What are Carbohydrates?
- Carbohydrates are essential biomolecules composed of carbon, hydrogen, and oxygen atoms, typically with a 2:1 hydrogen-to-oxygen ratio, as seen in water. However, not all carbohydrates strictly follow this rule. In biochemical contexts, carbohydrates are synonymous with saccharides, a term derived from the Greek word for sugar. These saccharides are divided into four main types: monosaccharides, disaccharides, oligosaccharides, and polysaccharides, with the simpler ones, monosaccharides and disaccharides, commonly known as sugars.
- Monosaccharides, like glucose and fructose, are the simplest forms of carbohydrates and serve as the fundamental building blocks. Disaccharides, such as sucrose and lactose, consist of two monosaccharide units. The more complex carbohydrates, oligosaccharides, and polysaccharides are composed of longer chains of monosaccharide units. Starch and glycogen, which are polysaccharides, serve as vital energy storage molecules in plants and animals, respectively. Another important polysaccharide, cellulose, provides structural support in plant cell walls.
- Carbohydrates play numerous roles in living organisms. Polysaccharides like starch and glycogen store energy, while cellulose and chitin serve as structural components in plants and arthropods. Furthermore, carbohydrates like ribose and deoxyribose are integral parts of nucleic acids, RNA and DNA, respectively. These biomolecules also contribute to critical processes like immune response, blood clotting, and cellular development.
- In human nutrition, carbohydrates are abundant in various foods. Starches, found in grains such as wheat, maize, and rice, as well as in potatoes, are major dietary sources of carbohydrates. Sugars, including glucose and fructose, are naturally present in fruits, honey, and some vegetables, while sucrose and lactose are common in table sugar and dairy products. Processed foods, including bread, pasta, and sweets, are also rich in carbohydrates.
- Dietary fiber, a form of carbohydrate, is crucial for maintaining digestive health. Though indigestible by humans, fibers like cellulose aid in bowel movements and support a healthy digestive system. Other fibers, such as resistant starch and inulin, nourish beneficial gut bacteria, which, in turn, produce short-chain fatty acids essential for gut health.
- Overall, carbohydrates are central to both biological functions and human nutrition, making them a crucial topic of study in biochemistry, biology, and nutrition. Their diverse roles range from providing energy and structural support to regulating complex biological processes.
Definition of Carbohydrates
Carbohydrates are organic molecules made of carbon, hydrogen, and oxygen that serve as a major source of energy in living organisms. They include sugars, starches, and fibers and are classified into monosaccharides, disaccharides, oligosaccharides, and polysaccharides. Carbohydrates play key roles in energy storage, structural support, and various biological processes.
Carbohydrates Formula
Carbohydrates are macromolecules that consist comprised of carbon (C) as well as hydrogen (H) and oxygen (O) and possess the standard Cx(H2O)y formula. Carbohydrates use the general formula Cx(H2O)y. Carbon’s hydrate is referred to as carbohydrates. They are composed of oxygen and hydrogen in the same proportions like water. It is worth noting that there are certain carbohydrate that don’t comply with the formula Cx(H2O)y such as 2-deoxyribose C5H10O4. However, the majority of them are in line with the formula Cx(H2O)y.
Carbohydrates are also referred to as sugars. They include partially methylated sugars as well as amino sugars and amino sugars that naturally occur and one nitro sugar that is natural is recognized. All carbohydrates are polyhydroxyaldehydes or ketones or other substances that release the same by hydrolysis.
Haworth projections depict the polysaccharides’ cyclic structure. Monosaccharides are composed of one of two groups: an aldehyde (aldose) or an ketose group (ketose) and a variety of OH groups. Straight chain sugars are cyclized in solution into ring-like structures with an linkage with ether. Glycosidic bonding occurs between monosaccharides, forming disaccharides and polysaccharides. Carbohydrates are utilized to provide energy sources and reserves.
Carbohydrate Sources
We all know that carbohydrates are an essential component of a human’s diet. Common sources of carbohydrates include:
- Potatoes
- Maze
- Milk
- Popcorn
- Bread
Characteristics of Carbohydrates
Carbohydrates represent a significant class of organic compounds essential for various biological functions, particularly as energy sources in living organisms. Understanding the characteristics of carbohydrates facilitates a deeper comprehension of their roles in nutrition, metabolism, and cellular structure.
- Organic Composition:
- Carbohydrates are organic compounds characterized primarily by the presence of carbon (C), hydrogen (H), and oxygen (O), often with nitrogen (N) as a minor component.
- They generally follow the empirical formula Cn(H2O)n\text{C}_n(\text{H}_2\text{O})_nCn(H2O)n, indicating a characteristic ratio of hydrogen to oxygen of approximately 2:1, similar to water.
- This ratio is a defining feature, leading to the term “carbohydrate,” which means “hydrates of carbon.” However, some carbohydrates do not strictly adhere to this formula, demonstrating the diversity within this class of compounds.
- Types of Carbohydrates:
- Carbohydrates can be classified into three main categories: monosaccharides, disaccharides, and polysaccharides.
- Monosaccharides are the simplest forms, consisting of single sugar units such as glucose and fructose.
- Disaccharides, like sucrose and lactose, are formed from the combination of two monosaccharides via glycosidic bonds.
- Polysaccharides, such as starch, glycogen, and cellulose, are long chains of monosaccharide units and serve various structural and energy-storage roles.
- Carbohydrates can be classified into three main categories: monosaccharides, disaccharides, and polysaccharides.
- Energy-Rich Biomolecules:
- Carbohydrates are crucial energy-rich biomolecules, providing a primary source of chemical energy for many organisms.
- The metabolism of glucose, particularly during cellular respiration, exemplifies their role in energy production.
- Through a series of enzymatic reactions, glucose is converted into pyruvate in glycolysis, followed by further oxidation in the Krebs cycle, culminating in ATP production through the electron transport chain.
- Nutritional Significance:
- As major nutrients, carbohydrates supply energy necessary for various biological functions.
- They are particularly important in human nutrition, providing readily available energy through dietary sources.
- While many carbohydrates are digestible, others exist in fibrous forms, contributing to dietary fiber.
- Dietary Fiber:
- Fibrous carbohydrates, including mucilages, pectins, gums, and structural components like cellulose and lignin, are not readily digestible by humans.
- These carbohydrates play vital roles in digestive health, promoting bowel regularity and preventing certain diseases.
- Ruminants, such as cattle and sheep, possess unique adaptations allowing them to digest fibrous plant materials that humans cannot.
- Symbiotic Relationships:
- In ruminants, specific symbiotic bacteria, such as Ruminococcus, Fibrobacter, Streptococcus, and Escherichia, inhabit the rumen and facilitate the degradation of cellulose into simpler carbohydrates.
- This symbiotic relationship illustrates the importance of carbohydrates not only as energy sources but also in promoting complex interspecies interactions that enhance nutrient availability.
Structure of Carbohydrates
Carbohydrates are organic molecules primarily composed of carbon, hydrogen, and oxygen atoms. Their structure can vary significantly, influencing their function in biological systems. Below is a detailed breakdown of carbohydrate structures:
- Basic Composition:
- Carbohydrates follow the empirical formula (CH₂O)ₙ, where “n” is the number of carbon atoms.
- They consist of aldehyde or ketone groups along with multiple hydroxyl (OH) groups attached to a carbon backbone.
- Monosaccharides:
- The simplest form of carbohydrates are monosaccharides, which serve as the fundamental units. These can be classified based on their functional groups:
- Aldoses: Monosaccharides containing an aldehyde group (-CHO) at the end of the carbon chain.
- Ketoses: Monosaccharides with a ketone group (C=O) within the carbon chain.
- Examples include glucose (an aldose) and fructose (a ketose).
- The simplest form of carbohydrates are monosaccharides, which serve as the fundamental units. These can be classified based on their functional groups:
- Open-Chain Structure:
- In this linear form, carbohydrates show a straight backbone of carbon atoms with hydroxyl groups attached to each carbon, except for the carbonyl group (aldehyde or ketone).
- Monosaccharides like glucose can exist in this form, especially in solution, but often convert to other structures.
- Hemi-Acetal Structure:
- When the aldehyde or ketone group in a monosaccharide reacts with a hydroxyl group within the same molecule, it forms a hemi-acetal (or hemi-ketal for ketoses).
- This reaction results in the formation of a ring structure, in which one oxygen atom forms a bridge between two carbon atoms. This intramolecular bond transforms the molecule into a cyclic structure.
- Haworth Structure:
- The cyclic form of monosaccharides is commonly represented in the Haworth structure, particularly for six-membered rings called pyranoses (e.g., glucose) and five-membered rings called furanoses (e.g., fructose).
- This structure provides a three-dimensional perspective of the sugar molecule, illustrating how the ring is closed and the relative positions of the functional groups.
- Polysaccharides and Oligosaccharides:
- Monosaccharides can link together to form longer chains known as polysaccharides (if the chain has many units) or oligosaccharides (if there are only a few units).
- Examples include starch, glycogen, and cellulose, where monosaccharides are joined by glycosidic bonds, creating larger, more complex carbohydrates.
- Modified Monosaccharides:
- Some carbohydrates contain modified monosaccharides where specific groups, such as hydroxyls, are replaced or removed.
- For instance, deoxyribose, a component of DNA, is a derivative of ribose where one hydroxyl group has been removed.
- Other examples include N-acetylglucosamine, which forms the repeating units in chitin, found in arthropod exoskeletons.
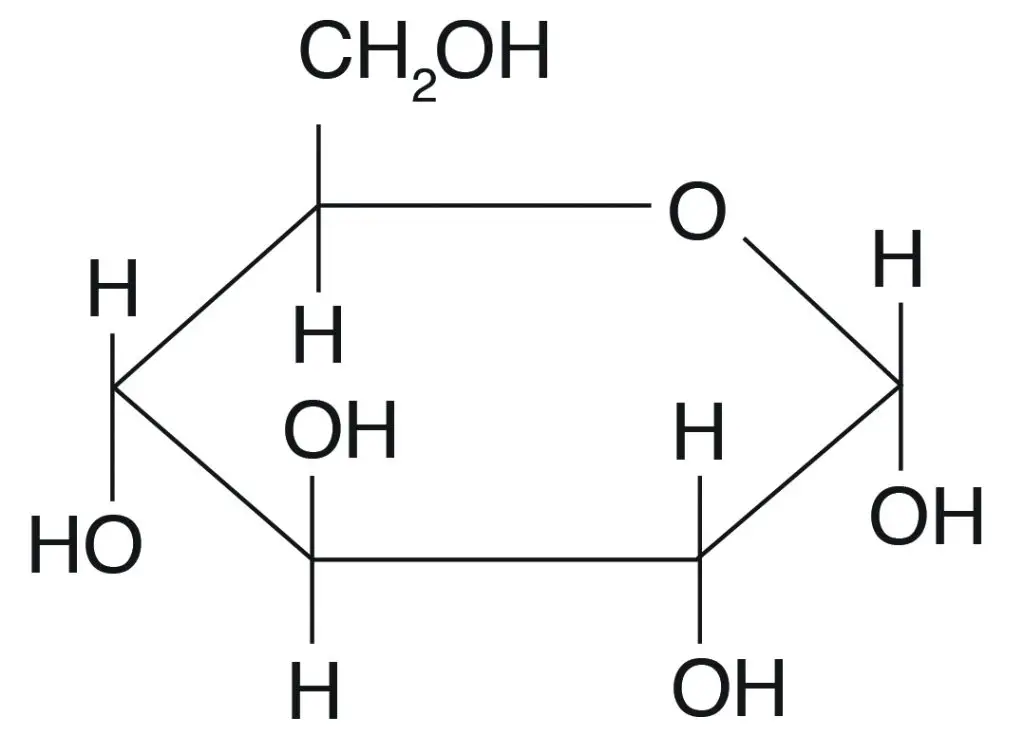
Properties of Carbohydrates
Carbohydrates are essential biomolecules with a wide range of physical and chemical properties. These properties contribute to their functionality in biological systems, food applications, and beyond. Understanding their characteristics, including stereochemistry, optical activity, isomerism, anomerism, and chemical reactivity, provides insight into their role in life processes and industrial applications.
- Stereoisomerism
Carbohydrates can exist as stereoisomers, compounds that share the same molecular formula but differ in the spatial arrangement of their atoms. A well-known example is glucose, which has two stereoisomers: D-glucose and L-glucose. These isomers are differentiated based on the arrangement around the penultimate carbon atom (the second to last carbon in the chain). - Optical Activity
Carbohydrates are optically active, meaning they can rotate the plane of polarized light. For example, glucose can exist as two optical isomers: (+) glucose, which rotates light in a clockwise direction, and (-) glucose, which rotates light counterclockwise. The direction of light rotation is an important property for distinguishing between different carbohydrate forms. - Diastereoisomers
Diastereoisomers are a type of stereoisomer where the configuration differs at one or more but not all chiral centers. In carbohydrates, diastereoisomers are created when the arrangement around specific carbons—like C2, C3, or C4—differs. For instance, glucose, mannose, and galactose are diastereoisomers of each other. - Anomerism
Anomerism refers to the specific configuration of a carbohydrate at its anomeric carbon, which is carbon 1 in aldoses and carbon 2 in ketoses. This property plays a key role in the behavior of carbohydrates in solutions and their reactivity, as it affects the molecule’s ability to form glycosidic bonds. - Chemical Reactivity
Carbohydrates exhibit several chemical reactions that are crucial for their identification and functional use:- Osazone Formation
Carbohydrates can react with excess phenylhydrazine to form osazone crystals, such as glucosazone from glucose. This reaction is used to identify and distinguish carbohydrates based on the shapes and melting points of the resulting osazones. - Benedict’s Test
Reducing sugars, including many monosaccharides and some disaccharides, undergo oxidation in the presence of Benedict’s reagent. When heated, they reduce the copper(II) ions in the reagent to copper(I) oxide, resulting in a color change from blue to orange-red or brick red, indicating the presence of reducing sugars. - Oxidation
Monosaccharides like D-glucose are reducing sugars due to their aldehyde group, which can be oxidized to carboxylic acids, such as D-gluconic acid. This oxidation forms the basis for various analytical tests for reducing sugars. - Reduction to Alcohols
In their open-chain forms, carbohydrates can undergo reduction reactions. Reducing agents such as sodium borohydride (NaBH4) can convert the carbonyl group in monosaccharides to an alcohol, forming compounds known as alditols.
- Osazone Formation
- Properties of Monosaccharides
- Sweet Taste
Most monosaccharides have a naturally sweet flavor. Among them, fructose is the sweetest, approximately 73% sweeter than sucrose (table sugar). - Solid at Room Temperature
Monosaccharides are typically found as crystalline solids at room temperature, contributing to their stability and ease of storage. - Water Solubility
Monosaccharides are highly soluble in water due to the numerous hydroxyl (OH) groups in their structures. This high solubility allows them to form syrups even with small amounts of water.
- Sweet Taste
- Properties of Disaccharides
- Sweetness
Disaccharides also have a sweet taste, though their sweetness levels vary. For example, sucrose (composed of glucose and fructose) is commonly recognized as table sugar. - Solubility
Like monosaccharides, disaccharides dissolve readily in water because of their hydroxyl groups. This makes them useful in food and beverage production. - Reducing Power
Certain disaccharides, such as maltose and lactose, are reducing sugars. They retain the ability to reduce other compounds due to a free anomeric carbon in their structure. - Hydrolysis
Disaccharides can undergo hydrolysis, breaking down into their monosaccharide components through reaction with water. Enzymes such as sucrase, maltase, and lactase facilitate this process, particularly in the digestive system.
- Sweetness
- Properties of Polysaccharides
- Molecular Weight and Size
Polysaccharides consist of long chains of monosaccharide units, resulting in high molecular weight. Their large size and complexity impart properties like high viscosity and gel formation in water-based systems. - Insolubility
Many polysaccharides, including cellulose and chitin, are insoluble in water due to their extensive hydrogen bonding and crystalline structures. This insolubility plays a key role in their function as structural components, such as in plant cell walls and the exoskeletons of arthropods. - Storage and Energy Reserve
Polysaccharides like starch in plants and glycogen in animals serve as storage forms of glucose. Their branched structures allow for efficient storage and quick access to glucose when energy is required. - Structural Integrity
Polysaccharides such as cellulose and chitin provide structural support in biological systems. For example, cellulose gives rigidity to plant cell walls, while chitin forms part of the exoskeleton in arthropods. - Functionality in the Food Industry
Certain polysaccharides, including pectin and agar, are used in food products as thickeners, stabilizers, and gelling agents. Their unique physical properties contribute to the texture and stability of various food items.
- Molecular Weight and Size
Classification of Carbohydrates – Types of Carbohydrates
Carbohydrates, an essential group of biomolecules, can be classified based on the number of sugar units they contain, ranging from simple to complex forms. These classifications help to understand their distinct structures and functions within living organisms.
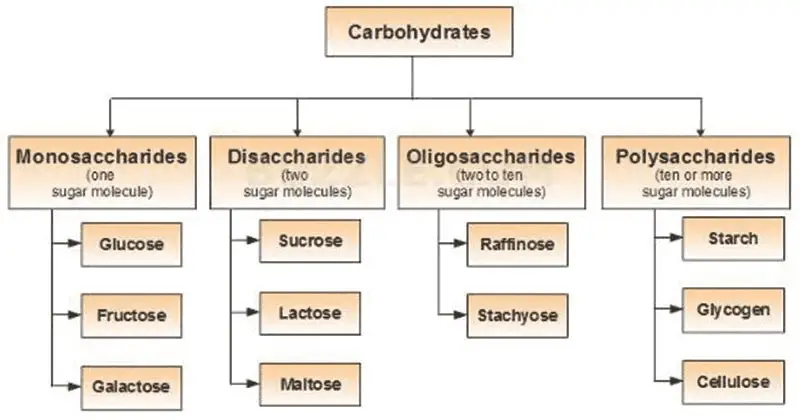
A. Monosaccharides
Monosaccharides represent the most fundamental unit of carbohydrates, characterized by their inability to be further hydrolyzed into simpler sugar molecules. As the basic building blocks of larger carbohydrate structures, these simple sugars are crucial for various biological processes.
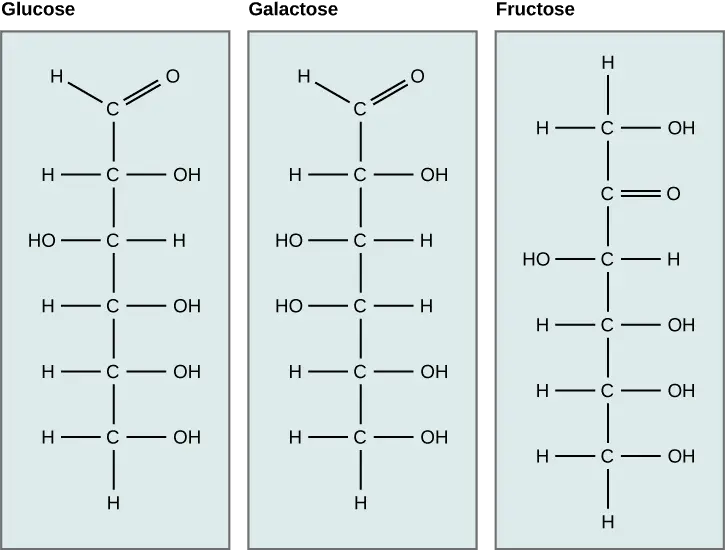
- Structure:
- Monosaccharides possess either a free aldehyde group (—CHO) or a ketone group (=CO), accompanied by two or more hydroxyl groups (—OH).
- They follow the general chemical formula (CH₂O)ₙ, where “n” indicates the number of carbon atoms, typically ranging from three to seven.
- The smallest monosaccharides include dihydroxyacetone and D- and L-glyceraldehydes, with n=3.
- Classification by Carbonyl Group:
- Aldoses: These monosaccharides contain an aldehyde group located at the terminal end of the molecule. Examples include glucose and galactose.
- Ketoses: These contain a ketone group within the structure, such as fructose.
- Classification by Carbon Number:
- Trioses: Monosaccharides with three carbon atoms.
- Tetroses: Monosaccharides with four carbon atoms.
- Pentoses: Monosaccharides with five carbon atoms.
- Hexoses: Monosaccharides with six carbon atoms, which are the most common type in nature.
- Heptoses: Monosaccharides with seven carbon atoms.
- D and L Forms:
- Monosaccharides can exist in D- or L- configurations based on the stereochemistry around the asymmetric carbon furthest from the carbonyl group. This classification affects their biochemical behavior and interactions.
- Examples of Monosaccharides:
- Glucose: Known as an aldohexose (C₆H₁₂O₆), glucose is the most abundant monosaccharide and can be found in both open-chain and cyclic forms. In its ring structure, it exhibits two anomers—α-glucose and β-glucose—differentiated by the position of the hydroxyl group on the anomeric carbon. Glucose is a primary energy source in human metabolism and is pivotal for cellular respiration.
- Fructose: A common ketose found in fruits and honey, fructose shares the same chemical formula as glucose but differs in structural arrangement. It plays a significant role in energy production and is utilized by the body as an energy source.
- Galactose: This aldohexose is primarily known for its presence in lactose, the sugar found in milk. Galactose is essential for various metabolic processes and contributes to energy production when metabolized.
- Ribose and Ribulose: Pentoses like ribose serve as structural components of nucleic acids (RNA) and play vital roles in cellular function and energy transfer through coenzymes. Ribulose, another pentose, is involved in the Calvin cycle of photosynthesis.
- Functions:
- Monosaccharides provide a rapid source of energy, essential for cellular metabolism. For instance, glucose is metabolized through glycolysis to produce adenosine triphosphate (ATP), the energy currency of cells.
- They act as precursors in the biosynthesis of larger biomolecules, including nucleic acids and complex carbohydrates.
- Monosaccharides contribute to the structural integrity of cellular components, forming part of glycoproteins and glycolipids that facilitate cell recognition and signaling.
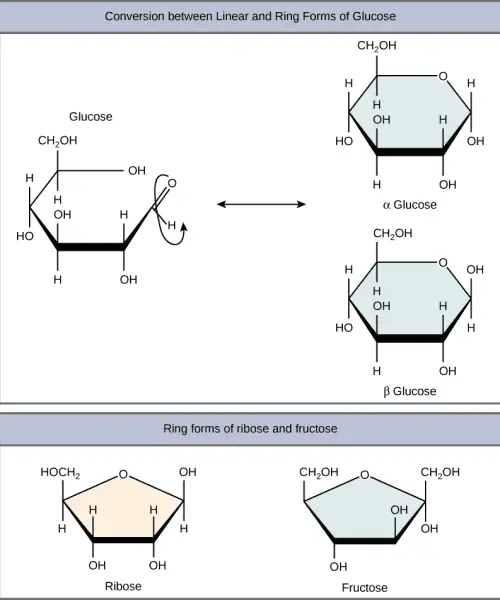
B. Disaccharides
Disaccharides are carbohydrates composed of two monosaccharide units linked together by a glycosidic bond. They are formed through a dehydration reaction, also known as condensation reaction, which involves the release of water as the hydroxyl group of one monosaccharide combines with the hydrogen of another. This process is fundamental in the synthesis of larger carbohydrate structures and plays a significant role in energy metabolism.
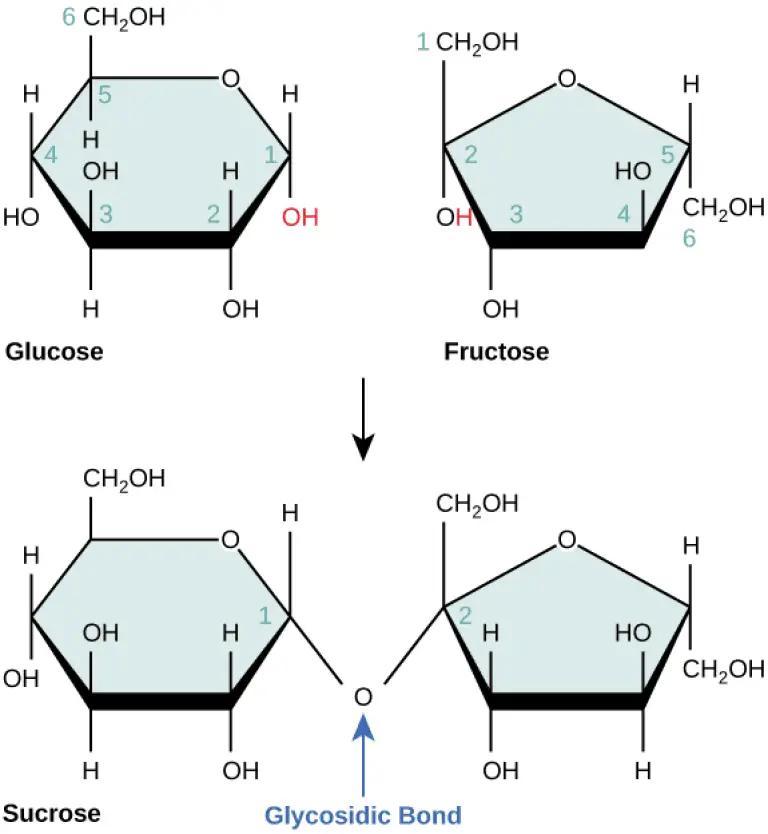
- Structure:
- The general chemical formula for disaccharides is C₁₂H₂₂O₁₁, indicating that they consist of two sugar units, each contributing to the overall structure.
- The bond formed between the two monosaccharides is termed a glycosidic bond, which can be classified as either alpha (α) or beta (β).
- An alpha bond is formed when the hydroxyl group on carbon-1 of the first monosaccharide is oriented below the ring plane.
- A beta bond occurs when this hydroxyl group is positioned above the ring plane.
- Examples of Disaccharides:
- Sucrose: Known as table sugar, sucrose is the most abundant disaccharide and is composed of one molecule of D-glucose and one molecule of D-fructose. Its systematic name is O-α-D-glucopyranosyl-(1→2)-D-fructofuranoside. Sucrose serves as a key product of photosynthesis and is a vital source of energy and carbon for plants.
- Lactose: This disaccharide, found naturally in mammalian milk, consists of one D-galactose and one D-glucose molecule. The systematic name for lactose is O-β-D-galactopyranosyl-(1→4)-D-glucopyranose. Lactose is essential for providing energy to young mammals.
- Maltose: Comprising two D-glucose molecules linked by an alpha-1,4 bond, maltose plays an important role as an intermediate in the digestion of starch and glycogen.
- Trehalose: Formed by two glucose molecules connected by an alpha-1,1 bond, trehalose serves as a crucial energy source for many organisms, particularly insects.
- Cellobiose: This disaccharide consists of two glucose units linked by a beta-1,4 bond and is significant in carbohydrate metabolism, particularly in the breakdown of cellulose.
- Gentiobiose: Comprising two glucose molecules linked by a beta-1,6 bond, gentiobiose is found in various plant glycosides and polysaccharides.
- Classification:
- Disaccharides can be categorized based on their reducing properties:
- Reducing Disaccharides: These contain a free hemiacetal unit that allows them to act as reducing agents. Examples include maltose and lactose.
- Non-Reducing Disaccharides: These lack a free hemiacetal and therefore cannot participate in oxidation reactions. Sucrose is a notable example of this category.
- Disaccharides can be categorized based on their reducing properties:
- Functions:
- Disaccharides serve as important energy sources in the diet. Upon hydrolysis, they release monosaccharides that enter metabolic pathways for energy production.
- Sucrose functions as a major source of carbon and energy in plants, while lactose provides energy in animals.
- Maltose is significant in the digestive processes of starch and glycogen, facilitating energy availability.
- Trehalose is vital for energy in insects, aiding in their survival and activity.
C. Polysaccharides
Polysaccharides are complex carbohydrates that consist of long chains of monosaccharide units linked together by glycosidic bonds. They serve essential functions in both structural integrity and energy storage across various organisms. As ubiquitous biomolecules, polysaccharides play a vital role in the biological systems of plants, animals, and microorganisms.
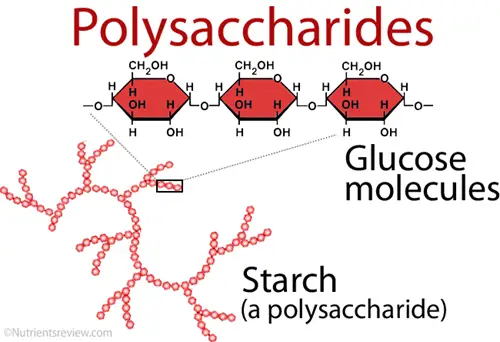
- Structure:
- Polysaccharides can be classified into two main categories: homopolysaccharides and heteropolysaccharides.
- Homopolysaccharides are composed of repeating units of a single type of monosaccharide. Common examples include:
- Cellulose: A linear polymer made of β-D-glucose units linked by β-1,4-glycosidic bonds, providing structural support in plant cell walls. It is the most abundant organic compound on Earth.
- Chitin: A polymer consisting of N-acetyl-D-glucosamine residues linked by β-1,4-glycosidic bonds. It serves a structural role in the exoskeletons of arthropods and the cell walls of fungi, making it the second most abundant biopolymer after cellulose.
- Starch: A storage polysaccharide in plants, composed of amylose (linear) and amylopectin (branched) forms of D-glucose linked by α-glycosidic bonds. It is the primary energy reserve for plants.
- Glycogen: The storage form of glucose in animals, structurally similar to amylopectin but more extensively branched. It is predominantly found in liver and muscle tissues, serving as a readily mobilized energy source.
- Heteropolysaccharides consist of two or more different types of monosaccharides. Examples include:
- Glycosaminoglycans (GAGs): Unbranched heteropolysaccharides that contain disaccharide repeating units. They include:
- Hyaluronic acid: Composed of D-glucuronic acid and N-acetylglucosamine, it plays a crucial role in maintaining the viscosity of synovial fluid and the structural integrity of connective tissues.
- Chondroitin sulfate: Involves D-glucuronic acid and N-acetylgalactosamine, serving as a major component of cartilage and aiding in resistance to compression.
- Heparin: Acts as a natural anticoagulant, preventing blood coagulation.
- Peptidoglycan: A polymer made up of alternating units of N-acetylglucosamine (NAG) and N-acetylmuramic acid (NAM), providing structural strength to bacterial cell walls and contributing to cell division.
- Agarose: Composed of repeating units of agarobiose, consisting of D-galactose and 3,6-anhydro-L-galactopyranose, it serves as a structural component in the cell walls of certain algae.
- Glycosaminoglycans (GAGs): Unbranched heteropolysaccharides that contain disaccharide repeating units. They include:
- Homopolysaccharides are composed of repeating units of a single type of monosaccharide. Common examples include:
- Polysaccharides can be classified into two main categories: homopolysaccharides and heteropolysaccharides.
- Classification:
- Polysaccharides can also be categorized based on their functional roles:
- Structural Polysaccharides: These provide mechanical stability to cells, tissues, and organisms. Examples include cellulose and chitin, which are critical for the structural integrity of plants and fungi, respectively.
- Storage Polysaccharides: These act as energy reserves, releasing monosaccharides when needed. Starch and glycogen are prominent storage polysaccharides, with starch being the primary energy source for plants and glycogen serving this purpose in animals.
- Polysaccharides can also be categorized based on their functional roles:
- Functions:
- Cellulose contributes to the rigidity of plant cell walls, supporting overall plant structure and growth. It is indigestible by humans but is an important component of dietary fiber, aiding in digestive health.
- Chitin provides structural support in various organisms, including insects and fungi, and is involved in the synthesis of cell walls in fungi.
- Starch serves as a major energy storage form in plants. Upon hydrolysis, it releases glucose, which can then enter metabolic pathways to fuel cellular processes.
- Glycogen is mobilized during periods of energy demand, such as fasting or vigorous activity, to maintain blood glucose levels and support metabolic functions.
- Hyaluronic acid is integral in maintaining hydration and lubrication in tissues, particularly in joints and the eyes.
- Peptidoglycan not only provides strength to bacterial cell walls but also plays a role in cell division, making it essential for bacterial reproduction.
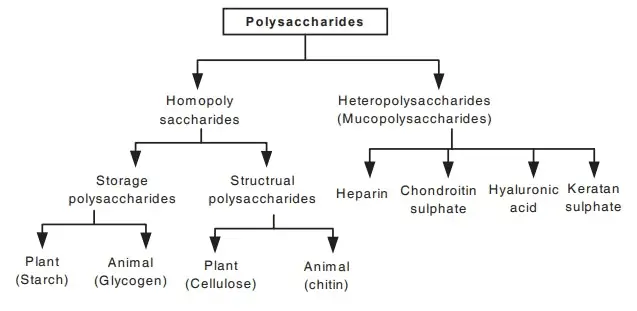
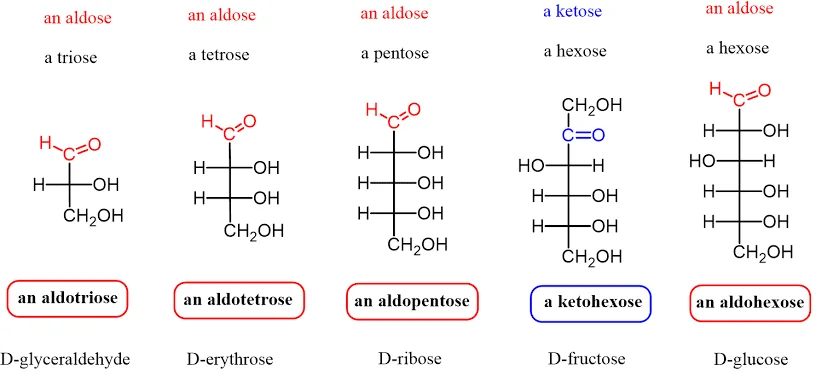
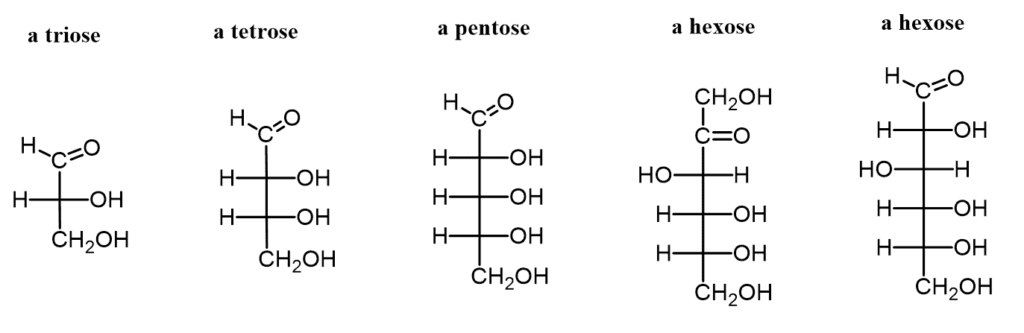
D. Oligosaccharides
Oligosaccharides are carbohydrate molecules that consist of 3 to 10 monosaccharide units linked together by glycosidic bonds. These compounds play significant roles in biological processes, serving as key components of glycoproteins and glycolipids, which are vital for various cellular functions.
- Structure:
- Oligosaccharides are classified based on the number of monosaccharide units they contain. Common classifications include:
- Trisaccharides: Composed of three monosaccharide units with the general formula C₃(H₂O)₁.
- Tetrasaccharides: Comprising four monosaccharide units with the formula C₄(H₂O)₂.
- Pentasaccharides: Formed from five monosaccharide units with the formula C₅(H₂O)₃.
- The monosaccharides in oligosaccharides are joined through glycosidic linkages, which are formed during a process known as glycosylation. This process involves the covalent attachment of a carbohydrate to an organic molecule, resulting in the formation of glycoproteins and glycolipids.
- Oligosaccharides are classified based on the number of monosaccharide units they contain. Common classifications include:
- Types of Glycosidic Linkages:
- N-Linked Oligosaccharides: These involve the attachment of oligosaccharides to the amino acid asparagine via a beta linkage to the nitrogen atom of the side chain. In eukaryotic organisms, N-linked glycosylation occurs at the membrane of the endoplasmic reticulum, whereas in prokaryotes, it takes place at the plasma membrane.
- O-Linked Oligosaccharides: These involve the attachment of oligosaccharides to the amino acids threonine or serine at the hydroxyl group of the side chain. O-linked glycosylation primarily occurs in the Golgi apparatus, where monosaccharide units are added to a fully formed polypeptide chain.
- Functions:
- Glycoproteins: Oligosaccharides attached to proteins form glycoproteins, which serve various essential functions, including:
- Antigenicity: They play a crucial role in immune recognition and response.
- Solubility: Glycoproteins influence the solubility of proteins in biological fluids.
- Resistance to proteases: They protect proteins from enzymatic degradation.
- Additionally, glycoproteins function as cell-surface receptors, cell-adhesion molecules, immunoglobulins, and tumor antigens, which are important in cell communication and immune responses.
- Glycolipids: These molecules consist of oligosaccharides attached to lipids. They are vital for:
- Cell recognition: Glycolipids facilitate the identification of cells by the immune system.
- Modulation of membrane proteins: They play a role in the function of membrane proteins that act as receptors, thereby influencing cellular signaling pathways.
- Lectins: Cells produce specific carbohydrate-binding proteins known as lectins, which interact with oligosaccharides on cell surfaces. Lectins mediate cell adhesion and play essential roles in various biological processes, including cell signaling and immune responses.
- Dietary Fiber: Oligosaccharides are components of plant tissues that contribute to dietary fiber. They are not fully digestible by humans, but they support digestive health by promoting beneficial gut bacteria and facilitating regular bowel movements.
- Glycoproteins: Oligosaccharides attached to proteins form glycoproteins, which serve various essential functions, including:
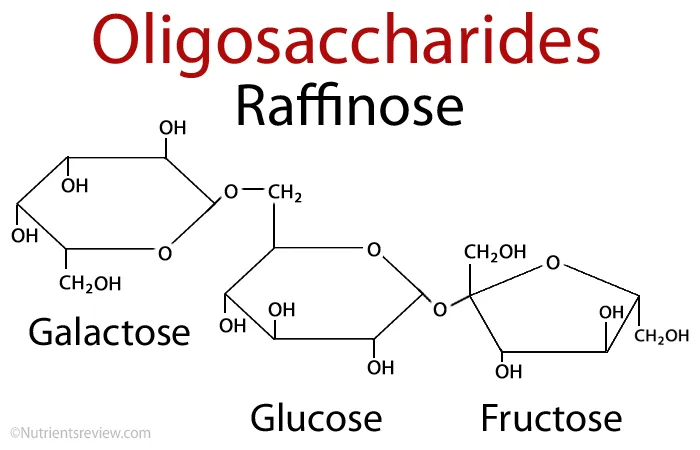
Functions of Carbohydrates
Carbohydrates serve critical roles in the biological systems of living organisms, contributing to energy production, structural integrity, cellular communication, and various metabolic processes. This multifaceted group of biomolecules is essential for overall health and functionality.
- Energy Source:
- Carbohydrates are recognized as the primary energy source for the body. Upon consumption, they are hydrolyzed into glucose, which cells utilize for metabolic processes.
- Particularly, glucose is vital for the brain and nervous system, which predominantly rely on carbohydrates to meet their energy demands.
- Energy Storage:
- Excess glucose from dietary intake is converted into glycogen, primarily stored in the liver and muscles.
- When the body requires immediate energy, such as during exercise or periods of fasting, glycogen can be broken down into glucose and released into the bloodstream, thus maintaining stable blood sugar levels.
- Structural Support:
- In the plant kingdom, carbohydrates like cellulose are integral components of cell walls, providing rigidity and structural support.
- In contrast, in the animal kingdom, chitin serves a similar role, forming the exoskeletons of arthropods, such as insects and crustaceans.
- Biosynthesis:
- Carbohydrates act as precursors in the biosynthesis of essential biomolecules.
- They play crucial roles in the production of lipids, proteins, and nucleic acids, thus facilitating cellular growth, repair, and overall maintenance.
- Cell Communication:
- Carbohydrates are often linked to proteins and lipids on the surface of cells, forming glycoproteins and glycolipids.
- These molecules function as surface antigens and receptors, enabling cell-to-cell communication and interactions with the extracellular environment.
- Immune Modulation:
- Certain dietary carbohydrates, particularly types of fiber, can influence the immune system.
- They support a healthy gut microbiota, enhance immune cell function, and contribute to the overall regulation of the immune response.
- Connective Tissue:
- Carbohydrates, especially glycosaminoglycans, are vital components of connective tissues.
- They provide structural integrity, elasticity, and hydration to tissues such as cartilage, tendons, and skin.
- Digestive Health:
- Carbohydrates rich in dietary fiber—found in whole grains, fruits, and vegetables—promote digestive health.
- They contribute to stool bulk, facilitate regular bowel movements, and support a healthy digestive system.
- Molecular Recognition:
- Carbohydrates are essential for molecular recognition processes.
- They play roles in the immune system’s ability to recognize pathogens, assist in cell adhesion and migration, and enhance the specificity of biological interactions.
- Fat Metabolism:
- Carbohydrates are involved in fat metabolism, preventing ketosis by ensuring that adequate glucose is available for energy production.
- Protein Sparing:
- By serving as the primary energy source, carbohydrates inhibit the breakdown of proteins for energy, preserving proteins for their crucial roles in growth, repair, and cellular function.
- Digestive Enzymes:
- The enzyme amylase is instrumental in the breakdown of starch into glucose, ultimately facilitating energy production necessary for metabolic activities.
Carbohydrates Examples
Carbohydrates are diverse biomolecules that play essential roles in biological processes. They can be classified into various categories based on their structure and complexity. The following examples illustrate the different types of carbohydrates, highlighting their unique characteristics and functions.
- Glucose:
- Glucose is a monosaccharide and a fundamental energy source for living organisms.
- It is a six-carbon compound with the molecular formula C₆H₁₂O₆ and exists in both open-chain and cyclic forms.
- Glucose is critical for cellular respiration and is a primary substrate for energy production.
- Galactose:
- Galactose is another monosaccharide, similar in structure to glucose but differs in the configuration of one hydroxyl group.
- It is often found as part of lactose in dairy products and plays a role in various metabolic pathways, including the synthesis of glycoproteins and glycolipids.
- Fructose:
- Fructose is a monosaccharide classified as a ketose due to the presence of a ketone group.
- Commonly found in fruits and honey, it serves as a sweetening agent and can be metabolized for energy.
- Fructose can also be converted to glucose in the liver, making it a vital component of energy metabolism.
- Maltose:
- Maltose is a disaccharide composed of two glucose units linked by an α-(1→4) glycosidic bond.
- It is produced during the digestion of starch and is found in malted foods and beverages.
- Maltose serves as an energy source that can be further hydrolyzed into glucose for metabolic processes.
- Sucrose:
- Sucrose, commonly known as table sugar, is a disaccharide formed from glucose and fructose.
- It is widely distributed in plants and is a significant source of energy in the human diet.
- Sucrose can be hydrolyzed into its constituent monosaccharides, providing readily available energy.
- Lactose:
- Lactose is a disaccharide consisting of glucose and galactose, primarily found in milk and dairy products.
- It is crucial for the nutrition of infants and can be broken down by the enzyme lactase into its monosaccharide components for absorption.
- Starch:
- Starch is a polysaccharide composed of numerous glucose units linked by glycosidic bonds.
- It is the primary storage carbohydrate in plants and is found in granules within chloroplasts, roots, tubers, and seeds.
- Starch can be hydrolyzed into glucose, providing energy when needed and serving as a significant component of the human diet.
- Cellulose:
- Cellulose is a polysaccharide consisting of β-(1→4) linked glucose units and is the main structural component of plant cell walls.
- It provides rigidity and support to plant cells, contributing to the overall structure of plants.
- Humans lack the enzymes necessary to digest cellulose, but it plays an important role as dietary fiber, promoting digestive health.
- Chitin:
- Chitin is a structural polysaccharide composed of N-acetylglucosamine units linked by β-(1→4) bonds.
- It is found in the exoskeletons of arthropods and the cell walls of fungi.
- Chitin provides strength and protection to these organisms, similar to the role cellulose plays in plants.
Common biological reactions involving carbohydrate
Carbohydrates are integral to various biological processes, engaging in a multitude of reactions essential for metabolism, energy production, and cellular function. Understanding these common reactions enhances comprehension of how carbohydrates interact with other biomolecules and their importance in living organisms.
- Photosynthesis:
- Photosynthesis is a biochemical process in which plants and other photosynthetic organisms synthesize simple sugars, primarily glucose, using carbon dioxide, water, and light energy.
- This process occurs in chloroplasts, where light-absorbing pigments, such as chlorophyll, capture sunlight, converting it into chemical energy.
- The overall reaction produces glucose, oxygen, and water, providing an energy source for the plant and oxygen for other organisms.
- Dehydration Synthesis:
- Dehydration synthesis, also known as condensation reaction, occurs when monosaccharides join together to form larger carbohydrates through glycosidic bonds.
- During this process, a molecule of water is released as a by-product, exemplified in the formation of disaccharides like sucrose from glucose and fructose.
- Further dehydration synthesis results in polysaccharides, such as starch and glycogen, which serve as energy storage molecules.
- Saccharification:
- Saccharification is the process of breaking down complex carbohydrates into simpler sugars, primarily glucose.
- This degradation typically involves hydrolysis, where water is used to cleave glycosidic bonds, facilitated by enzymatic action.
- In humans, saccharification begins in the mouth with salivary amylase, continuing in the small intestine where disaccharides are further broken down by enzymes such as maltase, lactase, and sucrase.
- Assimilation:
- Following digestion, monosaccharides are absorbed by the epithelial cells of the small intestine through the sodium ion-glucose symport system, involving glucose transporters (GluTs).
- After transport into the cells, monosaccharides like glucose are phosphorylated, trapping them within the cell as glucose-6-phosphate.
- This phosphorylated form can enter several metabolic pathways, including glycolysis for energy production, glycogenesis for storage as glycogen, and the Pentose phosphate pathway for synthesizing NADPH and nucleotides.
- Cellular Respiration:
- Cellular respiration is a series of metabolic processes that convert glucose into ATP, the primary energy currency of the cell.
- It comprises three main stages: glycolysis, the Krebs cycle, and oxidative phosphorylation.
- Glycolysis, occurring in the cytosol, converts glucose into pyruvate while producing a small yield of ATP and NADH.
- In the presence of oxygen, pyruvate enters the mitochondria, where it is fully oxidized through the Krebs cycle, generating additional electron carriers (NADH and FADH₂).
- The electron transport chain utilizes these carriers to produce a significant amount of ATP via chemiosmosis.
- Gluconeogenesis:
- Gluconeogenesis is essentially the reverse of glycolysis, wherein pyruvate and other non-carbohydrate precursors are converted into glucose.
- This process mainly occurs in the liver and is vital during fasting or intense exercise, ensuring a continuous supply of glucose for energy.
- Key steps begin in the mitochondria and conclude in the endoplasmic reticulum, with glucose-6-phosphate being hydrolyzed to form free glucose.
- Glycogenesis:
- Glycogenesis is the process of synthesizing glycogen from glucose, primarily occurring in the liver and muscle cells.
- It is stimulated when blood glucose levels are high, converting excess glucose into glycogen for storage.
- When energy is needed, glycogen can be broken down into glucose through glycogenolysis.
- Glycogenolysis:
- Glycogenolysis is the catabolic process of breaking down stored glycogen into glucose-1-phosphate, which is then converted into glucose-6-phosphate, entering glycolysis for energy metabolism.
- This process is crucial for maintaining blood glucose levels during periods of fasting or intense activity.
- Pentose Phosphate Pathway:
- The pentose phosphate pathway is a metabolic route that generates NADPH and five-carbon sugars (pentoses) from glucose, serving as an alternative to glycolysis.
- It occurs mainly in the liver, adipose tissue, and other metabolically active organs, playing a critical role in biosynthetic reactions and the immune response.
- Leloir Pathway (Galactose Metabolism):
- In the Leloir pathway, galactose, derived from lactose, is phosphorylated and subsequently converted into glucose-6-phosphate, allowing it to enter glycolysis.
- This pathway highlights the metabolic flexibility of carbohydrates in energy production.
- Fructose 1-Phosphate Pathway:
- This pathway enables fructose to enter glycolysis by being converted into intermediates that can eventually form glucose.
- It primarily occurs in tissues such as muscle and adipose tissue, illustrating the diverse sources of carbohydrates that can be metabolized for energy.
- Glucoregulation:
- Glucoregulation is the process of maintaining stable blood glucose levels, regulated by hormones such as insulin and glucagon.
- Insulin facilitates the uptake of glucose into cells, promoting glycogenesis, while glucagon stimulates glycogenolysis to increase blood glucose levels when necessary.
- Proper glucoregulation is essential for metabolic homeostasis, and disruptions can lead to disorders like diabetes mellitus or lactose intolerance.
Carbohydrates in Health and Disease
Carbohydrates are fundamental biomolecules involved in numerous physiological processes within the human body. Given their crucial roles, deviations in carbohydrate metabolism can lead to various health issues, significantly impacting overall well-being. Understanding the relationship between carbohydrates and disease mechanisms is vital for developing effective prevention and treatment strategies.
- Carbohydrate Metabolism and Disease:
- Aberrations in carbohydrate metabolism can provoke disease, highlighting the significance of maintaining balanced carbohydrate structures and concentrations.
- Dysregulation can lead to pathological conditions, such as diabetes mellitus, characterized by hyperglycemia resulting from impaired insulin secretion or action.
- Chronic hyperglycemia, in turn, induces tissue damage through mechanisms like protein glycation and the production of reactive oxygen species, contributing to complications such as retinopathy, nephropathy, neuropathy, and cardiovascular diseases.
- Diabetes Management:
- Effective management of diabetes necessitates strict control of carbohydrate intake and metabolism.
- Strategies include insulin therapy, oral hypoglycemic agents, dietary adjustments, and regular physical activity.
- These approaches aim to prevent long-term complications associated with unregulated blood glucose levels.
- Carbohydrate Structures in Disease:
- Beyond diabetes, specific alterations in carbohydrate structures have been linked to various conditions, including congenital disorders, cancer, and autoimmune diseases.
- Congenital disorders of glycosylation arise from defects in N-glycan synthesis pathways, leading to severe developmental impairments.
- In cancer, the density of cell surface glycan branching is often increased in tumorigenic cells, influencing metastasis and the progression of malignancies.
- Autoimmunity and Carbohydrate Interactions:
- In autoimmune diseases, such as Guillain-Barre syndrome, antibodies against glycoprotein glycans and glycolipids attack self-tissues, demonstrating the role of carbohydrates in immune dysregulation.
- The interplay between carbohydrates and the immune system underscores the importance of glycan structures in maintaining immune tolerance.
- Pathogen Interactions:
- Carbohydrates also facilitate interactions between pathogens and host cells.
- Viral and bacterial lectins bind to cell surface glycans, enabling infection and underscoring the dual role of carbohydrates in health and disease.
- This aspect emphasizes the potential of carbohydrates as targets for therapeutic interventions against infectious diseases.
- Future Directions in Research:
- Ongoing research aims to delineate the role of carbohydrates in various disease processes, potentially uncovering novel glycan biomarkers for early diagnosis and treatment.
- Identifying specific carbohydrate-related pathways may lead to the development of targeted therapies, enhancing disease management and prevention strategies.
Carbohydrate Digestion and Absorption
The digestion and absorption of carbohydrates is a complex process that begins in the mouth and continues through the small intestine, where polysaccharides are broken down into their monosaccharide components for utilization by the body. Understanding this process is critical for appreciating how carbohydrates function as a primary energy source for living organisms.
- Initial Digestion in the Mouth:
- Digestion begins in the oral cavity, where salivary amylase, an enzyme secreted by the salivary glands, hydrolyzes starches into smaller carbohydrate molecules, primarily maltose and dextrins.
- This enzymatic action initiates the breakdown of complex carbohydrates before they enter the stomach.
- Continued Digestion in the Small Intestine:
- The majority of carbohydrate digestion occurs in the small intestine, facilitated by pancreatic enzymes.
- Pancreatic α-amylase continues the digestion of starch and glycogen, breaking them down into maltose and dextrin.
- These smaller carbohydrates are further processed into oligosaccharides and disaccharides, preparing them for absorption.
- Role of Enterocytes:
- Enterocytes, the intestinal cells lining the small intestine, play a crucial role in completing carbohydrate digestion.
- These cells express various membrane-bound disaccharidases and cytosolic glycosidases that hydrolyze disaccharides and oligosaccharides into monosaccharides.
- Specific enzymes include:
- Maltase: hydrolyzes maltose into glucose.
- Sucrase: breaks down sucrose into glucose and fructose.
- Lactase: cleaves lactose into glucose and galactose.
- Absorption of Monosaccharides:
- The monosaccharides produced—glucose, galactose, and fructose—are absorbed across the intestinal epithelium into the bloodstream.
- This absorption occurs primarily through facilitated transport mechanisms, ensuring that these simple sugars enter circulation efficiently for distribution to tissues.
- Influence of Molecular Structure:
- The efficiency of carbohydrate digestion and absorption is significantly influenced by the molecular structure of the carbohydrates.
- For example, amylopectin, a branched form of starch, is more readily digested than amylose due to its structural characteristics.
- Conversely, fiber polysaccharides such as cellulose resist hydrolysis, preventing their breakdown and absorption.
- This resistance to digestion has important implications for dietary health, as fiber contributes to digestive health without being absorbed.
- Enzymatic Specificity:
- Each disaccharide requires a specific enzyme for digestion, underscoring the importance of enzyme availability.
- For instance, without sufficient levels of lactase, lactose cannot be effectively broken down, leading to lactose intolerance and associated symptoms.
- Summary of Digestive Enzymes:
- A variety of digestive enzymes are involved in carbohydrate breakdown, as summarized below:
Enzyme | Production Site | Substrate | Products |
---|---|---|---|
Salivary amylase | Salivary glands | Starch (amylose, amylopectin) | Maltose, dextrin |
Pancreatic α-amylase | Pancreas | Starch, glycogen | Maltose, dextrin |
Oligo-1,6-glucosidase | Small intestine | Oligosaccharides | Glucose |
Sucrase | Small intestine | Sucrose | Glucose, fructose |
Maltase | Small intestine | Maltose | Glucose |
Lactase | Small intestine | Lactose | Glucose, galactose |
Carbohydrate Energy Metabolism
Carbohydrate energy metabolism encompasses the biochemical processes through which carbohydrates are converted into usable energy within cells. This process is critical for sustaining cellular functions and overall metabolic health. Understanding the pathways involved in carbohydrate metabolism provides insights into energy production and regulation within various physiological contexts.
- Initial Steps: Glycolysis:
- Following digestion and absorption, monosaccharides such as glucose and fructose enter the glycolytic pathway, a crucial cytoplasmic process that converts six-carbon sugars into two three-carbon molecules known as pyruvate.
- Glycolysis produces a net gain of two ATP molecules and two NADH coenzymes, which are essential for cellular energy production.
- Role of Glucose:
- Glucose is the primary substrate for glycolysis in most cells. The phosphorylation of glucose, facilitated by the enzyme hexokinase, traps glucose within the cell by converting it to glucose-6-phosphate.
- This conversion commits glucose to subsequent catabolic processes. The glycolytic pathway generates several important intermediates, including glyceraldehyde 3-phosphate, dihydroxyacetone phosphate, 2,3-bisphosphoglycerate, and phosphoenolpyruvate.
- Multiple isoforms of glycolytic enzymes exist, differing in kinetic properties, allosteric regulation, and distribution across tissues, reflecting the specific metabolic requirements of different cell types.
- Aerobic and Anaerobic Conditions:
- In aerobic organisms, glucose is completely oxidized to maximize energy yield through further pathways such as the tricarboxylic acid (TCA) cycle and oxidative phosphorylation.
- Under anaerobic conditions, glycolysis can still provide energy via substrate-level phosphorylation, producing ATP without the need for oxygen. This makes glycolysis a fundamental metabolic pathway conserved across various species.
- Tissue-Specific Regulation:
- The regulation of glycolysis varies by tissue to meet specific energetic and biosynthetic demands. For instance, the liver uptakes and metabolizes glucose based on the body’s needs, while the brain consistently engages in glycolysis due to its high glucose requirement for energy.
- Metabolism of Other Monosaccharides:
- Besides glucose, other monosaccharides such as galactose and fructose also contribute to energy metabolism after undergoing specific processing steps.
- In the liver, galactose is converted to glucose-6-phosphate through the Leloir pathway, enabling its metabolism similarly to glucose.
- Fructose enters a distinct metabolic pathway initiated by phosphorylation via fructokinase, followed by cleavage by aldolase B, ultimately yielding glycolytic intermediates. However, fructose metabolism bypasses certain regulatory steps, leading to an unregulated production of substrates that can promote lipogenesis.
- Physiological Implications:
- The differences in metabolic pathways for glucose and fructose illustrate how carbohydrate structure influences metabolic function. The ability to regulate glycolysis based on tissue-specific needs ensures that energy production aligns with physiological demands.
- Understanding the dynamics of carbohydrate metabolism, including the impact of excessive fructose consumption, can provide insights into metabolic health and associated disorders.
What vegetables are high carbohydrates?
Some vegetables that are relatively high in carbohydrates include:
- Potatoes: One medium potato contains approximately 15-20 grams of carbohydrates.
- Sweet potatoes: One medium sweet potato contains approximately 24 grams of carbohydrates.
- Corn: One ear of corn contains approximately 15 grams of carbohydrates.
- Squash: One cup of cooked squash contains approximately 8-10 grams of carbohydrates.
- Peas: One cup of cooked peas contains approximately 20 grams of carbohydrates.
- Parsnips: One medium parsnip contains approximately 15 grams of carbohydrates.
It’s important to note that these vegetables also contain fiber, vitamins, minerals, and other nutrients that are beneficial to overall health. Vegetables should be included as part of a balanced diet along with other food groups, including fruits, grains, proteins, and fats.
Which enzymes break down carbohydrates?
Carbohydrates are broken down into simpler sugars by a group of enzymes known as carbohydrases. Some of the key enzymes involved in the breakdown of carbohydrates include:
- Amylases: These enzymes are produced by the salivary glands and pancreas. They break down complex carbohydrates such as starch into simpler sugars such as maltose and glucose.
- Lactases: These enzymes are produced by cells in the small intestine and break down lactose, a sugar found in milk and dairy products, into glucose and galactose.
- Sucrases: These enzymes are produced by cells in the small intestine and break down sucrose, a sugar commonly found in table sugar and many fruits, into glucose and fructose.
- Maltases: These enzymes are produced by cells in the small intestine and break down maltose, a sugar formed from the breakdown of starch, into glucose.
These enzymes work together to break down carbohydrates into simple sugars, which can then be absorbed into the bloodstream and used as energy by cells throughout the body.
Flashcard
What are carbohydrates?
Organic compounds made of carbon, hydrogen, and oxygen, primarily used as a source of energy.
Practice Quiz
FAQ
What are the monomers of carbohydrates?
The monomers of carbohydrates are simple sugars, also known as monosaccharides. Examples of monosaccharides include glucose, fructose, and galactose. These monosaccharides can combine to form larger carbohydrate molecules, such as disaccharides (such as sucrose, maltose) and polysaccharides (such as starch, cellulose).
What are the Polymer of carbohydrates?
The polymers of carbohydrates are long chains of monosaccharides linked together through glycosidic bonds. Some examples of carbohydrate polymers include:
Starch: A storage carbohydrate found in plants, made up of glucose units.
Cellulose: A structural carbohydrate found in plant cell walls, also made up of glucose units.
Glycogen: A storage carbohydrate found in animals, made up of glucose units.
Chitin: A structural carbohydrate found in the exoskeletons of arthropods and the cell walls of fungi, made up of N-acetylglucosamine units.
These carbohydrate polymers serve important roles in energy storage and as structural components in organisms.
What are the building blocks of carbohydrates?
The building blocks of carbohydrates are simple sugars, also known as monosaccharides. Monosaccharides are the basic units that make up larger carbohydrate molecules. The most common monosaccharides include glucose, fructose, and galactose. These monosaccharides can combine to form disaccharides such as sucrose and lactose, and polysaccharides such as starch and cellulose. The combination and arrangement of monosaccharides determine the type and function of the larger carbohydrate molecule.
What are carbohydrates?
Carbohydrates are a class of organic compounds that include sugars, starches, and fibers. They are one of the three main macronutrients, along with proteins and fats, that provide energy to the body. Carbohydrates can be classified as simple or complex, depending on their molecular structure. Simple carbohydrates are made up of one or two sugar units and are found in foods such as fruits, candy, and sugar. Complex carbohydrates are made up of three or more sugar units linked together and are found in foods such as bread, pasta, and potatoes. The body uses carbohydrates as its primary source of energy, and they play important roles in metabolism and cellular function.
What are complex carbohydrates?
Complex carbohydrates are a type of carbohydrate that are made up of long chains of simple sugars, known as monosaccharides. These chains can contain anywhere from three to thousands of monosaccharides, depending on the specific complex carbohydrate.
Complex carbohydrates are found in a variety of foods, including:
Grains and cereals
Starchy vegetables such as potatoes, corn, and peas
Legumes such as beans and lentils
Some fruits such as bananas and pears
Compared to simple carbohydrates, complex carbohydrates are digested more slowly and provide a slower release of energy. They are considered a healthier source of carbohydrates because they contain fiber and other nutrients, such as vitamins and minerals. Complex carbohydrates also help regulate blood sugar levels and promote feelings of fullness.
What do carbohydrates do?
Carbohydrates play a crucial role in the body and perform several important functions, including:
Energy production: Carbohydrates are an important source of energy for the body, particularly for the brain and central nervous system. The body can quickly convert carbohydrates into glucose, which is used as fuel for cells.
Metabolism regulation: Carbohydrates help regulate the body’s metabolism by providing energy for cellular processes and supporting the function of the endocrine system.
Structural support: Certain complex carbohydrates, such as cellulose and chitin, provide structural support in plant cell walls and in the exoskeletons of arthropods.
Digestion and absorption: Carbohydrates also play a role in the digestive process. Simple carbohydrates, such as fructose and glucose, are easily absorbed in the small intestine, while complex carbohydrates, such as fiber, are partially broken down in the large intestine and provide food for gut bacteria.
Satiety: Complex carbohydrates help regulate appetite and promote feelings of fullness. They slow down digestion and help regulate the release of insulin and glucose into the bloodstream, preventing spikes in blood sugar levels.
In summary, carbohydrates play a crucial role in energy production, metabolism regulation, structural support, digestion and absorption, and satiety. It is important to consume a balanced diet that includes a moderate amount of carbohydrates to support overall health and wellness.
How many carbohydrates in a glass of chardonnay?
The amount of carbohydrates in a glass of Chardonnay wine can vary, depending on the specific brand and alcohol content. On average, a 5-ounce glass of Chardonnay wine contains approximately 3-4 grams of carbohydrates.
Keep in mind that wine, like all alcoholic beverages, contains calories and should be consumed in moderation as part of a balanced diet. Overconsumption of alcohol can lead to a variety of health problems, including weight gain, liver disease, and an increased risk of certain cancers.
- Nelson, D. L., Cox, M. M. Lehninger Principles of Biochemistry. W.H. Freeman and Company, 2017.
Berg, J. M., Tymoczko, J. L., Gatto, G. J. Stryer, L. Biochemistry. W.H. Freeman and Company, 2018. - Campbell, M. K., Farrell, S. O. Biochemistry. Cengage Learning, 2017.
- Voet, D., Voet, J. G., Pratt, C. W. Fundamentals of Biochemistry: Life at the Molecular Level. Wiley, 2016.
- Shailja, & Singh, Parul & Researchgate, Ijmrr Analytics. (2024). Carbohydrate Structure and Role. 3. 52-72.
- Lennarz, W. J., Lane, M. D. Encyclopedia of Biological Chemistry. Elsevier Science, 2013.
- https://conductscience.com/structure-classification-and-functions-of-carbohydrates/
- https://openoregon.pressbooks.pub/biochemistry/chapter/2-7-structure-and-function-carbohydrates-biology-libretexts/
- https://www.biologyonline.com/dictionary/carbohydrate
- https://courses.lumenlearning.com/wm-biology1/chapter/reading-types-of-carbohydrates/
- https://www.lndcollege.co.in/syllabus/co_po/Carbohydrates%20-Classification,%20Structure%20and%20function.pdf
- https://www.khanacademy.org/science/ap-biology/chemistry-of-life/properties-structure-and-function-of-biological-macromolecules/a/carbohydrates