What is Calvin Cycle?
The Calvin Cycle, also known as the C3 cycle or Calvin-Benson cycle, is a critical biochemical process in photosynthesis that enables plants to convert carbon dioxide into organic compounds, primarily glucose. Named after Melvin Calvin, who discovered the pathway in the 1950s, this cycle occurs in the stroma of chloroplasts in plants, algae, and some bacteria, serving as the foundation for carbon fixation and biomass creation in photosynthetic organisms.
Though often referred to as the “dark reaction” or “light-independent reaction,” the Calvin Cycle operates predominantly when sunlight is available. This is because it relies on energy carriers—ATP and NADPH—produced in the light-dependent reactions of photosynthesis. While the Calvin Cycle itself does not use light directly, it depends on these energy-rich molecules generated by light reactions to drive its chemical processes.
The Calvin Cycle follows a three-phase process:
- Carboxylation: The cycle begins with carbon fixation, where the enzyme ribulose-1,5-bisphosphate carboxylase/oxygenase (RuBisCO) catalyzes the attachment of CO₂ to a five-carbon molecule, ribulose-1,5-bisphosphate (RuBP). This reaction creates a six-carbon intermediate that quickly splits into two molecules of 3-phosphoglycerate (3-PGA).
- Reduction: In this phase, ATP and NADPH from the light-dependent reactions are used to reduce 3-PGA into glyceraldehyde-3-phosphate (G3P). This three-carbon sugar can be further transformed into glucose and other carbohydrates that store energy and serve as structural materials for the plant.
- Regeneration of RuBP: A portion of G3P is recycled to regenerate RuBP, allowing the cycle to continue. This step requires additional ATP and ensures that CO₂ fixation can proceed without interruption, maintaining a continuous supply of carbohydrate precursors.
The Calvin Cycle not only produces glucose for the plant but also generates compounds that can later be used by animals through the food chain, converting solar energy into a form that can be stored and used over time. Unlike the immediate-use energy of ATP, sugars formed by the Calvin Cycle provide plants with long-term energy reserves, typically stored as starch or sucrose. Moreover, these sugars are foundational for synthesizing more complex biomolecules, including amino acids and nucleotides.
Overall, the Calvin Cycle is essential to the biosphere’s carbon cycle, playing a central role in converting atmospheric CO₂ into organic matter. It provides an energy-rich carbon skeleton, sustaining the growth of autotrophs and indirectly supporting heterotrophic life forms that depend on plant-based nutrients.
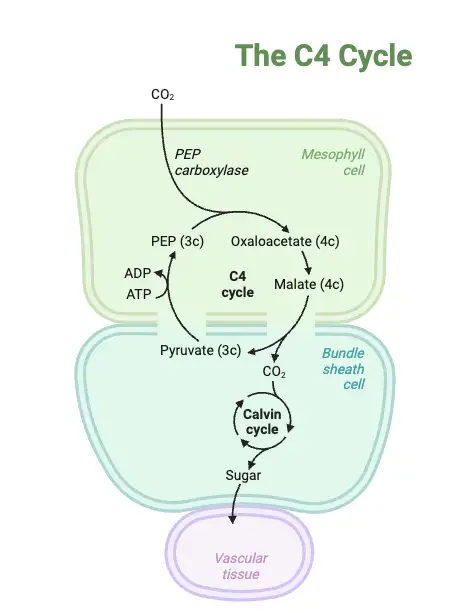
Definition of Calvin Cycle
The Calvin Cycle, also known as the C3 cycle, is a series of light-independent reactions in photosynthesis where plants convert carbon dioxide into glucose using energy from ATP and NADPH produced in the light-dependent reactions. This process occurs in the chloroplast stroma and is essential for carbon fixation and energy storage.
Steps of the Calvin Cycle
The Calvin Cycle, or C3 cycle, is a critical pathway in photosynthesis, consisting of three main steps: Carbon Fixation, Reduction, and Regeneration. Through these stages, plants convert atmospheric carbon dioxide into stable, energy-rich molecules that support cellular functions and growth.
1. Carbon Fixation
- In the initial stage, a molecule of carbon dioxide (CO₂) from the atmosphere binds with a five-carbon molecule called ribulose-1,5-bisphosphate (RuBP). This reaction, known as carbon fixation, is catalyzed by the enzyme RuBP carboxylase/oxygenase, commonly called RuBisCO.
- The combination of CO₂ and RuBP creates an unstable six-carbon compound that immediately breaks down into two molecules of 3-phosphoglyceric acid (3-PGA), each containing three carbons.
- RuBisCO, although slow, is essential for this process, and due to its abundance and role in carbon fixation, it is considered one of the most significant enzymes on Earth.
2. Reduction
- In the second stage, each 3-PGA molecule undergoes a reduction to form glyceraldehyde-3-phosphate (G3P), a three-carbon sugar. This reduction process utilizes energy derived from ATP and NADPH, produced during the light-dependent reactions of photosynthesis.
- Specifically, ATP donates energy, and NADPH provides electrons to convert 3-PGA into G3P. This transfer of electrons to 3-PGA is why the stage is termed “reduction.”
- By the end of this stage, six molecules of ATP and six molecules of NADPH have been consumed, and G3P is synthesized, storing solar energy in chemical bonds within the sugar molecules.
3. Regeneration of RuBP
- Some G3P molecules produced are set aside for the synthesis of glucose, while others proceed to the final stage, regeneration.
- In this complex, ATP-dependent series of reactions, G3P molecules are recycled to regenerate RuBP, the five-carbon molecule that will receive new CO₂ molecules and continue the cycle.
- The Calvin Cycle must repeat six times to produce one glucose molecule, as each turn of the cycle fixes one molecule of CO₂. Five out of six G3P molecules formed are recycled to regenerate RuBP, while the sixth G3P exits the cycle and ultimately contributes to glucose synthesis.
Calvin Cycle Diagram
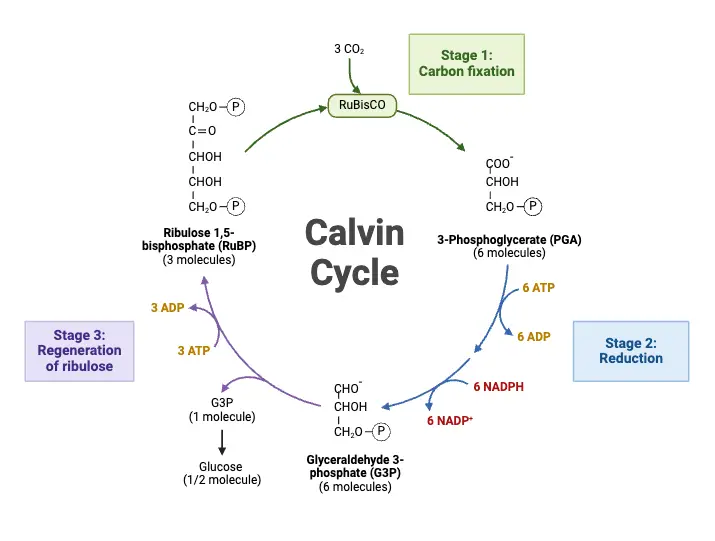
Products of the Calvin Cycle
The Calvin Cycle, central to photosynthesis, yields key products that serve as fundamental components for energy storage and cellular processes. The primary products are glyceraldehyde-3 phosphate (G3P) and glucose, with the process requiring a substantial input of ATP and NADPH.
- Carbon Fixation per Cycle: In each turn of the Calvin Cycle, one carbon atom is fixed, contributing incrementally to the formation of glucose. To synthesize one complete molecule of glucose, the cycle must go through six turns.
- Production of Glyceraldehyde-3 Phosphate (G3P): Every three cycles of the Calvin Cycle result in one molecule of G3P, a three-carbon sugar that serves as a precursor for glucose and other carbohydrates. G3P is a versatile molecule that plants can use in various biosynthetic pathways to produce essential compounds.
- Formation of Glucose: Two G3P molecules combine to form a single glucose molecule. Thus, six turns of the Calvin Cycle are required to produce the two G3P molecules necessary for glucose synthesis.
- Energy Requirements: The Calvin Cycle’s reduction and regeneration phases depend on energy-rich molecules produced in the light-dependent reactions of photosynthesis.
- Each cycle requires 3 ATP and 2 NADPH for the reduction of 3-phosphoglyceric acid (3-PGA) to G3P and for the regeneration of RuBP.
- For the synthesis of one glucose molecule, 18 ATP and 12 NADPH are consumed, underscoring the energy-intensive nature of the process.
Function of the Calvin Cycle
The Calvin Cycle is essential in photosynthesis, enabling the conversion of carbon dioxide from the atmosphere into three-carbon sugars. These sugars are fundamental for synthesizing other vital compounds like glucose, starch, and cellulose, forming the backbone of plant biomass. The cycle supports various biological functions:
- Building Plant Biomass: By fixing carbon directly from the atmosphere, the Calvin Cycle helps synthesize carbohydrates, which serve as both energy storage and structural components in plants. These compounds, particularly glucose, starch, and cellulose, are foundational for plant growth and development.
- Ecosystem Foundation: Since plants occupy the base of most ecosystems, the Calvin Cycle indirectly sustains food webs. It allows plants to store energy in a digestible form for herbivores, and by extension, it enables carnivores to access energy through herbivores. Thus, the Calvin Cycle supports the entire energy pyramid within ecosystems.
- Production of Biomolecules: The carbon structures generated in the Calvin Cycle are precursors for proteins, lipids, nucleic acids, and other biomolecules crucial to both plant and animal life. These molecules provide the raw materials for cellular structures and functions, contributing to life’s diversity.
- Regulating Atmospheric CO₂: The Calvin Cycle plays a significant role in balancing carbon dioxide levels in the atmosphere. By fixing CO₂, plants help mitigate greenhouse gas accumulation. However, human activities like deforestation and fossil fuel combustion reduce this natural carbon sink, raising environmental concerns.
- Marine Carbon Fixation: Photosynthetic organisms in marine ecosystems, such as phytoplankton, also use the Calvin Cycle to fix carbon from seawater. This contributes to maintaining CO₂ balance in oceans, which is a critical component of the global carbon cycle.
- Functionality in Extreme Environments: Certain organisms, including extremophiles, rely on the Calvin Cycle to thrive in harsh habitats, such as areas with limited water or high salinity. This cycle supports carbon assimilation, enabling life in diverse and challenging conditions.
- Distribution of G3P for Biosynthesis: The Calvin Cycle produces glyceraldehyde-3-phosphate (G3P), a three-carbon sugar that plants use for various biosynthetic pathways. Some G3P is transported out of chloroplasts into the cytoplasm, where it contributes to synthesizing lipids, amino acids, and other essential metabolites.
- Environmental Sensitivity and Adaptation: The activity of the Calvin Cycle is responsive to environmental factors like light, temperature, and water availability. Under stress, plants modulate this cycle to maintain carbon fixation efficiency, balance energy use, and limit oxidative damage.
- Oxygen Production Link: Although the Calvin Cycle itself does not generate oxygen, it depends on products of the light-dependent reactions, which release oxygen. This oxygen is critical for aerobic organisms and helps maintain Earth’s atmospheric balance.
- Isotopic Discrimination and Climate Studies: The Calvin Cycle exhibits a selective preference for carbon isotopes (e.g., carbon-12 over carbon-13), which scientists analyze to infer past environmental conditions. This isotopic discrimination is valuable for reconstructing historical climates from plant fossils and sedimentary data.
- Integration with Biogeochemical Cycles: The Calvin Cycle is intertwined with other cycles, such as nitrogen and phosphorus cycles, impacting nutrient dynamics and ecosystem productivity. Through these interactions, the cycle influences biodiversity and ecosystem functioning across different habitats.
Facts About the Calvin Cycle
The Calvin Cycle, also known as the C3 cycle or the dark reactions of photosynthesis, is a critical biochemical pathway through which plants convert carbon dioxide into organic compounds. Here are key facts about the Calvin Cycle that highlight its function, discovery, and importance.
- Discovery and Nobel Prize Recognition: Named after Melvin Calvin, who, along with colleagues Andrew Benson and James Bassham, mapped the carbon fixation pathway using radioactive carbon isotopes in the 1940s and 1950s. This groundbreaking work earned Calvin the Nobel Prize in Chemistry in 1961.
- Light-Independent Process: Often called the light-independent reactions, the Calvin Cycle does not directly use light. However, it relies on ATP and NADPH produced by the light-dependent reactions, making it indirectly dependent on sunlight.
- Carbon Fixation and Role of RuBisCO: The first step of the Calvin Cycle involves the fixation of carbon dioxide with a five-carbon molecule, ribulose bisphosphate (RuBP), catalyzed by the enzyme RuBisCO. This enzyme, though highly abundant, is relatively slow and often considered the rate-limiting factor of the cycle.
- Production of G3P and Regeneration of RuBP: During the cycle, ATP and NADPH are used to reduce 3-phosphoglycerate (3PGA) to glyceraldehyde-3 phosphate (G3P). Some G3P molecules continue to form glucose, while others regenerate RuBP, enabling the cycle to continue carbon fixation.
- Photorespiration: RuBisCO can mistakenly bind with oxygen instead of carbon dioxide, especially in hot, dry conditions. This process, called photorespiration, reduces the efficiency of carbon fixation and can impact plant growth. Some plants have evolved alternate photosynthetic pathways, like C4 and CAM, to mitigate photorespiration’s effects.
- Global Carbon Fixation: Under optimal conditions, the Calvin Cycle can fix billions of tons of carbon dioxide each year, a process essential for maintaining atmospheric balance and supporting life on Earth.
- Carbon Isotope Discrimination: The Calvin Cycle discriminates between carbon-12 and carbon-13 isotopes. This isotopic signature, preserved in plant tissues, provides valuable data for scientists studying climate history and ecological shifts.
- Evolutionary Impact: The evolution of the Calvin Cycle had a transformative effect on Earth’s atmosphere by decreasing carbon dioxide levels and increasing oxygen concentrations, thus playing a vital role in shaping the planet’s biosphere.
- Applications in Biotechnology: Researchers are exploring ways to enhance the Calvin Cycle’s efficiency through genetic engineering, aiming to improve crop productivity and adaptability in response to environmental stresses.
- Climate Change Mitigation: By sequestering carbon dioxide, the Calvin Cycle plays a role in combating climate change, converting CO₂ into stored plant biomass that can reduce atmospheric CO₂ levels.
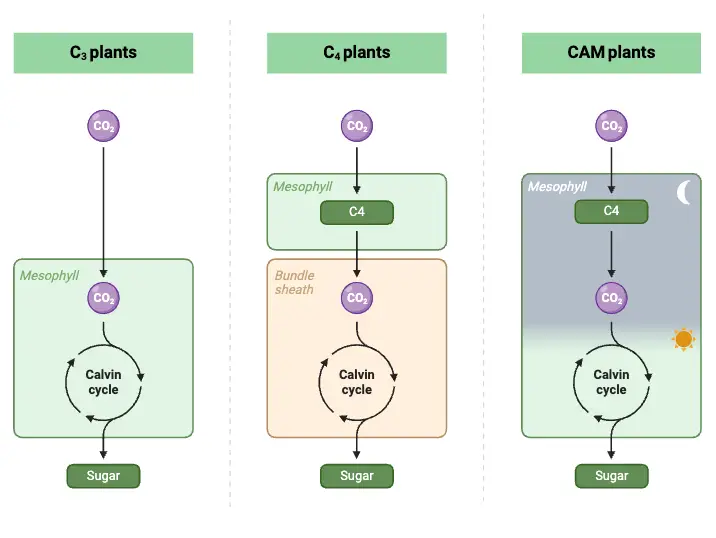
- https://byjus.com/biology/calvin-cycle/
- https://biologydictionary.net/calvin-cycle/
- https://www.geeksforgeeks.org/calvin-cycle-class-11/
- https://en.wikipedia.org/wiki/Calvin_cycle
- https://courses.lumenlearning.com/suny-biology1/chapter/the-calvin-cycle/
- https://flexbooks.ck12.org/cbook/ck-12-biology-flexbook-2.0/section/2.22/primary/lesson/calvin-cycle-bio/
- https://www.vedantu.com/biology/calvin-cycle
- https://www.biologyonline.com/dictionary/calvin-cycle