Plasmids are small circular molecules of DNA that exist independently of chromosomal DNA within a cell. Because they replicate independently, these molecules are called replicons. The plasmids have an origin of replication known as the ori site from which replication begins.
Plasmids encode a few of their own proteins, but when it comes to replication they rely on a mix of both. Although plasmids code for few (or even one) proteins and only those necessary for initiating replication at the ori site, the host cell provides the others. Essential enzymes such as DNA polymerases, helicases, primases, and ligases required for synthesizing and elongating new DNA consist of them.
Different functional sites are present in the ori region of plasmids. For example, oriV (origin of vegetative replication) supports normal replication of plasmid DNA, and oriT (origin of transfer) initiates DNA transfers with processes like conjugation. These two roles enables the plasmids to carry out their important functions for their maintenance and replication in the cellular unit.
These replication and transfer mechanisms allow replication and transfer of plasmids, which is why plasmids are vital in molecular biology and genetics. Vectors are simply them for manipulating or introducing outside genes into host cells providing a broad tool for analyzing gene expression and function; they are widely used in genetic engineering and biotechnology.
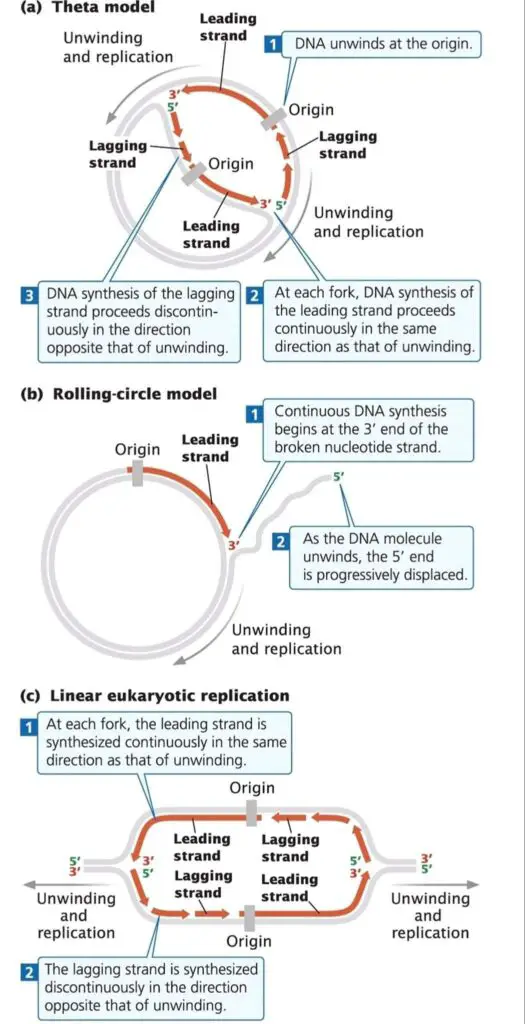
1. Rolling circle replication
Rolling circle replication (RCR) is an unidirectional type of nucleic acid replication that quickly produces multiple copies of circular DNA or RNA. In this way this is typically seen in plasmids, bacteriophage genomes, viroids and some eukaryotic viruses wherein they utilize this for replication of genetic material. The mechanism is characterized by its simplicity and swift synthesis, allowing for rapid in vitro amplification of genetic material.
The mechanism itself starts with the introduction of a single-stranded nick in the circular DNA molecule by a specific endonuclease. This nick reveals a free 3′-hydroxyl, and provides a primer for DNA polymerase. By extending the 3′ end, the polymerase begins replication, displacing the original strand as a single-stranded piece of DNA. This ejected strand might neatly fold back onto itself, or it might act as a template for the synthesis of a complementary strand, and in this manner concatamers, or long, linear DNA molecules, are produced.
In addition to its natural presence, rolling circle replication has been developed into an isothermal DNA amplification method, named rolling circle amplification (RCA). RCA enables fast, isothermal amplification of nucleic acids that can thus be applied in a variety of molecular biology and biomedical research settings.
Due to they are simplicity and efficiency this mechanism has drawn attention in several area of biosensing and nanotechnology. RCA utilizes its capacity to produce massive numbers of nucleic acids, which is harnessed by researchers for pathogen detection, genetic material analysis, and novel molecular device engineering.
Steps of Rolling circle replication
Rolling circle replication is a unidirectional DNA replication mechanism observed in various plasmids and bacteriophages. This method begins with circular double-stranded DNA (dsDNA) and results in the production of multiple copies of the genetic material. Here are the detailed steps of the process:
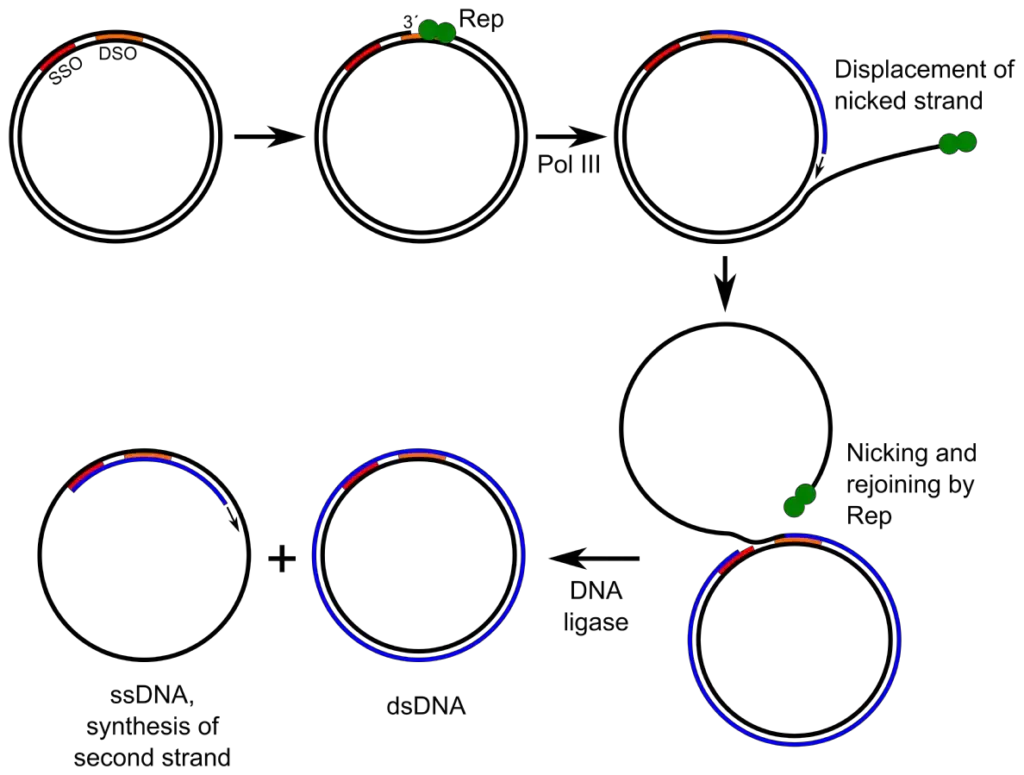
- Initiation and Nick Formation
The replication process begins at a specific origin of replication site known as the double-strand origin (DSO). A replication initiation protein, commonly referred to as the Rep protein, binds to the DSO. This protein, which contains an active tyrosine residue, introduces a nick into one strand of the dsDNA. The nick creates two ends: a 5′ end with a phosphate group and a 3′ end with a hydroxyl group. The Rep protein remains bound to the 5′ phosphate, while the free 3′ hydroxyl serves as the primer for DNA synthesis. - DNA Synthesis on the Leading Strand
DNA polymerase III utilizes the free 3′ hydroxyl group to initiate synthesis, using the intact (unnicked) strand as the template. This elongation proceeds unidirectionally around the circular DNA, resulting in the displacement of the nicked strand. The displaced strand remains single-stranded as the synthesis progresses. - Strand Separation and Lagging Strand Synthesis
A helicase enzyme, either host-encoded or part of the plasmid’s replication machinery, facilitates the separation of the nicked strand. The displaced single-stranded DNA (ssDNA) forms a lagging strand, which will later be converted into double-stranded DNA (dsDNA). Host-encoded machinery produces the complementary strand through the synthesis of Okazaki fragments. - Termination and Circularization of Displaced Strand
Once replication of the leading strand completes a full circle, the Rep protein catalyzes a phosphotransferase reaction. This transfers the 5′ phosphate from the tyrosine on the Rep protein back to the 3′ hydroxyl end of the DNA, resealing the nick. The displaced ssDNA forms a circular molecule and serves as the template for further replication. - Replication of the Single-Stranded DNA
The single-stranded origin (SSO) within the displaced DNA is recognized by host RNA polymerase, which synthesizes a primer. DNA polymerase III extends this primer to form a complementary strand, completing the synthesis of a double-stranded circular DNA. Subsequently, DNA polymerase I removes the RNA primer and replaces it with DNA. DNA ligase seals the final nicks, creating a fully functional dsDNA molecule. - Formation of Multiple Copies
This replication mechanism can produce a concatemer—a continuous head-to-tail series of ssDNA molecules—if replication continues without termination. These concatemers can later be processed into individual circular dsDNA molecules.
Key Features
- Unidirectional Process: The synthesis proceeds in a single direction around the circular template.
- Efficiency: The rolling circle mechanism enables the rapid production of multiple copies of plasmid DNA.
- Host Interaction: Host enzymes such as RNA polymerase, DNA polymerase, and helicase are critical for certain steps, particularly in converting ssDNA to dsDNA.
2. Theta Model of Replication
Common mechanism used by prokaryotes to replicate their circular DNA molecules, including plasmids, theta plasmid replication, also known as theta mode of replication. It is named for the intermediate structure that forms during replication and resembles the Greek letter theta (θ).
Unlike eukaryotes, whose DNA is linear and has multiple replication origins, most prokaryotes have circular DNA that multiplies in fusion around a single origin, the ori. All of the strands are called daughter strand DNA, while the original strands are called parent strand DNACurrently, helicase enzymes cleave the hydrogen bonds holding the two complementary strands of parental DNA together at the ori, thus generating a replication bubble sorted into two segments, each composed of an individual strand of DNA. This unwinding, exposes single-stranded templates for DNA synthesis.
DNA polymerase catalyzes the formation of new DNA strands, extending in the 5′ to 3′ direction. Thus, the replication forks grow around the circular DNA molecule in two opposite directions, creating the sinkilar and leading strands at the same time. In this manner, the DNA while repeating process begins to take the characteristic theta-like shape, which is a distinguishing feature of this mode of replication.
When the replication forks collide on the other side of the circular DNA, ligase enzymes are used to seal up any remaining nicks in the sugar-phosphate backbone so that the newly synthesized DNA is intact. This leads to two identical daughter DNA molecules, with each new molecule containing one strand of the parental DNA and one newly synthesized strand (as per the semi-conservative theory of DNA replication).
Plasmids and other small circular DNA molecules adopt this mode of replication, which is efficient and compatible with their maintenance in bacteria. It is a basic process fundamental to molecular biology and provides a model system to understand the mechanisms of DNA replication.
Steps of Theta Model of Replication
The theta model of replication is a well-studied process in bacterial DNA replication, particularly in organisms with circular chromosomes, such as Escherichia coli. This model involves the formation of a structure resembling the Greek letter theta (θ), which gives the process its name. The replication occurs in a bidirectional or unidirectional manner, and the steps are as follows:
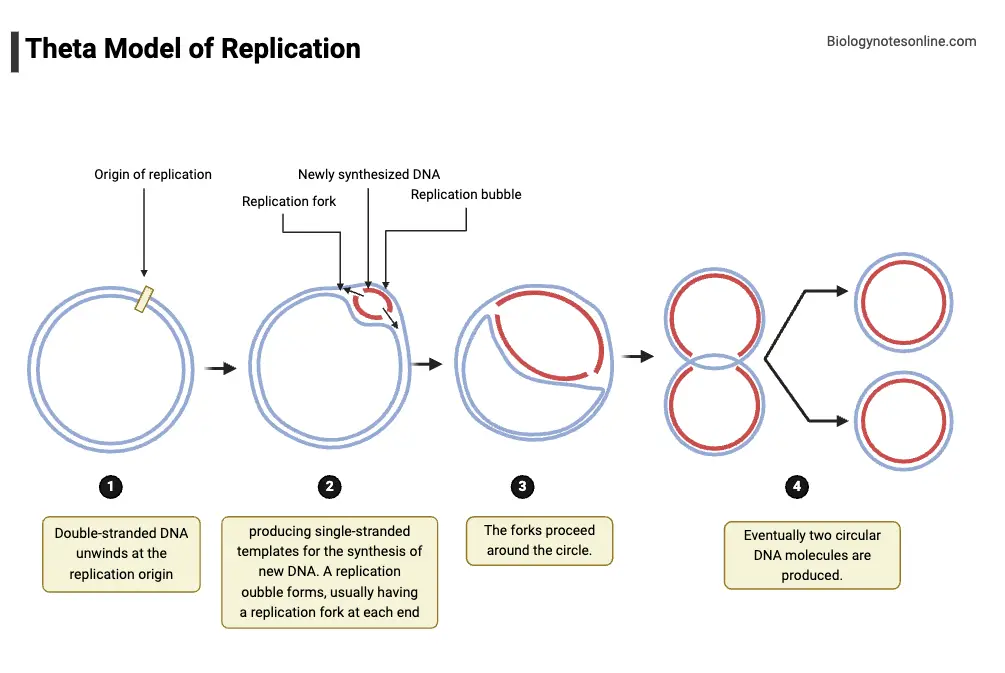
- Initiation at the Origin of Replication
Replication begins at a specific region called the origin of replication, where the double-stranded DNA (dsDNA) undergoes denaturation. The denaturation of the DNA creates single-stranded DNA (ssDNA) regions, collectively referred to as the replication bubble. This bubble is the site where the replication machinery assembles. - Formation of the Replication Fork
As the DNA unwinds, a Y-shaped structure, known as the replication fork, is formed. This structure is essential for the continuation of replication and is generated by the primasome—a protein complex consisting of several key enzymes. These enzymes include DnaG primase, DnaB helicase, and DnaC helicase assistant, among others. The helicase enzymes are responsible for unwinding the parental DNA strands, allowing for the replication to proceed. - Bidirectional Replication
In most cases, replication occurs bidirectionally, meaning that two replication forks form at the origin and move in opposite directions around the circular DNA molecule. As the forks advance, they synthesize new DNA strands in a semiconservative manner, meaning each new strand is composed of one original template strand and one newly synthesized strand. This bidirectional movement continues until the replication forks meet at a point diametrically opposite the origin. - Unwinding and Topoisomerase Activity
During replication, the parental double helix must unwind to allow the replication machinery access to the DNA. This unwinding introduces torsional strain in the DNA, which is resolved by the enzyme topoisomerase. Topoisomerase alleviates this strain by creating transient breaks in the DNA, allowing it to rotate and relieve the supercoiling before resealing the breaks. - Completion and Separation
Once replication is complete, the two newly synthesized daughter DNA molecules are separated. This occurs after the replication forks have met, and the entire circular DNA has been replicated. The process ensures that each daughter molecule receives a copy of the original genetic material, maintaining the integrity of the genome.
Key Characteristics of Theta Replication
- Bidirectional Process: Replication typically proceeds in both directions from the origin, generating two replication forks.
- Replication Bubble: The denaturation of the dsDNA creates a bubble, providing access for the replication machinery.
- Primasome Complex: A group of proteins that aids in the formation of the replication fork and facilitates the synthesis of RNA primers.
- Topoisomerase Function: Ensures the unwinding of the DNA is smooth and free from tension that could inhibit replication.
3. Replication of Linear Double-Stranded DNA (Bidirectional Replication)
The replication of linear double-stranded DNA (dsDNA) is a fundamental process employed by all cellular organisms and certain DNA viruses, including nuclear dsDNA viruses and bacteriophages. This method occurs within the host cell’s nucleus in eukaryotes and in the cytoplasm in prokaryotes. The process is bidirectional, meaning replication proceeds outward in two directions from a single origin of replication.
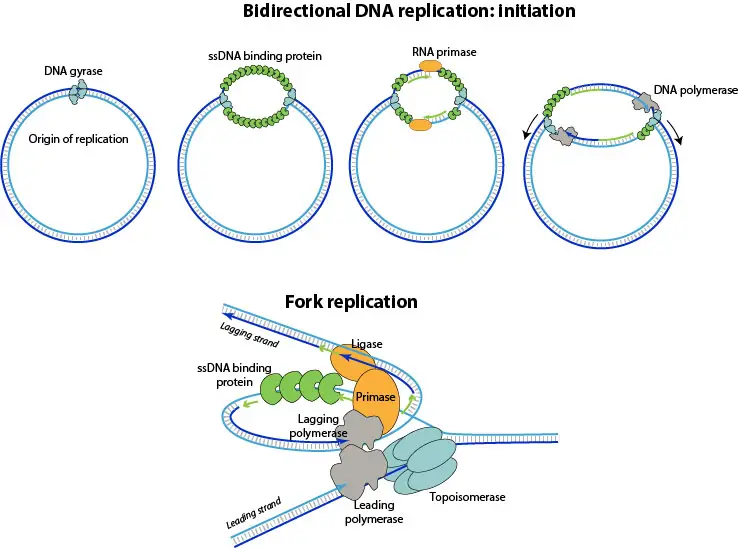
- Initiation at Origins of Replication
Replication begins at specific genomic regions called origins of replication. These sites are recognized by initiator proteins, which recruit the necessary replication machinery. At the origin, topoisomerase enzymes unwind the DNA to relieve torsional strain and allow access to the double helix. - Formation of the Replication Bubble
The unwinding of DNA generates a replication bubble, exposing single-stranded DNA (ssDNA) templates. These exposed strands are stabilized by ssDNA-binding proteins, which prevent reannealing and protect the DNA from nucleases. - Primer Synthesis
RNA primers are synthesized by a primase enzyme to provide the free 3′-hydroxyl groups necessary for DNA polymerase to initiate DNA synthesis. These primers are essential for replication of both the leading and lagging strands. - Elongation of DNA Strands
DNA polymerase begins elongation by synthesizing the leading strand continuously in the 5′ to 3′ direction using the exposed template strand. On the lagging strand, DNA synthesis occurs discontinuously, producing short segments called Okazaki fragments. Each fragment is initiated by an RNA primer synthesized by primase. - Processing of Okazaki Fragments
The RNA primers on the lagging strand are removed, and DNA polymerase fills in the resulting gaps with complementary DNA. DNA ligase then seals the nicks between Okazaki fragments, creating a continuous DNA strand. - Progression and Termination
The replication forks advance bidirectionally until they either meet at the opposite ends of the linear genome or at a termination site in circular genomes. This progression ensures complete duplication of the genetic material. - Separation of Daughter Molecules
After synthesis is complete, topoisomerase enzymes resolve the interlinked daughter DNA molecules. This step is critical for separating the newly synthesized DNA strands to prepare them for cell division.
Key Features of Bidirectional Replication
- Bidirectional Forks: Two replication forks form at the origin and move in opposite directions, enabling efficient replication of the entire genome.
- Leading and Lagging Strands: Continuous synthesis occurs on the leading strand, while discontinuous synthesis involving Okazaki fragments occurs on the lagging strand.
- Role of Topoisomerase: Prevents overwinding ahead of the replication fork and resolves daughter DNA molecules after replication.
4. Replication of the 5′ End of Linear Chromosomes (Telomere Replication)
Linear chromosomes, a common feature in eukaryotic organisms, present unique challenges during DNA replication, particularly at their 5′ ends. After DNA replication, the newly synthesized DNA strands are shorter at the 5′ end compared to the parental DNA strand. This results in a 3′ overhang on the daughter strands, with the overhangs situated at opposite ends of the chromosomes. This phenomenon occurs due to the inability of DNA polymerase to fully replicate the ends of linear chromosomes.
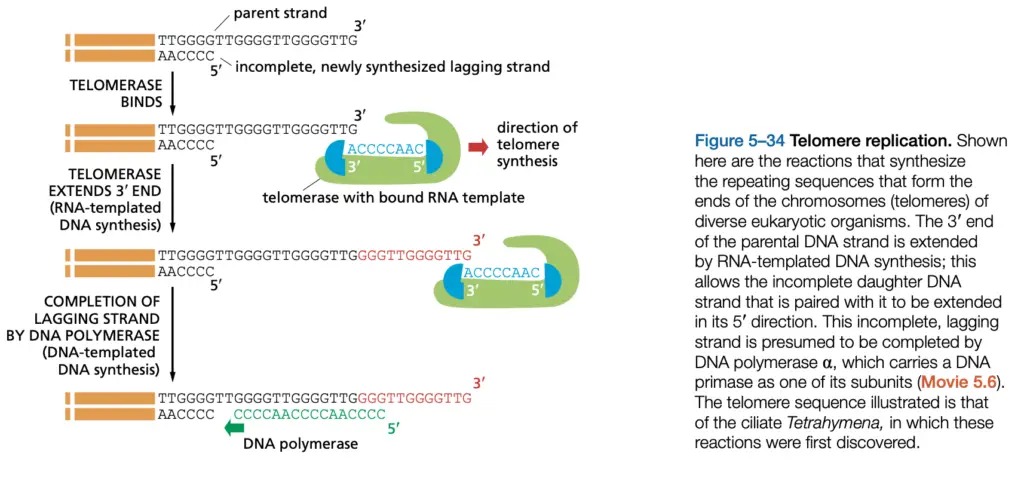
- The End Replication Problem
During the replication process, the RNA primer required for initiating DNA synthesis on the lagging strand is removed and replaced by DNA. However, the RNA primer at the 5′ end of the newly synthesized strand cannot be replaced by DNA. Enzymes such as RNase H and FEN1 facilitate the removal of RNA primers, but DNA polymerase is unable to extend the strand in the 5′ direction because no template strand exists for it to extend. This results in the production of a 3′ overhang, which shortens the DNA with each replication cycle. - Loss of Genetic Material
If this issue is not addressed, it leads to progressive shortening of the chromosome with each replication cycle, ultimately resulting in the loss of genetic material. As a result, the linear chromosomes would gradually shrink, potentially leading to the loss of essential genes and compromising cellular function. - Telomeres and Their Function
To counteract this shortening, eukaryotic cells possess specialized structures at the ends of their chromosomes called telomeres. Telomeres are repetitive nucleotide sequences that protect the chromosomes from degradation and prevent the loss of coding DNA during replication. They act as buffers, ensuring that the vital genetic information in the coding regions of the chromosomes remains intact after multiple rounds of cell division. - The Role of Telomerase
Telomerase, an enzyme complex, plays a crucial role in maintaining telomere length. Telomerase adds repetitive DNA sequences to the 3′ overhang, extending the telomeres and compensating for the loss of DNA during replication. This process is particularly important in germ cells, stem cells, and certain types of cancer cells, where the maintenance of telomere length is essential for prolonged cellular division. - Replication of Linear Chromosomes in Bacteria and Plasmids
Linear plasmids and bacterial chromosomes also face challenges during replication. For example, linear plasmids may have hairpin ends, where the 5′ and 3′ ends are covalently joined. This configuration helps prevent the recognition of these ends as DNA double-strand breaks, thus protecting them from exonucleases. In some systems, linear plasmids may utilize a terminal protein attached to the 5′ ends to help initiate replication. - Resolution of the Telomere Problem
The replication of the 5′ end of linear chromosomes is a highly regulated process involving both structural features like telomeres and enzymes like telomerase. By addressing the “end replication problem,” these mechanisms ensure that linear chromosomes can be faithfully replicated without losing vital genetic information over time.
- https://www.onlinebiologynotes.com/mechanism-of-plasmid-replication-theta-and-rolling-circle-dna-replication/
- https://biology.stackexchange.com/questions/112036/telomerase-and-end-replication-in-eukaryote
- https://oertx.highered.texas.gov/courseware/lesson/1679/student-old/?task=2
- https://viralzone.expasy.org/1939