering the oogonium through an opening in the oogonial wall, resulting in the formation of a diploid zygote.
- After fertilization, the zygote generally undergoes a period of dormancy. Upon the return of favorable conditions, the zygote germinates, dividing through reduction division to produce four daughter cells, which then develop into zoospores.
- Finally, the zoospores are released following the rupture of the zygote wall and subsequently germinate to form new haploid filaments.
- The sexual reproduction in Oedogonium is characterized as an advanced oogamous process involving male and female gametes:
- https://www.biologydiscussion.com/algae/life-cycle-algae/chlorophyta-class-important-features-and-orders/21009
- https://naturalhistory.si.edu/research/botany/research/algae/algae-classification
- https://www.biologyonline.com/dictionary/chlorophyta
- https://ddugu.ac.in/ePathshala_Attachments/STUDY349@353994.pdf
- https://www.uobabylon.edu.iq/eprints/publication_11_26270_754.pdf
- https://www.uobabylon.edu.iq/eprints/publication_12_3410_754.pdf