What are Biological Macromolecules?
- Biological macromolecules are vital large and complex molecules that underpin all forms of life. These macromolecules are primarily constructed from smaller subunits known as monomers, which bond together to form polymers. Understanding the four main classes of biological macromolecules—carbohydrates, proteins, nucleic acids, and lipids—illuminates their diverse roles in biological systems.
- Carbohydrates are formed from monosaccharides, which are simple sugar units linked by glycosidic bonds. These molecules serve multiple essential functions, including energy storage, structural support, and cell signaling. For instance, glucose acts as a primary energy source for cellular processes, while starch and glycogen serve as energy reserves in plants and animals, respectively. Additionally, cellulose, a structural polysaccharide found in plant cell walls, contributes to maintaining cell shape and integrity.
- Proteins are composed of long chains of amino acids connected by peptide bonds. They play critical roles in virtually every biological function. Enzymes, which are specialized proteins, catalyze biochemical reactions, significantly accelerating metabolic processes. Structural proteins provide support and shape to cells and tissues, while transport proteins facilitate the movement of substances across cellular membranes. Notable examples include hemoglobin, which transports oxygen in the bloodstream, and insulin, a hormone that regulates glucose levels in the body.
- Nucleic acids are polymers formed from nucleotides, each containing a sugar, a phosphate group, and a nitrogenous base. They serve as the carriers of genetic information, essential for heredity and protein synthesis. Deoxyribonucleic acid (DNA) encodes the genetic instructions for the development and functioning of living organisms, whereas ribonucleic acid (RNA) plays a crucial role in translating these instructions into proteins. Additionally, adenosine triphosphate (ATP) is a nucleotide that functions as an energy currency in cells, driving various biochemical reactions.
- Lipids, primarily composed of fatty acids and glycerol, are another essential class of biological macromolecules. They serve numerous functions, including energy storage, thermal insulation, and forming the structural framework of cell membranes. Triglycerides are the most common form of lipids, storing energy efficiently. Phospholipids, on the other hand, are key components of cell membranes, forming bilayers that separate the interior of the cell from its external environment. Cholesterol, a type of lipid, contributes to membrane fluidity and stability.
Definition of Biological Macromolecules
Biological macromolecules are large, complex molecules essential for life, typically classified into four main categories: carbohydrates, proteins, nucleic acids, and lipids. They are composed of smaller units called monomers, which are linked together to form polymers. These macromolecules play crucial roles in biological processes, including energy storage, structural support, information transmission, and catalysis of biochemical reactions.
Synthesis of Biological Macromolecules
The synthesis of biological macromolecules involves intricate biochemical processes essential for the structure and function of all living organisms. These macromolecules—proteins, carbohydrates, nucleic acids, and lipids—are constructed from smaller subunits called monomers, which are linked together through specific chemical reactions. Understanding these processes is fundamental for grasping how life functions at the molecular level.
- Monomer Construction: Biological macromolecules are formed by linking monomers through covalent bonds. Each class of macromolecule has its own specific type of monomer, contributing to the unique properties of the resulting polymer. For example, amino acids serve as the building blocks for proteins, while monosaccharides make up carbohydrates.
- Dehydration Reactions: The synthesis of macromolecules primarily occurs through dehydration reactions (also known as condensation reactions). During this process, two monomers combine, resulting in the release of a water molecule and the formation of a covalent bond. This reaction not only facilitates the creation of polymers but also contributes to the specific structural features of each macromolecule.
- Energy Investment: Dehydration reactions typically require an input of energy to form new bonds. This energy is necessary to overcome the activation barrier, enabling the monomers to join and form larger, more complex structures. Therefore, energy dynamics play a crucial role in the formation of biological macromolecules.
- Hydrolysis Reactions: Conversely, the breakdown of macromolecules into their constituent monomers occurs through hydrolysis reactions. During hydrolysis, water molecules are utilized to cleave the covalent bonds between monomers, resulting in the release of individual units. For each bond broken, a water molecule is consumed, thus demonstrating the importance of water in biochemical reactions.
- Specificity of Reactions: While the general mechanisms of dehydration and hydrolysis are consistent across all macromolecules, the specific reactions involved vary depending on the type of macromolecule. For instance, protein synthesis involves peptide bonds formed between amino acids, whereas polysaccharides result from glycosidic bonds formed between sugars.
- Emergence of Properties: It is essential to note that polymers exhibit properties that differ from those of their monomeric constituents. This phenomenon, often referred to as “emergent properties,” implies that the functional characteristics of a macromolecule—such as its osmotic pressure—are not merely a reflection of its individual components. Instead, they arise from the unique arrangement and interactions of these components in the polymeric structure.
- Biological Significance: The synthesis and breakdown of biological macromolecules are critical for numerous cellular processes, including metabolism, signaling, and structural integrity. Thus, understanding these processes provides valuable insights into how cells maintain homeostasis and respond to environmental changes.
Types of biological macromolecules
Biological macromolecules are essential components of all living organisms, serving as the building blocks for cellular structure and function. These macromolecules can be categorized into four primary types: nucleic acids, carbohydrates, proteins, and lipids. Each type has unique structures, building blocks, and functions, which are critical for the maintenance and regulation of life processes.
- Nucleic Acids:
- Nucleic acids are polymers made up of monomers known as nucleotides. Each nucleotide consists of a pentose sugar, a nitrogenous base, and a phosphate group.
- There are two main types of nucleic acids: deoxyribonucleic acid (DNA) and ribonucleic acid (RNA).
- DNA serves as the genetic blueprint, storing and transmitting hereditary information, while RNA plays a crucial role in translating these genetic instructions into proteins.
- DNA features a double-helix structure, with two complementary strands running in opposite directions, while RNA is typically single-stranded.
- Carbohydrates:
- Carbohydrates are composed of carbon, hydrogen, and oxygen atoms, generally following the empirical formula (CH₂O)n. They are categorized into monosaccharides, disaccharides, and polysaccharides based on their complexity.
- Monosaccharides, such as glucose and fructose, are the simplest form and serve as immediate energy sources.
- Disaccharides, formed from two monosaccharides, include sucrose and lactose, while polysaccharides, such as starch and cellulose, consist of long chains of monosaccharides linked by glycosidic bonds.
- These polymers provide energy storage and structural support, with cellulose playing a vital role in maintaining the integrity of plant cell walls.
- Proteins:
- Proteins are large, complex molecules made from chains of amino acids, which are linked by peptide bonds.
- Each protein’s unique function is determined by its specific sequence of amino acids, which folds into distinct three-dimensional structures.
- Proteins serve various roles, including acting as enzymes that catalyze biochemical reactions, transporting molecules across cell membranes, and providing structural support within cells and tissues.
- The structural organization of proteins is hierarchical, encompassing primary, secondary, tertiary, and quaternary levels, with each level contributing to the protein’s overall function.
- Lipids:
- Lipids are hydrophobic molecules primarily composed of carbon and hydrogen, with varying structures and functions.
- Major types of lipids include fats, oils, waxes, phospholipids, and steroids.
- Fats and oils serve as long-term energy storage, while phospholipids are essential components of cell membranes, forming bilayers that provide structural integrity.
- Steroids, characterized by a structure of four fused carbon rings, include cholesterol, which stabilizes cell membranes and serves as a precursor for steroid hormones.
Properties of Biological Macromolecules
Biological macromolecules exhibit a diverse array of properties that are fundamental to their functions within living organisms. These macromolecules—carbohydrates, lipids, proteins, and nucleic acids—differ significantly in their structures and roles, contributing to the complexity of life. Understanding these properties is essential for comprehending how these molecules interact within biological systems.
- Carbohydrates:
- Carbohydrates are primarily characterized by their composition of carbon, hydrogen, and oxygen, typically following the empirical formula (CH₂O)n.
- They are classified into three categories based on their structure: monosaccharides (simple sugars), disaccharides (two monosaccharides linked together), and polysaccharides (long chains of monosaccharides).
- The most common monosaccharide is glucose (C₆H₁₂O₆), which plays a crucial role in cellular metabolism, particularly in glycolysis.
- Carbohydrates contain multiple hydroxyl groups and one carbonyl group. If the carbonyl group is at the end of the molecule, it forms an aldehyde (aldose), while if it is in the middle, it results in a ketone (ketose).
- The structural diversity of carbohydrates affects their function; for example, polysaccharides like cellulose provide structural support in plant cell walls, while glycogen serves as an energy storage molecule in animals.
- Lipids:
- Unlike carbohydrates and proteins, lipids do not form polymers, making them unique among macromolecules. They are predominantly nonpolar and hydrophobic, meaning they do not readily mix with water.
- Lipids are essential for energy storage, with fats providing the most energy per gram (9 calories). They are composed of glycerol and fatty acids, forming structures like triglycerides.
- Fatty acids can be classified as saturated or unsaturated based on the presence of double bonds between carbon atoms. Saturated fatty acids lack double bonds, are typically solid at room temperature, and are predominantly found in animal fats. In contrast, unsaturated fatty acids contain one or more double bonds, are generally liquid at room temperature, and are found in plant oils.
- Phospholipids, crucial for forming biological membranes, consist of two fatty acids, a glycerol molecule, and a phosphate group. Their amphipathic nature—with a hydrophilic (water-attracting) head and hydrophobic (water-repelling) tails—allows them to form bilayers that constitute cell membranes.
- Steroids, characterized by a four-ring carbon structure, are another class of lipids. Cholesterol, a type of steroid, is integral to cell membrane fluidity and serves as a precursor for steroid hormones.
- Proteins:
- Proteins are polymers made from 20 different amino acids, which are linked by peptide bonds. The sequence of amino acids determines the protein’s unique properties and functions.
- Each amino acid consists of a central carbon atom bonded to an amino group, a carboxyl group, a hydrogen atom, and a variable side chain (R group) that dictates its chemical properties.
- Proteins are categorized based on their structural organization: primary (amino acid sequence), secondary (local folding patterns like alpha helices and beta sheets), tertiary (three-dimensional shape), and quaternary (multiple polypeptides interacting).
- The specific interactions among amino acids, influenced by their R groups, lead to the protein’s functional conformation. Changes in temperature, pH, or amino acid sequence can result in denaturation, altering protein function.
- Nucleic Acids:
- Nucleic acids are composed of nucleotides, which include a five-carbon sugar, a phosphate group, and a nitrogenous base.
- There are two main types: deoxyribonucleic acid (DNA) and ribonucleic acid (RNA). DNA stores genetic information and is structured as a double helix, while RNA is usually single-stranded and plays various roles in protein synthesis.
- The nitrogenous bases in nucleotides include adenine, thymine, guanine, cytosine, and uracil (in RNA). The specific sequence of these bases encodes genetic information critical for cellular functions.
What are Monomers and Polymers?
Monomers and polymers are fundamental concepts in chemistry, particularly in the study of macromolecules. Understanding these terms is essential for grasping the structure and function of various biological molecules, such as proteins, nucleic acids, carbohydrates, and lipids.
Monomers
- Definition: Monomers are small, simple molecules that can join together to form larger structures known as polymers. They are the basic building blocks of more complex molecules.
- Examples:
- Amino Acids: The monomers that make up proteins. There are 20 different amino acids, each with a specific side chain that determines its properties.
- Nucleotides: The monomers of nucleic acids like DNA and RNA. Each nucleotide consists of a nitrogenous base, a sugar (ribose or deoxyribose), and a phosphate group.
- Monosaccharides: The simplest form of carbohydrates, such as glucose and fructose, which can combine to form more complex sugars (disaccharides and polysaccharides).
Polymers
- Definition: Polymers are large molecules composed of repeating structural units (monomers) connected by covalent bonds. They can be very long chains and can have complex structures.
- Characteristics:
- Polymers can be natural or synthetic. Natural examples include proteins, nucleic acids, and polysaccharides, while synthetic examples include plastics like polyethylene and nylon.
- They often exhibit diverse properties, including elasticity, strength, and resistance to chemicals, depending on the arrangement and type of monomers used in their formation.
- Examples:
- Proteins: Polymers made up of amino acid monomers linked by peptide bonds. They perform various functions, including catalysis (enzymes), structure (collagen), and transport (hemoglobin).
- DNA and RNA: Polymers made of nucleotide monomers that carry genetic information and are involved in protein synthesis.
- Polysaccharides: Such as starch, glycogen, and cellulose, which are composed of monosaccharide monomers (like glucose) linked together. These serve functions like energy storage and structural support in cells.
Most macromolecules are polymers, built from monomers
Most macromolecules are polymers, which are large, chain-like structures formed by linking smaller subunits known as monomers. This structural relationship is foundational to understanding the complexity and diversity of biological molecules. Below is a detailed overview of how macromolecules are built and their implications in biological systems.
- Definition of Polymers and Monomers:
- A polymer is defined as a long molecule comprised of many similar or identical building blocks connected by covalent bonds.
- The repeating units in these chains are called monomers. They can also serve additional functions independent of their role in forming polymers.
- Formation of Polymers:
- The process of creating polymers involves a reaction known as a condensation reaction or dehydration reaction, in which monomers are linked together.
- During this reaction, a water molecule is released. One monomer contributes a hydroxyl group (–OH), while the other provides a hydrogen atom (–H), resulting in the formation of a covalent bond between them.
- This synthesis requires energy input from the cell and is facilitated by enzymes, which serve as catalysts to increase the reaction rate.
- Breaking Down Polymers:
- The reverse process of polymer formation is called hydrolysis. This reaction breaks the covalent bonds between monomers by adding water molecules.
- In hydrolysis, a hydrogen atom attaches to one monomer and a hydroxyl group attaches to the adjacent monomer, effectively separating them.
- This reaction is crucial during digestion; for instance, organic polymers from food are too large for absorption in their original form. Enzymes in the digestive tract catalyze the hydrolysis of these polymers into monomers, which can then be absorbed by cells lining the gut and transported into the bloodstream.
- Reassembly of Monomers:
- Once in the bloodstream, these monomers can be utilized by body cells. Cells employ dehydration reactions to assemble the absorbed monomers into new polymers, which perform specific functions tailored to the needs of the cell type.
- This process emphasizes the versatility of cells in utilizing available resources to create the necessary molecules for their function and survival.
- Diversity of Polymers:
- The vast array of polymers in living organisms arises from the combinations of a relatively small set of common monomers, typically ranging from 40 to 50. This limited number of building blocks can be arranged in numerous combinations, much like the 26 letters of the alphabet can be combined to form countless words.
- Each cell type within an organism has a unique assortment of macromolecules, reflecting its specific functions and roles. Furthermore, there is variability not only among cells of the same individual but also greater diversity between unrelated individuals and species.
Structure and Function of Biological Macromolecules
1. Carbohydrates serve as fuel and building material
Carbohydrates are essential macromolecules that serve as both fuel and building materials in living organisms. Their diverse structures and functions make them fundamental to various biological processes. Below is a detailed overview of carbohydrates, emphasizing their roles in energy provision and structural integrity.
- Carbohydrates – Definition, Examples, Structure, Types, and Functions
- Carbohydrates – Monosaccharides, Disaccharides, Polysaccharides
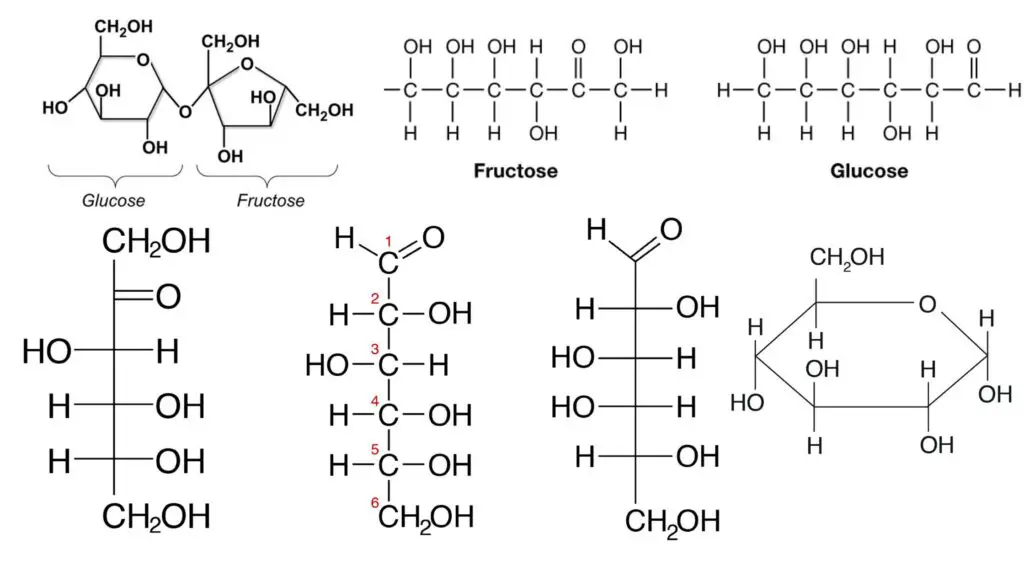
- Types of Carbohydrates:
- Carbohydrates encompass sugars and their polymers, which can be classified into three main categories: monosaccharides, disaccharides, and polysaccharides.
- Monosaccharides: These are the simplest carbohydrates, consisting of single sugar units. They generally follow the empirical formula CH2OCH_2OCH2O and can be categorized based on the location of their carbonyl group and the number of carbon atoms. For instance, glucose (an aldose) and fructose (a ketose) are structural isomers with distinct properties.
- Disaccharides: Formed from two monosaccharides linked by a condensation reaction, disaccharides serve as short-term energy sources. Examples include sucrose (glucose + fructose), lactose (glucose + galactose), and maltose (two glucose molecules).
- Polysaccharides: These are long chains of monosaccharides linked together. They can serve various functions, including energy storage and structural roles.
- Function as Fuel:
- Monosaccharides, particularly glucose, are primary energy sources for cellular activities. They provide the carbon skeletons necessary for synthesizing other biological molecules, including amino acids and fatty acids.
- In living organisms, carbohydrates are metabolized to release energy. This energy fuels various biological processes, making carbohydrates vital for growth and maintenance.
- Storage Polysaccharides:
- Polysaccharides such as starch and glycogen function primarily as energy storage molecules.
- Starch: Composed entirely of glucose monomers, starch is the main storage form of carbohydrates in plants. It exists in two forms: amylose, which is unbranched and helical, and amylopectin, which is branched and more complex. Plants store excess glucose as starch granules in plastids, such as chloroplasts, and can mobilize these reserves when energy is required.
- Glycogen: The storage form of glucose in animals, glycogen is highly branched and primarily stored in the liver and muscle tissues. It serves as a readily available energy source, with animals capable of hydrolyzing glycogen to release glucose when needed.
- Structural Polysaccharides:
- Carbohydrates also play a crucial role in providing structural integrity.
- Cellulose: As a major component of plant cell walls, cellulose is a polysaccharide formed from beta glucose monomers. The unique glycosidic linkages create straight chains that enable hydrogen bonding between strands, resulting in strong microfibrils that contribute to plant rigidity. Despite being indigestible for humans, cellulose aids in digestive health by providing dietary fiber, promoting intestinal movement.
- Chitin: Another important structural polysaccharide, chitin is found in the exoskeletons of arthropods and the cell walls of many fungi. Similar to cellulose, chitin contains nitrogen-containing appendages, contributing to its strength and resilience.
- Digestive Interactions:
- While humans cannot digest cellulose due to the inability to hydrolyze beta linkages, some microorganisms possess cellulase enzymes that enable them to break down cellulose into glucose monomers. This capability is crucial for herbivores like cows and termites, which rely on symbiotic relationships with these microbes to access the energy stored in cellulose.
- The indigestible nature of cellulose serves a functional purpose in human nutrition. As it passes through the digestive tract, cellulose stimulates mucus secretion and enhances bowel movements, promoting digestive health.
2. Lipids are a diverse group of hydrophobic molecules
Lipids represent a diverse group of hydrophobic molecules that play crucial roles in biological systems. Unlike other macromolecules, lipids do not form polymers but exhibit a range of structures and functions that contribute significantly to cellular processes. This overview details the various types of lipids and their essential roles in energy storage, membrane structure, and signaling.
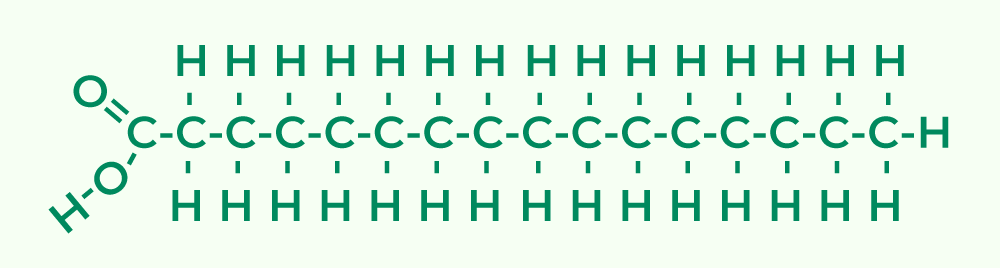
- Hydrophobic Nature of Lipids:
- The defining characteristic of lipids is their hydrophobicity, which results from their high proportion of hydrocarbons. These hydrocarbons form nonpolar covalent bonds, leading to little or no affinity for water.
- This hydrophobic property allows lipids to separate from aqueous environments, a feature that is crucial for their biological functions.
- Types of Lipids:
- Lipids are primarily categorized into three groups: fats, phospholipids, and steroids.
- Fats:
- Fats, also known as triglycerides, are large molecules formed from smaller components through dehydration reactions. They consist of glycerol and fatty acids.
- Glycerol is a three-carbon alcohol with a hydroxyl group (-OH) on each carbon atom.
- Fatty Acids consist of a carboxyl group (-COOH) attached to a long hydrocarbon chain, usually containing 16 to 18 carbon atoms. The long chains of nonpolar C—H bonds confer hydrophobic properties to fats.
- Fats are classified based on the saturation of their fatty acids:
- Saturated Fatty Acids: Contain no carbon-carbon double bonds, resulting in straight chains that can pack tightly. These fats are typically solid at room temperature and are commonly found in animal fats.
- Unsaturated Fatty Acids: Possess one or more double bonds, creating kinks in the chain that prevent tight packing. These fats are usually liquid at room temperature and are found in plant oils and fish fats.
- The primary function of fats is energy storage, as they store more than twice the energy per gram compared to polysaccharides like starch. This energy density is particularly advantageous for animals, allowing them to carry compact energy reserves.
- Adipose Tissue:
- In mammals, fats are stored in adipose tissue, which serves as a long-term energy reservoir. Adipose cells can expand or contract based on fat storage and mobilization needs. This tissue also provides cushioning for vital organs and functions as insulation, particularly in marine mammals such as whales and seals.
- Phospholipids:
- Phospholipids are essential components of cell membranes. They are structurally similar to fats but have two fatty acids linked to glycerol and a phosphate group at the third position.
- The phosphate group carries a negative charge, creating a hydrophilic (water-attracting) head, while the fatty acid tails remain hydrophobic.
- In aqueous environments, phospholipids spontaneously arrange themselves into bilayers, with hydrophilic heads facing outward and hydrophobic tails tucked inward. This bilayer forms a semipermeable barrier that is fundamental to cellular structure and function.
- Steroids:
- Steroids are another class of lipids characterized by a carbon skeleton with four fused rings. Variations in functional groups attached to these rings lead to different steroid molecules.
- Cholesterol is a notable steroid that serves multiple functions, including maintaining membrane fluidity in animal cells. It also acts as a precursor for the synthesis of various hormones, including sex hormones.
- While cholesterol is essential for physiological functions, elevated blood cholesterol levels can lead to cardiovascular diseases, particularly when combined with high intake of saturated and trans fats.
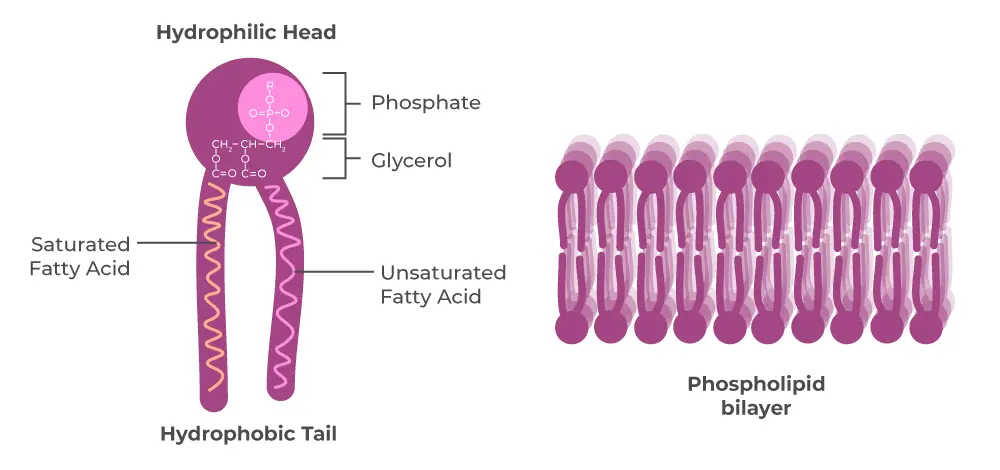
3. Proteins have many structures, resulting in a wide range of functions
Proteins are fundamental biomolecules that account for more than half of the dry mass of most cells, participating in nearly every biological process. Their structural complexity enables them to perform a diverse array of functions, making them essential for life. This overview elucidates the various structures of proteins and how these structures correlate with their multifaceted functions.
- Diverse Functions of Proteins:
- Proteins play pivotal roles in structural support, storage, transport, cellular signaling, movement, and immune defense against pathogens.
- Most notably, enzymes, a specific type of protein, act as catalysts in biochemical reactions, regulating metabolic pathways by accelerating chemical reactions without being consumed in the process.
- The human body alone contains tens of thousands of different proteins, each tailored to fulfill specific roles, highlighting the significance of protein diversity.
- Building Blocks of Proteins:
- Proteins are polymers composed of amino acid monomers. There are 20 different amino acids, each characterized by a unique side chain, or R group, which determines its specific properties and functions.
- Amino acids consist of an asymmetric alpha carbon bonded to four groups: a hydrogen atom, a carboxyl group (-COOH), an amino group (-NH₂), and a variable R group. The variation among R groups contributes to the wide range of protein functions.
- Amino acids can be categorized based on their side chains into three groups: hydrophobic, hydrophilic (polar), and charged (acidic or basic).
- Peptide Bond Formation:
- Amino acids link together via peptide bonds formed during dehydration reactions. This process involves the removal of a hydroxyl group from the carboxyl end of one amino acid and a hydrogen atom from the amino group of another.
- Repeated peptide bond formation results in polypeptide chains, which can range from a few to thousands of amino acids long. The unique sequence of amino acids in a polypeptide is termed its primary structure.
- Protein Structure Levels:
- Primary Structure: This refers to the specific sequence of amino acids in a polypeptide chain, which is determined by genetic information. For instance, the primary structure of lysozyme, an antibacterial enzyme, consists of 129 amino acids.
- Secondary Structure: This level involves local folding patterns within a polypeptide, stabilized by hydrogen bonds between the backbone constituents. Common secondary structures include alpha helices and beta-pleated sheets, contributing to the overall stability of proteins, such as silk fibers.
- Tertiary Structure: This structure arises from interactions among R groups, including hydrogen bonds, ionic bonds, and hydrophobic interactions. The tertiary structure is critical for the protein’s overall shape and function.
- Quaternary Structure: This level of organization occurs when two or more polypeptide chains aggregate to form a functional protein. Hemoglobin, for example, has a quaternary structure with four subunits that facilitate oxygen transport.
- Determining Protein Function through Conformation:
- The specific three-dimensional shape of a protein is crucial for its function, as it dictates the protein’s interactions with other molecules. For instance, enzymes bind specific substrates to catalyze biochemical reactions, while antibodies recognize foreign substances.
- Proteins can undergo denaturation due to changes in environmental conditions such as pH, temperature, or salt concentration, which disrupt the stabilizing forces maintaining their conformation. Denaturation often results in loss of function.
- Protein Folding and Chaperonins:
- The folding process of a polypeptide into its functional conformation occurs spontaneously, assisted by various intramolecular forces and bonds. The role of chaperonins—special proteins that facilitate correct folding without dictating the final structure—can be vital in this process.
- Advanced techniques like X-ray crystallography and nuclear magnetic resonance (NMR) spectroscopy are employed to study protein conformations, helping researchers visualize the complex shapes that proteins adopt.
- Implications of Protein Structure:
- Even minor alterations in a protein’s primary structure can significantly impact its function. For instance, a single amino acid substitution in hemoglobin can lead to sickle-cell disease, showcasing the delicate balance between structure and function.
- As biochemists continue to unravel the sequences and structures of proteins, the understanding of their functions in various biological contexts deepens, illustrating the importance of protein diversity and complexity in life.
4. Nucleic acids store and transmit hereditary information
- DNA – Definition, Structure, Properties, Types, Functions
- RNA – Definition, Structure, Types, Application
- Nucleotides and Nucleosides – Definition, Structure, Function, Types
Nucleic acids play a critical role in storing and transmitting hereditary information, serving as the molecular foundation for biological inheritance. They are essential for the reproduction of complex organisms and the continuation of genetic material from one generation to the next. This discussion will explore the two primary types of nucleic acids, their structural components, and their functional significance in heredity and protein synthesis.
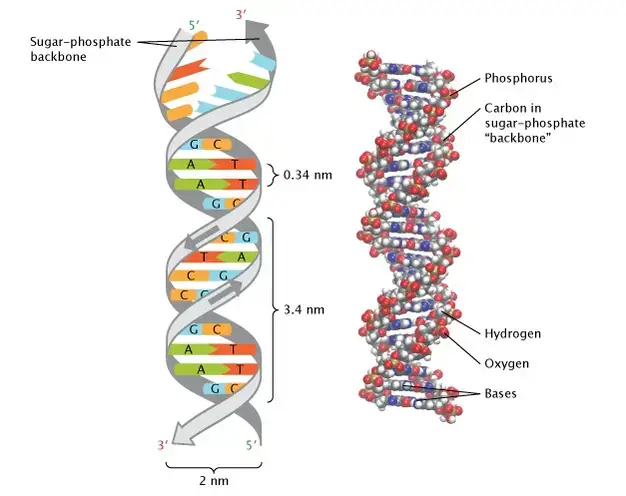
- Types of Nucleic Acids:
- There are two main types of nucleic acids: ribonucleic acid (RNA) and deoxyribonucleic acid (DNA).
- Both types of nucleic acids are vital for allowing living organisms to replicate their genetic components and pass them on to offspring.
- Functions of DNA and RNA:
- DNA serves as the genetic blueprint for an organism, providing instructions for its replication and directing RNA synthesis. RNA, in turn, plays a pivotal role in translating the genetic information contained in DNA into proteins.
- The central dogma of molecular biology outlines the flow of genetic information: DNA is transcribed into RNA, which is then translated into proteins. Therefore, DNA is not only responsible for encoding genetic information but also for orchestrating the synthesis of proteins, which carry out various cellular functions.
- Structure of Nucleic Acids:
- Nucleic acids are polymers made up of monomer units known as nucleotides. Each nucleotide consists of three components: a nitrogenous base, a pentose sugar, and a phosphate group.
- The nitrogenous bases can be categorized into two groups: purines (adenine and guanine) and pyrimidines (cytosine, thymine, and uracil). In RNA, uracil replaces thymine, which is found in DNA.
- The sugar component is ribose in RNA and deoxyribose in DNA, differing by the presence of an oxygen atom on the second carbon of the sugar. The combination of a sugar and a nitrogenous base forms a nucleoside, while the addition of a phosphate group produces a nucleotide.
- Formation of Nucleic Acid Polymers:
- Polynucleotides, such as DNA and RNA, are formed when nucleotides link together through covalent bonds known as phosphodiester linkages. These linkages occur between the hydroxyl group on the 3′ carbon of one nucleotide and the phosphate group on the 5′ carbon of another.
- This results in a repeating sugar-phosphate backbone with nitrogenous bases protruding from the sides. The two ends of the nucleic acid strand are distinct: one end has a phosphate group attached to the 5′ carbon (5′ end), and the other has a hydroxyl group attached to the 3′ carbon (3′ end).
- Gene Structure and Function:
- A gene, defined as a unit of inheritance, is comprised of DNA that encodes the information necessary for producing specific proteins. Each gene consists of hundreds to thousands of nucleotides, forming unique sequences that determine the primary structure of proteins.
- The primary structure of a protein, which is the specific sequence of amino acids, dictates its three-dimensional conformation and, subsequently, its function. This underscores the importance of the linear order of bases in DNA, which provides the instructions for protein synthesis.
- DNA Replication and Inheritance:
- The DNA molecule is characterized by its double helix structure, which consists of two polynucleotide strands wound around each other. This structure was elucidated by James Watson and Francis Crick in 1953.
- The two strands of DNA run in opposite directions, a configuration known as antiparallel. Base pairing rules dictate that adenine pairs with thymine and guanine pairs with cytosine, creating complementary strands.
- During cell division, each strand of DNA serves as a template for the synthesis of a new complementary strand, ensuring that genetic information is accurately replicated and passed to daughter cells. This mechanism is fundamental for maintaining genetic continuity across generations.
- Molecular Evidence of Evolution:
- Nucleic acids not only facilitate heredity but also provide a molecular basis for understanding evolutionary relationships among species. DNA and protein sequences can reveal similarities and differences that reflect common ancestry.
- For example, a comparison of hemoglobin polypeptide sequences shows that closely related species, such as humans and gorillas, have very few amino acid differences, while more distantly related species, like humans and frogs, exhibit greater divergence.
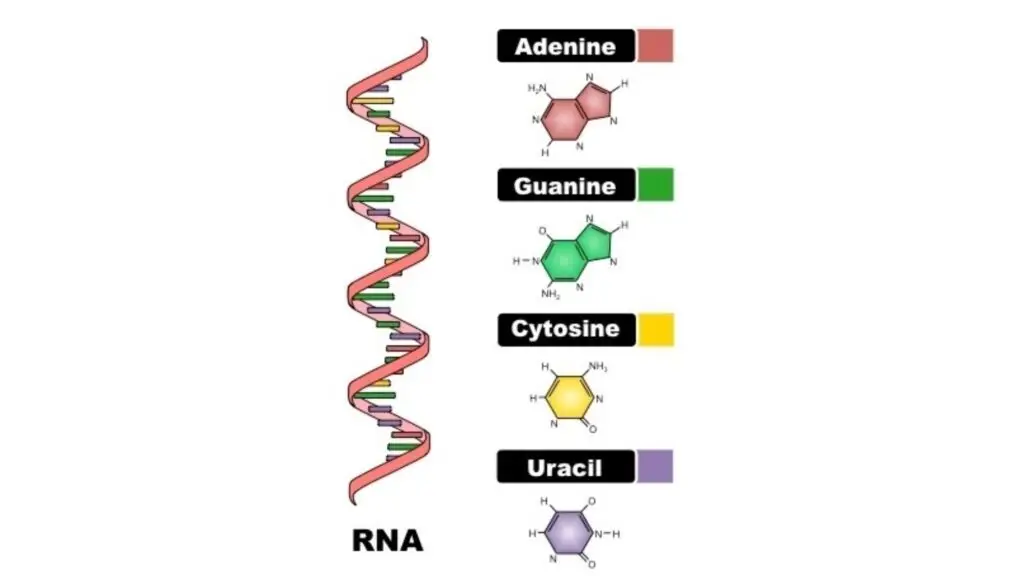
Examples of Macromolecules
Biological macromolecules are large, complex molecules essential for various functions in living organisms. They can be categorized into several major types, each with specific examples that illustrate their roles and properties. Understanding these examples is crucial for grasping the significance of macromolecules in biological systems.
- Synthetic Fibers:
- Synthetic fibers such as nylon, rayon, and spandex are composed entirely of macromolecules. These materials are created through a multi-step process.
- Initially, monomers undergo chemical reactions to form prepolymers, which are liquid precursors of the macromolecule.
- Next, these prepolymers are processed through a spinning technique. In this stage, the liquid solidifies to achieve the desired thickness and structure. This transformation is a critical step in manufacturing fibers that are widely used in textiles and various industrial applications.
- Synthetic fibers such as nylon, rayon, and spandex are composed entirely of macromolecules. These materials are created through a multi-step process.
- Genetic Transfer:
- DNA (deoxyribonucleic acid) serves as the primary genetic material in organisms, encoding the instructions necessary for life.
- During the process of meiosis, which is crucial for sexual reproduction, DNA undergoes significant changes. It is during this process that DNA is divided and fragmented, with the remaining nucleotides playing a vital role in transferring genetic information to gametes (sperm and egg cells). This transfer is essential for the continuation of genetic information from one generation to the next.
- DNA (deoxyribonucleic acid) serves as the primary genetic material in organisms, encoding the instructions necessary for life.
- Monomers and Polymers:
- Macromolecules can be understood fundamentally as polymers, which are long chains formed from molecular subunits known as monomers.
- Carbohydrates, proteins, and nucleic acids are notable examples of these polymers, each exhibiting unique structural and functional properties.
- Carbohydrates consist of sugar monomers, which link to form complex structures such as starch and cellulose, providing energy storage and structural support in plants.
- Proteins are made up of amino acid monomers that combine in specific sequences to create polypeptides. These proteins perform a wide variety of functions, including catalyzing biochemical reactions, supporting cellular structure, and facilitating communication between cells.
- Nucleic acids, including DNA and RNA, consist of nucleotide monomers. These nucleotides encode genetic information and are crucial for protein synthesis, ensuring that the cellular functions are executed correctly.
- Carbohydrates, proteins, and nucleic acids are notable examples of these polymers, each exhibiting unique structural and functional properties.
- Macromolecules can be understood fundamentally as polymers, which are long chains formed from molecular subunits known as monomers.
Functions of Macromolecules
Macromolecules play critical roles in the structure and function of living organisms. They are involved in various biological processes and are fundamental to cellular integrity, energy storage, and information transfer. Below is an overview of the primary types of macromolecules and their essential functions:
- Carbohydrates:
- Serve as a primary energy source for cells, providing quick energy through simple sugars (monosaccharides) such as glucose.
- Play a vital role in energy storage; for example, plants store energy in the form of starch, while animals use glycogen.
- Provide structural support; cellulose in plant cell walls and chitin in the exoskeletons of arthropods contribute to rigidity and strength.
- Participate in cell recognition and signaling processes; carbohydrates on the cell surface act as recognition sites for cell-cell interactions and communication.
- Proteins:
- Perform a wide range of functions, including serving as enzymes that catalyze biochemical reactions, thus speeding up metabolic processes.
- Act as structural components, providing support and shape to cells and tissues, as seen in collagen in connective tissues and keratin in hair and nails.
- Function in transport; proteins like hemoglobin carry oxygen in the blood, while membrane proteins facilitate the transport of molecules across cell membranes.
- Play roles in signaling and communication, where hormones (e.g., insulin) and receptors regulate physiological processes and maintain homeostasis.
- Lipids:
- Serve as long-term energy storage molecules, providing more than twice the energy per gram compared to carbohydrates.
- Form essential components of cell membranes; phospholipids create a bilayer that provides a barrier between the interior of the cell and its environment, regulating the movement of substances.
- Act as signaling molecules; steroid hormones, derived from lipids, regulate various biological functions, including growth, metabolism, and reproduction.
- Provide insulation and protection; fats serve to insulate body temperature in animals and protect vital organs from physical damage.
- Nucleic Acids:
- Store and transmit genetic information; DNA encodes the hereditary information required for the development and functioning of organisms.
- Facilitate protein synthesis; RNA plays various roles in translating the genetic code from DNA into proteins, with messenger RNA (mRNA), transfer RNA (tRNA), and ribosomal RNA (rRNA) all contributing to this process.
- Participate in regulatory functions; certain RNA molecules, such as microRNA, regulate gene expression and protein synthesis.
Flashcard
What are biological macromolecules?
Large, complex molecules essential for life, including carbohydrates, proteins, nucleic acids, and lipids.
Quiz Practice
- https://www.khanacademy.org/science/ap-biology/chemistry-of-life/properties-structure-and-function-of-biological-macromolecules/a/hs-biological-macromolecules-review
- https://library.fiveable.me/ap-bio/unit-1/properties-biological-macromolecules/study-guide/aAm3FBn4yaR06XhOtFLI
- https://byjus.com/biology/macromolecule/
- https://www.geeksforgeeks.org/lipids-function-structure-example/
- https://openstax.org/books/biology-ap-courses/pages/3-chapter-summary#:~:text=Proteins%2C%20carbohydrates%2C%20nucleic%20acids%2C,bonds%20to%20form%20larger%20polymers.
- https://www.kaptest.com/study/ap-biology/ap-biology-notes-biological-molecules/?srsltid=AfmBOopLar_mpDlnpwA-AHNVc-arg3q_D3kbKklJXAqQSaCXpL9u6gYT
- https://course-notes.org/biology/outlines/chapter_5_the_structure_and_function_of_macromolecules
- https://biologydictionary.net/ap-biology/1-3-introduction-to-biological-macromolecules/
- https://inspiritvr.com/properties-of-biological-macromolecules-study-guide/