What are Biosensors?
- A biosensor is a specialized analytical device that transforms biological responses into electrical signals. These devices play a crucial role in detecting and measuring concentrations of various biological substances. Even when a biosensor doesn’t directly interact with a biological system, it is still capable of identifying parameters of biological relevance.
- Biosensors work by linking a biological sensing element, such as enzymes, antibodies, or nucleic acids, with a transducer. The transducer acts as a detector, converting the biological interaction into an electrical signal. The first practical biosensors were electrochemical sensors, designed to measure different analytes, which laid the foundation for the modern development of biosensing technologies.
- In essence, a biosensor consists of three key components. First is the sensor, a sensitive biological material such as tissues or microorganisms. The second part is the transducer, which detects the interaction between the analyte and the biological element, using methods like electrochemical or optical detection. Finally, the third component includes the associated electronics, responsible for processing the signal and displaying the results.
- The definition of a biosensor extends to devices that integrate biological recognition with a transduction system, providing either qualitative or semi-quantitative information. Simply put, biosensors detect changes in biological processes and translate these changes into electrical signals that can be analyzed for various applications, from medical diagnostics to environmental monitoring.
Definition of Biosensors
A biosensor is a device that detects biological changes and converts them into an electrical signal, typically by combining a biological sensing element with a transducer for applications like medical diagnostics or environmental monitoring.
Components of Biosensors
Biosensors consist of several key components that work together to detect biological processes and convert them into measurable signals. The components of a biosensor are outlined below:
- Analyte:
The analyte is the specific substance that the biosensor is designed to detect. For example, in a glucose biosensor, glucose is the analyte of interest. The analyte interacts with other components to initiate the detection process. - Bioreceptor:
A bioreceptor is a biological molecule that specifically recognizes the analyte. Common types of bioreceptors include enzymes, antibodies, cells, aptamers, and DNA. When the analyte binds to the bioreceptor, a bio-recognition event occurs, generating a detectable signal such as changes in light, heat, pH, charge, or mass. - Transducer:
The transducer converts the signal from the bioreceptor–analyte interaction into a measurable form of energy, such as an electrical or optical signal. This process is known as signalization. The intensity of the signal is generally proportional to the amount of analyte present, enabling quantification. - Electronics:
The electronic component processes the raw signal from the transducer. It amplifies, conditions, and often converts the signal from an analog form to digital. These processed signals are then prepared for interpretation by the display system. The electronics ensure that the signal is suitable for accurate analysis. - Display:
The display presents the results in a user-friendly format, such as a numerical value, graph, or table. This part of the biosensor often includes both hardware and software components, and it allows the user to easily interpret the detected signal.
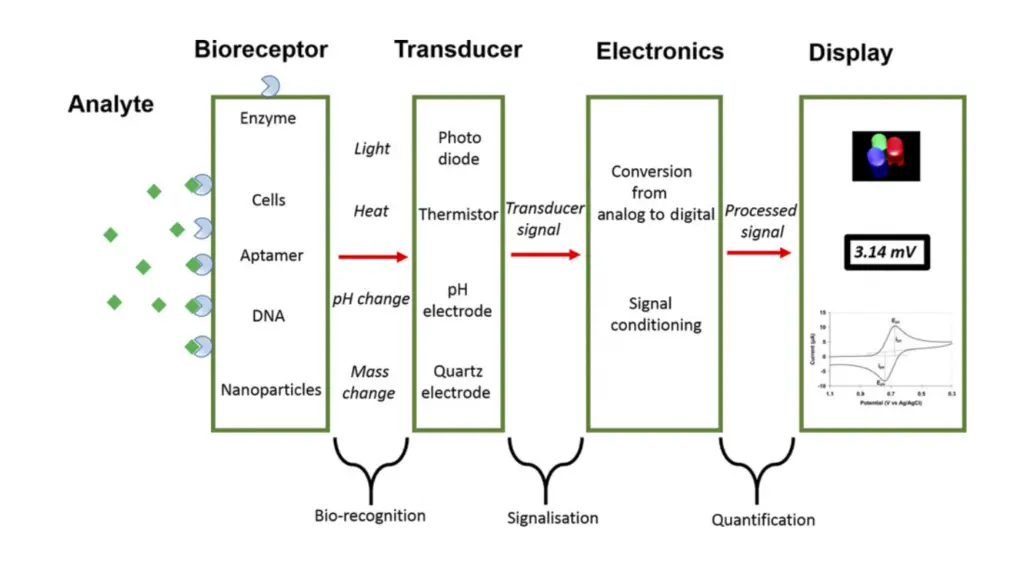
Historical background
The development of biosensors has a rich historical background, dating back to early scientific discoveries in electrochemistry and enzyme activity. Below are key milestones in the historical progression of biosensors:
- 1906 – M. Cremer’s Discovery:
The origins of biosensors trace back to 1906 when M. Cremer discovered that the concentration of an acid in a liquid is proportional to the electric potential across a glass membrane. This marked the early understanding of electrical responses in chemical systems. - 1909 – Introduction of pH Concept:
Søren Peder Lauritz Sørensen introduced the concept of pH in 1909, establishing the foundation for measuring hydrogen ion concentrations. By 1922, W.S. Hughes developed an electrode for pH measurement, which laid the groundwork for future electrochemical biosensors. - 1930s – Enzyme Immobilization:
Between 1909 and 1922, Griffin and Nelson pioneered the immobilization of enzymes, such as invertase, on substrates like aluminum hydroxide and charcoal. This was a significant step toward developing biorecognition elements in biosensors. - 1956 – The Clark Electrode:
The first true biosensor was developed by Leland C. Clark, Jr. in 1956 for oxygen detection. Known as the ‘Clark electrode,’ this device is regarded as a major breakthrough, earning Clark the title of the “father of biosensors.” - 1962 – Amperometric Enzyme Electrode:
Leland Clark further advanced biosensor technology by demonstrating an amperometric enzyme electrode for glucose detection in 1962, which became a cornerstone for glucose biosensors used in medical diagnostics. - 1969 – Potentiometric Biosensor:
In 1969, Guilbault and Montalvo developed the first potentiometric biosensor to detect urea, marking the expansion of biosensors into more varied chemical analytes. - 1970 – Ion-Sensitive Field-Effect Transistor (ISFET):
The invention of the ion-sensitive field-effect transistor (ISFET) by Bergveld in 1970 introduced semiconductor technology into biosensing, further enhancing sensor sensitivity and miniaturization. - 1975 – First Commercial Biosensor:
Yellow Spring Instruments (YSI) developed the first commercial biosensor for glucose detection in 1975, revolutionizing the commercial application of biosensors in the medical field. - 1970s – Fiber-Optic Biosensors and Microbe-Based Sensors:
Fiber-optic biosensors for gases like carbon dioxide and oxygen were introduced by Lubbers and Opitz in 1975. In the same year, Suzuki and colleagues developed the first microbe-based immunosensor, broadening the scope of biosensor applications. - 1980s – Surface Plasmon Resonance (SPR) and Mediated Amperometric Biosensors:
The 1980s saw the development of fiber-optic biosensors for glucose detection and the introduction of surface plasmon resonance (SPR) immunosensors by Liedberg et al. in 1983. By 1984, ferrocene was used with glucose oxidase to create the first mediated amperometric biosensor. - 1990s – Commercial Expansion and Handheld Devices:
In 1990, Pharmacia Biacore introduced an SPR-based biosensor for commercial use. In 1992, i-STAT launched the first handheld blood biosensor, making biosensing technology more accessible in clinical settings.
Since these foundational developments, biosensors have become an interdisciplinary field, combining principles from physics, chemistry, biology, micro- and nano-technology, and electronics. The field has expanded considerably, with over 84,000 scientific reports indexed from 2005 to 2015, reflecting its growing importance across various industries, especially in healthcare and environmental monitoring.
Characteristics of a biosensor
Below is an overview of the main characteristics of a biosensor:
- Selectivity:
Selectivity is a critical feature of a biosensor, referring to its ability to detect a specific analyte even in the presence of other substances or contaminants. This feature is vital in medical diagnostics, where samples like blood or urine may contain numerous molecules. The bioreceptor must interact only with the target analyte, minimizing false positives. For instance, antibody-antigen interactions showcase high selectivity, where the bioreceptor is specific to one molecule. - Sensitivity:
Sensitivity defines the biosensor’s capacity to detect small changes in analyte concentration and generate a measurable signal. This feature is often described by the limit of detection (LOD), which refers to the smallest quantity of analyte that can be identified. Sensitivity is critical in medical and environmental monitoring applications, where detecting trace amounts of substances is necessary, such as the detection of prostate-specific antigen (PSA) in blood. - Reproducibility:
Reproducibility is the ability of the biosensor to provide consistent results across repeated experiments under the same conditions. It ensures that the biosensor’s performance is reliable. This feature depends on the precision and accuracy of the transducer and electronic components. High reproducibility indicates that the biosensor can be trusted to deliver stable results in routine diagnostic or industrial use. - Stability:
Stability measures how well a biosensor resists changes in performance due to environmental disturbances such as temperature fluctuations or humidity. Long-term stability is crucial in applications requiring continuous monitoring, where drift in output signals could lead to errors. The stability is influenced by both the transducer and the bioreceptor. Bioreceptors with high affinity for the analyte tend to increase stability by forming strong bonds, reducing the likelihood of signal variation over time. - Linearity:
Linearity refers to how accurately the biosensor’s output signal correlates with different concentrations of the analyte. Ideally, the biosensor should produce a straight-line response across a range of analyte concentrations. This property provides insight into the biosensor’s resolution, which is the smallest detectable change in analyte concentration. A high degree of linearity is essential for applications that require not only the detection of an analyte but also the precise measurement of its concentration over a broad range. - Response Time:
Response time is the period required for the biosensor to generate a signal following the interaction between the analyte and the bioreceptor. Shorter response times are advantageous, especially in real-time monitoring applications, where rapid feedback is necessary. - Detection Limit:
The detection limit defines the lowest concentration of the target analyte that the biosensor can detect and produce a measurable response. In medical diagnostics, for example, the detection limit must be low enough to identify disease markers at early stages when their concentrations are minimal. - Resolution:
Resolution is the smallest change in analyte concentration that can be detected by the biosensor. A high-resolution biosensor can distinguish between small variations in concentration, which is particularly important in applications requiring precise measurements.
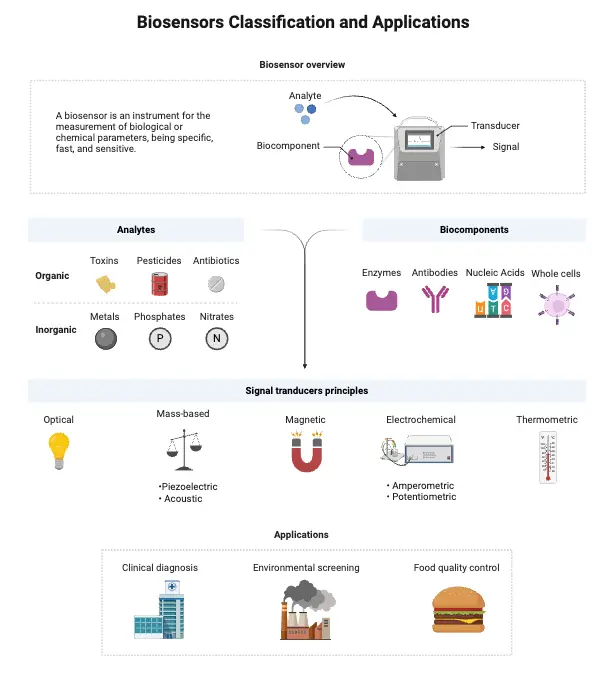
Principle of a Biosensor
- Biosensors operate fundamentally on the principles of biorecognition and signal transduction. They integrate biological recognition elements with transducers to convert biochemical interactions into measurable electrical signals. The core component of many biosensors is the immobilized biological material, often an enzyme, which is prepared through various techniques, including physical entrapment and covalent binding. This immobilization ensures that the biological component maintains its activity while remaining in close proximity to the transducer.
- Upon introduction of the target analyte, a specific interaction occurs between the analyte and the immobilized enzyme. This interaction results in the formation of a bound analyte complex, which triggers a biochemical change in the enzyme. Consequently, the nature of this change can lead to the release of products, which may include heat, gases, or ions. Such changes are crucial, as they enable the transducer to convert these biochemical responses into electrical signals.
- The transducer plays a pivotal role in this process. It detects the alterations in the biochemical properties of the enzyme and translates them into electrical signals. This electroenzymatic process represents a key mechanism by which biochemical reactions are transformed into quantifiable data. The output from the transducer is an electrical signal that corresponds directly to the concentration of the analyte in question, providing a clear indication of its presence and quantity.
- Furthermore, the electrical signals generated by the transducer are typically amplified to enhance their readability. This amplification is essential for accurate analysis and representation of the data. Ultimately, the transformed electrical signal can be displayed in a physical format, such as a digital readout, allowing for straightforward interpretation and assessment.
Working of a Biosensor
The operation of a biosensor involves several critical steps that transform biochemical interactions into quantifiable electrical signals. Below is a detailed explanation of this process:
- Biological Recognition and Transduction:
The core of a biosensor lies in the interaction between the biological sensing element, typically an enzyme, and the transducer. This interaction leads to the generation of a biological signal, which the transducer converts into an electrical response. - Signal Generation:
The transducer outputs either a current or voltage signal, depending on the type of enzyme used. If the output is current, it requires conversion to voltage using an operational amplifier (Op-Amp) based current-to-voltage converter to facilitate further processing. - Signal Characteristics:
The electrical signals generated are often of low amplitude and can be obscured by high-frequency noise. This noise may stem from electrical interference or inherent fluctuations in the electronic components of the transducer. - Signal Amplification:
To enhance the low-level signal, it undergoes amplification. An Op-Amp based amplifier is typically employed to increase the signal strength, allowing it to be more distinguishable from background noise. - Filtering:
Following amplification, the signal is subjected to a low-pass RC filter. This filtering process smooths out unwanted high-frequency noise, thereby improving the clarity of the resultant signal. - Signal Processing Unit:
The processed signal is then directed to a signal conditioning unit, which is responsible for further refining the signal. At this stage, the output is classified as an analog signal, directly representing the biological quantity being measured. - Conversion to Digital Signal:
While the analog signal can be displayed on an LCD for immediate analysis, it is typically converted to a digital signal for easier manipulation and storage. This conversion occurs in a microcontroller, which processes the analog input and formats it for display or data logging. - Output Display:
The final output can be presented in various formats, often visualized on a digital display. This output provides users with critical information regarding the concentration of the analyte, facilitating informed decision-making based on the biosensor’s measurements.
Types of Biosensors
Biosensors are analytical devices that integrate biological components with electronic systems to detect chemical substances. Their classification can be approached from two primary angles: the biological receptors they utilize and the transduction elements that convert biological responses into measurable signals. This framework enables a comprehensive understanding of the various biosensor types and their applications.
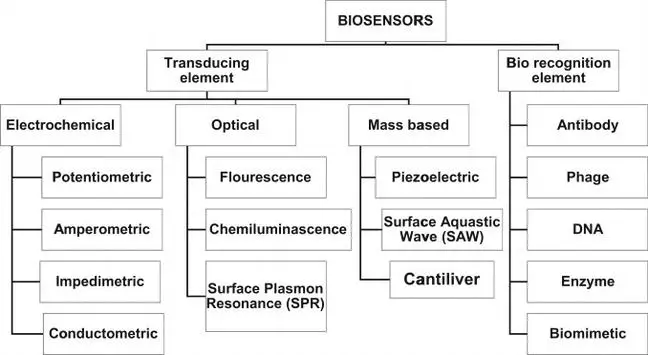
1. Classification Based on Biological Receptors
Biological receptors play a crucial role in the specificity and functionality of biosensors. The primary categories are enzyme-based and DNA-based biosensors.
1.1 Enzyme-Based Biosensors
- Overview: Enzyme-based biosensors are the most extensively studied and commercially utilized biosensors. They leverage enzymes to monitor biochemical reactions and detect specific metabolites in real time.
- Mechanism: Enzymatic reactions are facilitated through immobilization techniques such as van der Waals forces, ionic, or covalent bonding. The first notable enzyme biosensor, developed by Updike and Hicks in 1967, enabled glucose detection, marking a significant advancement in biosensor technology.
- Applications: Common examples include glucose and urea biosensors, with glucose biosensors being predominant due to their application in diabetes management.
- Advantages: These biosensors are characterized by their longevity and reusability since enzymes remain unchanged after reactions, thus extending their operational lifespan.
1.2 DNA-Based Biosensors
- Overview: DNA biosensors utilize nucleic acids as recognition elements to detect specific proteins or small compounds.
- Mechanism: They rely on the hybridization of DNA probes with target sequences, facilitated by stable hydrogen bonding. The efficiency of this hybridization is pivotal for the sensor’s sensitivity and selectivity.
- Applications: DNA biosensors are utilized in various fields, including medical diagnostics and environmental monitoring, to identify pathogens or genetic markers.
- Challenges: Achieving high selectivity while minimizing non-specific interactions is essential for effective DNA biosensing.
2. Classification Based on Transduction Elements
The classification of biosensors by transduction elements is widely recognized and includes electrochemical, mass-based, and optical biosensors.
2.1 Electrochemical Biosensors
- Overview: These biosensors measure electrical changes that occur due to interactions between the analyte and the sensor surface.
- Mechanism: The output signal is typically a change in current or voltage, which correlates to the concentration of the analyte.
- Subcategories:
- Conductometric Biosensors: Measure changes in conductivity resulting from biochemical reactions. While less common due to lower sensitivity, they can detect specific interactions.
- Potentiometric Biosensors: Monitor changes in voltage due to ion concentration variations. They are applied in clinical diagnostics for pathogen detection.
- Amperometric Biosensors: These are prevalent for detecting electroactive species through current measurements during redox reactions.
- Impedimetric Biosensors: Track impedance changes linked to antigen-antibody interactions, useful in clinical and industrial applications.
2.2 Mass-Based Biosensors
- Overview: Mass-based biosensors, particularly piezoelectric sensors, detect changes in mass due to the binding of analytes.
- Mechanism: The sensor’s oscillation frequency shifts as mass is added, leading to a measurable electrical signal.
- Example: The Quartz Crystal Microbalance (QCM) is a notable piezoelectric biosensor extensively used in various applications.
2.3 Optical Biosensors
- Overview: Optical biosensors utilize light to detect changes in biological interactions, often employing techniques such as surface plasmon resonance (SPR).
- Mechanism: These sensors detect shifts in refractive index or light absorption as biomolecular interactions occur at the sensor surface.
- Advantages: They enable real-time, label-free detection of biomolecules, making them valuable for applications in healthcare, environmental monitoring, and food safety.
- Challenges: Traditional optical biosensors require precise alignment and sophisticated optics, which can complicate their deployment.
Applications of biosensors
Biosensors have a wide range of applications across various fields. Here are some key areas:
- Medical Diagnostics: Biosensors are used for the detection of diseases through blood glucose monitoring, cholesterol levels, and other biomarkers.
- Environmental Monitoring: They can detect pollutants in water and soil, helping to monitor environmental health.
- Food Safety: Biosensors are used to detect pathogens, toxins, and allergens in food products to ensure safety and quality.
- Pharmaceuticals: They aid in drug development by monitoring biological interactions and assessing the efficacy of new compounds.
- Bioprocessing: In industrial biotechnology, biosensors monitor fermentation processes and optimize conditions for microbial production.
- Wearable Technology: Biosensors are integrated into wearable devices for real-time health monitoring, such as heart rate, hydration levels, and fitness tracking.
- Agriculture: They help monitor soil health, crop conditions, and plant diseases, allowing for precision agriculture practices.
- Biodefense: Biosensors are used to detect biological threats and pathogens in security and defense applications.
FAQ
Biosensors are sophisticated analytical devices that combine biological components, such as enzymes, antibodies, or nucleic acids, with electronic systems to detect specific chemical substances. They operate by converting a biological response into an electrical signal, enabling quantitative analysis of various analytes. These devices are widely used in multiple fields, including healthcare, environmental monitoring, food safety, and biotechnology. Their ability to provide real-time, accurate, and sensitive detection makes them invaluable tools in modern science and industry.
There are several types of biosensors, broadly categorized into enzyme-based, DNA-based, electrochemical, mass-based, and optical biosensors. Each type is tailored for specific applications and detection mechanisms. For example, enzyme-based biosensors utilize enzymes for specific reactions, while optical biosensors rely on light interactions to detect analyte presence. The diversity in biosensor types allows for targeted applications across various scientific and industrial fields.
Biosensors have a wide range of applications. In the medical field, they are used for monitoring glucose levels in diabetic patients, detecting pathogens in clinical samples, and identifying biomarkers for various diseases. Environmental applications include detecting pollutants in water and air, while food safety biosensors can identify contaminants or spoilage indicators in food products. Additionally, biosensors are used in bioprocessing and industrial applications to monitor fermentation processes and other biochemical reactions.
Biosensors facilitate the detection and quantification of specific substances through the interaction between a biological recognition element and an analyte. Upon binding, a measurable signal is generated, which is then processed and interpreted to provide information about the concentration of the analyte present. This functionality allows biosensors to be used for diagnostic purposes, environmental assessments, and quality control in various industries.
Biosensors function through a two-step process: recognition and transduction. The recognition element, which can be an enzyme, antibody, or nucleic acid, selectively binds to the target analyte. This interaction induces a change that is captured by the transducer, converting the biological response into a measurable electrical signal. The signal is then amplified and processed to provide quantitative data about the analyte concentration, allowing for accurate detection and analysis.
A biosensor is an integrated analytical device designed to detect specific chemical or biological substances. It comprises two main components: a biological recognition element that interacts with the target analyte and a transducer that converts the recognition event into an electrical signal. This combination enables sensitive and specific detection, making biosensors crucial for various applications, including clinical diagnostics, environmental monitoring, and food safety analysis.
Biosensors can detect a diverse array of substances, including biomolecules such as glucose, cholesterol, and proteins, as well as pathogens, toxins, and environmental pollutants. For instance, glucose biosensors are widely used to monitor blood sugar levels, while DNA biosensors can identify specific genetic sequences in diagnostic tests. The specificity and sensitivity of biosensors make them effective tools for detecting even low concentrations of target analytes in complex samples.
Electrochemical biosensors operate by measuring changes in electrical properties, such as current or voltage, resulting from interactions between an analyte and the sensor surface. When the target analyte binds to the recognition element, it triggers a redox reaction that produces a measurable electrical signal. The signal is proportional to the concentration of the analyte, allowing for accurate quantification. Common examples include amperometric, potentiometric, and conductimetric sensors, each utilizing different electrochemical principles for detection.
Biosensors work by integrating biological and electronic systems to detect specific analytes. The process begins with the binding of the target analyte to a biological recognition element, which can be an enzyme, antibody, or nucleic acid. This interaction causes a biochemical change that is detected by the transducer, which converts the change into an electrical signal. The output signal is then processed and analyzed to determine the concentration of the analyte, enabling real-time monitoring and analysis.
The concept of biosensors was pioneered in the 1960s, with the first practical biosensor being developed by Updike and Hicks in 1967 for glucose detection. This innovation marked a significant milestone in analytical chemistry, leading to the proliferation of various biosensor technologies that have since been applied in diverse fields, including healthcare, environmental science, and food safety. The evolution of biosensors has continued, with ongoing research leading to more sophisticated and sensitive devices.
Biosensors involve linking biological recognition elements—such as enzymes, antibodies, or nucleic acids—with electronic circuitry. This integration allows the biosensor to convert biological interactions into measurable signals, enabling the detection of specific analytes. The choice of recognition element significantly impacts the sensor's specificity and sensitivity, making this linkage a crucial aspect of biosensor design and functionality.
A biosensor is a highly specialized analytical device designed to detect specific chemical or biological substances. It consists of two primary components: a biological recognition element that selectively interacts with a target analyte and a transducer that converts the interaction into a measurable signal. This integration enables sensitive and specific detection, making biosensors vital tools in clinical diagnostics, environmental monitoring, and food safety applications.
Biosensors are analytical devices that combine biological elements with electronic systems to detect specific substances. A prominent example is the glucose biosensor, which uses glucose oxidase as the recognition element. When glucose in a sample binds to the enzyme, a biochemical reaction occurs, producing an electrical signal proportional to the glucose concentration. This technology is crucial for diabetes management, allowing patients to monitor their blood sugar levels effectively.
Various materials and technologies are employed in biosensors, including enzymes (for enzymatic biosensors), antibodies (for immunosensors), and nucleic acids (for DNA biosensors). Additionally, electrochemical sensors, piezoelectric sensors, and optical sensors are widely used for specific detection applications. The choice of biosensor type depends on the target analyte and the required sensitivity and specificity for the application.
The specific invention date for biosensors used in fingerprint analysis is not well-documented. However, the application of biosensors in biometric systems has developed alongside advancements in sensor technology and has been integrated into various security and identification systems over the past two decades. The combination of biosensors with fingerprint recognition enhances the accuracy and reliability of biometric authentication.
The invention of biosensors can be traced back to Updike and Hicks, who developed the first practical glucose biosensor in 1967. This pioneering work laid the foundation for subsequent advancements in biosensor technology, leading to the creation of various types of biosensors that are now widely used in clinical diagnostics, environmental monitoring, and food safety, among other applications. Their contributions significantly impacted the field of analytical chemistry.
The cost of biosensors can vary significantly depending on their type, complexity, and application. Basic biosensors may cost as little as a few dollars, while more advanced systems, particularly those used in clinical diagnostics or specialized research, can range from several hundred to thousands of dollars. Factors influencing the price include the technology used, the specificity required, and the manufacturing processes involved in producing the biosensor.
- Mehrotra P. Biosensors and their applications – A review. J Oral Biol Craniofac Res. 2016 May-Aug;6(2):153-9. doi: 10.1016/j.jobcr.2015.12.002. Epub 2016 Jan 6. PMID: 27195214; PMCID: PMC4862100.
- Tetyana, P., Morgan Shumbula, P., & Njengele-Tetyana, Z. (2021). Biosensors: Design, Development and Applications. IntechOpen. doi: 10.5772/intechopen.97576
- Bhalla N, Jolly P, Formisano N, Estrela P. Introduction to biosensors. Essays Biochem. 2016 Jun 30;60(1):1-8. doi: 10.1042/EBC20150001. PMID: 27365030; PMCID: PMC4986445.
- https://www.electronicshub.org/types-of-biosensors/
- https://www.onlinebiologynotes.com/biosensors-components-working-principle-and-types/
- https://www.biologydiscussion.com/enzymes/biosensors/biosensors-features-principle-and-types-with-diagram/10240
- https://ijariie.com/AdminUploadPdf/BIOSENSORS__PRINCIPLE__TYPES_AND_APPLICATIONS_ijariie4676.pdf?srsltid=AfmBOooJeuQ7kN8339UyNyEiUUErjsjmp24kM6kTzc3zYrONazexvMy5
- https://www.turito.com/learn/biology/biosensors-grade-9
- https://www.news-medical.net/health/What-are-Biosensors.aspx