What is Vitamin C (Ascorbic Acid)?
- Vitamin C, scientifically termed as ascorbic acid, is a vital water-soluble nutrient predominantly found in citrus fruits, vegetables, and certain dietary supplements. It plays a pivotal role in various physiological processes, including tissue repair, collagen synthesis, and the enzymatic production of specific neurotransmitters. Collagen, a crucial protein, relies on Vitamin C for its formation, making the vitamin indispensable for wound healing and maintaining the integrity of skin, blood vessels, and bones.
- One of the remarkable properties of Vitamin C is its function as an antioxidant. Antioxidants are compounds that neutralize free radicals, which are harmful molecules that can damage cells and contribute to aging and diseases. In its role as a reducing agent, Vitamin C donates electrons to various enzymatic and non-enzymatic reactions, converting it to an oxidized state. This oxidized form can be reverted to its reduced state through specific enzymatic mechanisms, ensuring a balance in the body.
- Furthermore, Vitamin C is essential for the proper functioning of several enzymes and is crucial for the immune system’s optimal performance. It is noteworthy that while most animals can synthesize Vitamin C internally, certain species, including humans, must obtain it from dietary sources. This makes the regular intake of Vitamin C-containing foods, such as citrus fruits, kiwifruit, broccoli, and strawberries, vital for health.
- There is ongoing research on the potential benefits of Vitamin C supplementation. Some studies suggest that it may reduce the duration of common cold symptoms, although its preventive effects remain a topic of debate. Moreover, its impact on conditions like cancer, cardiovascular diseases, and dementia is still under investigation.
- Vitamin C’s significance is further underscored by its historical context. Its discovery in 1912 and subsequent isolation in 1928 marked significant milestones in nutritional science. By 1933, it became the first vitamin to be chemically produced. Its pivotal role in preventing scurvy, a disease resulting from Vitamin C deficiency, further emphasizes its importance in human health.
- In the realm of plant biology, Vitamin C serves as a substrate for the enzyme ascorbate peroxidase, which uses ascorbate to neutralize excess hydrogen peroxide, converting it to water and oxygen.
- In conclusion, Vitamin C, or ascorbic acid, is an indispensable nutrient with multifaceted roles in human health. From its antioxidant properties to its significance in collagen synthesis and immune function, its contributions to physiology are vast and vital. Ensuring adequate intake, either through diet or supplementation, is essential for maintaining optimal health and preventing deficiencies.
Definition of Vitamin C (Ascorbic Acid)
Vitamin C, also known as ascorbic acid, is a water-soluble essential nutrient found in various foods, primarily in citrus fruits. It plays a crucial role in tissue repair, collagen synthesis, and acts as an antioxidant, protecting cells from damage. Humans must obtain it from dietary sources as they cannot produce it internally.
Properties of Ascorbic acid (Vitamin C)
- Physical Properties:
- Appearance: Ascorbic acid is a crystalline substance that is colorless and odorless.
- Taste: It has a mildly sour taste.
- Optical Activity: Ascorbic acid is optically active, with only the L-isomer exhibiting antiscorbutic (anti-scurvy) properties.
- Solubility:
- Water Solubility: It is soluble in water, making it a water-soluble vitamin.
- Alcohol Solubility: Ascorbic acid can dissolve in alcohol.
- Insolubility: It does not dissolve in chloroform, solvent ether, or light petroleum.
- Stability and Reactivity:
- Oxidation: Ascorbic acid is prone to oxidation, especially in the presence of metals like copper and iron. However, it remains stable around aluminum. This oxidative sensitivity explains the loss of ascorbic acid in foods cooked in copper utensils.
- Alkali Sensitivity: The vitamin is rapidly degraded by alkaline substances, but it remains stable in weak acid solutions. This stability in acidic environments is why baking soda adversely affects ascorbic acid content, while steam cooking has a minimal impact.
- Preservation: Ascorbic acid content can diminish during the drying and storage of vegetables. However, freezing does not detrimentally affect this vitamin. Minimal loss is observed when canning citrus and tomato juices.
- Chemical Properties:
- Reducing Agent: Due to its easily oxidizable nature, ascorbic acid acts as a potent reducing agent, participating in various biochemical reactions.
Sources of Vitamin C (Ascorbic Acid)
Vitamin C, also known as ascorbic acid, is an essential nutrient that cannot be synthesized by humans due to a genetic mutation. Therefore, it must be obtained from dietary sources. The primary sources of Vitamin C include:
- Fruits:
- Citrus Fruits: Such as oranges, lemons, grapefruits, and limes.
- Berries: Including strawberries, raspberries, blueberries, and cranberries.
- Tropical Fruits: Like kiwi, mangoes, pineapples, and guavas.
- Melons: Such as cantaloupe and watermelon.
- Other Fruits: Tomatoes, cherries, and gooseberries (amla).
- Vegetables:
- Leafy Greens: Spinach and mustard greens.
- Cruciferous Vegetables: Broccoli, cauliflower, and Brussels sprouts.
- Root Vegetables: Potatoes (especially the skin).
- Peppers: Both green and red bell peppers.
- Others: Peas, bean sprouts, and cabbage.
It’s worth noting that while many fruits and vegetables are rich in Vitamin C, milk is a minimal source of this nutrient. To ensure adequate intake, it’s recommended to consume a varied diet rich in fruits and vegetables.
Chemical structure of ascorbic acid (Vitamin C)
- Nomenclature:
- Generic Name: The compound is universally termed as l-ascorbic acid.
- Alternate Names: It is also referred to as ascorbate and antiscorbutic vitamin.
- Molecular Structure:
- Basic Framework: The molecule of l-ascorbic acid comprises an asymmetrical configuration of six carbon atoms (C6H8O6), bearing a structural resemblance to glucose.
- Molecular Weight & Physical Properties: The molecular weight of ascorbic acid is 176. It has a melting point ranging between 190–192°C (with decomposition) and a density of approximately 1.65 g/cm3.
- Solubility: l-Ascorbic acid is highly soluble in water, yielding a clear, colorless to slightly yellow solution. However, its solubility diminishes in alcohol and is virtually insoluble in chloroform, ether, and benzene.
- Acidity: The compound exhibits two pKa values: 4.2 and 11.6, with a pH range of 2.2–2.5 in a 5% (w/v) water solution.
- Chemical Stability:
- Oxidation: Ascorbic acid is inherently unstable, being susceptible to oxidation, especially when exposed to light, oxygen, elevated temperatures, alkaline conditions, and specific metals like copper. This oxidative vulnerability renders it a potent reducing agent.
- Physical Form: Typically, ascorbic acid manifests as a white or slightly yellowish crystalline powder. In its crystalline state, it remains chemically stable when dry.
- Derivatives:
- Variants: Numerous derivatives of ascorbic acid exist, including sodium l-ascorbate, calcium l-ascorbate, zinc-ascorbate, 6-palmityl-l-ascorbic acid, and ascorbyl monophosphate calcium sodium salt.
- Production: These derivatives are synthesized through various chemical reactions. For instance, ascorbic acid is derived from sodium ascorbate via cation exchange, while calcium ascorbate results from the interaction of ascorbic acid with calcium carbonate.
- Biological Activity:
- Hexose Derivative: Ascorbic acid is a hexose derivative, bearing a structural similarity to monosaccharides.
- Oxidative Forms: l-Ascorbic acid can be oxidized to dehydroascorbic acid, a reversible reaction. Both forms are biologically active. However, the D-ascorbic acid isomer is biologically inert. The predominant form in plasma and tissues is the reduced ascorbic acid, with a ratio of ascorbic acid to dehydroascorbic acid in many tissues being 15:1.
Vitamin C metabolism
- Vitamin C, predominantly recognized as ascorbic acid, plays a pivotal role in various biochemical processes, largely attributed to its inherent reducing properties. Its metabolic functions are intricately linked to its oxidation mechanisms, which are influenced by pH variations and not necessarily by the presence of air or external oxidizing agents.
- The metabolic pathway of ascorbic acid involves a series of oxidation steps. Initially, ascorbic acid can be reversibly oxidized to dehydroascorbic acid under specific conditions such as exposure to copper, mildly alkaline environments, and elevated temperatures.
- Dehydroascorbic acid, possessing a notably brief half-life, can undergo both reversible and irreversible oxidation within tissues. In a mildly acidic environment (pH 4.0), dehydroascorbic acid can irreversibly transform into 2,3-diketo-l-glutonic acid, commonly referred to as diketogulonic acid. This irreversible transformation is expedited in neutral to alkaline pH levels.
- Notably, diketogulonic acid, despite being a potent reducing agent, lacks the anti-ascorbutic properties of its precursor. In specific acidic conditions, in the presence of H2S, dehydroascorbic acid can also revert to ascorbic acid. Both ascorbic and dehydroascorbic acids exhibit equivalent anti-ascorbutic effects.
- The subsequent oxidation phase produces l-threonic acid and oxalic acid, a reaction that predominantly occurs in alkaline conditions (pH 7-9). Occasionally, carbon dioxide may emerge as a byproduct of vitamin C oxidation, especially at elevated concentrations.
- In humans, the metabolic trajectory of ascorbic acid encompasses its reversible oxidation to dehydroascorbic acid. This can either be reduced back to ascorbic acid or undergo hydrolysis to form diketogulonic acid, which subsequently oxidizes to produce various compounds including oxalic acid, threonic acid, xylose, xylonic acid, and lyxonic acid.
- The redox reactions categorize ascorbic acid as the reduced form of vitamin C, while dehydroascorbic acid represents its oxidized counterpart. It’s imperative to note that only the l-isomer of ascorbic acid exhibits biological activity, with other isomers like d-ascorbic acid, d-isoascorbic acid, and l-isoascorbic acid being biologically inert.
- The excretion of ascorbic acid, both its absorbed and unabsorbed forms, can occur in both conjugated and non-conjugated manners. It can undergo partial conjugation with sulfate to yield ascorbate-2-sulphate, which is subsequently excreted via urine. The unaltered form of ascorbic acid, along with its metabolites, is also excreted in urine.
- In certain species like guinea pigs, rats, and rabbits, high doses of unabsorbed ascorbic acid can be oxidized to carbon dioxide by intestinal flora, representing a primary excretory mechanism for vitamin C. The equilibrium between ascorbic acid and dehydroascorbic acid is contingent on the cellular redox status.
Recommended dietary allowance (RDA)
The Recommended Dietary Allowance (RDA) is a guideline that specifies the daily intake level of specific nutrients required to meet the needs of nearly all (97-98%) healthy individuals in a particular age and gender group. The RDA aims to ensure adequate nutritional status, taking into consideration growth, maintenance, and prevention of deficiency diseases.
For vitamin C, the RDA varies based on age, gender, and life stage:
- Infants:
- 0-6 months: 40 mg/day
- 7-12 months: 50 mg/day
- Children:
- 1-3 years: 15 mg/day
- 4-8 years: 25 mg/day
- 9-13 years: 45 mg/day
- Adolescents:
- Boys 14-18 years: 75 mg/day
- Girls 14-18 years: 65 mg/day
- Adults:
- Men 19 years and older: 90 mg/day
- Women 19 years and older: 75 mg/day
- Pregnancy:
- 18 years and younger: 80 mg/day
- 19 years and older: 85 mg/day
- Lactation:
- 18 years and younger: 115 mg/day
- 19 years and older: 120 mg/day
- Special Considerations:
- Smokers may require an additional 35 mg/day due to increased oxidative stress and metabolic turnover of vitamin C.
Biological functions of Vitamin C
- Collagen Synthesis: Ascorbic acid acts as a coenzyme in the hydroxylation of amino acids, proline and lysine, during the transformation of protocollagen to collagen. This post-translational modification is orchestrated by enzymes – lysyl hydroxylase and prolyl hydroxylase. The presence of vitamin C, molecular oxygen, and α-ketoglutarate is imperative for this reaction. The resultant hydroxyproline and hydroxylysine are quintessential for collagen cross-linking, thereby fortifying the collagen fiber.
- Neurotransmitter Synthesis: The synthesis of serotonin, a neurotransmitter, from tryptophan involves a hydroxylation reaction facilitated by Vitamin C.
- Folate Metabolism: The enzyme Dihydrofolate reductase (DHFR) is activated by Vitamin C, which in turn plays a role in converting 7,8-dihydrofolate (DHF) to 5,10-methylene tetrahydrofolate (THF).
- Lipid Metabolism: Vitamin C aids in cholesterol biosynthesis by activating the enzyme 7-α-hydroxylase.
- Hemoglobin Production: Ascorbic acid promotes iron absorption from the intestine, which is indispensable for hemoglobin synthesis.
- Steroid Hormone Production: The biosynthesis of corticosteroid hormones involves hydroxylation reactions catalyzed by Vitamin C.
- Endocrine System: The adrenal gland and gonads require substantial amounts of Vitamin C for their maintenance and optimal functioning.
- Disease Prevention: Vitamin C, in conjunction with Vitamins A and E, can delay the onset of cataracts and has been linked to the prevention of coronary heart diseases and tumor formation.
- Antioxidant Activity: Vitamin C acts as a protective agent against the oxidation of cellular components by free radicals, peroxides, and superoxides. It also enhances the stability of other vitamins, such as Vitamin A, E, and some B vitamins.
- Immune System Enhancement: Vitamin C bolsters the immune system by stimulating antibody formation and enhancing phagocytosis. It is also involved in the neutralization of bacterial toxins, especially endotoxins.
- Anti-inflammatory and Anti-aging Properties: Vitamin C exhibits anti-inflammatory effects by inhibiting inflammatory mediators like tumor necrosis factor alpha. Its antioxidant properties also contribute to its anti-aging effects, promoting collagen formation and protecting existing collagen.
- Depigmenting Effect: Ascorbic acid acts as a potent depigmenting agent, useful in treating various hyperpigmentation conditions. It inhibits melanogenesis through multiple mechanisms, including the prevention of free radical production and direct suppression of the tyrosinase enzyme.
- Bone Morphogenesis: Vitamin C is instrumental in bone development, given that bones comprise an organic matrix, predominantly collagen, and inorganic constituents like calcium and phosphate.
- Iron and Hemoglobin Dynamics: Vitamin C augments iron absorption by maintaining it in its ferrous state, courtesy of its inherent reducing potential. It also facilitates the synthesis of ferritin, the iron storage protein, and the liberation of iron from it. Furthermore, vitamin C aids in the conversion of methemoglobin back to hemoglobin and is pivotal in the catabolism of hemoglobin to bile pigments.
- Tryptophan Pathway: In the biosynthesis of the neurotransmitter serotonin, vitamin C is indispensable for the hydroxylation of tryptophan to hydroxytryptophan.
- Tyrosine Metabolism: Within the tyrosine metabolic pathway, vitamin C catalyzes the oxidation of p-hydroxy phenylpyruvate to homogentisic acid.
- Folic Acid Dynamics: The biologically active form of folic acid is tetrahydrofolate (FH4). Vitamin C is requisite for the biogenesis of FH4 and, in conjunction with FH4, plays a role in erythrocyte maturation.
- Peptide Hormone Generation: Several peptide hormones possess a carboxyl terminal amide derived from terminal glycine. The hydroxylation of this glycine, mediated by peptidylglycine hydroxylase, mandates the presence of vitamin C.
- Corticosteroid Hormone Synthesis: The adrenal gland, especially during stress episodes, is enriched with ascorbic acid. It is postulated that vitamin C is vital for hydroxylation reactions in the biosynthesis of corticosteroid hormones.
- Vitamin Conservation: As a potent antioxidant, ascorbic acid shields other vitamins, including vitamins A, E, and certain B-complex vitamins, from oxidative degradation.
- Immunomodulation: Vitamin C amplifies the production of immunoglobulins and bolsters the phagocytic capabilities of leukocytes.
- Cataract Prophylaxis: Regular intake of vitamin C can mitigate the risk of cataract development.
- Protection Against Chronic Ailments: Owing to its antioxidant properties, vitamin C can potentially reduce the susceptibility to diseases such as cancer, coronary heart diseases, and cataracts.
Biosynthesis of Vitamin C
- Vitamin C, predominantly sourced from plants, plays a pivotal role in various physiological processes. Intriguingly, while the majority of animals possess the capability to endogenously produce ascorbic acid from glucose via the uronic acid pathway, certain species, including humans, some primates, guinea pigs, and bats, lack this biosynthetic ability. This deficiency in endogenous synthesis is attributed to the absence of a crucial enzyme, L-gulonolactone oxidase.
- L-gulonolactone oxidase functions as a catalyst in the terminal phase of the ascorbic acid biosynthesis pathway. Specifically, it facilitates the conversion of L-glucono-1,4-lactone into L-ascorbic acid. The absence of this enzyme in the aforementioned species necessitates the external dietary intake of vitamin C to meet physiological demands and prevent deficiency-related complications.
- In essence, while the biosynthesis of vitamin C is a common metabolic feature in many animals, the lack of the enzyme L-gulonolactone oxidase in certain species underscores the importance of dietary sources of this vital nutrient.
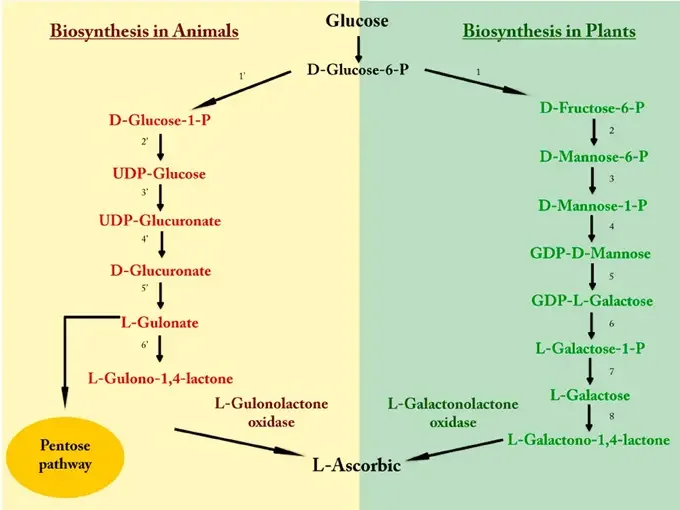
Biosynthesis of Vitamin C in Animals:
Vitamin C synthesis in animals, especially in some mammals, starts from glucose. The following is a step-by-step breakdown:
- Glucose Conversion: Glucose is initially converted into D-Glucose-6-Phosphate (D-Glucose-6-P).
- Formation of D-Glucose-1-Phosphate: The D-Glucose-6-P is then isomerized to form D-Glucose-1-Phosphate (D-Glucose-1-P).
- Synthesis of UDP-Glucose: D-Glucose-1-P undergoes activation with UTP (Uridine Triphosphate) to form UDP-Glucose.
- Formation of UDP-Glucuronate: UDP-Glucose is oxidized to form UDP-Glucuronate.
- Production of D-Glucuronate: A decarboxylation step results in the formation of D-Glucuronate from UDP-Glucuronate.
- L-Gulonate Synthesis: D-Glucuronate is then reduced to form L-Gulonate.
- L-Gulono-1,4-lactone Formation: L-Gulonate undergoes a ring-closure reaction catalyzed by the enzyme L-Gulonolactone oxidase to form L-Gulono-1,4-lactone.
- Pentose Pathway: L-Gulono-1,4-lactone enters the Pentose pathway, ultimately leading to the synthesis of L-Ascorbic acid (Vitamin C).
Biosynthesis of Vitamin C in Plants:
Plants have a different pathway for synthesizing Vitamin C, which involves the following steps:
- Glucose Conversion: Just like in animals, the process in plants also begins with the conversion of glucose into D-Glucose-6-Phosphate.
- Isomerization to D-Fructose-6-Phosphate: D-Glucose-6-P is isomerized to produce D-Fructose-6-Phosphate.
- Conversion to D-Mannose-6-P: D-Fructose-6-P is transformed into D-Mannose-6-Phosphate.
- Formation of D-Mannose-1-P: This is then converted to D-Mannose-1-Phosphate.
- Synthesis of GDP-D-Mannose: Activation of D-Mannose-1-P results in the formation of GDP-D-Mannose.
- Production of GDP-L-Galactose: GDP-D-Mannose undergoes epimerization to produce GDP-L-Galactose.
- Conversion to L-Galactose-1-P: GDP-L-Galactose is further converted to L-Galactose-1-Phosphate.
- Formation of L-Galactose: A dephosphorylation step results in the synthesis of L-Galactose.
- L-Galactono-1,4-lactone Formation: L-Galactose is oxidized by the enzyme L-Galactonolactone oxidase to form L-Galactono-1,4-lactone.
- Vitamin C Synthesis: L-Galactono-1,4-lactone is then directly converted into L-Ascorbic acid, also known as Vitamin C.
Vitamin C route of administration
- Oral Route:
- The most prevalent method of vitamin C intake is orally, typically in the form of tablets, powders, or solutions.
- This route is often utilized in multivitamin supplements, ensuring systemic distribution of the vitamin.
- Parenteral Route:
- Intravenous administration is reserved for specific conditions, such as advanced cancer therapy or severe illnesses, serving as an adjunct treatment.
- Notably, the plasma concentration of vitamin C post intravenous administration can be significantly higher than that achieved through oral intake. This elevation can reach up to 25-70 fold, leading to increased urinary excretion levels.
- However, precise control over plasma levels is challenging with this route.
- Topical Route:
- Direct application to the skin is another method, especially beneficial for peripheral structures like the skin, which may not receive adequate vitamin C from oral intake.
- Despite its advantages, the epidermal absorption of ascorbic acid is limited due to its water-soluble nature. To enhance penetration, esterified forms like ascorbyl palmitate and magnesium ascorbyl phosphate are employed.
- The absorption efficiency is influenced by the acidity and concentration of the topical solution. An acidic pH below 3.5 is optimal, and concentrations up to 20% ensure maximum absorption and tissue saturation.
- Transdermal Route:
- This encompasses techniques like sonophoresis and nanoparticle delivery, aiming to enhance the absorption of hydrophilic drugs, including ascorbic acid.
- Sonophoresis utilizes ultrasound waves to increase the kinetic energy of the drug, facilitating deeper penetration through skin layers.
- Nanoparticles offer a solution to the instability of l-ascorbic acid by encapsulating it in various formulations, ensuring controlled release and enhanced penetration.
- Injection:
- Direct injection of vitamin C, such as intraperitoneal administration, has been explored in specific studies. For instance, a study in 2004 observed a reduction in inflammatory cytokines and an improvement in tissue collagen post vitamin C injection.
Vitamin C bioavailability – Absorption, Distribution, and Excretion
1. Absorption Mechanisms of Vitamin C: Vitamin C, also known as ascorbic acid, possesses a hydrophilic nature, which aids its absorption across various regions such as the buccal mucosa, stomach, and small intestine. While passive diffusion is the primary mechanism in the buccal mucosa, the small intestine employs an active transport mechanism. This active transport is facilitated by the sodium vitamin C transporter type 1 (SVCT1), which also plays a role in the retina’s vitamin C transport. Another transporter, SVCT2, is responsible for vitamin C transport to organs like the brain, lung, liver, heart, and skeletal muscles. Interestingly, glucose has been identified as an inhibitor of this absorption process.
2. Distribution Patterns of Vitamin C: Once absorbed, Vitamin C is disseminated throughout the body tissues. It is found in high concentrations in the adrenal gland, pituitary gland, and retina. Conversely, its levels are relatively lower in the kidneys and muscles.
3. Excretion Pathways of Vitamin C: The kidneys play a pivotal role in the excretion of Vitamin C and its metabolites, primarily in the form of oxalate salts. The urinary excretion rate of Vitamin C is contingent on the intake dose. For instance, at an intake of less than 100 mg/day, no detectable Vitamin C is found in urine. However, as the intake increases, so does the percentage excreted. Notably, at higher doses, a significant amount of unmetabolized Vitamin C is excreted, with the concentration in blood and tissues also increasing. The body’s response to these elevated doses includes increased excretion through the kidneys and sweat.
4. Storage Dynamics of Vitamin C: Vitamin C is stored in various organs and body fluids. While it is always present in its reduced form (ascorbic acid) in human blood, its levels vary in different organs. For instance, in 1936, it was observed that organs like the pancreas, spleen, and thymus gland had lower ascorbic acid levels. Additionally, certain conditions, such as neural diseases and alcoholism, have been linked to Vitamin C deficiency.
5. Dosage and Safety Considerations: The absorption efficiency of Vitamin C varies with the dose. While smaller doses (15-30 mg) have an absorption rate of up to 98%, this rate drops to around 50% for larger doses (1000-1250 mg). The plasma saturation level of ascorbic acid is approximately 70 μM, which regulates its renal excretion. It’s noteworthy that despite its rapid excretion due to its water-soluble nature, Vitamin C is considered safe in standard doses. The recommended daily allowance (RDA) has been set at 75 mg for women and 90 mg for men, with a maximum bioavailability achieved at 500 mg.
Deficiency symptoms
- Scurvy: A hallmark of vitamin C deficiency, scurvy is typified by gingival alterations, specifically spongy and inflamed gums. This gingival inflammation often progresses to soreness and can lead to dental instability, resulting in loose teeth.
- Hematological Aberrations: Anemia, a reduction in the number or functionality of red blood cells, is a notable consequence of ascorbic acid deficiency. Additionally, the integrity of blood vessels is compromised, rendering them fragile and prone to rupture.
- Joint Pathology: Individuals deficient in vitamin C often present with joint swelling, indicative of inflammatory processes at play.
- Immunological Impairment: A pivotal role of vitamin C lies in bolstering the immune system. Consequently, its deficiency translates to diminished immunocompetence, rendering individuals susceptible to infections.
- Endocrine Disruptions: The adrenal cortex and gonads, integral components of the endocrine system, exhibit attenuated hormonal activity in the absence of adequate vitamin C.
- Skeletal Complications: Osteoporosis, characterized by reduced bone density and increased fracture risk, is a potential sequela of prolonged vitamin C deficiency.
- Wound Healing Delays: Vitamin C plays a quintessential role in tissue repair and regeneration. Its deficiency impedes the wound healing process, leading to prolonged recovery times.
- Antioxidant Property Impairment: Vitamin C’s antioxidant capabilities are paramount in neutralizing free radicals and preventing cellular damage. A deficit in this vitamin compromises its antioxidant functions, potentially leading to cellular and tissue damage.
Implications of Excessive Ascorbic Acid Intake (Hypervitaminosis C)
- In the annals of vitamin research, Linus Pauling’s 1970 proposition stands out as a significant and controversial recommendation. Pauling fervently advocated for the ingestion of substantial quantities of ascorbic acid, suggesting dosages as high as 18 g/day, which is a staggering 300-fold increase over the daily recommended intake. His primary rationale was the prevention and mitigation of the common cold. This audacious recommendation, often paraphrased as “store vitamin C in large quantities and consume in grams,” ignited widespread debate within the scientific community.
- Subsequent research has provided clarity on this matter. While it has been established that such megadoses of vitamin C do not outright prevent the onset of the common cold, there is evidence to suggest that they can attenuate the duration and severity of its symptoms. This beneficial effect is postulated to arise from ascorbic acid’s role in enhancing leukocyte functionality.
- Despite the lack of consensus on its prophylactic efficacy against the common cold, high doses of vitamin C (ranging from 1-4 g/day) continue to be administered in specific scenarios, such as wound recovery and post-traumatic care. Given its potent antioxidant properties, ascorbic acid undeniably confers certain health advantages.
- However, it is essential to approach the topic of vitamin C megadoses with caution. While ascorbic acid in its native form is generally considered non-toxic, its oxidized counterpart, dehydroascorbic acid, has been identified as potentially harmful. Additionally, the metabolic breakdown of vitamin C yields oxalate, a compound implicated in the genesis of renal calculi or kidney stones. While there have been conflicting reports, some suggest that excessive vitamin C intake might elevate the risk of urinary stone formation.
- In summary, while vitamin C is indispensable for various physiological processes, its excessive consumption, especially in megadoses, warrants careful consideration and further scientific scrutiny.
References
- https://www.geeksforgeeks.org/ascorbic-acid-formula/
- https://www.brainkart.com/article/Vitamin-C_34113/
- https://byjus.com/chemistry/ascorbic-acid/