What is a Cell?
- A cell is the fundamental unit of life, acting as the smallest structure capable of carrying out all life’s essential processes. It serves as the building block for all living organisms, whether they are single-celled organisms like bacteria or complex multicellular organisms like humans. Robert Hooke first discovered cells, and since then, the field of cell biology has expanded to explain how these tiny units function and sustain life.
- Cells contain cytoplasm, a gel-like fluid enclosed by a cell membrane. The cytoplasm houses essential biomolecules such as proteins, nucleic acids, and lipids, as well as specialized structures called organelles. Organelles within the cytoplasm, such as the nucleus, mitochondria, and ribosomes, have distinct roles that enable cells to function efficiently. For example, the nucleus holds the cell’s genetic material, while mitochondria generate energy through cellular respiration.
- In terms of size and number, cells can vary widely across organisms. Single-celled organisms like bacteria consist of one cell that performs all life functions. In contrast, multicellular organisms have a wide variety of cell types, each specialized for particular tasks. Humans, for example, have billions of cells with varying shapes and sizes, adapted to perform unique functions.
- Despite their differences, all cells share common structural components and operate under similar biological principles. These include providing structure, processing nutrients for energy, and ensuring the organism’s growth and reproduction. Furthermore, cells contain genetic material, which not only determines the organism’s characteristics but also guides cellular activities and division.
Definition of Cell
A cell is the smallest, basic unit of life that performs essential functions such as growth, reproduction, and energy processing, and serves as the structural and functional building block of all living organisms.
Discovery of Cells
The discovery of cells marked a turning point in biological science, revealing that all living organisms are composed of these basic units. Understanding cells and their functions has deepened our comprehension of life and its processes.
- Robert Hooke’s Initial Discovery (1665):
- In 1665, Robert Hooke became the first scientist to discover cells. He examined a thin slice of cork using a compound microscope.
- He observed small, box-like structures that resembled tiny rooms or “cells” (a term derived from the Latin word for small rooms).
- However, due to the limitations of his microscope’s magnification, Hooke could only see the outer walls of the cells, not their internal components. He mistakenly believed these were non-living structures.
- Antonie van Leeuwenhoek’s Advancements:
- Later, Antonie van Leeuwenhoek, using a more advanced microscope with better magnification, made further discoveries in cell biology.
- Leeuwenhoek observed living cells in samples of pond water, noting that these cells exhibited movement, which he interpreted as evidence of life.
- He referred to these moving entities as “animalcules,” emphasizing their living nature, unlike Hooke’s earlier non-living observation of cork cells.
- Robert Brown’s Identification of the Nucleus (1833):
- In 1833, Robert Brown, a Scottish botanist, made a significant discovery regarding cell structure.
- While examining orchid cells, he identified the presence of a prominent structure within the cell, which he named the “nucleus.”
- This discovery provided the first detailed insight into the internal components of a cell, further advancing our understanding of cellular organization.
The Origin of Cells
The origin of cells and the emergence of life are subjects deeply rooted in the history of scientific exploration. Current understanding suggests that cells, as fundamental units of life, originated approximately 3.8 billion years ago. These first cells marked the beginning of an unbroken chain of life, which continues in the organisms we see today. This narrative explores the processes that contributed to cell origin, the evolution of cell theory, and experiments that refuted earlier beliefs in spontaneous generation.
- Cell Theory Fundamentals
- The cell theory emphasizes that all living things are composed of cells or their derivatives, that cells are the smallest unit of life, and that cells arise only from pre-existing cells.
- Historically, it was believed that life could emerge spontaneously from non-living matter, an idea known as spontaneous generation. This notion was ultimately refuted through various scientific experiments, solidifying the concept that life arises solely through the reproduction of existing cells.
- Evolution from a Common Ancestor
- All present-day cells are thought to have descended from a common prokaryotic ancestor, diverging into three primary lineages: archaebacteria, eubacteria, and eukaryotes.
- In prokaryotes, cell division occurs through binary fission, a simpler process compared to eukaryotes, where cells undergo mitosis to produce identical cells and meiosis to generate haploid sex cells.
- Testing Cell Origin and Biogenesis
- Early scientists, including Redi and Spallanzani, conducted experiments to disprove spontaneous generation. Later, Louis Pasteur’s swan-neck flask experiment became pivotal in demonstrating that life does not spontaneously arise in sterilized environments unless exposed to existing microorganisms.
- Pasteur’s work reinforced the principle of biogenesis—the idea that life only comes from pre-existing life, a cornerstone of modern biology.
- Conditions for the First Cells (Abiogenesis)
- The origin of the first cells likely required specific conditions, such as a reducing atmosphere and high temperatures, which are not common on present-day Earth. This unique environment catalyzed the formation of organic molecules necessary for life.
- The four hypothesized stages for the spontaneous origin of cells include:
- Synthesis of Organic Compounds: Miller and Urey’s experiment demonstrated that amino acids and other organic molecules could form under conditions simulating early Earth, involving a mix of gases (hydrogen, methane, ammonia) and electrical discharges.
- Formation of Polymers: Inorganic compounds and thermal energy from hydrothermal vents could have facilitated the assembly of organic molecules into polymers, such as proteins.
- Membrane Formation: Phospholipids, forming naturally into vesicles, could create enclosed environments, essential for cellular functions.
- Inheritance Mechanisms: RNA, with properties enabling it to self-replicate and act as a catalyst, is hypothesized as the initial genetic material, setting the stage for inheritance.
- The Endosymbiotic Theory and Eukaryotic Cells
- The development of eukaryotic cells is explained by the endosymbiotic theory, which proposes that certain organelles, like mitochondria and chloroplasts, originated from symbiotic relationships between primitive cells.
- In this theory, a larger host cell engulfed smaller bacteria, which then became permanently integrated. This symbiosis enabled the smaller cells to contribute energy to the host, while the host provided protection, leading to a mutually beneficial relationship over time.
- Evidence supporting endosymbiosis includes the fact that mitochondria and chloroplasts contain their own DNA and replicate independently, similar to bacteria.
- Advancements and Implications of Cell Theory
- Cell theory highlights two important implications:
- Continuity of Life: The fact that all cells come from pre-existing cells underlines a continuous lineage from the earliest forms of life to all present-day organisms.
- Rejection of Spontaneous Generation: This principle refines the understanding of life’s origins and shapes biological research, emphasizing that cells cannot arise independently under current Earth conditions.
- Cell theory highlights two important implications:
Through centuries of research, scientists have unraveled complex processes underlying the origin and evolution of cells. These findings underscore an intricate connection between early organic compounds and modern cellular life, explaining how life as we know it may have evolved from the simplicity of abiotic chemistry to the complexity of living organisms.
Characteristics of Cells
Cells are the basic structural and functional units of all living organisms. They carry out essential processes that sustain life, and their intricate organization enables them to perform a variety of vital functions. Below are the key characteristics that define cells:
- Structural Support:
- Cells provide the fundamental structure that supports the body of an organism. Each cell contributes to the shape and stability of tissues and organs, forming the building blocks of life.
- Internal Organization:
- The interior of a cell is highly organized. Various individual organelles within the cytoplasm are enclosed by membranes, allowing them to carry out specialized tasks. This compartmentalization is crucial for maintaining the cell’s internal environment and enabling different processes to occur simultaneously.
- Nucleus and Genetic Information:
- The nucleus, the cell’s central organelle, holds the genetic material (DNA) that governs cell growth, reproduction, and function. This genetic information is vital for passing traits to the next generation and directing the cell’s activities.
- Most cells contain a single nucleus, which acts as the control center for cellular operations.
- Membrane-bound Organelles:
- Besides the nucleus, the cytoplasm contains various membrane-bound organelles, each with specific roles that contribute to the cell’s overall function. These organelles ensure that biochemical reactions are efficiently organized and carried out.
- Mitochondria and Energy Production:
- Mitochondria, often called the “powerhouses” of the cell, are double-membrane-bound organelles responsible for producing energy. They perform energy transactions, converting nutrients into ATP (adenosine triphosphate), the energy currency that powers cellular processes essential for survival.
- Lysosomes and Waste Management:
- Lysosomes play a critical role in the digestion and breakdown of unwanted or harmful materials within the cell. They contain enzymes that degrade waste products, helping to maintain cellular cleanliness and preventing damage from toxic substances.
- Endoplasmic Reticulum and Molecule Synthesis:
- The endoplasmic reticulum (ER) is a vital organelle responsible for synthesizing, processing, and sorting molecules. It assists in producing proteins and lipids, then directs these molecules to their appropriate destinations, contributing to the cell’s internal organization and functionality.
Types of Cells
Cells, often compared to highly organized factories, work together to maintain the life processes of an organism. Each type of cell has specialized functions and structures suited to its role. Based on their structural differences, cells are broadly classified into two main types:
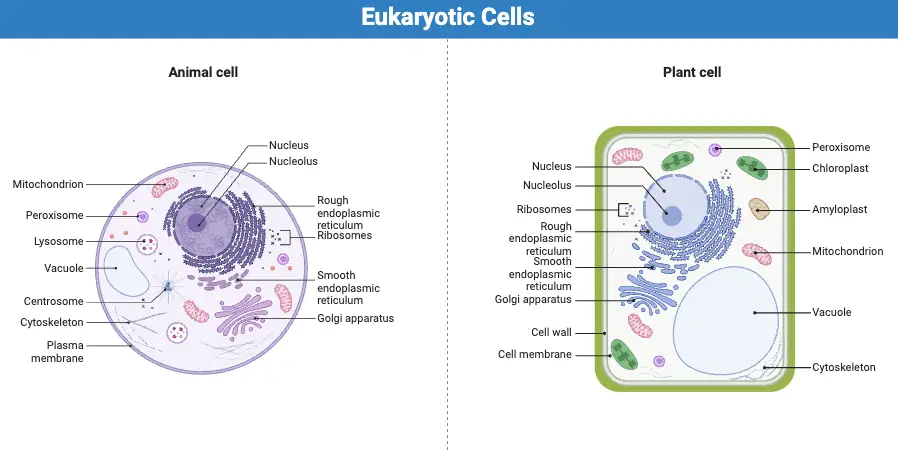
- Prokaryotic Cells:
- Prokaryotic cells lack a true nucleus. Instead, their genetic material is suspended in a region known as the nucleoid.
- These cells are typically single-celled microorganisms, including bacteria, archaea, and cyanobacteria.
- Prokaryotes are much smaller in size, ranging from 0.1 to 0.5 µm in diameter, making them simpler in structure.
- Their hereditary material consists of either DNA or RNA, and unlike eukaryotes, prokaryotes do not have membrane-bound organelles.
- Reproduction in prokaryotes occurs primarily through binary fission, a type of asexual reproduction where a cell splits into two identical offspring. Additionally, some prokaryotes engage in conjugation, a process often considered a form of genetic exchange rather than true sexual reproduction.
- Eukaryotic Cells:
- Eukaryotic cells are more complex and characterized by a true nucleus, which houses the cell’s genetic material.
- These cells are much larger than prokaryotes, typically measuring between 10–100 µm in diameter.
- Eukaryotic cells are found in a wide range of organisms, including plants, fungi, protozoans, and animals.
- The plasma membrane surrounding eukaryotic cells plays a crucial role in regulating the movement of nutrients, electrolytes, and other substances in and out of the cell. It also facilitates communication between cells.
- Eukaryotes reproduce both sexually and asexually, depending on the organism and environmental conditions.
- Contrasting Features in Eukaryotic Cells:
- There are distinct differences between plant and animal eukaryotic cells. For example, plant cells contain chloroplasts, which are essential for photosynthesis, and large central vacuoles for storage. In contrast, animal cells lack these structures but possess other organelles such as lysosomes for digesting cellular waste.
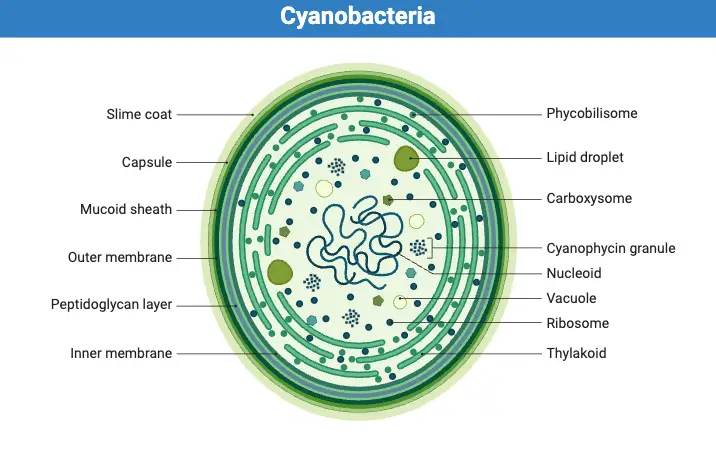
Structure of Cell
The structure of a cell is fundamental to its function, consisting of various components that work together to sustain life. Each part of the cell has a distinct role in carrying out essential biological processes. The following are the key structural elements of a cell:
- Cell Membrane:
- The cell membrane, also known as the plasma membrane, forms the outer boundary of the cell, enclosing all other organelles such as the cytoplasm and the nucleus.
- It serves a protective function, separating the cell from its external environment while regulating the movement of substances in and out. The membrane is porous, allowing selective substances to pass through while maintaining internal balance.
- In multicellular organisms, the cell membrane helps maintain communication between adjacent cells and their environment, forming a barrier that prevents damage and leakage of internal components.
- Cell Wall:
- The cell wall is unique to plant cells and some prokaryotes. It lies outside the cell membrane and provides additional support and protection.
- Composed of cellulose, hemicellulose, and pectin, the cell wall gives plant cells their rigid and stiff structure, helping to maintain their shape and withstand mechanical shocks.
- This structure plays a crucial role in ensuring the stability of plant cells, especially as plants are immobile and need enhanced protection against environmental factors.
- Cytoplasm:
- The cytoplasm is a jelly-like substance that fills the space between the cell membrane and the nucleus. It acts as a medium in which most cellular processes occur.
- It contains numerous organelles, such as the endoplasmic reticulum, mitochondria, and ribosomes, which are suspended in the cytoplasm and perform various functions related to growth, metabolism, and energy production.
- The cytoplasm facilitates the transportation of materials within the cell and plays an active role in cellular reactions.
- Nucleus:
- The nucleus is the control center of the cell, containing the genetic material (DNA) that directs the cell’s activities, including growth, maturation, division, and programmed cell death.
- It is enclosed by the nuclear envelope, which separates the DNA from the rest of the cell, protecting it from damage.
- The nucleus not only houses the hereditary material but also coordinates vital cellular functions by transmitting signals to other parts of the cell.
Cell Organelles
Cells contain a variety of specialized structures called organelles, each performing distinct functions that are vital for the cell’s overall operation. These organelles contribute to key processes such as protein synthesis, energy production, and waste management, ensuring the survival and proper functioning of the cell. Below is a breakdown of the primary cell organelles and their respective roles:
- Nucleolus:
- The nucleolus is responsible for the synthesis of ribosomes, which are essential for protein production. It also plays a role in regulating cell activities and facilitating cell reproduction.
- Nuclear Membrane:
- The nuclear membrane, also known as the nuclear envelope, surrounds and protects the nucleus by creating a boundary between the nucleus and the rest of the cellular components. This separation ensures that the genetic material is safeguarded from external influences.
- Chromosomes:
- Chromosomes are thread-like structures within the nucleus that carry the cell’s hereditary information. In humans, there are 23 pairs of chromosomes in each cell, determining traits such as sex and genetic inheritance.
- Endoplasmic Reticulum (ER):
- The endoplasmic reticulum is a network of membranes involved in the synthesis and transport of proteins and lipids. It plays a significant role in carbohydrate metabolism and the production of lipids and steroids, distributing these substances throughout the cell.
- Golgi Bodies:
- Known as the cell’s “post office,” the Golgi apparatus packages and sorts materials like proteins and lipids for transport to their appropriate destinations. It modifies and prepares molecules for secretion or use within the cell.
- Ribosomes:
- Ribosomes are the sites of protein synthesis. These tiny structures either float freely in the cytoplasm or attach to the ER, facilitating the assembly of amino acids into proteins based on the genetic instructions from the nucleus.
- Mitochondria:
- Referred to as the “powerhouse of the cell,” mitochondria are responsible for producing ATP (adenosine triphosphate), the primary energy source for cellular processes. Through cellular respiration, mitochondria convert nutrients into usable energy.
- Lysosomes:
- Lysosomes contain enzymes that digest and break down waste materials, cellular debris, and foreign substances that enter the cell. Due to their ability to digest cellular components, they are sometimes called the cell’s “suicide bags,” as they can trigger cell death if necessary for cell renewal.
- Chloroplasts:
- Found in plant cells, chloroplasts are the organelles responsible for photosynthesis, the process by which plants convert sunlight into chemical energy. Chloroplasts contain chlorophyll, the pigment that absorbs light energy.
- Vacuoles:
- Vacuoles are storage organelles that hold water, nutrients, and waste products. In plant cells, the central vacuole also helps maintain turgor pressure, supporting the cell’s structure and contributing to its rigidity.
Cell Size
The size of cells plays a crucial role in their function, growth, and ability to exchange materials with their environment. While cells vary greatly in size depending on their type and function, their small size is generally necessary to maintain efficient interactions with their surroundings. The need to optimize the exchange of nutrients, gases, and waste products places limitations on how large a cell can become. The relationship between a cell’s surface area and volume is key to understanding why cells remain small. Here are the important concepts that explain the constraints on cell size:
- Surface Area-to-Volume Ratio:
- As a cell increases in size, its volume grows at a faster rate than its surface area. This imbalance presents a challenge for cells because the surface area represents the space available for the exchange of materials, such as nutrients and gases, between the cell and its external environment. The larger the volume, the more nutrients the cell requires, yet a relatively small surface area would limit the amount of material that can diffuse in and out efficiently.
- For instance, if a cell were to grow too large, it would struggle to obtain sufficient nutrients to sustain its metabolic needs, as its surface area would not be able to support the increased demands of its enlarged volume. Therefore, cells remain small to ensure that their surface area can adequately support the transport of essential materials.
- Adaptations in Larger Cells:
- Some larger cells, like certain types of white blood cells, have evolved mechanisms to overcome the challenges posed by their size. These cells may contain multiple nuclei, allowing them to meet the increased demand for protein synthesis and RNA production needed for cellular functions. This multi-nucleation helps maintain efficiency despite their larger size.
- Moreover, cells that require higher metabolic activity, such as those found lining the small intestine, develop structures that increase their surface area without significantly increasing volume. For example, cells in the intestine form protrusions called microvilli, which increase the surface area available for nutrient absorption. These microvilli help the cell cope with the demands of transporting materials into the cell, even as the overall cell size increases.
- Importance of Cell Size in Function:
- The size of a cell is not arbitrary; it is closely linked to its function. For example, small cells with a high surface area-to-volume ratio are efficient in absorbing nutrients and expelling waste, which is critical for cells with high metabolic rates. Conversely, specialized cells that require more complex functions, such as muscle cells or neurons, may be larger but have adaptations like elongated shapes or branching structures to facilitate material exchange and communication.
Scale of Measurements for Cell Size
Understanding the scale of measurements for cell size is fundamental in biology, as cells are the basic units of life and vary greatly in size depending on their type and function. This knowledge allows researchers and students to conceptualize the dimensions of cells relative to more familiar units of measurement. Cells are measured using the metric system, which includes units such as millimeters, micrometers, and nanometers. Here are the key aspects of measuring cell size:
- Metric Units and Conversions:
- The metric system is commonly used for measuring cell dimensions due to the small scale of most cells. The following conversions are essential for understanding cell size:
- 1 centimeter (cm) = 10 millimeters (mm) = 10−2 meters (m)
- 1 millimeter (mm) = 1000 micrometers (µm) = 10−3 m
- 1 micrometer (µm) = 1000 nanometers (nm) = 10−6 m
- 1 nanometer (nm) = 10−3 µm
- These measurements help illustrate the minute size of cells, which typically ranges from micrometers to nanometers.
- The metric system is commonly used for measuring cell dimensions due to the small scale of most cells. The following conversions are essential for understanding cell size:
- Surface Area and Volume Calculations:
- When considering the physical properties of cells, it is helpful to visualize them as cube-like structures. For example, if a cell has a length of one unit (u), its surface area can be calculated as follows:
- Surface Area: Surface Area=height×width×number of sides×number of boxes=1u×1u×6×1=6u2
- Volume: Volume=height×width×length×number of boxes=1u×1u×1u×1=1u3
- Surface-Area-to-Volume Ratio:
Ratio=Area/Volume = 6/1 = 6
- When considering the physical properties of cells, it is helpful to visualize them as cube-like structures. For example, if a cell has a length of one unit (u), its surface area can be calculated as follows:
- Impact of Cell Size on Surface Area-to-Volume Ratio:
- Considering a larger cell with a length of three units, the calculations yield:
- Surface Area: Surface Area=3u×3u×6×1=54u2
- Volume: Volume=3u×3u×3u×1=27u3
- Surface-Area-to-Volume Ratio: Ratio = 54/27 = 2
- This illustrates how the ratio decreases as cell size increases, indicating that larger cells have a reduced capacity for nutrient exchange compared to smaller cells.
- Considering a larger cell with a length of three units, the calculations yield:
- Comparison of Cell Sizes:
- In practical biological contexts, most cells are significantly smaller than the period at the end of this sentence. For instance:
- The smallest known prokaryotic cells have diameters around 400 nanometers (nm).
- Eukaryotic cells typically range between 1–100 micrometers (µm), with mouse cells measuring approximately 10 µm in diameter.
- An exception to this size range is seen in eggs, which are among the largest single cells. For example, an ostrich egg can be over 10,000 times larger than a mouse cell, showcasing the diversity in cell size across different organisms.
Cell Shape
The diverse shapes of cells observed in both prokaryotic and eukaryotic organisms illustrate the critical relationship between structure and function in biological systems. Each cell type has developed a specific morphology that enhances its functional capabilities, enabling organisms to adapt to various environments and perform specialized tasks effectively. Below are key aspects that highlight the significance of cell shape:
- Structure-Function Relationship:
- The principle of structure-function relationship is evident in how the shape of a cell is tailored to its specific role within an organism. This adaptability ensures that cells can perform their functions efficiently, contributing to the overall survival and functionality of the organism.
- Specialized Cell Shapes:
- Neurons:
- Neurons possess elongated structures with long, thin extensions known as axons and dendrites. These extensions facilitate rapid transmission of chemical and electrical signals across the nervous system, enabling quick communication between different parts of the body.
- Red Blood Cells (Erythrocytes):
- The unique biconcave shape of red blood cells enhances their ability to deform and navigate through narrow capillaries. This design increases the surface area for gas exchange, allowing for efficient transport of oxygen and carbon dioxide throughout the bloodstream.
- Pollen Grains:
- Pollen grains have spiky structures that assist in adherence to pollinators, such as insects or animals. This sticky morphology is crucial for successful pollination, enabling the transfer of genetic material from one flower to another.
- Chlamydomonas:
- The algae Chlamydomonas is characterized by its whip-like flagella, which aid in locomotion through water. The flagella’s shape allows the organism to swim efficiently, facilitating nutrient acquisition and reproduction.
- Neurons:
- Adaptation to Environment:
- The various shapes of cells are not arbitrary; they are adaptations to the specific environmental conditions and functional demands of the organism. For example, cells involved in absorption, such as those lining the intestines, often exhibit increased surface area through microvilli to maximize nutrient uptake.
- Role of Cell Shape in Functionality:
- The shape of a cell can influence its metabolic activity, communication abilities, and overall performance. For instance, a cell with a larger surface area relative to its volume can transport materials more efficiently, a critical factor in cellular respiration and nutrient absorption.
Relationship between Cell Size and Surface Area-to-Volume Ratio
The relationship between cell size and the surface area-to-volume ratio plays a crucial role in cellular function and efficiency. As cells grow, their volume increases at a faster rate than their surface area, which directly impacts their ability to exchange materials with their environment. Understanding this relationship is essential for comprehending why cells maintain specific sizes and how their shapes facilitate their roles within living organisms.
- Surface Area and Volume Calculation:
- The surface area-to-volume ratio (SA) is calculated by dividing the total surface area of a cell by its volume. This ratio provides insight into the efficiency of material exchange between the cell and its surroundings.
- For example, if a cell is modeled as a cube, the surface area (SA) can be calculated using the formula: Surface Area=Height × Width × Number of Sides
and the volume (V) can be determined by: Volume=Height×Width×Length
- Impact of Cell Size on Ratio:
- As a cell increases in size, its volume grows more rapidly than its surface area. For instance, if a cell doubles in size, its volume increases by a factor of eight (2^3), while its surface area only increases by a factor of four (2^2). Consequently, the SAratio diminishes, meaning there is less surface area available for material exchange relative to the volume of the cell.
- Efficient Material Exchange:
- A higher surface area-to-volume ratio is advantageous for smaller cells, as it facilitates more efficient exchange of nutrients, waste products, and gases through the cell membrane. For example, small cells can absorb nutrients and eliminate waste more rapidly than larger cells, which may struggle to meet metabolic demands due to their reduced SAratio.
- This efficiency is particularly important for cells that rely on diffusion for transport, such as those found in tissues where rapid exchange is essential for maintaining homeostasis.
- Inverse Proportionality:
- The relationship between cell size and the surface area-to-volume ratio is inversely proportional. As cell size increases, the SAratio decreases. This inverse relationship limits the maximum size a cell can attain while still performing essential functions effectively. Cells must balance their size with the need for efficient material exchange to support their metabolic activities.
- Biological Implications:
- The limitations imposed by the surface area-to-volume ratio influence the morphology of various cell types. For instance, many specialized cells, such as those lining the intestines, have adaptations like microvilli that increase their surface area, thus enhancing nutrient absorption.
- Additionally, multicellular organisms have developed complex structures, such as tissues and organs, to maintain efficient exchange systems, enabling larger overall sizes while ensuring individual cells remain at optimal sizes for functionality.
Factors Affecting Cell Size and Surface Area-to-Volume Ratio
Cell size and the corresponding surface area-to-volume ratio are influenced by various biological factors. These factors are essential for understanding how cells adapt their structure to meet functional demands and maintain efficient metabolic processes.
- Cell Shape:
- The shape of a cell significantly impacts its surface area-to-volume ratio. Cells that are irregularly shaped or elongated, such as neurons and microvilli found in the small intestine, possess a higher surface area-to-volume ratio compared to spherical cells of the same volume. This increased ratio facilitates enhanced material exchange, crucial for cellular function.
- Membrane Structures:
- Cells can also increase their surface area through structural adaptations such as membrane invaginations or infoldings. For instance, the cristae found in mitochondria and the thylakoid membranes in chloroplasts expand the surface area available for specific cellular processes—like respiration and photosynthesis—without a corresponding increase in cell volume. This adaptation allows for more efficient biochemical reactions and energy production.
- Adaptations in Unicellular Organisms:
- Unicellular organisms often exhibit adaptations that allow them to alter their shape and surface area-to-volume ratio based on their environmental needs. For example, amoebae can extend pseudopodia for feeding and locomotion, effectively changing their surface area for nutrient absorption or movement. This flexibility is vital for survival, especially in fluctuating environments.
- Specialization in Multicellular Organisms:
- In multicellular organisms, specialized cells and tissues develop to maintain efficient exchange of materials over larger distances. For instance, the circulatory and respiratory systems work together to ensure that nutrients and gases are transported effectively throughout the body. This specialization helps maintain homeostasis, ensuring that individual cells function optimally despite being part of a larger organism.
- Metabolic Demands:
- The metabolic activity of a cell also influences its size and shape. Cells with high metabolic rates often require larger surface areas to accommodate increased transport needs for nutrients and waste removal. Thus, cells involved in secretion, absorption, or other intensive metabolic processes may evolve shapes that enhance their surface area-to-volume ratios.
Limitations of Cell Size
The size of a cell is inherently constrained by several biological and physical limitations that directly affect its functionality and efficiency. Understanding these limitations is crucial for comprehending the cellular processes and adaptations that support life.
- Constraints on Material Exchange:
- As a cell grows, its surface area-to-volume ratio decreases significantly. This reduction limits the rate of exchange of materials—such as nutrients, waste products, and gases—across the cell membrane. Therefore, larger cells may struggle to uptake sufficient nutrients, leading to deficiencies and the accumulation of metabolic waste.
- The efficiency of molecular diffusion within the cell also diminishes as size increases. This inefficiency can result in uneven distribution of essential materials, adversely affecting cellular reactions. The slower diffusion rates in larger cells may hinder metabolic processes critical for maintaining cellular homeostasis.
- Homeostasis Challenges:
- Larger cells face increased challenges in maintaining homeostasis due to the greater distance between the cell surface and the cell’s interior. This physical separation can impede the rapid exchange of materials necessary for cellular balance, thus complicating the cell’s ability to respond effectively to environmental changes.
- Consequently, the efficiency of vital cellular processes, such as signal transduction and nutrient uptake, may be compromised in larger cells. The struggle to achieve equilibrium can lead to cellular stress and a diminished ability to adapt to fluctuations in the external environment.
- Energy Requirements and Cellular Processes:
- The energy demands of larger cells also present limitations. As cell size increases, the energy required to sustain cellular functions and transport materials across greater distances within the cell escalates. This increased energy demand can strain the cell’s resources, potentially reducing overall cellular efficiency and productivity.
- Additionally, larger cells may encounter difficulties in adequately synthesizing adenosine triphosphate (ATP), the energy currency of the cell. Efficient ATP production is essential for driving metabolic processes, and any shortfall can directly impact the cell’s operational capacity.
- Genetic Control and Regulation:
- The relationship between cell size and genetic regulation further constrains cell growth. As cells increase in size, the ratio of DNA to cytoplasm decreases. This diminishing ratio can limit the cell’s ability to effectively control various cellular processes, including gene expression and protein synthesis.
- The reduced availability of genetic material relative to cytoplasmic volume may hinder the cell’s capacity to produce necessary proteins in response to metabolic demands. This limitation can result in impaired cellular functions and the inability to meet the physiological needs of the organism.
Adaptations for Surface Area-to-Volume Optimization
Cells have developed a variety of adaptations to optimize their surface area-to-volume ratio, facilitating efficient exchange of materials and supporting essential biological functions. These adaptations are critical for maintaining cellular homeostasis and ensuring that metabolic processes proceed effectively.
- Cell Shape and Membrane Modifications:
- Many cells adopt irregular or elongated shapes to enhance their surface area without a corresponding increase in volume. For instance, neurons possess long, thin extensions known as axons and dendrites. These structures maximize the surface area available for signal transmission, allowing rapid communication between nerve cells.
- Microvilli, found in the epithelial cells of the small intestine, are finger-like projections that dramatically increase surface area for nutrient absorption. The expanded surface area facilitates greater interaction with digested food, enhancing the efficiency of nutrient uptake.
- The biconcave shape of red blood cells is another adaptation that maximizes surface area for efficient gas exchange while minimizing cell volume. This unique morphology allows for optimal oxygen transport as the cells navigate through narrow capillaries.
- Cells can also develop invaginations or infoldings of their cell membranes to expand the surface area available for specific functions. For example, the cristae within mitochondria provide a large surface area for ATP synthesis, optimizing energy production within the cell. Similarly, thylakoid membranes in chloroplasts increase the surface area available for photosynthesis, promoting effective light absorption and conversion into chemical energy.
- Multicellular and Colonial Adaptations:
- In multicellular organisms, the evolution of specialized cells and tissues allows for efficient transport of materials across larger distances. The circulatory system is a prime example, as it facilitates the movement of nutrients, waste products, and gases throughout the body, overcoming the limitations imposed by distance and cell size.
- The respiratory system also plays a vital role in optimizing surface area for gas exchange. Structures such as alveoli in the lungs significantly increase the surface area available for oxygen and carbon dioxide exchange, enhancing respiratory efficiency.
- Some unicellular organisms exhibit remarkable adaptability by changing their shape to increase surface area for various functions. Amoebae, for instance, can extend pseudopodia for feeding and locomotion. This ability to modify shape in response to environmental conditions allows them to optimize nutrient absorption and mobility.
- In certain cases, cells may aggregate to form colonies or filamentous structures, effectively increasing their collective surface area while maintaining a small individual cell size. Examples include spherical colonies of algae, such as Volvox, and filamentous cyanobacteria. These adaptations enhance nutrient acquisition and environmental interaction while preserving the advantages of smaller cells.
Cell Theory
Cell theory, a fundamental principle in biology, was initially proposed by German scientists Theodor Schwann, Matthias Schleiden, and Rudolf Virchow. It serves as a unifying concept that applies to all living organisms, describing the basic structural and functional unit of life. This theory laid the foundation for our understanding of biological organization. The key principles of cell theory include:
- All living organisms are composed of one or more cells:
- This postulate emphasizes that whether an organism is unicellular (composed of a single cell) or multicellular (composed of many cells), cells are the basic building blocks that form the structure of every living entity.
- The cell is the basic unit of life:
- Cells are the smallest unit that can perform all life processes, such as metabolism, growth, and reproduction. Therefore, the cell is the fundamental unit of both structure and function in living organisms.
- All cells arise from pre-existing cells:
- This principle establishes that new cells are produced from the division of existing cells. It rejects earlier theories of spontaneous generation, instead stating that the continuity of life is maintained through cellular reproduction.
As biology advanced, modern revisions of cell theory were developed to account for more complex cellular functions. These additional postulates expand upon the original theory and include:
- Energy flow occurs within cells:
- All living cells undergo metabolic processes where energy is produced, stored, and utilized to maintain cellular activities. This energy flow is essential for sustaining life.
- Genetic information is passed from one cell to another:
- The transmission of genetic material, stored in DNA, ensures the continuity of life. During cell division, DNA is replicated and passed on to daughter cells, preserving genetic traits and enabling inheritance.
- All cells share a similar chemical composition:
- Despite the diversity of organisms, the chemical makeup of cells, including molecules like proteins, lipids, carbohydrates, and nucleic acids, remains largely consistent across species. This similarity underscores the shared biochemical processes that drive cellular functions.
Together, these principles form a cohesive framework for understanding the structure and function of cells, reinforcing the idea that cells are central to all forms of life. Cell theory not only highlights the universality of cellular organization but also underscores the continuity of life from one generation to the next through cellular reproduction and genetic transmission.
Functions of Cell
Cells perform a variety of crucial functions essential for the survival, growth, and reproduction of organisms. Each cell operates as a self-contained unit while contributing to the overall function of the organism. The primary functions of cells can be outlined as follows:
- Provides Support and Structure:
- Cells serve as the structural foundation of all living organisms. In multicellular organisms, cells group together to form tissues, providing support and shape to the organism. For example, the cell wall in plants and the cell membrane in animals are vital in maintaining the organism’s physical structure. The skin in animals consists of multiple layers of cells, while in plants, xylem cells provide structural support to help the plant remain upright.
- Facilitates Growth through Mitosis:
- Growth in organisms is facilitated through the process of mitosis, where a single parent cell divides to produce two genetically identical daughter cells. This process allows the organism to grow by increasing the number of cells. Mitosis is a critical part of tissue repair, cellular replacement, and overall growth in multicellular organisms.
- Enables Transport of Substances:
- Cells regulate the transport of nutrients, gases, and waste products across their membranes. Nutrients required for metabolic activities enter the cell, and waste products are expelled. This transport can occur through passive mechanisms, such as diffusion, where molecules like oxygen and carbon dioxide move across the cell membrane following a concentration gradient. Alternatively, active transport involves the use of cellular energy to move larger or charged molecules against the concentration gradient, ensuring cells receive necessary materials for survival.
- Energy Production:
- Cells are responsible for producing energy, which is crucial for driving biological processes. In plant cells, energy is produced through photosynthesis, where light energy is converted into chemical energy in the form of glucose. In animal cells, energy is produced through cellular respiration, where glucose is broken down to release ATP (adenosine triphosphate), the cell’s energy currency. This energy is used to fuel various cellular activities, from growth to movement.
- Aids in Reproduction:
- Cells play a central role in reproduction through processes such as mitosis and meiosis. Mitosis allows for asexual reproduction, where daughter cells are genetically identical to the parent cell. This is important for growth, tissue repair, and maintenance of an organism’s body. Meiosis, on the other hand, is crucial for sexual reproduction, leading to the formation of gametes (sperm and egg cells). Meiosis results in daughter cells that are genetically different from the parent cells, contributing to genetic diversity in sexually reproducing organisms.
How Did the First Cell Appear?
The appearance of the first cell on Earth likely unfolded through a series of complex biochemical steps, gradually evolving from simple molecules into self-replicating, membrane-bound structures. Below, we explore these stages in a structured sequence, outlining the chemical and environmental conditions necessary for the formation of the first cell.
- Formation of Organic Molecules
Organic molecules like proteins, nucleic acids, sugars, and fats are fundamental building blocks for life. The origin of these molecules may have involved two main scenarios:- Terrestrial Origin
On early Earth, the synthesis of complex organic molecules could have occurred in extreme conditions. Laboratory experiments, like the Miller-Urey experiment, simulated these conditions by exposing mixtures of CO₂, methane, ammonia, and hydrogen to electric discharges and heat. This led to the formation of amino acids, sugars, and other precursors of life. - Extraterrestrial Origin
Some organic molecules may have arrived on Earth via comets and meteorites. These celestial bodies often contain complex organic compounds, which could have contributed to the pool of molecules necessary for life.
- Terrestrial Origin
- Formation of Organic Polymers
While simple organic molecules are essential, the first cell required polymers, such as proteins and nucleic acids. Several theories propose how these polymers may have formed:- Heating and Drying Cycles
Semi-dry conditions, along with heating, can facilitate the formation of chains from simple organic molecules. This process may have generated polymers under early Earth conditions. - Mineral Surface Catalysis
Minerals play a crucial role by concentrating and catalyzing reactions among organic molecules. They may have served as scaffolds, allowing molecules to interact and form more complex structures. - Hydrothermal Vents and Hot Springs
High-temperature environments like hydrothermal vents provide conditions that could facilitate polymer formation. Minerals in these locations concentrate and catalyze organic reactions, especially in freshwater environments with lower ion concentrations that support membrane formation.
- Heating and Drying Cycles
- Lipid Membranes and Compartmentalization
Membranes, composed of lipid molecules, were a key step in the evolution of the first cell, creating a barrier that separates internal and external environments:- Formation of Lipid Vesicles
Lipids can spontaneously form vesicles or membrane-bound structures in aqueous environments. This allows the concentration of organic molecules within a protected space, enhancing the efficiency of reactions by preventing the diffusion of critical compounds. - Advantages of Membranes
Membranes support homeostasis, allowing protocells to maintain optimal internal conditions. They also enable “selfish” evolution by ensuring that advantageous molecules are not shared with neighboring structures, supporting the protocell’s growth and competitive survival.
- Formation of Lipid Vesicles
- Development of Protocells
Protocells represent an early, membrane-bound assembly of molecules that could divide and grow:- Growth and Division
Vesicles may have expanded by absorbing small molecules from the environment. As they grew, these vesicles eventually divided, a process that potentially led to self-replicating, cell-like structures. - Internal Molecular Concentration
Inside these vesicles, organic molecules—such as amino acids and nucleotides—could concentrate and react, eventually leading to the formation of more complex molecular systems.
- Growth and Division
- Self-Replication and the Emergence of Genetic Material
A crucial development in the origin of the first cell was the ability to replicate molecular structures:- Polymer Copying and Mutations
Within the vesicles, certain polymers, possibly RNA molecules, may have started to self-replicate. Errors in replication led to variations, giving rise to molecules with different structures and properties. This variability introduced the possibility of natural selection among protocells. - Autoreplication and Natural Selection
Membrane-bound vesicles with efficient self-replicating molecules had advantages over others, leading to competition for resources and the beginning of Darwinian evolution.
- Polymer Copying and Mutations
- The RNA World Hypothesis
The RNA World model suggests that RNA molecules, capable of both storing genetic information and catalyzing reactions, might have been the earliest self-replicating structures.- Ribozymes and RNA Replication
Certain RNA molecules, known as ribozymes, can perform catalytic functions. RNA could have copied itself through complementary base pairing, with mutations further increasing diversity and competition.
- Ribozymes and RNA Replication
- The Metabolic World Hypothesis
An alternative model, the Metabolic World, proposes that a network of chemical reactions involving various molecules developed prior to cellular organization.- Chemical Networks and Early Membranes
Instead of a single self-replicating molecule, this model suggests that complex molecular interactions within a membrane-bound structure laid the groundwork for cellular life.
- Chemical Networks and Early Membranes
- The Genetic Code and Transition to DNA
At a certain point, the synthesis of proteins began, with RNA assisting in the process. This introduced a genetic code where sequences of nucleotides corresponded to amino acids, forming the basis for protein synthesis.- DNA as the Genetic Material
Eventually, genetic information was transferred from RNA to the more stable DNA. This shift allowed for easier replication and repair of genetic material, setting the foundation for modern cellular life.
- DNA as the Genetic Material
This progression—from simple organic molecules to protocells with membranes, self-replicating systems, and genetic coding—illustrates a plausible sequence for the appearance of the first cell. Each step reflects a combination of chemical interactions, environmental influences, and molecular evolution, forming a framework for the earliest stages of life on Earth.
Practice MCQ
- https://library.fiveable.me/ap-bio/unit-2/cell-size/study-guide/3oB8hJyGwvYACz8XlUmG
- https://biologydictionary.net/ap-biology/2-3-cell-size/
- https://www.khanacademy.org/science/ap-biology/cell-structure-and-function/cell-size/e/cell-size-exercise
- https://byjus.com/biology/cells/
- https://www2.nau.edu/lrm22/lessons/cell_size/cell_size.html
- https://flexbooks.ck12.org/cbook/ck-12-advanced-biology/section/4.5/primary/lesson/cell-size-and-shape-advanced-bio-adv/
- https://library.fiveable.me/ap-bio-hiram-high-school-glenn-fall-24/unit-3/cell-size/study-guide/boh9FjvX6qCqIUBy
- https://hvspn.com/captivate-podcast/apbio56/
- https://studyrocket.co.uk/revision/ap-biology-college-board/cell-structure-and-function/cell-size
- https://croteaubio.wordpress.com/ap-bio-tutorials/tutorials/cell-size/
- https://biologyjunction.com/cell-size/
- https://www.britannica.com/science/cell-biology
- https://byjus.com/biology/cells-size-shape-count/
- https://www.mrgscience.com/topic-15-origin-of-cells.html
- https://www.cliffsnotes.com/study-guides/biology/biology/the-origin-and-evolution-of-life/origin-of-cells
- https://mmegias.webs.uvigo.es/02-english/5-celulas/1-origen_celula.php
- https://www.ncbi.nlm.nih.gov/books/NBK9841/