What is Vibrio cholerae?
- Vibrio cholerae is a Gram-negative bacterium responsible for cholera, a severe diarrheal disease. It is a facultative anaerobe, meaning it can survive with or without oxygen, and has a distinctive comma-shaped appearance. V. cholerae naturally inhabits brackish or saltwater environments, where it attaches to chitin found in the shells of aquatic animals like crabs and shrimp.
- Though Vibrio bacteria are predominantly found in the marine environment, only a few species, including V. cholerae, are pathogenic to humans. These bacteria are classified into choleragenic and non-choleragenic groups. The choleragenic strains, particularly O1 and O139, are responsible for cholera outbreaks. Other species like Vibrio parahaemolyticus, Vibrio vulnificus, and Vibrio fluvialis fall into the non-choleragenic category and typically cause less severe illnesses.
- Cholera is primarily transmitted through contaminated water or food, particularly seafood, which has been infected by fecal matter from an infected person. Once ingested, V. cholerae invades the intestinal mucosa, leading to watery diarrhea and vomiting. The rapid loss of fluids can result in severe dehydration, which, if untreated, can lead to death.
- Vibrio cholerae is characterized by the presence of a flagellum at one pole, which aids in movement, and pili scattered across its surface, which help the bacterium adhere to host tissues. The bacterium’s metabolism is both respiratory and fermentative, allowing it to thrive in a variety of conditions.
- The cholera toxin (CT) produced by V. cholerae is a major factor in the severity of the disease. This toxin, responsible for the characteristic “rice-water stool”, is encoded by genes carried within a temperate bacteriophage (CTXφ). The bacterium only produces the toxin when the viral DNA integrates into its own.
- Additionally, V. cholerae is known for its ability to regulate gene expression through quorum sensing, a process by which bacteria communicate to coordinate behavior. In the case of cholera, quorum sensing allows the bacteria to prolong host survival by limiting nutrient intake, which in turn modulates host immune responses.
- Cholera remains a major public health concern, particularly in areas with poor sanitation. Despite the rarity of cholera outbreaks in developed countries, due to proper sanitation and water treatment, the disease continues to affect millions globally, with an estimated 91,000 deaths annually.
- Treatment for cholera involves oral rehydration solutions (ORS) and antibiotics, such as fluoroquinolones and tetracyclines, which help reduce the severity of the illness. Ringer’s lactate, a solution of salts and water, is also commonly used to restore lost fluids.
- Cholera outbreaks often occur in developing countries, where sanitation and hygiene practices are insufficient. These outbreaks can spread rapidly, especially in areas with contaminated water supplies, further highlighting the importance of water treatment and access to clean drinking water.
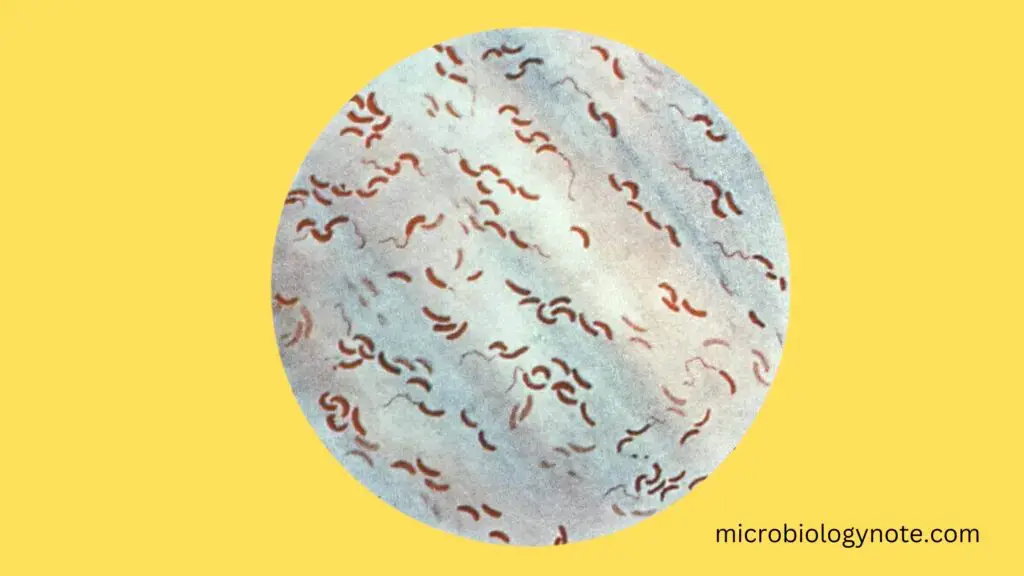
Geographical Distribution and Habitat of Cholera
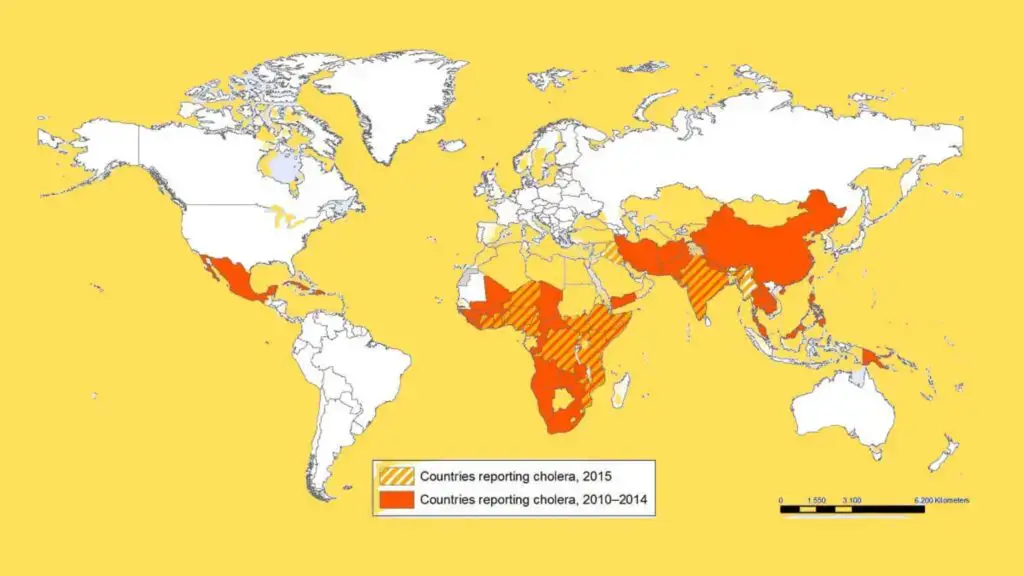
Cholera is a global health issue, primarily affecting parts of Africa and Asia, though it has also spread to other regions. The condition is rare in developed countries, but still a persistent threat in many developing areas.
Geographical Distribution
- Endemic, Epidemic, and Pandemic Patterns:
- Cholera can occur in endemic, epidemic, or pandemic forms.
- In 2004, cholera affected 56 countries, reporting 101,338 cases and 2,345 deaths. The majority of these cases were in Africa and Asia.
- Pandemics of Cholera:
- Six pandemics occurred in the 19th century, with major outbreaks in Europe and the United States, leading to significant death tolls, including over 115,000 deaths in 1832 and 50,000 deaths in 1856.
- The seventh pandemic began in 1961 and spread across five continents by 1991, causing widespread outbreaks in South and Central America, as well as parts of the United States and Canada.
- Unlike the earlier pandemics caused by V. cholerae biotype Classical, the seventh pandemic was driven by the Eltor biotype of V. cholerae, originating in Asia in the 1960s. It reached Africa in the 1970s, and South America in the 1990s.
- Key Features of the Seventh Pandemic:
- It was the first pandemic to originate outside the Indian subcontinent—beginning in Sulawesi (Indonesia), then spreading to Hong Kong, the Philippines, and India by 1964. By 1966, it had spread through the Indian subcontinent and West Asia.
- The pandemic was caused by the Eltor biotype, whereas earlier pandemics were caused by the Classical biotype.
- The Eltor biotype caused less severe illness than its predecessor, with a higher proportion of mild and asymptomatic infections, leading to a lower mortality rate but a higher carrier rate.
- The Eltor strain eventually replaced the Classical strain, making the latter rare in countries like India, although a resurgence of Classical strains was observed in Bangladesh.
- Emergence of New Strains:
- In 1992, a new strain, V. cholerae O139, emerged and caused an epidemic in India, which then spread to Bangladesh by 1993, leading to cholera outbreaks in both countries.
Habitat of Vibrio cholerae
- Primary Habitat:
- Vibrio cholerae is a saltwater bacterium that primarily inhabits marine ecosystems, particularly in association with plankton.
- The bacteria thrive and multiply in contaminated water, especially during warm and hot months of the year.
- Human Involvement:
- Vibrio cholerae does not naturally reside in healthy humans. However, it can inhabit the small intestine of infected individuals, where it causes cholera.
The bacterium’s ability to grow in aquatic environments and its reliance on contaminated water sources contribute to its spread, especially in regions with inadequate sanitation and water treatment.
Vibrio cholerae Classification
Vibrio cholerae, the bacterium responsible for cholera, is classified based on several key characteristics. The primary factors for this classification include serogroups, serotypes, and biotypes.
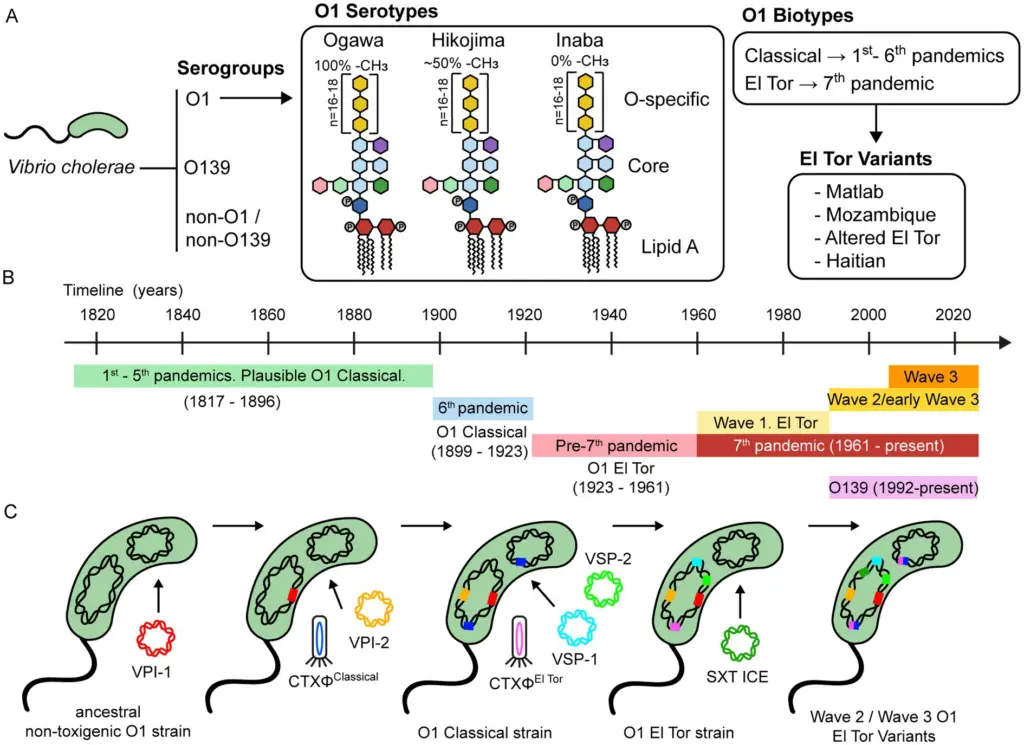
- Serogroups
Vibrio cholerae is divided into more than 200 serogroups based on the O-antigen of its lipopolysaccharide (LPS).- O1 and O139: These serogroups are the primary cause of cholera and epidemics. They can produce cholera toxin (CTX), which leads to severe diarrheal disease.
- Non-O1/non-O139: These serogroups lack the ability to produce cholera toxin and are typically associated with minor outbreaks, such as gastroenteritis, bacteremia, and wound infections. They do not cause cholera.
- A notable feature of non-O1 serogroups, including O139, is that over 85% of them have a capsule, which plays a crucial role in virulence for extraintestinal infections.
- Serotypes within O1
Vibrio cholerae O1 strains are further classified into three serotypes:- Ogawa: These strains have methylated terminal perosamine in their LPS.
- Inaba: These strains are characterized by unmethylated terminal perosamine.
- Hikojima: This serotype expresses both methylated and unmethylated O-antigens. It is rare and often considered a transitional form, typically appearing during serotype switching between Ogawa and Inaba.
- Biotype
V. cholerae O1 strains are also classified into two biotypes:- Classical: This biotype is historically associated with earlier cholera outbreaks.
- El Tor: El Tor biotype strains are more efficient at spreading between hosts, survive better in environmental and gut conditions, and are more likely to result in asymptomatic carriers. They tend to be more successful in transmission compared to Classical strains, which have a higher rate of symptomatic infections.
Characteristics of Vibrio cholerae
Vibrio cholerae is a versatile and highly motile bacterium that possesses several distinctive characteristics which are crucial for both its identification and its ability to cause disease.
- Shape and Size:
- Gram-negative rod: V. cholerae is a gram-negative bacterium, which means it does not retain the crystal violet stain used in Gram’s method of staining.
- Comma-shaped or curved-rod: Typically, it appears comma-shaped, but in some laboratory cultures, it can appear as a straight rod.
- The bacteria are small, measuring 0.3 μm in diameter and 1.3 μm in length, though the size can vary slightly depending on the growth conditions.
- Motility:
- V. cholerae is highly motile, thanks to the presence of a single polar flagellum at one end of the bacterium.
- This flagellum enables the bacterium to exhibit darting motility, meaning it can swim rapidly in a directed manner.
- The average swimming velocity is around 75.4 μm/sec, which allows V. cholerae to move efficiently through its environment.
- Environmental Tolerance:
- V. cholerae can tolerate a range of environmental conditions, making it quite resilient.
- It thrives in a temperature range from 14°C to 40°C.
- The bacterium is capable of surviving in media with up to 6% NaCl, which is higher than most non-halophilic bacteria.
- It can also survive in alkaline media that would typically kill most other intestinal bacteria, though it is sensitive to acidic conditions.
- Metabolism and Oxygen Requirements:
- V. cholerae is an aerobe, meaning it primarily requires oxygen for growth.
- Unlike other vibrio species that are facultative anaerobes, V. cholerae cannot grow without oxygen for long periods.
- It is capable of both respiratory and fermentative metabolism, allowing it to adapt to different environmental conditions.
- Surface Structures:
- The bacterium features a flagellum at one cell pole, which is responsible for its motility.
- It also has pili on its surface, which may play a role in attachment to host tissues.
- Growth and Culture:
- In initial isolates, V. cholerae often appears slightly curved, but as the culture ages or under laboratory conditions, it can become more rod-like.
- These structural features help distinguish V. cholerae from other bacteria and play a role in its pathogenicity.
These characteristics help Vibrio cholerae to survive in diverse environments, including brackish water and the human gut, where it can cause cholera. Its motility, adaptability, and ability to thrive in alkaline conditions are key to its success as a pathogen.
Morphology of Vibrio cholerae
Vibrio cholerae has a distinct structure that helps it survive and thrive in various environments, including the human gut, where it causes cholera. Here’s a breakdown of its key morphological features:
- Shape and Size:
- Comma-shaped: V. cholerae is most commonly seen as a comma-shaped bacillus, a characteristic feature that makes it stand out.
- Pleomorphic: In older cultures, the bacterium can show a variety of shapes, making it pleomorphic.
- The bacterium typically measures 1–3 μm in length and 0.5–0.8 μm in diameter. This size is important for its ability to fit into tight spaces and be mobile in the human intestine.
- Cell Arrangement:
- V. cholerae can sometimes appear as S-shaped or spiral forms. This happens when two or more cells are lined up end-to-end.
- Under the microscope, especially in stained smears from cholera cases, the bacteria often arrange themselves in parallel rows. This is referred to as the “fish in stream” appearance, a term coined by Robert Koch.
- Motility:
- V. cholerae is actively motile.
- It moves using a single polar flagellum, a structure that allows it to exhibit a darting-type motility, giving it a characteristic movement pattern.
- When observed under a microscope, it often appears as a “swarm of gnats,” which indicates its highly active and rapid motion.
- Other Features:
- The bacterium does not form spores, does not have a capsule, and is non-acid-fast. These features contribute to its ability to survive in certain environments but also indicate a lack of some common protective mechanisms found in other bacteria.
Cultural Characteristics and Biochemical Reactions of Vibrio cholerae
Vibrio cholerae thrives under specific growth conditions and exhibits several distinct biochemical reactions that aid in its identification and differentiation. Below is a detailed breakdown of these characteristics.
Cultural Characteristics
- Aerobic Growth:
- V. cholerae is strongly aerobic and grows best in oxygen-rich environments.
- Anaerobic conditions result in slower, limited growth.
- Temperature & pH Preferences:
- It grows within a temperature range of 16–40°C, with an optimum temperature of around 37°C.
- The bacterium prefers an alkaline environment with a pH range of 7.4–9.6 (optimum pH of 8.2).
- Sodium chloride is essential for optimal growth, with a concentration range of 0.5–1%. High salt concentrations (5% and above) inhibit its growth, but it can grow without salt, unlike other halophilic bacteria.
- Media for Growth:
V. cholerae grows on a variety of media:- Nonselective Media:
- On nutrient agar, colonies appear moist, translucent, and about 1–2 mm in diameter with a bluish or greenish tinge.
- On MacConkey agar, colonies are colorless initially but turn reddish after prolonged incubation due to lactose fermentation.
- On blood agar, biotype Eltor produces hemolytic colonies, while Classical strains produce a greenish discoloration.
- In gelatin stab cultures, the bacterium produces funnel-shaped liquefaction after 3 days at 22°C.
- In peptone water, a fine surface pellicle forms after 6–9 hours of incubation.
- Transport Media:
- VR Medium: Used to maintain viability during transport. Contains crude sea salt and peptone, with a pH of 8.6–8.8.
- Cary–Blair Medium: A solid medium with a buffered solution, ideal for transport and maintaining bacterial viability. It has an alkaline pH of 8.
- Enrichment Media:
- Alkaline Peptone Water (APW): Promotes growth of V. cholerae by inhibiting other intestinal bacteria. It has a pH of 8.6 and is used for stool enrichment.
- Monsur’s Taurocholate Tellurite Peptone Water: Also used for stool enrichment, this medium has a pH of 9.0 and contains potassium tellurite to select for V. cholerae.
- Selective Media:
- TCBS Medium (pH 8.6): Contains sucrose and produces yellow colonies from V. cholerae due to sucrose fermentation. Non-sucrose fermenters, like V. parahaemolyticus, form blue-green colonies.
- Monsur’s GTTA Medium (pH 8.5): Contains potassium tellurite and selects for V. cholerae, producing translucent colonies with grayish-black centers.
- Alkaline BSA (pH 8.2): Similar colony formation to nutrient agar, used to isolate V. cholerae.
- Nonselective Media:
Biochemical Reactions
- Catalase & Oxidase Tests:
- V. cholerae is catalase positive and oxidase positive, important for differentiating it from other bacteria.
- Sugar Fermentation:
- V. cholerae ferments several sugars, including glucose, sucrose, maltose, mannitol, and mannose, but does not produce gas in the process.
- It is a late lactose fermenter, fermenting lactose after several days of incubation.
- It does not ferment arabinose, inositol, or dulcitol.
- Indole Production & Nitrate Reduction:
- V. cholerae forms indole and reduces nitrates to nitrites.
- The cholera red reaction is a result of this process. Adding concentrated sulfuric acid to a 24-hour peptone water culture at 37°C turns the medium a reddish-pink color due to the formation of nitroso-indole.
- Other Reactions:
- Methyl Red Positive: V. cholerae shows a positive methyl red test, indicating mixed acid fermentation.
- Urease Negative: It does not produce urease, which is a common trait for many enteric bacteria.
- Gelatin Liquefaction: It liquefies gelatin, a trait that helps in its identification.
- Decarboxylates Lysine & Ornithine: V. cholerae decarboxylates lysine and ornithine but does not decarboxylate arginine.
- Biotype Differences:
- Classical and Eltor biotypes of V. cholerae show some variation in biochemical reactions.
- These differences include variable results in the Voges-Proskauer reaction, hemolysis of sheep RBCs, and hemagglutination of chick RBCs.
Cell Wall Components and Antigenic Structure of Vibrio cholerae
Vibrio cholerae possesses a unique antigenic structure that plays a key role in its identification and classification. Understanding these components, including the somatic O antigen and flagellar H antigen, helps in the serological identification and biotyping of the bacteria.
Cell Wall Components
- Vibrio cholerae, like other Gram-negative bacteria, contains lipopolysaccharide (LPS) in its outer membrane.
- While LPS itself doesn’t contribute directly to the pathogenesis of cholera, it does play a crucial role in the immune response when a killed V. cholerae vaccine is administered.
Antigens of Vibrio cholerae
- Somatic O Antigen:
- This antigen is part of the bacterial cell wall.
- It is a group-specific antigen, making it essential in the serological identification of V. cholerae.
- Flagellar H Antigen:
- Found in the flagella, the H antigen is heat-labile and is shared across all strains of V. cholerae.
- This antigen is crucial for distinguishing different types of V. cholerae based on their flagellar composition.
Serological Classification
- Vibrio cholerae is classified according to the somatic carbohydrate O antigen into various serotypes.
- This classification was first proposed by Gardner and Venkatraman in 1935.
- More than 200 serotypes of V. cholerae have been identified, with the two major groups being:
- V. cholerae O1
- Non-O1 V. cholerae
- V. cholerae O1 strains:
- These strains react with specific antisera to the O1 group and are called cholera vibrios or agglutinable vibrios.
- V. cholerae O1 is further subdivided into two biotypes:
- Eltor: Often associated with the more recent cholera pandemics.
- Classical: The historical biotype, now rare but still present in some regions.
- These biotypes are further divided into subtypes based on their O antigen structure:
- Ogawa: Contains antigens A and B.
- Inaba: Contains antigens A and C.
- Hikojima: Contains all three antigens (A, B, C).
- Hikojima strains can be agglutinated by both Ogawa and Inaba antisera, while Ogawa and Inaba strains are agglutinated only by their specific antisera.
- Non-O1 V. cholerae strains:
- These strains do not agglutinate with O1-specific antisera, earning them the name noncholera vibrios or nonagglutinating vibrios.
- They are classified into 138 serotypes ranging from O2 to O139.
- V. cholerae O139 is the only non-O1 serotype that causes cholera in humans. The other non-O1 serotypes do not lead to cholera.
Phage Typing
- Phage typing helps classify V. cholerae into different types based on susceptibility to bacteriophages.
- V. cholerae O1 (biotype Classical) is divided into five types based on sensitivity to four specific phages (I, II, III, IV).
- V. cholerae O1 (biotype Eltor) is divided into six types, based on reactions with the same phages, plus an additional fifth phage.
Biotyping
- Biotyping of V. cholerae can also be done based on fermentation patterns of sugars such as mannose, sucrose, and arabinose.
- This classification method, first proposed by Heiberg in 1934, places V. cholerae into Group I of the fermentation classification.
- Over time, additional groups have been added, but V. cholerae remains a member of Group I based on its fermentation profile.
Virulence Factors of Vibrio cholerae
The virulence factors of Vibrio cholerae are essential to the bacterium’s ability to infect and cause cholera. These factors work together to disrupt normal intestinal function, promote adhesion to the intestinal mucosa, and enhance the pathogen’s survival within the host.
Key Virulence Factors:
- Cholera Toxin:
- The cholera toxin (CT) is a central player in the pathogenesis of cholera. It consists of two subunits: CTxA (active) and CTxB (binding).
- Biological Functions:
- The A1 subunit of the toxin stimulates the production of cyclic adenosine monophosphate (cAMP) in the host’s intestinal epithelial cells.
- This results in the conversion of ATP to cAMP, which disrupts ion transport, leading to the inhibition of sodium and chloride absorption and the activation of chloride excretion.
- The imbalance in ion transport causes large volumes of water to be secreted into the intestinal lumen, leading to severe diarrhea.
- Cholera toxin also increases skin capillary permeability, earning it the name permeability factor. This effect can be demonstrated through the skin bluing test in animals, where the injection of cholera toxin increases capillary permeability, turning the skin blue upon subsequent injection of Evans blue dye.
- Diagnostic Tests for Cholera Toxin:
- Rabbit ileal loop test: Injection of V. cholerae culture or filtrate into a ligated ileal loop in rabbits causes fluid accumulation, demonstrating the action of cholera toxin.
- ELISA and RIF: Methods to detect the presence of cholera toxin in samples.
- cAMP chemical estimation: Measuring cAMP levels in cells exposed to cholera toxin.
- Toxin Coregulated Pilus (TCP):
- TCP is a type IV pilus that is crucial for the adhesion of V. cholerae to the mucosal cells of the intestines. Without this pilus, the bacteria would struggle to establish infection in the host.
- Accessory Colonization Factor (ACF):
- This factor aids in the adhesion of V. cholerae to the intestinal mucosa, promoting colonization and persistence in the gut.
- Hemagglutination-Protease (HAP or Mucinase):
- This enzyme, which acts as both an agglutinin and a zinc-dependent protease, plays a role in disrupting the intestinal mucus barrier.
- It cleaves mucins and fibronectin, and also aids in the release of free vibrios from the mucosal surface into the intestinal lumen.
- HAP is involved in intestinal inflammation, which helps the bacteria in maintaining its presence in the intestines.
- Neuraminidase:
- This enzyme breaks down neuraminic acid, increasing the availability of receptors for cholera toxin on the intestinal cell surface, thereby enhancing the bacterium’s ability to bind and infect the host.
- Siderophores:
- These molecules are critical for sequestering iron, a nutrient essential for bacterial growth and survival. Siderophores help V. cholerae acquire iron from the host, aiding in its virulence and persistence.
Overview of Biological Functions:
- Cholera toxin:
- Inhibits sodium and chloride absorption.
- Causes hypersecretion of water and electrolytes, leading to diarrhea.
- Increases capillary permeability.
- Toxin coregulated pilus:
- Facilitates adhesion to intestinal cells.
- Accessory colonization factor:
- Aids in bacterial adhesion to the mucosa.
- Hemagglutination-protease:
- Induces inflammation and helps release bacteria from mucosal attachment.
- Neuraminidase:
- Increases toxin receptor availability.
- Siderophores:
- Sequester iron for bacterial growth and survival.
Mode of Infection of Vibrio cholerae
The pathogenesis of cholera is complex, involving several stages and interactions between Vibrio cholerae and the host’s intestinal system. It starts when the bacteria enter the body and ultimately causes the characteristic watery diarrhea.
- Entry into the Host:
- Vibrio cholerae is typically ingested orally through contaminated water or food.
- Upon reaching the stomach, it encounters the acidic environment. Most vibrios are highly vulnerable to the stomach’s acidity, which acts as a defense mechanism.
- Reduced stomach acidity (pH above 5) increases the chances of infection, making the host more susceptible to cholera.
- Surviving the Stomach and Reaching the Small Intestine:
- V. cholerae uses its motility, chemotaxis, and production of enzymes (such as hemagglutinin and protease) to traverse through the stomach and reach the small intestine.
- In the small intestine, V. cholerae encounters the mucous layer of the gut, where it uses hemagglutinin and protease to break down mucin and fibronectin, components of the mucosal barrier.
- Adherence to Intestinal Wall:
- After disrupting the mucosal layer, the bacteria adhere to the intestinal epithelium. This is primarily facilitated by the Toxin Coregulated Pilus (TCP).
- The interaction of TCP, cholera toxin (CT), and other virulence factors is regulated by the Tox R gene, which acts as the master switch for virulence gene expression.
- Production of Cholera Toxin and Its Effect:
- Once adhered to the intestinal cells, V. cholerae begins to produce cholera toxin (CT).
- The A1 subunit of cholera toxin activates adenylate cyclase, increasing the levels of cAMP in the intestinal epithelial cells.
- The elevated cAMP levels interfere with sodium absorption and activate the chloride excretion in the intestinal cells.
- This disruption leads to the accumulation of sodium chloride in the intestinal lumen, causing an imbalance in osmolarity.
- To balance the osmotic pressure, large quantities of water are secreted into the intestinal lumen, overwhelming the absorption capacity and leading to diarrhea.
- Characteristics of Cholera Diarrhea:
- The resulting diarrhea is isotonic but contains higher concentrations of potassium and bicarbonate.
- This excessive fluid loss, combined with electrolyte imbalance, is what makes cholera a severe, life-threatening disease.
- Role of the V. cholerae O139 Strain:
- The O139 strain of V. cholerae shares similar pathogenic mechanisms with the O1 strain but has two distinct virulence factors: a unique O139 lipopolysaccharide (LPS) and an O antigen capsule.
- These factors enhance the virulence of the O139 strain, increasing its resistance to human serum.
- This enhanced resistance may sometimes lead to the development of bacteremia during infection.
Genome and Evolution of Virulence of Vibrio cholerae
Vibrio cholerae’s genome reveals a complex evolutionary story that shapes its virulence and ability to cause cholera outbreaks. This bacterium carries two nonhomologous circular chromosomes, Chr1 and Chr2, each contributing uniquely to its survival and pathogenicity.
- Chromosome Structure:
- Chr1 is the larger chromosome at 2.96 Mb with a G+C content of 47.7%. It houses essential genes for cell functions, including DNA replication, transcription, translation, and cell-wall biosynthesis. It also carries critical virulence genes that encode toxins, adhesins, and surface antigens.
- Chr2 is smaller at 1.07 Mb with a G+C content of 46.9%. It has fewer core cellular function genes but contains a significant number of mobile genetic elements (MGEs), including prophages, genomic islands (GIs), and integrative and conjugative elements (ICEs).
- Mobile Genetic Elements (MGEs):
- The genome of V. cholerae contains several MGEs that contribute to its virulence and adaptation. These elements play key roles in acquiring new traits, such as antibiotic resistance and the ability to cause disease.
- Notable MGEs include bacteriophages, genomic islands, and ICEs, which are particularly important in the strains responsible for pandemics.
- Evolution of Pandemic Strains:
- The evolution of V. cholerae’s virulence is thought to be driven by the acquisition of MGEs, a process influenced by environmental factors. A proposed evolutionary pathway suggests that a common ancestor of the seventh pandemic strains diversified through a sequence of genetic acquisitions.
- Vibrio Pathogenicity Islands (VPI): The acquisition of the O1 antigen was followed by the addition of Vibrio pathogenicity islands, specifically VPI-1 and VPI-2. These islands encode the toxin-coregulated pilus (TCP), a receptor for the CTXΦ bacteriophage, crucial for cholera toxin production.
- Biotype Divergence: The split between the Classical and El Tor biotypes happened due to the independent acquisition of distinct CTXΦ bacteriophages and additional genetic elements like the Vibrio seventh pandemic islands (VSP-1 and VSP-2). These elements are found in El Tor strains but are absent in Classical strains isolated before the 1920s.
- Horizontal Gene Transfer and Antibiotic Resistance:
- Horizontal gene transfer (HGT) has been key to the adaptation of V. cholerae, particularly in the context of antibiotic resistance. The SXT ICE, a self-transmissible element carrying multiple antibiotic resistance genes, was acquired by strains in the seventh pandemic.
- The presence of SXT ICE was a defining feature of Wave 2 and Wave 3 strains, signaling a shift in the population dynamics of V. cholerae. These strains underwent multiple CTXΦ substitutions, resulting in the emergence of new El Tor variant strains.
- Vibrio cholerae O139 Strains:
- The O139 serogroup, which emerged in the 1990s, also carries the SXT ICE, marking it as another example of genetic acquisition through horizontal gene transfer. These strains have similar genomic characteristics to the later waves of the seventh pandemic.
Pathogenesis of Vibrio cholerae
Vibrio cholerae, the bacterium responsible for cholera, follows a distinct pathogenesis involving several key steps from entry into the human body to infection and transmission. Understanding these processes highlights how the bacterium thrives and spreads in the human digestive system.
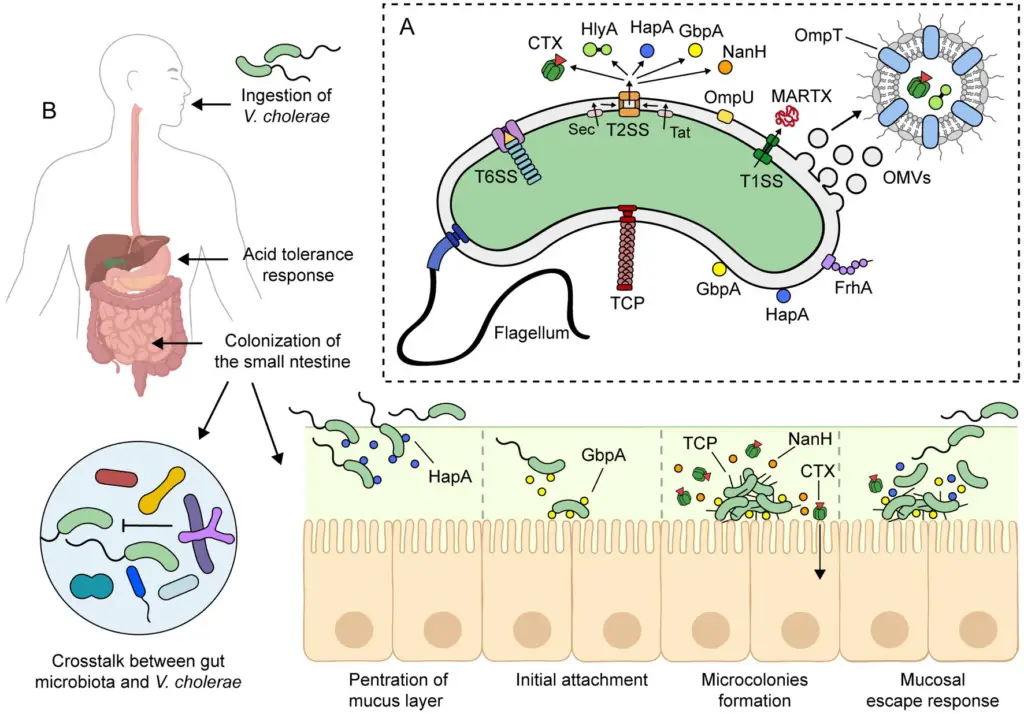
- Ingestion and Initial Adaptation
- After ingestion, V. cholerae passes through the stomach, where it faces harsh acidic conditions.
- The bacterium responds to this acidity with an acid tolerance response (ATR), aided by proteins like OmpU, CadC, and HepA.
- Despite this, a high bacterial load (around 10⁸ cells) is needed for infection in healthy individuals, with a lower dose effective when stomach acid is neutralized by antacids.
- Survival in the Small Intestine
- Upon reaching the small intestine, V. cholerae adapts to antimicrobial agents, including bile salts and antimicrobial peptides.
- The bacterium activates the ToxR regulon, adjusting its outer membrane protein (OMP) profile. This includes upregulating OmpU, which is more resistant to bile salts compared to OmpT, allowing V. cholerae to thrive in this hostile environment.
- Colonization and Mucus Penetration
- The small intestine’s mucosal layer, 100-400 μm thick, poses a challenge for colonization. V. cholerae uses its flagellum to move through this mucus layer to the epithelial surface.
- Non-motile strains are much less efficient at colonization, underscoring the importance of flagellar motility.
- Enzymes like HapA and TagA help in breaking down the mucus, enabling the bacteria to penetrate and colonize the intestinal epithelium.
- Colonization Resistance and Host Immune Evasion
- V. cholerae faces competition from the gut microbiota and host immune defenses. It combats these through its type VI secretion system (T6SS), a molecular syringe that can kill bacterial competitors, particularly those within the gut microbiota.
- Differences in the microbiota composition across individuals may explain varying susceptibilities to cholera.
- Attachment to Intestinal Epithelium
- V. cholerae attaches to intestinal epithelial cells (IECs) via GbpA, a protein regulated by quorum sensing and expressed at low bacterial density.
- GbpA binds mucin and stimulates its secretion from IECs, which further enhances GbpA expression, fostering colonization.
- Other adhesins, including OmpU and FrhA, also contribute to the bacterium’s ability to adhere to the intestinal lining.
- Toxin Production and Disease Manifestation
- After attachment, V. cholerae forms microcolonies and begins to express two key virulence factors: CTX (cholera toxin) and the toxin-coregulated pilus (TCP).
- CTX is secreted into the intestinal lumen, where it is taken up by IECs. The toxin disrupts the cells’ cAMP signaling, causing a massive efflux of electrolytes and water, leading to profuse watery diarrhea, a hallmark of cholera.
- TCP mediates adherence and microcolony formation, essential for the infection’s progression. Both CTX and TCP are regulated by environmental signals like bile and bicarbonate.
- Bacterial Shedding and Transmission
- In the later stages of infection, when nutrients are depleted, the bacteria enter a bacteriostasis phase, where they downregulate virulence factor production.
- Some bacteria undergo a mucosal escape response, detaching from the epithelial surface and becoming motile again, which facilitates further spread.
- Hyperinfectious State and Environmental Transmission
- Bacteria shed in the feces can be in a hyperinfectious state, characterized by high motility and increased infectivity. This state lasts only a few hours but plays a crucial role in cholera transmission.
- Infected individuals can shed bacteria for up to 10 days, even without symptoms, contributing to environmental contamination and the potential for new infections.
Mechanism of Action of Cholera Toxin
Cholera toxin (CTX) is a powerful virulence factor produced by Vibrio cholerae, responsible for causing the life-threatening watery diarrhea in cholera. Here’s how CTX works its damage:
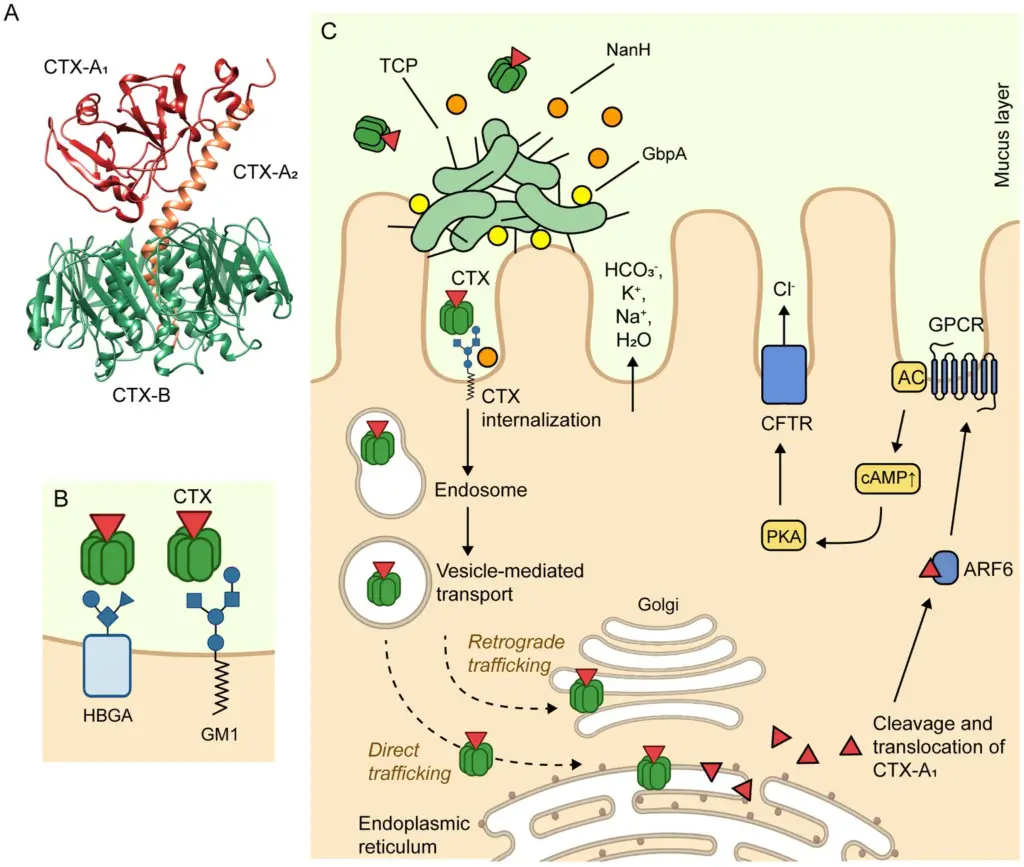
- CTX Structure
The toxin is made up of two key components:- CTX-A subunit: A heterodimer consisting of two polypeptides, CTX-A1 (22 kDa) and CTX-A2 (5 kDa), linked by a disulfide bond.
- CTX-B subunit: A pentamer composed of five identical polypeptides (11.6 kDa), which forms the binding structure of the toxin.
- Binding to Host Cells
- CTX-B binds to GM1 gangliosides, which are specific receptors located on the apical surface of intestinal epithelial cells (IECs).
- This binding can also involve histo-blood group antigens (HBGAs), providing a secondary binding site for the toxin.
- Internalization Process
Once CTX-B binds to GM1 or HBGAs, it triggers the internalization of the toxin into the IECs.- The internalization occurs through clathrin-dependent or caveolae- and clathrin-independent endocytosis pathways.
- After endocytosis, CTX is transported to the early endosome, then to the Golgi apparatus, and eventually reaches the endoplasmic reticulum (ER).
- Activation of CTX-A1
Within the ER, the CTX-A1 subunit dissociates from CTX-A2 and CTX-B.- The activated CTX-A1 then exits the ER and enters the cytosol.
- In the cytosol, CTX-A1 is activated by ADP ribosylation factor 6 (ARF6).
- Effect on Cell Signaling
The activated CTX-A1 catalyzes the ADP ribosylation of a G protein-coupled receptor (GPCR), which activates adenylyl cyclase (AC).- AC converts ATP to cyclic AMP (cAMP), significantly increasing intracellular cAMP levels.
- High cAMP levels activate protein kinase A (PKA), which phosphorylates chloride channels known as cystic fibrosis transmembrane conductance regulator (CFTR) proteins.
- Resulting Effect
- Phosphorylated CFTR chloride channels open up, leading to the efflux of chloride ions (Cl−) into the intestinal lumen.
- Along with chloride, bicarbonate (HCO3−), sodium (Na+), and potassium (K+) are secreted.
- This electrolyte imbalance causes water to flood into the lumen, resulting in the secretory diarrhea characteristic of cholera.
Immune Response to Cholera
Cholera infection triggers a multi-layered immune response, involving both innate and adaptive components, to fight off Vibrio cholerae. This pathogen induces a short-term protective immunity for most patients who recover, typically lasting around three years. The immune response to cholera is complex and varies depending on factors such as age, nutritional status, co-infections, and even blood type. Understanding this immune reaction is critical in the context of both natural infection and vaccination efforts. Below is a breakdown of the immune system’s response during cholera infection.
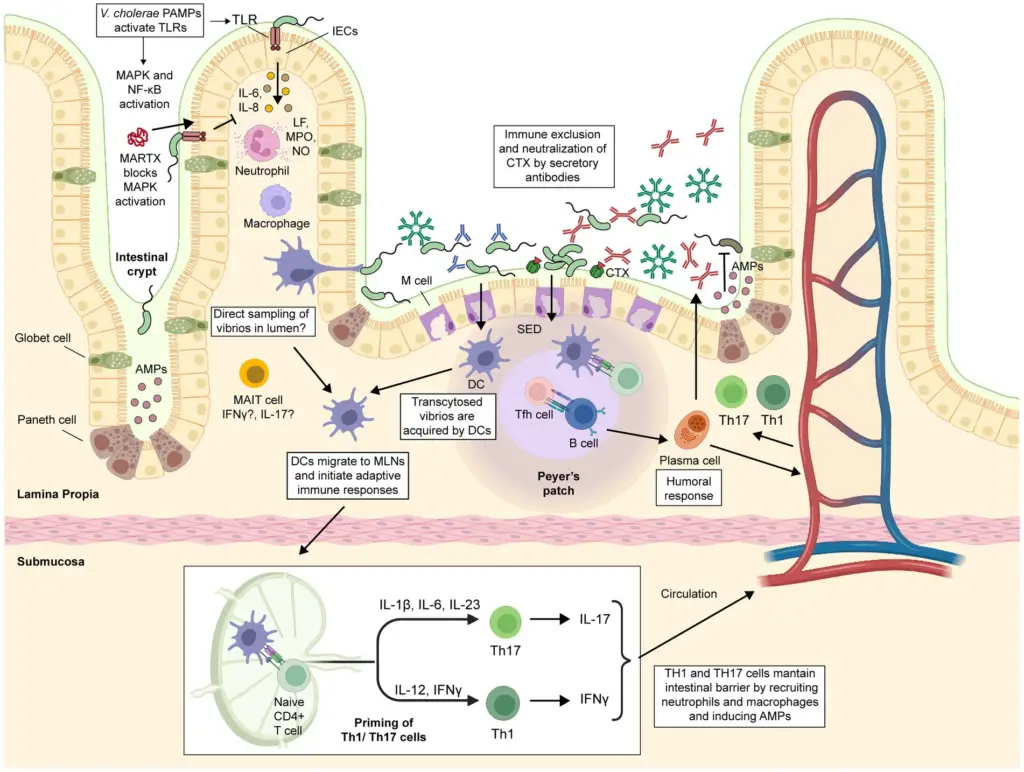
Innate Immune Response
- Initial Infection Phase:
- Cholera has traditionally been seen as a non-inflammatory disease, but it’s now known to induce some degree of inflammation, particularly during the acute phase of infection.
- Changes in the duodenal mucosa, like widening of intracellular spaces, occur, accompanied by immune cell infiltration, suggesting an inflammatory response.
- Intestinal epithelial cells (IECs) act as the first line of defense by creating a physical barrier and releasing cytokines upon detecting V. cholerae components like LPS, flagellins, and CTX.
- Recognition of these pathogen-associated molecular patterns (PAMPs) by pattern recognition receptors (PRRs) on IECs activates critical immune signaling pathways like MyD88, MAPK, and NF-κB.
- Cytokines such as IL-6, IL-8, IL-1β, and CCL2 are released to recruit neutrophils, monocytes, and dendritic cells to the site of infection.
- Key Cytokine Roles:
- IL-8 attracts neutrophils and activates phagocytosis.
- IL-6 helps neutrophil activation and degranulation.
- CCL2 recruits monocytes, dendritic cells, and memory T-cells to the infection site.
- Other immune cells, such as MAIT cells, are activated, possibly assisting in antibody production at the mucosal surface.
- Neutrophils and Their Role:
- Once neutrophils reach the infection site, they release metabolites like lactoferrin (LF), myeloperoxidase (MPO), and nitric oxide (NO), which are known to help kill V. cholerae.
- Elevated levels of these substances are detected in cholera patients, and LF, in particular, plays a significant role in killing the bacteria.
- Immune Evasion Mechanisms by V. cholerae:
- V. cholerae can evade the host’s immune response by secreting MARTX toxins that block key immune pathways, such as the MAPK pathway, preventing neutrophil recruitment.
- This suggests that immune responses, while crucial for defense, could also be manipulated by the bacteria to enhance their survival.
Adaptive Immune Response
- Humoral Immunity:
- The body produces specific antibodies against cholera antigens like CTX and O-specific polysaccharides (OSP), including IgM, IgG, and secretory IgA (sIgA).
- These antibodies can neutralize CTX, block bacterial adhesion, and aid in the clearance of V. cholerae by opsonizing the bacteria for immune cell attack.
- Vibriocidal antibodies are particularly important, as they mediate bacterial killing, especially in the bloodstream.
- However, while high vibriocidal antibody titers (VAT) are protective, they are not always sufficient to prevent reinfection, as some individuals with high VAT still get infected.
- T Cell-Mediated Immunity:
- In the acute phase, cholera infection induces Th1 (IFN-γ) and Th17 (IL-17) responses, which are key for the body’s defense against mucosal pathogens.
- The Th17 response is crucial for managing bacterial infections at mucosal surfaces, and this response plays a major role in fighting cholera.
- Follicular helper T (Tfh) cells are also activated during infection and play a vital role in the formation of germinal centers, promoting antibody production and the development of memory B cells.
- Memory Cells and Long-Term Immunity:
- After recovery, the body retains memory B cells and gut-homing T cells, which can rapidly respond to future V. cholerae infections.
- These memory cells maintain long-term immunity, with gut-homing CD4+ T cells and IgA antibodies serving as a key part of mucosal protection.
- IgA antibodies can neutralize bacteria directly at the mucosal surface, preventing V. cholerae from adhering to the intestinal epithelium.
- Complement and Antibody-Dependent Immunity:
- Antibodies can activate complement, aiding in bacterial clearance, but this process is less effective at the mucosal level due to low complement activity in the intestine.
- IgG antibodies, while important, might enter the intestinal lumen either via passive leakage or through active transport mechanisms (e.g., FcRn), though the exact mechanism is still under investigation.
Clinical Syndromes of Cholera
Cholera is an acute diarrheal illness caused by Vibrio cholerae, characterized by severe dehydration and electrolyte imbalance. The condition progresses rapidly and can be fatal without timely treatment.
Key Clinical Features:
- Incubation Period:
- The incubation period is short, usually ranging from 2 to 3 days after ingestion of contaminated water or food.
- Main Symptoms:
- Watery Diarrhea: The most prominent symptom of cholera is profuse, watery diarrhea, which can be extremely severe. In severe cases, patients may lose up to 250 mL per kg of body weight in stool each day.
- Vomiting: Often begins early in the disease and is associated with decreased gastric and intestinal motility.
- Abdominal Cramps: Severe abdominal cramping, often linked to the distension of the small intestine from fluid loss, is commonly seen.
- Cholera Stool Characteristics:
- Colorless and Odorless: The stool is typically watery, resembling the consistency and color of water washed off from cooked rice, known as “rice water stool.”
- Lack of Inflammatory Response: The stool contains few leukocytes, with no erythrocytes, as V. cholerae does not trigger significant inflammation in the intestinal mucosa.
- Electrolyte Imbalance: The stool is bicarbonate-rich, containing little protein.
- Rapid Onset of Dehydration:
- Dehydration develops very quickly, often within hours of the onset of symptoms. This rapid loss of fluids is a hallmark of cholera, setting it apart from other diarrheal diseases.
- The loss of fluids and electrolytes can lead to hypovolemic shock, metabolic acidosis, and hypokalemia.
- Severe Complications:
- Hypovolemic Shock: As dehydration progresses, shock may occur due to a significant drop in blood volume.
- Cardiac Arrhythmia: Electrolyte imbalances, particularly low potassium levels, can lead to dangerous irregular heart rhythms.
- Renal Failure: Due to severe dehydration and the associated loss of circulatory volume, kidney failure can develop.
- Electrolyte Imbalance: As a result of the massive fluid loss, the body experiences significant electrolyte disturbances that need immediate correction.
- Hypoglycemia: In some cases, a drop in blood sugar can occur, further complicating the condition.
- Case Fatality Rate:
- In untreated patients, the case fatality rate can range from 25% to 50%.
- With prompt and adequate treatment—especially fluid and electrolyte replacement—the fatality rate drops to less than 1%.
- V. cholerae O139:
- The O139 strain of V. cholerae causes a clinical presentation similar to the O1 strain but may occasionally lead to bacteremia, an invasive form of the disease that can complicate the infection.
Reservoir, Source, and Transmission of Cholera Infection
Cholera is primarily spread through water and humans, with both playing significant roles in the ongoing transmission cycle. Humans serve as the primary reservoirs, with certain groups contributing more to the spread of the disease.
Reservoirs of Cholera Infection
- Humans:
- Both chronic and convalescent carriers are crucial in spreading the infection. These individuals continue to excrete V. cholerae intermittently in their stool for several years.
- Chronic carriers are particularly important, as they may not show symptoms but can still transmit the bacteria.
- Water:
- Contaminated water serves as a primary reservoir. V. cholerae thrives in water that has been exposed to fecal contamination.
- Cholera is often prevalent in areas with poor sanitation and limited access to safe drinking water.
- Animals:
- Animals do not play a role in the transmission of cholera. The bacteria are not transmitted through animals.
Source of Infection
- Food and Water Contamination:
- The primary source of infection is the ingestion of contaminated food or water.
- Cholera outbreaks typically occur in communities with low hygiene standards, where clean water and sanitation systems are inadequate.
- Infective Dose:
- The infective dose of V. cholerae is relatively high, usually more than 106 organisms per milliliter of water or food. Most organisms are killed by stomach acid, so only a large number of bacteria can overcome this barrier.
- Conditions like achlorhydria, where the stomach lacks sufficient acid, increase susceptibility to infection, as the bacteria can survive and infect the person more easily.
Transmission Mechanism
- Ingestion:
- The infection is most commonly transmitted when contaminated water or food is ingested. This is why the disease is widespread in places with poor water treatment systems.
- Direct Contact:
- Direct person-to-person transmission is rare. Cholera typically spreads through the consumption of contaminated food or water rather than through direct contact with an infected person.
- Vulnerability:
- People of all ages are susceptible to cholera. However, infants may have some protection against the infection due to maternal antibodies passed through breastfeeding.
Laboratory Diagnosis of Cholera Infection
Diagnosing cholera involves several laboratory methods to identify Vibrio cholerae in clinical samples. The process begins with specimen collection and continues through various tests to confirm the presence of the pathogen.
Specimen Collection
- Stool Samples:
- The preferred specimen is fresh stool, ideally collected before any antibiotic treatment is given.
- Sterile soft rubber catheter (24–26 size) can be used to collect stool, or liquid stool can be placed directly in a screw-capped container.
- Avoid using bedpans as they risk contamination or contain disinfectants that may interfere with the sample.
- Rectal swabs are useful when collecting samples from convalescent patients, especially those no longer passing watery stools.
- Vomitus is not a recommended specimen for diagnosis.
- If there is any delay in transport, samples should be kept at 4°C or placed in transport media such as alkaline peptone water or Monsur’s taurocholate transport media.
- For longer delays, Cary–Blair medium or VR fluid can preserve the sample.
- In the absence of transport media, watery stool can be soaked in blotting paper and sent in a plastic envelope.
Microscopy
- Dark-field Microscopy:
- A useful method to demonstrate the motility of V. cholerae and its reaction to antisera.
- This is a rapid method of examining stool samples collected either directly or after 6 hours of enrichment.
- Direct Immunofluorescence:
- A rapid alternative to dark-field microscopy for visualizing vibrios in stool.
- Gram Stain:
- A Gram-stained smear is not recommended for cholera diagnosis, as it does not reliably identify V. cholerae.
Culture
- Enrichment:
- Specimens should be inoculated into enrichment media (like alkaline peptone water) and incubated for 6–8 hours before transferring to selective and non-selective media.
- Selective Media:
- Thiosulfate-citrate-bile salts-sucrose (TCBS) agar is used for selective growth. V. cholerae produces yellow colonies on this medium.
- Non-selective Media:
- Other media, such as MacConkey agar, BSA, and blood agar, are also used. V. cholerae produces non-lactose-fermenting colonies on MacConkey agar.
- Incubation:
- Plates should be incubated at 37°C overnight. After incubation, the colony appearance helps identify V. cholerae.
Bacterial Identification
- Biochemical Tests:
- A series of biochemical tests are performed to identify V. cholerae. These include:
- Oxidase test
- Amino acid utilization (lysine, ornithine, arginine)
- Sugar fermentation
- Sheep RBC hemolysis
- Polymyxin B sensitivity
- Serotyping is performed using specific polyvalent antisera to confirm the bacterial strain.
- A series of biochemical tests are performed to identify V. cholerae. These include:
- Slide Agglutination:
- V. cholerae O1 can be identified using slide agglutination with specific antisera. The agglutination of bacterial colonies indicates a positive result.
- Inaba and Ogawa antisera are used to distinguish between V. cholerae serotypes.
- Biotyping:
- If agglutination is positive, the next step is biotyping to differentiate between the Classical and El Tor biotypes. Tests include:
- Hemolysis of sheep RBCs
- Chick RBC agglutination
- Sensitivity to polymyxin B
- VP test
- Bacteriophage sensitivity
- If agglutination is positive, the next step is biotyping to differentiate between the Classical and El Tor biotypes. Tests include:
- Non-O1 Strains:
- Vibrio isolates not agglutinated by V. cholerae O1 antisera are identified as non-O1 cholera vibrio. These are tested with H antigen antisera and, if positive, may be tested for O139 using specific antisera.
Carrier Diagnosis
- Repeated Stool Cultures:
- V. cholerae is excreted intermittently by carriers, making repeated stool cultures essential for diagnosis.
- After using purgatives like mannitol or magnesium sulfate, stool collection is often more successful for detecting V. cholerae in carriers.
- Bile Collection:
- Bile collected by duodenal intubation may also help diagnose carriers, as it can reveal V. cholerae even when stool cultures are negative.
Serodiagnosis
- Complement-Dependent Vibriocidal Antibody Assay:
- This test measures antibodies against V. cholerae and is used mainly for seroepidemiological studies.
- Antitoxin assays also help detect vibrio antibodies in serum.
- These tests are not typically used for diagnosing active cases but are useful for tracking exposure and immunity.
Treatment of Cholera Infection
Cholera treatment primarily focuses on fluid and electrolyte replacement and, to a lesser extent, antibiotic therapy. Proper management is crucial to avoid complications like hypovolemic shock and to ensure a quick recovery.
Fluid and Electrolyte Replacement
- Key Treatment Strategy:
- The cornerstone of cholera treatment is fluid replacement, especially before severe dehydration sets in.
- Oral rehydration therapy (ORT) is the most effective and widely used approach to replace fluids.
- Oral Rehydration Therapy (ORT):
- The ORT solution consists of glucose, sodium chloride, potassium chloride, and sodium citrate.
- Glucose helps absorb sodium in the small intestine, while the salts correct the electrolyte imbalance and reverse acidosis.
- This solution is cost-effective and simple to use, making it highly successful in regions where cholera is common.
- Cereal-based Preparation:
- Rice soup, when thoroughly cooked and salted, works as an alternative to ORT, providing hydration and aiding recovery.
- Intravenous Fluids:
- For more severe cases, especially in the presence of intense dehydration, intravenous fluid therapy is often necessary to rehydrate the patient quickly.
Antibiotic Therapy
- Secondary Role of Antibiotics:
- Antibiotics are not the primary treatment for cholera but are used to shorten the illness and reduce fluid loss.
- They also help clear the Vibrio cholerae bacteria from the intestine faster.
- Common Antibiotics:
- Vibrio cholerae is generally sensitive to:
- Tetracycline
- Ciprofloxacin
- Erythromycin
- Vibrio cholerae is generally sensitive to:
- Treatment Based on Age and Condition:
- For adults, tetracycline or doxycycline is the drug of choice.
- For children, trimethoprim-sulfamethoxazole is commonly prescribed.
- Pregnant women are usually treated with pyrazolidone.
- Antibiotic Resistance:
- Recent reports indicate ciprofloxacin-resistant strains have emerged, particularly in areas like Kolkata, India. This underscores the need for continuous monitoring and appropriate antibiotic selection.
Prevention and Control of Cholera Infection
Preventing and controlling cholera involves multiple strategies, with a strong focus on hygiene, water safety, and early intervention. The main approaches to combat cholera can be grouped into general preventive measures and vaccination.
General Preventive Measures
- Early Identification and Case Management:
- Recognizing cholera cases quickly and managing them effectively can reduce the spread and severity of the disease.
- Immediate treatment helps prevent complications like dehydration and hypovolemic shock.
- Improved Water Supply and Sanitation:
- Contaminated water is a primary source of cholera transmission. Ensuring access to clean, safe drinking water is critical.
- Proper waste disposal and sanitation systems help prevent the bacteria from contaminating water sources.
- Personal Hygiene:
- Good hygiene practices, such as handwashing with soap, significantly reduce the risk of cholera.
- Avoiding the consumption of uncooked food and unboiled water also plays a major role in preventing infection.
- Health Education:
- Educating communities about cholera transmission and prevention methods is essential, especially in high-risk areas.
- Awareness campaigns can inform people about the importance of safe drinking water and proper food handling.
Cholera Vaccination
- Cholera Vaccines:
- Vaccination is another crucial step in cholera prevention. There are oral cholera vaccines available that can provide protection, particularly in high-risk areas.
- While vaccination is effective, it should complement other preventive measures, as no single strategy is enough to prevent outbreaks on its own.
FAQ
What is Vibrio cholerae food poisoning?
Vibrio cholerae food poisoning, commonly known as cholera, is a bacterial infection caused by the ingestion of food or water contaminated with the Vibrio cholerae bacterium. It leads to severe watery diarrhea and dehydration.
What are the symptoms of Vibrio cholerae food poisoning?
The symptoms of Vibrio cholerae food poisoning include sudden onset of profuse, watery diarrhea often described as “rice water” stools, vomiting, rapid dehydration, muscle cramps, and electrolyte imbalances.
How does Vibrio cholerae contaminate food?
Vibrio cholerae bacteria can contaminate food through the use of contaminated water during food preparation or processing. Seafood, particularly shellfish, is a common source of contamination as Vibrio cholerae naturally inhabits marine environments.
How is Vibrio cholerae food poisoning diagnosed?
Diagnosis is typically based on clinical symptoms and a history of possible exposure to contaminated food or water. Laboratory tests, such as stool culture or rapid diagnostic tests, may be used to confirm the presence of Vibrio cholerae.
What is the treatment for Vibrio cholerae food poisoning?
Treatment primarily involves oral or intravenous rehydration therapy to replace lost fluids and electrolytes. Antibiotics may also be prescribed to shorten the duration of symptoms and reduce the spread of the bacteria.
Can Vibrio cholerae food poisoning be prevented?
Yes, Vibrio cholerae food poisoning can be prevented through proper sanitation and hygiene practices, such as using safe drinking water, practicing good hand hygiene, thoroughly cooking food, and avoiding raw or undercooked seafood.
What are the high-risk foods for Vibrio cholerae contamination?
High-risk foods for Vibrio cholerae contamination include raw or undercooked seafood, particularly shellfish like oysters, clams, and mussels. These seafood items can become contaminated if harvested from contaminated waters.
Can Vibrio cholerae food poisoning be transmitted from person to person?
While person-to-person transmission is rare, it can occur in certain situations, especially in overcrowded or unsanitary conditions. The primary mode of transmission is through the ingestion of contaminated food or water.
Is Vibrio cholerae food poisoning common in developed countries?
Vibrio cholerae food poisoning is more commonly reported in low-income, developing, and underdeveloped countries where access to clean water and proper sanitation may be limited. Developed countries generally have better infrastructure and hygiene practices that minimize the risk.
What should I do if I suspect I have Vibrio cholerae food poisoning?
If you suspect you have Vibrio cholerae food poisoning, it is important to seek medical attention promptly. Treatment with rehydration therapy and antibiotics, if necessary, can help alleviate symptoms and prevent complications.
- Mandal, S., & Mandal, M. (2014). VIBRIO | Vibrio cholerae. Encyclopedia of Food Microbiology, 708–716.
- Cholera. StatPearls [online]. Available at: https://www.ncbi.nlm.nih.gov/books/NBK470232/
- Foodborne Pathogenic Vibrios: Antimicrobial Resistance. PMC [online]. Available at: https://www.ncbi.nlm.nih.gov/pmc/articles/PMC8278402/
- Vibrio cholerae Infection. StatPearls [online]. Available at: https://www.ncbi.nlm.nih.gov/books/NBK526099/
- Cholera, Vibrio cholerae O1 and O139, and Other Pathogenic Vibrios. NCBI [online]. Available at: https://www.ncbi.nlm.nih.gov/books/NBK8407/
- Vibrio cholerae – A guide to Food poisoning. Medic8 [online]. Available at: https://www.medic8.com/healthguide/food-poisoning/vibrio-cholerae.html
- Morris, J. G. (2017). Cholera and Other Vibrioses. International Encyclopedia of Public Health, 1–8.
Venkateswaran, K. (1999). VIBRIO | Standard Cultural Methods and Molecular Detection Techniques in Foods. Encyclopedia of Food Microbiology, 2248–2258. - Jones, J. L. (2014). VIBRIO | Introduction, Including Vibrio parahaemolyticus, Vibrio vulnificus, and Other Vibrio Species. Encyclopedia of Food Microbiology, 691–698.
- Baker-Austin, C., Oliver, J.D., Alam, M. et al. Vibrio spp. infections. Nat Rev Dis Primers 4, 1–19 (2018). https://doi.org/10.1038/s41572-018-0005-8.
- Meltzer, E., & Schwartz, E. (2007). Cholera: A Travel History of the First Modern Pandemic. Travel Medicine, 287–298.
- Finkelstein RA. Cholera, Vibrio cholerae O1 and O139, and Other Pathogenic Vibrios. In: Baron S, editor. Medical Microbiology. 4th edition. Galveston (TX): University of Texas Medical Branch at Galveston; 1996. Chapter 24. Available from: https://www.ncbi.nlm.nih.gov/books/NBK8407/
- https://www.frontiersin.org/journals/medicine/articles/10.3389/fmed.2023.1155751/full
- https://www.sciencedirect.com/topics/medicine-and-dentistry/vibrio-cholerae