What are bacteria?
- Bacteria are microscopic, single-celled organisms belonging to the prokaryotic domain, meaning their cellular structure lacks a membrane-bound nucleus and organelles. They are classified under the domain Bacteria in the three-domain system, which also includes Archaea and Eukaryota. The term “bacterium” originates from the Greek word baktērion, meaning “staff” or “cane,” referring to the rod-like shape of the first bacteria observed.
- Bacteria are among the earliest known life forms on Earth and exist in nearly all environments, including soil, water, air, and extreme habitats like acidic hot springs and deep-sea hydrothermal vents. They play essential roles in ecological processes such as the nutrient cycle, where they recycle nutrients, decompose organic matter, and fix atmospheric nitrogen. Some extremophilic bacteria, found in environments with high levels of hydrogen sulfide or methane, convert these compounds into energy, sustaining ecosystems in harsh conditions.
- These organisms form complex relationships with plants, animals, and humans. While many bacteria are harmless or beneficial, such as those aiding digestion in the human gut, others are pathogenic and cause diseases like tuberculosis, cholera, and syphilis. Respiratory infections caused by bacteria are among the most common causes of death globally. Antibiotics are widely used to treat bacterial infections, but their overuse has led to a rise in antibiotic-resistant strains.
- Bacteria have significant applications in various industries. They are utilized in the production of fermented foods like cheese and yogurt, sewage treatment, oil spill remediation, and biomining processes to extract metals. They are also critical in biotechnology, including the development of antibiotics and other chemical products.
- Historically, bacteria were once thought to be simple plants, classified under the group Schizomycetes. Modern research has since redefined them as prokaryotes, with fundamental differences from eukaryotic organisms. Genetic studies revealed that prokaryotes include two distinct groups: Bacteria and Archaea, which evolved from a shared ancient ancestor. This distinction underscores the complexity and diversity within microbial life.
- Bacteria exhibit a wide range of forms, functions, and ecological roles, making them indispensable to life on Earth and invaluable in scientific research and industrial applications.
Definition of Bacteria
Bacteria are microscopic, single-celled prokaryotic organisms lacking a nucleus and membrane-bound organelles, found in diverse environments, and essential for ecological processes, industrial applications, and human health.
Origin and Early Evolution of Bacteria
Bacteria represent some of the earliest life forms on Earth, with origins dating back approximately 4 billion years. They played a central role in shaping the planet’s biological and evolutionary history, influencing the development of other life forms.
- Earliest Life Forms:
- Bacteria evolved from simple, unicellular microorganisms.
- They dominated Earth’s biosphere for roughly 3 billion years, alongside archaea, before complex life forms emerged.
- Fossilized bacterial structures, such as stromatolites, provide evidence of their early existence but lack morphological detail to track specific evolutionary timelines.
- Molecular Evidence of Evolution:
- Genetic analysis has been pivotal in reconstructing bacterial phylogeny.
- Studies suggest bacteria were the first lineage to diverge from the shared ancestor of archaea and eukaryotes.
- The last universal common ancestor (LUCA) of bacteria and archaea likely existed between 2.5 and 3.2 billion years ago and may have been a hyperthermophile, thriving in extreme heat.
- Transition to Land:
- Bacteria are believed to be among the first organisms to colonize terrestrial environments, approximately 3.22 billion years ago.
- Role in Eukaryotic Evolution:
- The divergence of archaea and eukaryotes marked a major evolutionary milestone, with bacteria playing a key role in this process.
- Eukaryotic cells likely originated through endosymbiotic relationships between ancestral archaea and bacteria.
- Ancient proto-eukaryotic cells incorporated alphaproteobacteria, giving rise to mitochondria or hydrogenosomes, essential organelles in all known eukaryotic cells.
- Later, some eukaryotes with mitochondria engulfed cyanobacteria-like organisms, resulting in chloroplasts found in algae and plants. This process, known as primary endosymbiosis, further diversified life on Earth.
- Endosymbiosis and Cellular Complexity:
- The symbiotic integration of bacteria into host cells not only gave rise to mitochondria and chloroplasts but also significantly enhanced cellular energy production and metabolic capabilities.
- These events laid the foundation for the emergence of complex multicellular organisms.
Size of Bacteria
Bacteria are microscopic organisms, and their size varies greatly across different species. While most bacteria fall within a narrow size range, certain species can exceed the typical dimensions significantly.
General Size Range of Bacteria
- Bacteria typically range in size from 0.2 μm to 100 μm.
- The size of bacteria plays a critical role in their ability to perform specific functions, including nutrient uptake, motility, and environmental adaptation.
Size of Specific Bacterial Shapes
- Cocci (Spherical):
- The diameter of cocci generally ranges from 0.2 μm to 1.0 μm.
- This size range applies to most cocci species, which are typically small but can vary slightly depending on the arrangement or specific species.
- Bacilli (Rod-shaped):
- Bacilli are typically 1.0 μm to 5 μm in length and 0.5 μm to 1.0 μm in diameter.
- This shape allows bacilli to have a more elongated structure, aiding in their motility and surface area for nutrient absorption.
- Spirochetes (Spiral):
- These bacteria are usually 20 μm in length and 0.1 μm to 1.0 μm in diameter.
- Their unique spiral shape and dimensions help them move through viscous environments, such as mucus or soil, with greater efficiency.
Extremes in Size
- Smallest Bacilli:
- The smallest known bacilli are Pelagibacter ubique, measuring between 370–890 nm in length and 120–200 nm in diameter.
- These tiny bacteria are among the smallest living organisms known, thriving in oceanic environments.
- Smallest Cocci:
- The smallest cocci are Mycoplasma genitalium, which have a diameter of 200–300 nm.
- Despite their small size, these bacteria are capable of living and reproducing within the cells of their host organisms.
- Largest Bacteria:
- The largest known bacteria is Thiomargarita namibiensis, with a diameter of 0.75 mm.
- This massive size allows the bacterium to be visible to the naked eye, an extreme outlier in the bacterial size range.
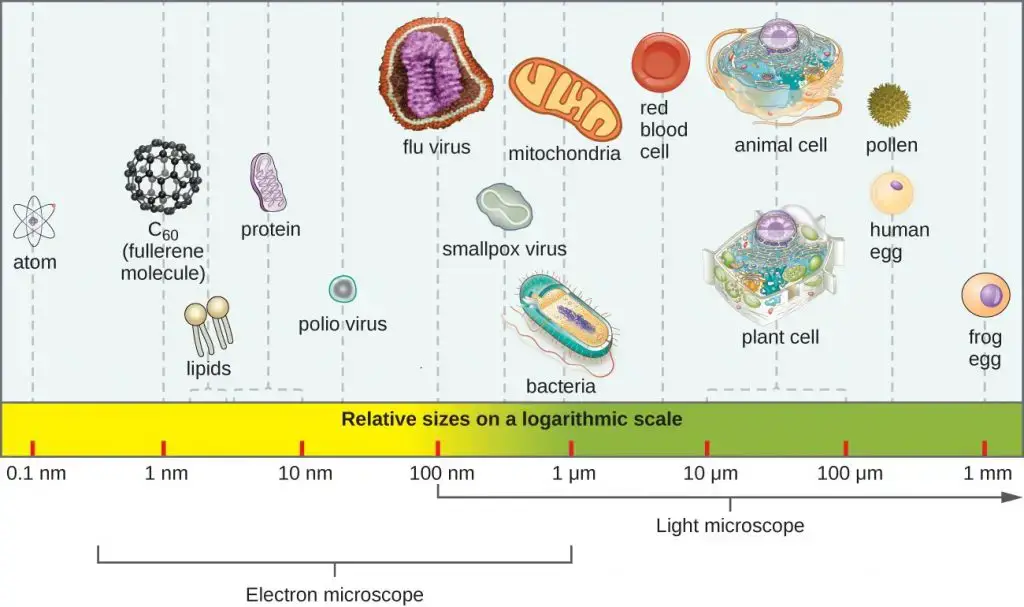
Habitat of Bacteria
Bacteria thrive in virtually every environment on Earth, from the most hospitable to the most extreme. They adapt to a wide variety of physical and chemical conditions, making them integral to ecological systems and biological processes.
- Terrestrial Habitats:
- Soil contains an enormous population of bacteria, with a single gram hosting about a billion cells.
- These organisms are critical for nutrient recycling and breaking down organic matter, supporting soil fertility and plant growth.
- Bacteria also play roles in detoxifying harmful substances, maintaining ecological balance.
- Aquatic Environments:
- Oceans and seas are home to approximately 3×10263 \times 10^{26}3×1026 bacteria, contributing up to 50% of the oxygen in Earth’s atmosphere.
- Freshwater lakes, geothermal springs, and arctic ice harbor diverse bacterial populations, some adapted to extreme temperatures or chemical compositions.
- In deep-sea ecosystems, bacteria convert hydrogen sulfide and methane into energy, sustaining life in environments devoid of sunlight.
- Atmospheric Presence:
- Airborne bacteria are surprisingly abundant, with a cubic meter of air containing around 100 million cells.
- Their presence in the atmosphere influences processes like cloud formation and weather patterns.
- Symbiotic Relationships:
- Bacteria inhabit the surfaces and interiors of plants and animals, often forming mutualistic or commensal associations.
- These relationships are essential for processes like digestion, nitrogen fixation in plants, and disease resistance.
- Extreme Environments:
- Cold Environments: Cryptoendoliths survive at subzero temperatures in Antarctica, embedded within rock formations.
- High Temperatures: Species like Thermus aquaticus thrive in geysers at 70–100 °C, where enzymes like Taq polymerase are derived for scientific applications.
- High Radiation: Deinococcus radiodurans withstands radiation doses of up to 5 MRad.
- Saline Conditions: Some bacteria endure hypersaline environments, such as the Dead Sea and Great Salt Lake, where salt concentrations exceed 47%.
- Acidic and Alkaline Conditions: Certain species adapt to acidic pH levels as low as 3, while betaproteobacteria tolerate alkaline conditions at pH 12.8.
- Space: Bacillus subtilis demonstrated survival on a NASA satellite for six years, showcasing bacterial resilience to vacuum and radiation.
- Deep Underground: Bacteria have been discovered 3.2 kilometers below Earth’s surface, surviving in high-pressure, nutrient-scarce conditions.
- High Pressure: In the Mariana Trench, species like Moritella and Shewanella endure pressures exceeding 1200 atmospheres.
Movement of Bacteria
Bacteria have evolved various methods for movement, enabling them to navigate different environments. Their motility mechanisms are essential for processes like finding food or avoiding harmful conditions. These mechanisms include flagellar motion, twitching, gliding, and even interactions with host cells.
- Flagella-based Movement
- Many bacteria move using flagella, which are long protein filaments that rotate to create propeller-like motion.
- Flagella are powered by a motor at the base, using the electrochemical gradient across the membrane for energy.
- The flagellum consists of about 20 proteins, with additional proteins required for its assembly and regulation.
- Flagella can be arranged in several ways:
- Monotrichous: A single flagellum at one end.
- Amphitrichous: A flagellum at each end.
- Lophotrichous: Clusters of flagella at one or both poles.
- Peritrichous: Flagella distributed over the entire surface.
- The spirochaetes group has flagella in the periplasmic space between membranes, contributing to their characteristic helical movement.
- Tumbling and Swimming
- Bacteria like E. coli use two primary types of movement: forward swimming and tumbling.
- Swimming occurs when flagella rotate in one direction, propelling the bacteria forward.
- Tumbling happens when flagella rotate in the opposite direction, causing a random reorientation of the bacterium.
- This alternating pattern of swimming and tumbling results in a three-dimensional, random walk, aiding in the bacteria’s search for favorable environments.
- Twitching Motility
- Gliding Motility
- Other bacteria glide across surfaces through mechanisms that remain less understood but do not rely on flagella or pili.
- Gliding enables bacteria to move smoothly over solid surfaces without the visible movement of appendages.
- Chemotaxis and Other Taxis
- Many bacteria respond to environmental cues in processes known as taxes, which include:
- Chemotaxis: Movement toward or away from chemicals.
- Phototaxis: Movement toward or away from light.
- Magnetotaxis: Movement in response to magnetic fields.
- These behaviors allow bacteria to move strategically in response to specific stimuli.
- Many bacteria respond to environmental cues in processes known as taxes, which include:
- Myxobacteria Behavior
- A unique group, myxobacteria, moves in coordinated waves on solid surfaces.
- As they move, they form fruiting bodies that contain spores, enabling them to survive harsh conditions.
- Intracellular Motility
- Some pathogenic bacteria, such as Listeria and Shigella, can move inside host cells by exploiting the host’s cytoskeleton.
- They promote actin polymerization at one end of their cell, forming a tail that propels them through the host’s cytoplasm.
Communication of Bacteria
Bacteria have evolved diverse methods to communicate with each other and coordinate behavior, a crucial aspect of their survival and interaction with the environment. This communication can involve chemical signals, light emission, and the creation of complex structures that allow for shared benefits and synchronized actions.
- Bioluminescence in Bacteria
- Some bacteria are capable of generating light through a chemical process called bioluminescence.
- This ability is often found in bacteria living in close association with fish and other larger animals.
- The light likely serves to attract these animals, which may provide a habitat or other benefits for the bacteria.
- Biofilm Formation
- Many bacteria form biofilms, multicellular structures where cells live in close proximity and exchange molecular signals.
- These biofilms enable bacteria to perform coordinated, collective actions, offering advantages like protection and resource sharing.
- Biofilm formation includes a cellular division of labor, where different bacterial cells perform specialized tasks that benefit the group.
- Within biofilms, bacteria can access resources that individual cells might not be able to use effectively.
- They also gain protection from external threats, including antagonistic microorganisms or environmental stresses.
- Bacteria in biofilms can exhibit up to 500 times more resistance to antibacterial agents than individual, free-living cells (planktonic bacteria) of the same species.
- Quorum Sensing
- Quorum sensing is a critical form of intercellular communication in bacteria, allowing them to sense population density and coordinate behavior accordingly.
- As bacterial populations grow, they release signaling molecules known as autoinducers or pheromones.
- These molecules accumulate as the population increases, signaling the bacteria to change gene expression in response.
- Quorum sensing ensures that certain processes, like digestive enzyme secretion or bioluminescence, are only triggered when a sufficient number of bacteria are present to make the process effective.
- Benefits of Coordinated Behavior
- Through quorum sensing and biofilm formation, bacteria can optimize survival and enhance their capabilities in complex environments.
- The ability to cooperate in large groups allows bacteria to perform tasks more efficiently, such as defending against antibiotics or competing for resources.
- Differentiating into distinct cell types within biofilms helps optimize the overall function and resilience of the bacterial community.
External Structure of a Bacterium
The external structures of bacteria serve critical functions, ranging from motility to protection and interaction with their environment. These components, located outside the cell wall, vary in form and function depending on the bacterial species.
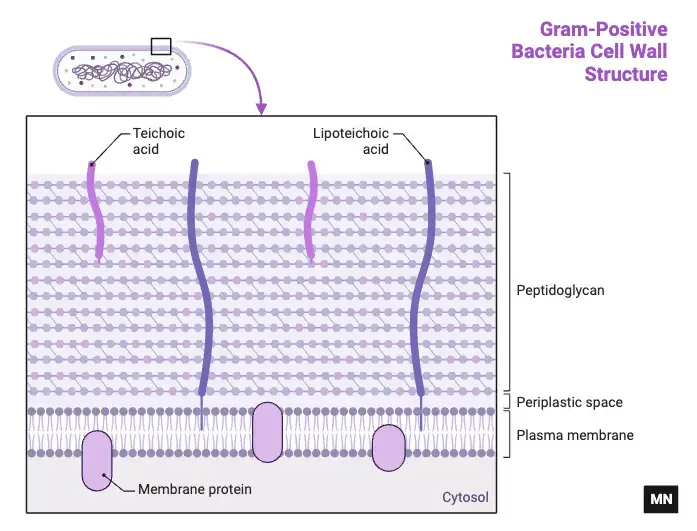
- Flagella:
- These are long, filamentous structures, typically 4–5 μm in length and 0.01–0.03 μm in diameter, responsible for bacterial motility.
- The structure consists of three parts:
- Filament: The outermost, threadlike component made of the protein flagellin.
- Hook: A short, curved segment connecting the filament to the basal body, facilitating rotary motion.
- Basal Body: A complex of rings embedded in the cell wall and plasma membrane. Gram-positive bacteria possess one pair of rings, while Gram-negative bacteria have two. This structure generates energy for flagellar motion and polymerizes the flagellum.
- Functions: Movement, chemotaxis, and contributions to pathogenicity.
- Pili/Fimbriae:
- These are short, straight, non-helical filaments approximately 0.5 μm long and 0.01 μm wide, composed of the protein pilin.
- Found primarily in Gram-negative bacteria, they are more numerous and shorter than flagella.
- Sex Pili: Larger structures (10–20 μm long) that facilitate DNA transfer during bacterial conjugation, usually present in small numbers (1–4).
- Functions: Attachment to host cells and surfaces, and in the case of sex pili, genetic exchange.
- Capsule:
- A viscous, outermost layer surrounding the cell wall, composed mainly of polysaccharides or polypeptides with about 98% water.
- Capsules can be classified into macro-capsules (>0.2 μm thick) or micro-capsules (<0.2 μm thick).
- Some bacteria produce a slime layer, a loosely organized proteinaceous covering.
- Functions: Protection from desiccation, resistance to phagocytosis, adherence, and shielding from enzymes.
- Sheath and Prosthecae:
- Sheath: A hollow tube that encloses chain-forming aquatic bacteria, providing mechanical support.
- Prosthecae: Semi-rigid extensions of the cell wall and plasma membrane that enhance nutrient absorption and assist in adhesion.
- Cell Wall:
- A rigid external structure made primarily of peptidoglycan, measuring 10–25 μm in thickness. It serves as a protective barrier and maintains cellular shape.
- Gram-Positive Cell Wall:
- Thick layers of peptidoglycan (40–90%), containing teichoic acids, teichuronic acids, and neutral sugars.
- Surface teichoic acids function as antigens and contribute to cell integrity.
- Stains purple with crystal violet during Gram staining due to the high peptidoglycan content.
- Gram-Negative Cell Wall:
- A thinner layer of peptidoglycan with additional complexity from lipoproteins, an outer membrane, and lipopolysaccharides (LPS).
- LPS Structure: Includes Lipid-A (toxic component), a core oligosaccharide, and O-polysaccharide (antigenic variability).
- Stains pink with safranin during Gram staining due to lower peptidoglycan levels.
- Acid-Fast Cell Wall: Contains large quantities of mycolic acids, giving resistance to acid-alcohol decolorization.
- Wall-Less Bacteria: Mycoplasma lacks a cell wall, resulting in a flexible and variable shape. Other forms include protoplasts, spheroplasts, and L-forms.
- Gram-Positive vs. Gram-Negative Cell Walls:
- Thickness: Gram-positive (20–80 nm) is thicker than Gram-negative (10–15 nm).
- Peptidoglycan Content: Higher in Gram-positive bacteria.
- Lipid Content: Low in Gram-positive (2–5%) and higher in Gram-negative (15–20%).
- Components: Gram-positive walls have teichoic and teichuronic acids, while Gram-negative walls contain lipoproteins, lipopolysaccharides, and an outer membrane.
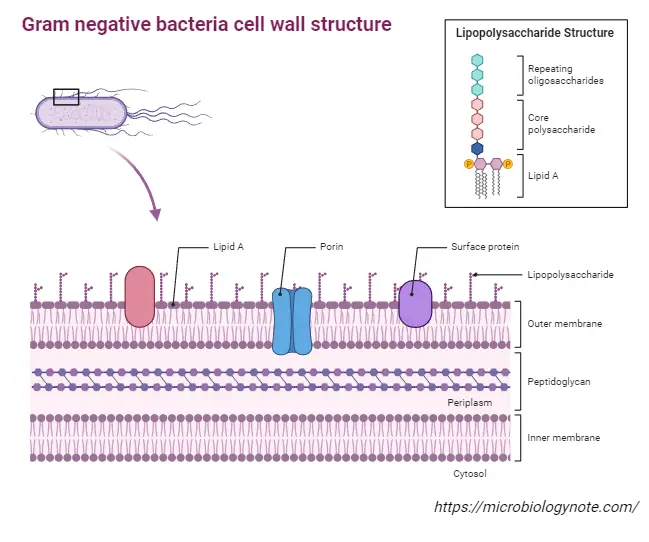
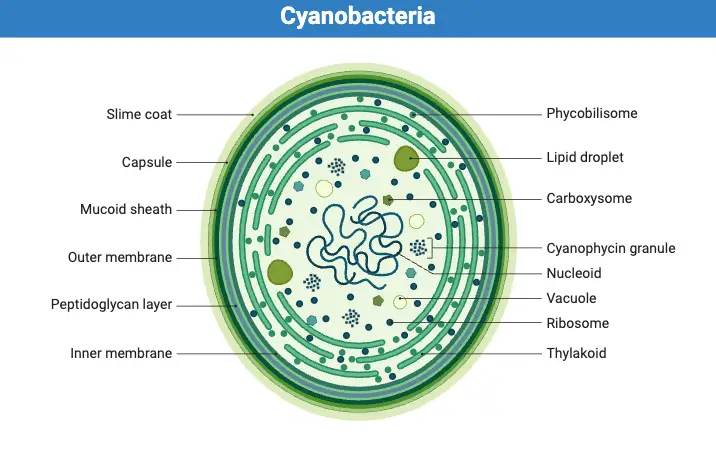
Internal Structure of Bacteria
The internal components of bacteria include the plasma membrane and the structures enclosed within it. These elements support essential cellular functions and ensure the organism’s survival and adaptability in various environments.
Key Internal Structures
- Cell Membrane (Plasma Membrane):
- The innermost layer beneath the cell wall, composed of a phospholipid bilayer, approximately 5–10 nm thick.
- Unlike eukaryotic membranes, bacterial plasma membranes lack sterols, except in Mycoplasma. Instead, they contain sterol-like molecules called hapanoids for stability.
- Fatty acid composition includes saturated, unsaturated, and modified variants (e.g., methyl, hydroxyl, or cyclic groups).
- Embedded porin proteins facilitate the passive transport of nutrients and ions.
- Functions:
- Regulates nutrient and ion exchange through selective permeability.
- Serves as a site for electron transport and oxidative phosphorylation.
- Cytoplasm:
- A viscous, colloidal substance containing dissolved organic and inorganic molecules.
- Unlike eukaryotes, bacteria lack membrane-bound organelles. Key components include ribosomes, mesosomes, inclusion bodies, and nucleic acids.
- Ribosomes:
- Composed of 70S ribosomes, smaller than eukaryotic 80S types, with two subunits: 50S and 30S.
- Function as protein and enzyme synthesis sites.
- Targeted by antibiotics like erythromycin, macrolides, and aminoglycosides.
- Mesosomes:
- Folded or vesicular structures formed by the invagination of the plasma membrane.
- Functionally analogous to mitochondria, providing a site for respiratory enzymes.
- Inclusion Bodies:
- Serve as storage sites for nutrients.
- Types:
- Organic inclusion bodies: Contain glycogen or polyhydroxybutyrate granules.
- Inorganic inclusion bodies: Contain polyphosphate or sulfur granules.
- Bacterial Nucleus (Nucleoid):
- A region containing double-stranded DNA (dsDNA), either circular or coiled.
- Lacks a nuclear membrane, nucleolus, and nucleoplasm.
- Contains chromosomal DNA in the nucleoid region and plasmids as extrachromosomal DNA.
- Endospores:
- Formed by certain bacteria during adverse conditions as a survival strategy.
- Transition into vegetative cells under favorable conditions.
- Structure:
- Core: Contains condensed cytoplasm and the nucleoid.
- Spore wall: Innermost layer, composed of peptidoglycan.
- Cortex: Thick layer with unique peptidoglycan.
- Protein coat: Outermost protective layer made of keratin-like protein, ensuring impermeability.
The internal structure of bacteria reflects a highly efficient and adaptive design, essential for survival in diverse and often hostile environments.
Interactions of Bacteria with Other Organisms
Bacteria interact with a wide range of organisms, forming a variety of relationships that can benefit or harm the involved species. These interactions can be categorized into mutualism, commensalism, and parasitism, each playing a distinct role in ecological and biological processes.
- Commensalism
- Commensal bacteria live on plants and animals without causing harm.
- In humans, these bacteria colonize areas like the skin, airways, and the gut, where they are known as normal flora.
- Commensals can sometimes invade other parts of the body and cause infections. For example, Escherichia coli, typically harmless in the gut, can lead to urinary tract infections.
- Similarly, streptococci, which are common in the mouth, can cause heart disease under certain conditions.
- Predation
- Some bacteria, known as predatory bacteria, actively kill and consume other microorganisms.
- Bacteria such as Myxococcus xanthus form swarms that attack and digest other bacteria.
- Other predators may attach to their prey and absorb nutrients or invade host cells to reproduce inside them.
- These predatory bacteria evolved from saprophages, which initially consumed decaying organisms, later adapting to capture and kill living microorganisms.
- Mutualism
- Mutualistic relationships are crucial for the survival of certain bacteria, involving intimate partnerships that provide benefits to both organisms.
- In interspecies hydrogen transfer, anaerobic bacteria produce hydrogen as a byproduct while consuming organic acids like butyric or propionic acid. This hydrogen is used by methanogenic archaea, which help maintain low hydrogen levels, allowing the bacteria to continue growing.
- Another example is the nitrogen-fixing bacteria found in the rhizosphere of plants. These bacteria convert nitrogen gas into nitrogenous compounds, making nitrogen available to plants that cannot fix it themselves.
- The human gut also hosts a variety of bacteria that contribute to health by synthesizing essential vitamins like folic acid, vitamin K, and biotin, and fermenting undigestible carbohydrates. These bacteria are vital for gut immunity and inhibit the growth of harmful pathogens through competitive exclusion.
- Vitamin B12 Synthesis
- Vitamin B12 is essential for many organisms, but only bacteria and some archaea have the enzymes necessary to synthesize it.
- This vitamin plays a key role in DNA synthesis and cellular metabolism, especially in the nervous system by aiding myelin production.
- Many animals rely on bacteria to obtain vitamin B12 through the food chain, demonstrating how bacteria support broader biological functions.
- Pathogenesis
- When bacteria engage in parasitic interactions, they are classified as pathogens, which can cause significant diseases in humans and animals.
- Pathogenic bacteria can lead to infections such as tetanus, cholera, typhoid fever, syphilis, and tuberculosis, among others.
- Some bacteria, such as Staphylococcus and Streptococcus, normally exist as part of the human flora but can cause infections when the body’s defense mechanisms are compromised.
- Obligate intracellular parasites, like Rickettsia and Chlamydia, depend entirely on host cells for reproduction and cause diseases such as typhus and pneumonia.
- Bacterial Toxins
- Pathogenic bacteria can produce toxins, either endotoxins (released from broken bacterial cells) or exotoxins (secreted by live bacteria).
- Clostridium botulinum, for example, produces an exotoxin that causes respiratory paralysis.
- Some exotoxins can be inactivated and used to make toxoid vaccines to prevent diseases caused by these toxins.
- Antibiotic Resistance and Treatment
- Antibiotics are used to treat bacterial infections. They can be bacteriocidal (kill bacteria) or bacteriostatic (prevent bacterial growth).
- Antibiotics target specific bacterial processes, such as protein synthesis. For instance, chloramphenicol and puromycin inhibit bacterial ribosomes but not those of eukaryotic cells.
- However, widespread antibiotic use in human medicine and agriculture has led to the development of antibiotic-resistant bacteria, which complicates treatment efforts.
- Preventive measures, such as sterilization of surgical instruments and the use of antiseptics, are critical to reduce bacterial infections.
Shapes and Arrangement of Bacteria
Bacteria are classified into distinct shapes that impact their structure, function, and interaction with the environment. These shapes can further determine how bacteria group together, leading to a variety of arrangements.
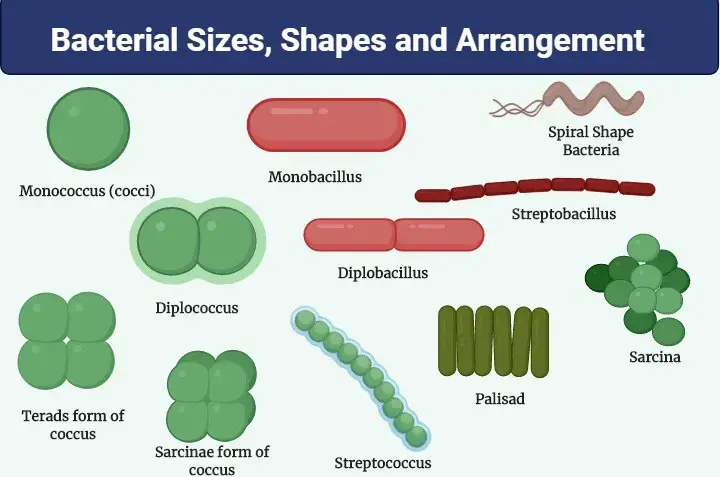
Primary Bacterial Shapes
- Cocci (Spherical):
- Cocci are round or oval bacteria, and their arrangement depends on how they divide and the plane in which they do so.
- Monococci: Single spherical bacteria, e.g., Micrococcus luteus.
- Diplococci: Pair of cocci arranged side by side, e.g., Neisseria spp., Moraxella catarrhalis.
- Streptococci: Cocci form long chains, e.g., Streptococcus pyogenes.
- Staphylococci: Irregular clusters resembling a bunch of grapes, e.g., Staphylococcus aureus.
- Tetrad: A group of four cocci, e.g., Aerococcus urinae.
- Sarcinae: Groups of eight cocci, e.g., Sarcina spp., Clostridium maximum.
- Bacilli (Rod-shaped):
- Bacilli are elongated, rod-like bacteria, and their arrangement reflects how the cells divide.
- Bacillus/Mono–bacillus: Single rod-shaped bacteria, e.g., Salmonella enterica, Bacillus cereus.
- Diplobacilli: A pair of rod-shaped bacteria, e.g., Moraxella bovis, Bacillus licheniformis.
- Streptobacilli: Bacilli arranged in long chains, e.g., Streptobacillus moniliformis.
- Palisade: Rods form a fence-like arrangement, e.g., Corynebacterium diphtheriae.
- Coccobacilli: Short, rounded rods, e.g., Chlamydia spp., H. influenzae.
- Spiral:
- These bacteria have a helical or twisted shape, contributing to their motility and ability to navigate through fluids.
- Spirilla: Long, rigid, spiral-shaped bacteria, e.g., Spirilla spp..
- Spirochetes: Flexible, corkscrew-shaped bacteria, e.g., Spirochetes spp..
- Comma-shaped:
- Bacteria with a curved, comma-like shape.
- Vibrio: Characterized by a comma or curved rod shape, e.g., Vibrio spp..
Other Uncommon Shapes
- Filamentous: Long, thread-like bacteria, e.g., Actinobacteria, Candidatus savagella.
- Star-shaped: Bacteria forming a star-like structure, e.g., Stella vacuolata.
- Appendaged/Budding: Bacteria that form appendages or buds, e.g., Hypomicrobium, Rhodomicrobium.
- Pleomorphic: Bacteria that vary in shape, e.g., Mycoplasma spp..
- Chinese letter-like: Bacteria arranged in a characteristic pattern resembling Chinese letters, e.g., Corynebacterium spp..
- Lobed: Bacteria with a lobed shape, e.g., Sulfolobus spp..
- Stalked: Bacteria with a stalk-like structure, e.g., Caulobacter crescentus.
- Sheathed: Bacteria surrounded by a protective sheath, e.g., Leptothrix, Clonothrix.
Classification of Bacteria
Bacteria can be classified using various schemes based on structural, functional, and environmental factors. These schemes provide insight into the diversity of bacterial life and allow researchers to better understand bacterial behavior, identification, and treatment strategies. The primary classification methods are based on Gram staining, oxygen requirements, temperature preferences, flagella arrangements, and nutritional modes.
1. Classification Based on Gram Staining
This method divides bacteria into two broad categories, based on their cell wall structure.
- Gram-Positive Bacteria
- Retain the crystal violet dye during Gram staining, appearing purple.
- Have a thick peptidoglycan layer in their cell walls.
- Examples: Staphylococcus, Streptococcus, Enterococcus, Corynebacterium.
- Gram-Negative Bacteria
- Lose the crystal violet dye but retain the red/pink color from safranine counterstaining.
- Possess a thin peptidoglycan layer, often surrounded by an outer membrane.
- Examples: Escherichia, Salmonella, Shigella, Neisseria.
Key Differences:
- Gram-Positive Bacteria
- Gram-Negative Bacteria
- Thin peptidoglycan, outer membrane, fimbriae or pili.
- Exospores form, endotoxins produced, no teichoic acid.
2. Classification Based on Oxygen Requirements
Bacteria can also be categorized by how they use or tolerate oxygen.
- Aerobic Bacteria
- Require oxygen for respiration and cannot survive in anoxic environments.
- Examples: Pseudomonas aeruginosa, Mycobacterium tuberculosis.
- Facultative Anaerobes
- Can survive in both oxygen-rich and oxygen-poor environments, including microaerophilic conditions.
- Examples: Escherichia coli, Klebsiella pneumoniae.
- Anaerobic Bacteria
- Only respire anaerobically and cannot survive in oxygenated conditions.
- Examples: Clostridium perfringens, Bacteroides.
3. Classification Based on Temperature Preferences
Temperature tolerance is another distinguishing feature in bacterial classification.
- Psychrophiles
- Thrive at temperatures below 15°C.
- Examples: Chryseobacterium, Polaromonas.
- Mesophiles
- Grow best between 15°C and 45°C, most human pathogens fall into this category.
- Examples: Escherichia coli, Staphylococcus aureus.
- Thermophiles
- Prefer temperatures above 45°C, often found in hot environments.
- Examples: Bacillus thermophilus, Pyrococcus.
4. Classification Based on Flagella Arrangement
Flagella arrangement is used to identify bacteria that possess them, determining their motility and movement.
- Atrichous
- Bacteria without flagella.
- Examples: Staphylococcus, Streptococcus.
- Monotrichous
- Bacteria with a single flagellum located at one pole.
- Examples: Campylobacter, Vibrio cholerae.
- Lophotrichous
- Bacteria with multiple flagella at one end.
- Examples: Helicobacter pylori, Pseudomonas fluorescens.
- Peritrichous
- Bacteria with flagella distributed all around the surface.
- Examples: Escherichia coli, Salmonella Typhi.
- Amphitrichous
- Bacteria with flagella at both poles.
- Examples: Alcaligenes faecalis, Nitrosomonas.
5. Classification Based on Mode of Nutrition
Bacteria can also be classified by how they acquire energy and carbon.
- Autotrophic Bacteria
- Can synthesize their own organic compounds from inorganic materials.
- Photoautotrophs: Use sunlight for energy (e.g., Cyanobacteria).
- Chemoautotrophs: Use chemicals for energy (e.g., Thiobacillus, Methanobacterium).
- Heterotrophic Bacteria
- Obtain energy by consuming organic compounds.
- Examples: Escherichia coli, Staphylococcus aureus.
- Saprophytic Bacteria
- Break down dead organic material to derive energy.
- Examples: Clostridium thermosaccharolyticum, Pseudomonas denitrificans.
Reproduction in Bacteria
Bacteria reproduce rapidly, often with impressive efficiency. Their methods of reproduction are primarily asexual, though there are some mechanisms that resemble sexual reproduction. The processes can be broken down into the following categories:
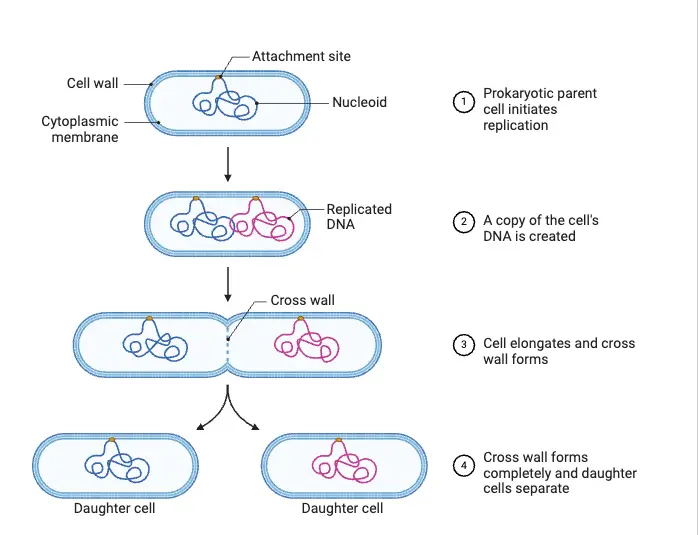
- Binary Fission
- Most common form of reproduction
- A single bacterium divides into two identical daughter cells under optimal conditions.
- Steps:
- The bacterium absorbs nutrients and grows to its maximum size.
- DNA replication occurs, producing two copies of the genetic material.
- The two DNA molecules migrate to opposite poles of the cell.
- A transverse septum forms, splitting the cell and creating two daughter cells.
- Conidia Formation
- Seen in filamentous bacteria, especially within actinomycetes (e.g., Streptomyces, Micromonospora, Rhodomicrobium).
- A form of asexual reproduction that involves the formation of conidia, which are reproductive spores that eventually grow into new bacterial cells.
- Budding
- Occurs when a small protuberance or “bud” forms on the side of the bacterium.
- DNA replicates, and one copy enters the bud.
- The bud separates and grows into a daughter cell, seen in species such as Planctomyces, Rhodomicrobium vannielia, and Hyphomicrobium.
- Endospore Formation
- A survival mechanism in response to harsh environmental conditions.
- Seen in certain Gram-positive bacteria like Bacillus, Clostridium, and Sporosarcina.
- The bacterium’s DNA is surrounded by a thick, resistant wall, forming a structure known as an endospore. This protects the genetic material until conditions improve.
- Transformation
- A form of genetic exchange that involves the uptake of foreign DNA.
- DNA from one bacterium enters another bacterium of the same species, leading to genetic recombination.
- The DNA enters the cell through the extracellular environment, modifying the recipient bacterium’s genetic makeup.
- Conjugation
- Involves direct cell-to-cell contact, where DNA is transferred from a donor to a recipient.
- The donor cell develops a sex pilus, which attaches to the recipient cell, forming a conjugation tube.
- DNA fragments are transferred through this tube, resulting in genetic exchange.
- Transduction
- A mechanism of genetic transfer mediated by bacteriophages (viruses that infect bacteria).
- DNA is transferred from one bacterium to another via the bacteriophage, which inserts the donor’s DNA into the recipient cell.
Examples of Media Used for the Growth of Bacteria
Bacterial growth relies on various media types that provide essential nutrients or specific conditions to support different bacterial species. Below are the major categories of media used for bacterial growth, each designed to meet distinct needs.
Solid Media
- Nutrient Agar: This is a versatile medium that supports the growth of a wide range of bacteria, particularly non-fibrous species. It’s a common choice in many microbiology labs.
- MacConkey Agar: Selective for Gram-negative bacteria, this medium also differentiates lactose fermenters from non-fermenters. It produces a clear color distinction, with lactose fermenters turning pink.
- Blood Agar: An enriched medium, ideal for growing fastidious organisms. It’s also used to observe bacterial hemolytic activity, helping to identify bacteria based on their ability to lyse red blood cells.
- Chocolate Agar: This agar is specifically used for growing organisms that require extra nutrients, such as Neisseria gonorrhoeae, which thrive in this enriched environment due to the lysed red blood cells.
Semi-Solid Media
- Mannitol Motility Medium: Designed to test bacterial motility and the fermentation of mannitol. It helps identify bacteria capable of moving in a semi-solid medium.
- Hugh and Leifson’s Medium: This medium is useful for determining bacterial metabolic pathways, specifically oxidative versus fermentative metabolism.
- Stuart’s and Amies Media: Transport media used to maintain bacterial viability during transportation to labs. These media help preserve the bacteria until they can be cultured.
Liquid Media (Broth)
- Nutrient Broth: A general-purpose liquid medium that supports the growth of a broad range of bacterial species, often used in fermentation studies.
- Tryptic Soy Broth (TSB): A commonly used liquid medium that supports a wide variety of fastidious organisms, making it a reliable choice in laboratory experiments.
- MR-VP Broth: Used specifically for testing fermentation pathways in enteric bacteria, distinguishing between mixed acid fermentation and butanediol fermentation.
Based on Nutritional Content
- Basal Media
- Peptone Water: A simple medium providing basic nutrients for non-fastidious bacteria. It’s often used as a base in bacterial cultivation.
- Enriched Media
- Blood Agar: Contains whole red blood cells to enhance the growth of fastidious organisms, which require extra nutrients for optimal growth.
- Chocolate Agar: Features lysed red blood cells that make nutrients more available, catering to organisms with higher nutritional needs.
- Selective Media
- Bile Salt Agar: Selectively inhibits Gram-positive bacteria while allowing the growth of Gram-negative organisms like Salmonella and Shigella.
- Differential Media
- MacConkey Agar: Differentiates lactose fermenters from non-fermenters, with lactose fermenters producing pink colonies and non-fermenters resulting in colorless colonies.
Based on Oxygen Requirements
- Aerobic Media: Designed to support the growth of aerobic bacteria, these media include basic options like nutrient agar and broth.
- Anaerobic Media: These media cater to anaerobic bacteria and are often supplemented with reducing agents to create an oxygen-free environment, critical for cultivating anaerobes.
Bacterial Identification Methods
Bacterial identification is essential in clinical, environmental, and research settings. Methods are divided into phenotypic and genotypic categories, each offering distinct approaches to determine bacterial species.
Phenotypic Methods
- Gram Staining: This classic technique sorts bacteria into two groups based on their cell wall properties. Gram-positive bacteria stain purple, while Gram-negative bacteria turn pink, helping to narrow down species.
- Colony Morphology: The appearance of bacterial colonies on solid media provides immediate visual clues. Characteristics such as size, shape, color, and texture are observed to distinguish species.
- Biochemical Tests: Several tests assess bacterial metabolic processes. Key examples include:
- Catalase Test: Differentiates catalase-positive bacteria (e.g., Staphylococcus) from catalase-negative bacteria (e.g., Streptococcus).
- Oxidase Test: Identifies bacteria that produce cytochrome c oxidase.
- Fermentation Tests: Determines if bacteria can ferment specific sugars.
- Endospore Staining: Certain bacteria, like Bacillus and Clostridium, form endospores. This method highlights those species by identifying their endospore presence.
- MALDI-TOF Mass Spectrometry: This advanced method analyzes the protein profile of bacteria, enabling fast and accurate species identification compared to traditional methods.
- Automated Systems: Platforms like VITEK 2 and BIOLOG use biochemical tests in automated, high-throughput formats to identify bacteria based on metabolic patterns.
Genotypic Methods
- Polymerase Chain Reaction (PCR): PCR amplifies specific DNA sequences for quick bacterial identification. The 16S rRNA gene is often targeted because it is highly conserved among bacteria.
- Real-Time PCR: This technique builds on standard PCR by allowing the quantification of DNA as it’s amplified, improving speed and precision in identifying microorganisms.
- Microarray-Based Identification: In this method, amplified DNA hybridizes with species-specific probes on a microarray, enabling the simultaneous detection of multiple bacterial species.
- Sequencing Techniques:
- 16S rRNA Sequencing: The gold standard for bacterial identification, this method sequences the 16S ribosomal RNA gene, which is found in all bacteria.
- Internal Transcribed Spacer (ITS) Sequencing: Primarily used for fungi, this sequencing technique can also apply to bacteria in some cases.
- Ribotyping: Focuses on variations in rRNA genes, helping to classify and identify bacteria based on their genetic differences.
- Phage Typing: This method relies on bacteriophages to differentiate bacterial strains by their susceptibility patterns. It’s often used for tracing bacterial outbreaks in epidemiological studies.
- Serological Testing: This involves detecting specific antigens or antibodies to identify closely related bacterial strains. It’s particularly useful for distinguishing between similar species.
Bacterial Metabolism
Bacterial metabolism covers the biochemical processes that allow bacteria to extract energy and nutrients for growth and reproduction. Bacteria can utilize various pathways, depending on the environmental conditions and available resources.
Heterotrophic Metabolism
Heterotrophic bacteria obtain energy by oxidizing organic compounds. There are two primary processes involved in this metabolic mode:
- Respiration:
- Aerobic respiration occurs when oxygen serves as the terminal electron acceptor. This pathway fully oxidizes glucose, yielding up to 38 ATP molecules per glucose. It is one of the most efficient forms of energy production.
- Anaerobic respiration takes place in the absence of oxygen. Other compounds, such as nitrate or sulfate, act as electron acceptors. This results in less energy production compared to aerobic respiration.
- Fermentation:
- This anaerobic process involves the partial breakdown of organic compounds like sugars, without the need for oxygen. While less efficient, fermentation yields 2 ATP molecules per glucose. The byproducts can include lactic acid or ethanol, which can help identify specific bacterial species.
Autotrophic Metabolism
Autotrophic bacteria synthesize their own organic compounds from inorganic sources. These bacteria can perform two distinct processes to obtain energy:
- Chemolithotrophy:
- These bacteria oxidize inorganic compounds like hydrogen sulfide, ammonia, or ferrous iron. This process does not require sunlight and is often seen in extremophiles or certain soil bacteria.
- Photosynthesis:
- Some bacteria, such as cyanobacteria, perform photosynthesis using light to convert carbon dioxide into glucose. This process can either be:
- Oxygenic: Produces oxygen as a byproduct.
- Anoxygenic: Does not produce oxygen.
- Some bacteria, such as cyanobacteria, perform photosynthesis using light to convert carbon dioxide into glucose. This process can either be:
Central Metabolic Pathways
Bacteria use several essential metabolic pathways to break down carbohydrates and other substrates:
- Glycolysis:
- The Embden-Meyerhof pathway is the primary glycolytic pathway. It converts glucose into pyruvate, producing small amounts of ATP and NADH.
- Krebs Cycle (Citric Acid Cycle):
- After glycolysis, pyruvate is further oxidized in the Krebs cycle, generating additional ATP and electron carriers like NADH and FADH2, which feed into the electron transport chain for more energy production.
- Pentose Phosphate Pathway:
- This alternative pathway provides reducing power in the form of NADPH and generates precursors for biosynthesis, contributing to overall cellular metabolism.
- Entner-Doudoroff Pathway:
- This pathway is an alternative to glycolysis used by some bacteria. It converts glucose into pyruvate but with fewer enzymatic steps involved.
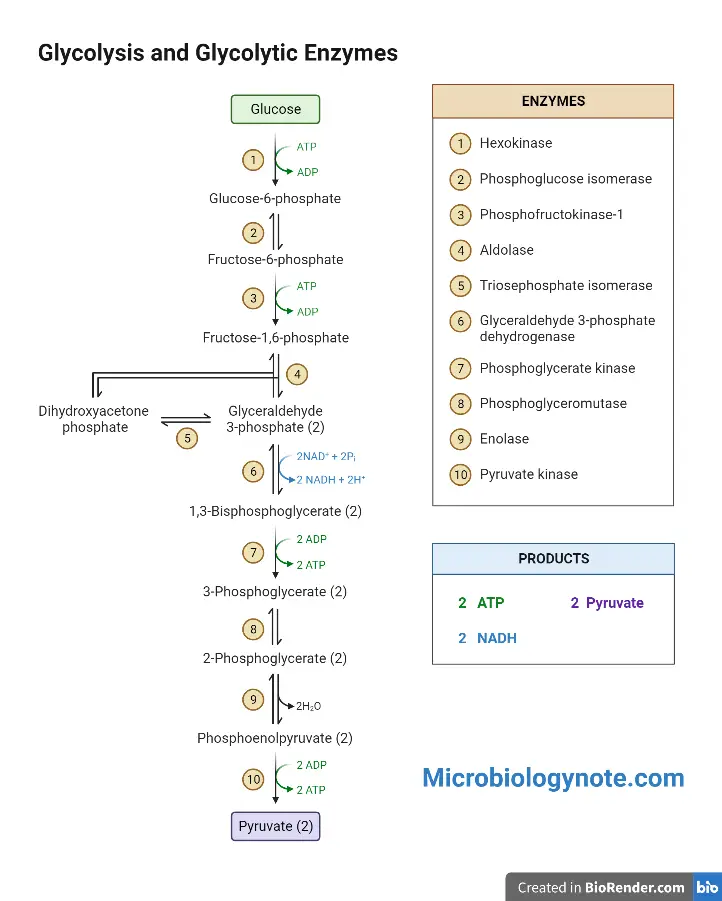
Catabolism of Other Biomolecules
Bacteria can also break down other types of biomolecules to generate energy:
- Lipid Catabolism:
- Triglycerides are broken down by lipases into fatty acids and glycerol. These products can then enter pathways like glycolysis or the Krebs cycle for further energy extraction.
- Protein Catabolism:
- Proteins are degraded into amino acids through the action of proteolytic enzymes. These amino acids can then be deaminated or converted into intermediates that feed into the central metabolic pathways.
Factors Affecting Bacterial Growth
Bacterial growth is influenced by various environmental factors. These factors dictate how well bacteria can survive, reproduce, and thrive. Here’s a breakdown of the key conditions that shape bacterial growth:
- Water Availability / Water Activity
- Bacteria need water to maintain osmotic pressure, support metabolic processes, and regulate their physiology and pH balance.
- A water activity level of 0.91 or above is typically required for optimal growth.
- Without sufficient water, bacterial metabolism and function are compromised.
- Nutritional Requirements
- Different bacteria have varying nutritional needs, with some requiring minimal nutrients while others have high demands.
- Fastidious bacteria are those with strict nutritional needs. They require specific growth factors or environmental conditions.
- Non-fastidious bacteria can grow under nutrient-limited conditions.
- Bacterial growth increases with higher nutrient concentrations but only up to a certain point. Beyond that, additional nutrients don’t necessarily enhance growth rates.
- Temperature
- Temperature plays a critical role in bacterial growth. Each species has an optimal temperature range.
- Bacteria are classified based on their temperature requirements:
- Mesophiles: Grow best around 37°C, which is the temperature of the human body. This group includes many pathogens.
- Thermophiles: Thrive in hotter conditions, often above 45°C.
- Psychrophiles: Adapt to cold environments, with optimal growth below 15°C.
- Gaseous Concentration
- The concentration of oxygen (O₂) and carbon dioxide (CO₂) can significantly impact bacterial growth.
- Strict aerobes require high O₂ levels for growth.
- Facultative aerobes can grow in low O₂ environments, utilizing oxygen when available but not requiring it.
- Anaerobes cannot survive in the presence of oxygen and rely on anaerobic conditions for growth.
- pH / Hydrogen Ion Concentration
- The pH level of the environment directly affects bacterial growth by influencing enzyme activity, protein structure, and membrane integrity.
- Most bacteria grow best in a neutral pH range of 6.5 to 7.5.
- Acidophiles thrive in acidic environments with pH values below 5.
- Alkaliphiles prefer environments with a pH above 9.
- Salinity
- Salt concentration impacts bacterial growth by affecting homeostasis and enzymatic activity.
- Halophiles are bacteria that require high salt concentrations for optimal growth, often found in saline environments like salt lakes or seawater.
- Light Intensity
- Some bacteria, specifically phototrophic bacteria, rely on light to produce food through photosynthesis. These bacteria thrive in environments where light is abundant.
Diseases Caused by Bacteria
Bacterial infections, caused by pathogenic bacteria, can lead to a wide range of diseases in humans. While most bacteria are harmless or even beneficial, a small percentage are responsible for various illnesses. To be classified as pathogenic, bacteria must meet Koch’s Postulates, which provide a framework for identifying disease-causing organisms.
Here are some common bacterial diseases and their causative agents:
- Tuberculosis
- Causative Bacteria: Mycobacterium tuberculosis
- Affects the lungs, causing chronic cough, chest pain, and difficulty breathing.
- Pneumonia
- Causative Bacteria: Klebsiella pneumoniae, Streptococcus pneumoniae, and others
- Leads to inflammation of the lungs, characterized by cough, fever, and difficulty breathing.
- Urinary Tract Infection (UTI)
- Causative Bacteria: E. coli, Klebsiella, Proteus, Staphylococcus, and others
- Results in pain during urination, frequent urges to urinate, and possible blood in the urine.
- Meningitis
- Causative Bacteria: Neisseria meningitidis, Streptococcus pneumoniae, and others
- Causes inflammation of the membranes surrounding the brain and spinal cord, leading to headache, fever, and stiff neck.
- Gastroenteritis
- Causative Bacteria: E. coli, Clostridium, Salmonella, Shigella, and others
- Leads to stomach cramps, diarrhea, vomiting, and fever.
- Typhus and Rocky Mountain Spotted Fever
- Typhoid Fever
- Causative Bacteria: Salmonella Typhi and Salmonella Paratyphi
- Symptoms include high fever, abdominal pain, and diarrhea.
- Cholera
- Causative Bacteria: Vibrio cholerae
- Characterized by severe diarrhea, vomiting, and dehydration, often from contaminated water.
- Tetanus
- Causative Bacteria: Clostridium tetani
- Causes muscle stiffness and spasms, commonly after a deep wound becomes infected.
- Syphilis
- Causative Bacteria: Treponema pallidum
- A sexually transmitted infection that causes sores, rashes, and potentially severe complications if untreated.
- Influenza
- Causative Bacteria: Haemophilus influenzae
- Often causes respiratory symptoms such as fever, cough, and fatigue.
- Cellulitis or Wound Infections
- Causative Bacteria: Staphylococcus aureus, Streptococcus pyogenes, and others
- Results in inflammation and infection of the skin and underlying tissue, often caused by bacteria entering through cuts or wounds.
Uses and Applications of Bacteria
Bacteria are far more than just disease-causing organisms. They are integral to numerous processes that benefit both the environment and human society. These microorganisms serve vital roles in nutrient recycling, biotechnological applications, food production, and even energy generation.
- Nutrient Recycling
- Bacteria play a crucial role in recycling essential nutrients such as nitrogen, carbon, sulfur, phosphorus, and oxygen.
- Their involvement in biogeochemical cycles, especially through assimilation and dissimilation of organic compounds, ensures the continuous availability of these elements in nature.
- Atmospheric Oxygen Regulation
- Photosynthetic bacteria, like Cyanobacteria and Green Sulfur Bacteria, contribute significantly to oxygen production during photosynthesis.
- This process supports life on Earth by helping regulate atmospheric oxygen levels.
- Biodegradation and Waste Management
- Bacteria are responsible for biodegradation, composting, decomposition, and bioremediation.
- They break down organic wastes and dead organisms, playing an essential role in managing and recycling waste in the environment.
- Industrial Enzyme Production
- Several bacterial species are used in the industrial production of enzymes.
- Bacteria produce enzymes like amylase, lipase, cellulases, proteases, and polymerases, which have applications in industries ranging from food processing to pharmaceuticals.
- Biotechnological Applications
- Genetically modified bacteria are employed in biotechnology to produce valuable substances such as hormones (e.g., insulin) and enzymes.
- Bacteria’s ability to work in controlled environments makes them ideal for producing biologically important molecules.
- Biogas Production
- Anaerobic bacteria are integral to the fermentation process that produces biogas (methane), which is used as an alternative energy source.
- This process helps in waste management and contributes to the generation of renewable energy.
- Antibiotic Production
- Genera like Actinomycetes are a primary source of antibiotics used in medicine.
- Bacteria have been harnessed in the pharmaceutical industry to develop life-saving antibiotics.
- Probiotics
- Certain bacterial species, including Bifidobacterium, E. coli, and Lactobacillus, are used as probiotics.
- These bacteria are beneficial to human health, helping with digestion and immune function.
- Fermented Food Production
- Bacteria play a vital role in the production of fermented foods such as dairy products, sausages, and fruit juices.
- Fermentation not only preserves food but also enhances its nutritional value and flavor.
- Bioremediation of Pollutants
- Bacteria are involved in the cleanup of environmental pollutants like oil spills, heavy metals, and toxic wastes.
- This bioremediation process helps to restore contaminated ecosystems.
- Genetic Engineering and Research
- Bacteria are essential tools in genetic engineering and molecular biology.
- Their genes are used to create genetically modified organisms (GMOs), pushing advancements in research and biotechnology.
- Bacterial Fuel Cells
- A novel technology is emerging where bacteria are used to convert chemical energy into electrical energy.
- This bacterial fuel cell concept presents a potential alternative energy source, offering eco-friendly energy production.
- Agriculture
- In agriculture, bacteria are used as bio-pesticides, bio-fertilizers, and bio-insecticides.
- They help improve crop yields and reduce the reliance on chemical pesticides.
- Pioneers of Life
- Bacteria were the first life forms on Earth and continue to thrive in harsh environments like deserts and rocks.
- All modern eukaryotic organisms evolved from ancient bacteria over two billion years ago.
- Normal Flora in Humans
- Bacteria also exist as part of the normal flora in the human body.
- They play a protective role by fighting off invading pathogens, supporting immune functions, and assisting in digestion.
Benefits of Bacteria
Bacteria, despite their negative reputation, offer a range of benefits across various sectors, including human health, the environment, and industrial applications.
- Health Benefits
- Digestive Health: Beneficial bacteria, particularly those found in the gut microbiome, assist in breaking down complex carbohydrates and synthesizing essential vitamins, aiding overall digestive processes.
- Immune System Support: Certain bacteria play a critical role in enhancing immune function. They help train the immune system to recognize and defend against harmful pathogens.
- Probiotics Production: Bacteria are used to produce probiotics, which are incorporated in supplements and fermented foods such as yogurt and kimchi. These probiotics can improve gut health, prevent antibiotic-associated diarrhea, and may assist in managing conditions like ulcerative colitis and infant colic.
- Infection Prevention: Beneficial bacteria compete with harmful pathogens for resources, producing substances that inhibit pathogen growth. This helps maintain skin health and prevents infections.
- Nutrient Absorption: The presence of bacteria in the gut enhances the absorption of nutrients, making the digestive system more efficient at utilizing energy from food.
- Environmental Benefits
- Nutrient Cycling: Bacteria decompose organic matter, recycling essential nutrients back into the ecosystem. This is vital for maintaining soil health and promoting plant growth.
- Bioremediation: Certain bacterial species break down environmental pollutants, providing valuable assistance in cleaning contaminated sites through bioremediation.
- Nitrogen Fixation: Some bacteria convert atmospheric nitrogen into forms that plants can use, contributing to agricultural productivity and ecosystem stability.
- Industrial Applications
- Food Production: Bacteria are central to the fermentation process, which is used to produce a variety of food and beverage items like cheese, yogurt, sauerkraut, and beer. This not only enhances flavor but also extends the shelf life of these products.
- Biotechnology: Bacteria are utilized in biotechnology to produce valuable products like enzymes, antibiotics, and other pharmaceuticals, which have significant applications in the medical field.
Disadvantages of Bacteria
While bacteria are crucial in various ecological and industrial processes, they also present several challenges. These microorganisms, particularly pathogenic types, can cause harm to both human health and the economy.
- Human Diseases
- Pathogenic bacteria are responsible for a broad range of diseases, from mild to life-threatening.
- Every year, bacterial infections contribute to thousands of deaths worldwide.
- Food and Pharmaceutical Spoilage
- Bacteria are a major cause of spoilage in food and pharmaceutical products.
- The food and pharmaceutical industries incur substantial financial losses due to bacterial contamination, affecting product safety and quality.
- Soil Fertility Decline
- Certain bacteria, such as denitrifying bacteria and sulfur-oxidizing bacteria, can degrade soil fertility.
- This reduction in soil quality impacts crop yields and the overall productivity of agricultural land.
- Damage to Crops and Animals
- Bacteria also pose a threat to crop plants and domestic animals, leading to diseases that diminish agricultural output.
- These bacterial infections can result in significant economic losses for farmers and the agricultural industry.
- Degradation of Organic Products
- Bacteria contribute to the deterioration of valuable organic products like furniture and textiles.
- Over time, bacterial activity causes wear and tear, reducing the lifespan of these goods.
Example of Bacteria
Bacteria serve different functions, with some contributing positively to health and the environment, while others can be harmful. Here’s a breakdown of each.
Beneficial Bacteria
- Lactobacillus: A well-known genus found in fermented dairy products like yogurt. Lactobacillus acidophilus helps with digestion and supports gut health by preventing harmful bacteria from growing.
- Bifidobacterium: These bacteria are prevalent in the gut microbiome, supporting digestion and protecting against harmful pathogens. Bifidobacterium bifidum may also help alleviate symptoms of irritable bowel syndrome (IBS).
- Escherichia coli (E. coli): Though some strains are harmful, many are beneficial. These strains assist in food digestion and nutrient absorption while preventing harmful bacteria from colonizing the gut.
- Streptomyces: These soil bacteria are key players in antibiotic production. They also decompose organic matter, contributing to nutrient cycling in the environment.
- Rhizobacteria: Found in soil, these bacteria enhance plant growth by fixing nitrogen, a critical nutrient for plants.
Harmful Bacteria
- Salmonella: A genus of bacteria that causes foodborne illnesses. Infection typically leads to symptoms like diarrhea, fever, and stomach cramps, often from consuming contaminated food or water.
- Escherichia coli (pathogenic strains): While many strains of E. coli are harmless, certain pathogenic strains like E. coli O157:H7 cause severe food poisoning, often linked to undercooked meat or contaminated produce.
- Staphylococcus aureus: Known for causing skin infections, food poisoning, and toxic shock syndrome. It produces harmful toxins that lead to illness if ingested.
- Clostridium difficile (C. diff): This bacterium causes severe diarrhea and colitis, particularly following antibiotic treatments that disrupt the balance of gut bacteria.
- Mycobacterium tuberculosis: The bacterium responsible for tuberculosis (TB), primarily affecting the lungs but also capable of impacting other body systems.
Economic Importance of Bacteria
Bacteria are not only essential for maintaining ecological balance, but they also play a pivotal role in many industries. Their ability to assist in processes like nitrogen fixation, fermentation, and biodegradation makes them invaluable across agriculture, food production, biotechnology, and environmental management. Below are the key ways bacteria contribute to various sectors:
- Agriculture
- Nitrogen Fixation: Some bacteria, including Rhizobium, reside in legume root nodules and convert atmospheric nitrogen into a usable form for plants. This reduces the dependency on synthetic fertilizers, resulting in cost savings and less environmental harm.
- Soil Health: By decomposing organic matter, bacteria recycle nutrients, improving soil fertility and supporting plant growth.
- Food Industry
- Fermentation: Various bacterial species are integral to the production of fermented foods, including:
- Lactobacillus: Used in the creation of yogurt, cheese, and other dairy products.
- Other bacteria play roles in fermenting bread (like sourdough) and beverages such as beer and vinegar.
- Preservation: The fermentation process helps preserve food by producing organic acids that prevent spoilage.
- Fermentation: Various bacterial species are integral to the production of fermented foods, including:
- Biotechnology
- Genetic Engineering: Escherichia coli and other bacteria are central to recombinant DNA technology, enabling the production of pharmaceuticals such as insulin and human growth hormone.
- Bioprocessing: Bacteria are used to produce enzymes that have various applications, including in detergent manufacturing, food processing, and biofuel production.
- Waste Treatment
- Biodegradation: Bacteria break down organic waste in sewage treatment plants, converting it into simpler substances that help mitigate environmental pollution.
- Oil Spill Cleanup: Certain bacteria can degrade the hydrocarbons found in oil spills, supporting environmental recovery efforts.
- Chemical Production
- Bioleaching: Bacteria can extract valuable metals from low-grade ores, offering a more eco-friendly alternative to conventional mining.
- Organic Compound Production: Bacteria are used to produce chemicals like ethanol, acetone, and organic acids, which are essential for various industrial processes.
- Pharmaceuticals
- Antibiotics Production: Many antibiotics, such as penicillin, streptomycin, and amoxicillin, are derived from bacteria, which have revolutionized medicine.
- Vaccines: Bacteria play a role in the development of vaccines for several infectious diseases.
- Jurtshuk P Jr. Bacterial Metabolism. In: Baron S, editor. Medical Microbiology. 4th ed. Galveston (TX): University of Texas Medical Branch at Galveston; 1996. Chapter 4. PMID: 21413278.
- Woese CR, Fox GE. Phylogenetic structure of the prokaryotic domain: the primary kingdoms. Proc Natl Acad Sci U S A. 1977 Nov;74(11):5088-90. doi: 10.1073/pnas.74.11.5088. PMID: 270744; PMCID: PMC432104.
- Jurtshuk P Jr.. Bacterial Metabolism. In: Baron S, editor. Medical Microbiology. 4th edition. Galveston (TX): University of Texas Medical Branch at Galveston; 1996. Chapter 4. Available from: https://www.ncbi.nlm.nih.gov/books/NBK7919/
- https://www.pnas.org/doi/full/10.1073/pnas.74.11.5088
- https://www.ncimb.com/microbial-identification/identification-methods-for-bacteria-and-fungi/
- https://www.britannica.com/science/bacteria/Bacterial-metabolism
- https://bio.libretexts.org/Bookshelves/Microbiology/Microbiology_(OpenStax)/08:Microbial_Metabolism/8.E:_Microbial_Metabolism(Exercises)
- https://bio.libretexts.org/Courses/North_Carolina_State_University/MB352_General_Microbiology_Laboratory_2021_(Lee)/08:_Bacterial_Identification/8.03:_Introduction_to_Bacterial_Identification_using_Genotypic_methods
- https://bitesizebio.com/36644/methods-microbial-identification/
- https://www.ncimb.com/microbial-identification/identification-methods-for-bacteria-and-fungi/
- https://www.cd-genomics.com/microbioseq/methods-for-microbial-detection-and-identification.html
- https://byjus.com/biology/classification-of-culture-media/
- https://www.ramauniversity.ac.in/online-study-material/pharmacy/bpharma/iiisemester/pharmaceuticalmicrobiology/lecture-5.pdf
- https://conductscience.com/culture-media/
- https://www.sigmaaldrich.com/IN/en/technical-documents/technical-article/microbiological-testing/microbial-culture-media-preparation/types-of-media-in-microbiology
- https://www.microrao.com/micronotes/culture_media.pdf
- https://www.the-scientist.com/exploring-the-landscape-of-bacterial-culture-media-72052
- https://www.sigmaaldrich.com/IN/en/technical-documents/technical-article/microbiological-testing/microbial-culture-media-preparation/types-of-media-in-microbiology
- https://en.wikipedia.org/wiki/Culture_media
- https://en.wikipedia.org/wiki/Bacteria
- https://byjus.com/biology/bacteria/