What is Transgenic Animal?
- A transgenic animal is an organism whose genome has been deliberately modified by the introduction of foreign genes. These foreign genes, known as transgenes, come from an external source, and are artificially inserted into the animal’s DNA. This process, referred to as “transgenesis,” allows the transgenes to be inherited by future generations and function within the host organism’s genome.
- The transgenes can either be introduced to add new functions or to disable (knock out) an existing gene. In many cases, the goal is to study gene function, disease mechanisms, or improve specific traits in animals. Advancements in molecular biology and genetic engineering have enabled the development of various transgenic animals, including mice, livestock, fish, and even primates. These animals serve as valuable models for studying gene regulation, cancer development, and immune responses, and they are also used to explore the genetic basis of diseases.
- The first transgenic animal, a mouse, was developed in 1974 by Rudolf Jaenisch, marking a significant milestone in biotechnology. Over time, the process became more refined, leading to the development of genetically modified animals capable of passing on the transgene to their offspring. By the early 1980s, transgenic mice were being used to study a variety of human genes, including oncogenes, which are involved in cancer development.
- Transgenic technology has not only contributed to basic research but also holds commercial and therapeutic applications. For example, genetically modified livestock can be designed to produce valuable proteins in their milk, such as human tissue plasminogen activator (tPA), which is used to treat blood clots. The technology has expanded to include a wide range of animals, including fish, pigs, and goats, all of which have been engineered to possess traits beneficial to human medicine and agriculture.
Definition of Transgenic Animal
A transgenic animal is an organism that has had a foreign gene (transgene) deliberately inserted into its genome, allowing the gene to be expressed and passed on to future generations.
Steps for Production of Transgenic Animals
The production of transgenic animals involves a detailed and systematic approach to ensure the successful incorporation and expression of foreign genes. This process comprises several essential steps, each critical to achieving the desired genetic modifications.
- Construction of a Transgene:
- Promoter: The transgene is initiated with a promoter sequence that regulates gene expression. This element ensures that the introduced gene is activated in specific tissues or at designated developmental stages.
- Gene to be Expressed: This is the actual gene of interest that will impart new traits or functions to the animal. The choice of gene is fundamental for the intended outcomes.
- Termination Sequence: This sequence marks the end of the transcription process, ensuring that gene expression occurs accurately and within appropriate limits.
- Introduction of the Foreign Gene into the Animal:
- Pronuclear Microinjection Method: In this technique, the transgene is directly injected into the pronucleus of a fertilized egg. This allows for immediate integration into the animal’s genome, facilitating the creation of a genetically modified organism.
- Embryonic Stem Cell Method: Alternatively, the transgene can be introduced into embryonic stem cells. These modified cells are subsequently implanted into a host embryo. This method provides a more controlled integration process and the potential to produce chimeric animals, which contain both genetically modified and non-modified cells.
- Screening for Transgenic Positives:
- Polymerase Chain Reaction (PCR): After introducing the transgene, PCR is employed to identify successful integrations. This technique amplifies specific DNA sequences, enabling researchers to confirm the presence and integration site of the foreign gene.
- Integration Site Considerations: It is crucial to recognize that not all integrations will lead to functional gene expression. Transgenes may integrate into transcriptionally inactive regions, resulting in silencing and failure to express the desired traits.
- Further Animal Breeding:
- Breeding Strategies: The final step involves breeding the successfully modified animals. Heterozygous offspring, which possess one copy of the transgene, are selectively bred to produce homozygous strains containing two copies. This increases the likelihood of stable and consistent expression of the introduced traits across generations.
Methods for Introduction of the Foreign Gene into the Animal
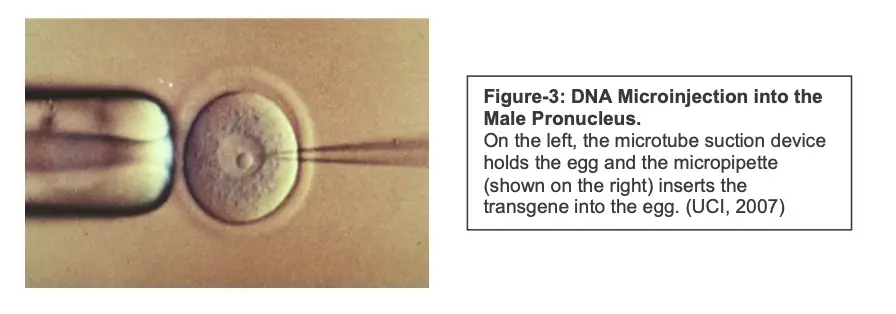
1. DNA Microinjection Method
DNA microinjection is a foundational technique in the field of genetic engineering, particularly for the creation of transgenic animals. This method involves the precise introduction of foreign DNA into the pronucleus of a fertilized embryo, enabling the integration of the transgene into the animal’s genome. The following sections detail the key steps, advantages, limitations, and applications of this technique.
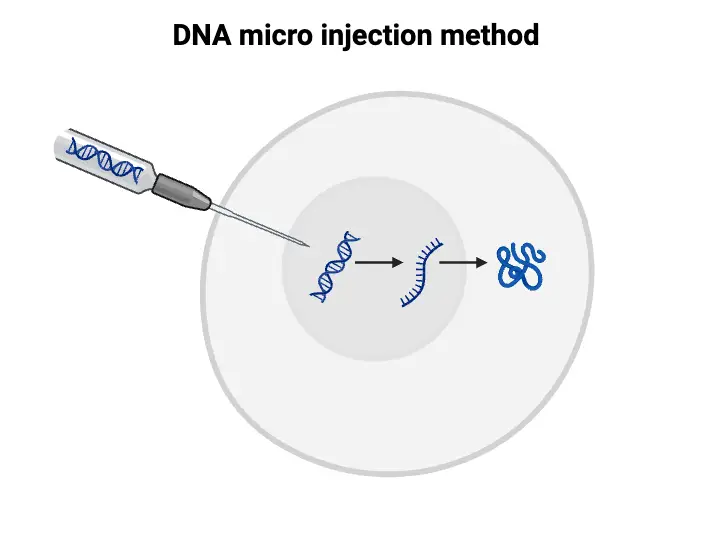
Key Steps in the DNA Microinjection Process
- Egg Collection and Fertilization:
- Eggs are harvested from a donor female, typically through a controlled surgical procedure.
- The collected eggs are fertilized in vitro using sperm from a male. This step allows for meticulous control over the fertilization process and is essential for subsequent stages.
- Microinjection into Male Pronucleus:
- Following fertilization, the injected DNA is introduced into the male pronucleus of the zygote. The male pronucleus, originating from the sperm, is identifiable before it merges with the female pronucleus.
- A fine microsyringe is employed to deliver the transgene accurately. This stage is crucial, as it occurs prior to the zygote’s first division, facilitating the integration of the foreign DNA into the host genome.
- The integration of the transgene is random, which can lead to variability in expression and functionality.
- Zygote Implantation:
- After microinjection, the zygote is cultured until it reaches the blastocyst stage, approximately five days post-fertilization. This stage is characterized by a hollow sphere of cells.
- The developing embryo is then implanted into the uterus of a pseudopregnant female, which has been hormonally prepared to support embryo development following mating with a sterile male.
- Screening for Transgenic Offspring:
- Once the offspring are born, they are screened to verify whether the transgene has been successfully incorporated into their genomes. Techniques such as Polymerase Chain Reaction (PCR) are commonly utilized for this purpose.
- Given the random nature of transgene integration, not all offspring will express the transgene, necessitating careful screening to identify successful transgenics.
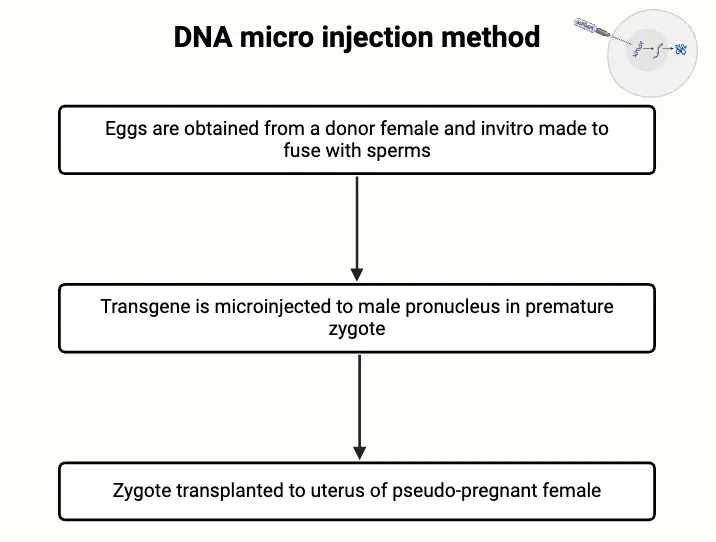
Advantages and Limitations
Advantages:
- Versatility: This method is applicable to a wide range of species, including mice, rats, sheep, goats, pigs, cows, and fish.
- Simplicity: The DNA microinjection process is relatively straightforward and has successfully produced numerous transgenic models, contributing significantly to genetic research.
Limitations:
- Random Integration: The transgene integrates at random locations in the genome, which may result in poor expression or integration into transcriptionally inactive regions.
- Labor and Cost Intensity: The procedure can be labor-intensive and expensive, particularly in species with slower reproductive cycles or limited embryo availability, such as certain livestock.
Applications
The DNA microinjection method is crucial for various aspects of genetic research and biotechnology:
- Gene Function Studies: It enables researchers to explore gene functions across different species.
- Model Organisms: Transgenic models created through this method are instrumental in studying genetic diseases, developmental biology, and more.
- Pharmaceutical Production: The technique supports the development of transgenic livestock for pharmaceutical applications, potentially producing valuable therapeutic proteins.
2. The Retroviral Vector Method
The retroviral vector method is a widely employed technique for generating transgenic animals, utilizing the natural ability of retroviruses to integrate their genetic material into a host organism’s genome. This method offers an efficient means of transferring genes, making it a valuable tool in genetic research and biotechnology. Below is a detailed explanation of the key steps, applications, advantages, and limitations of this technique.
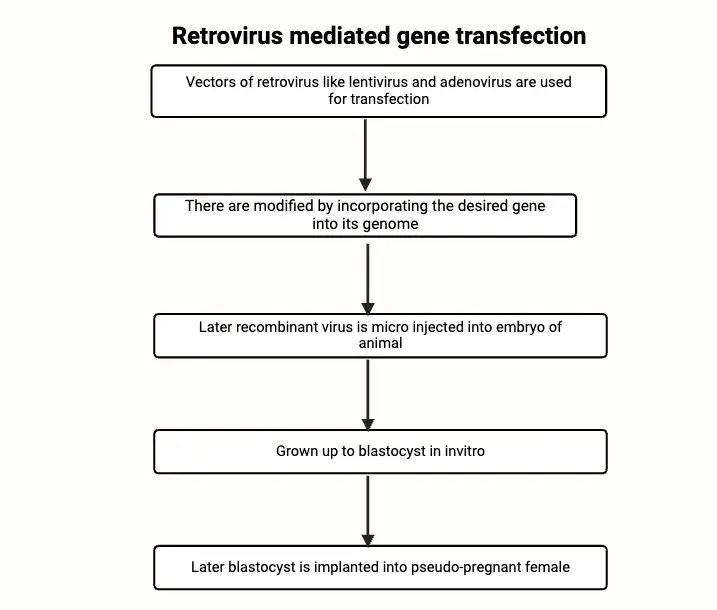
Key Steps in the Retroviral Vector Method
- Retrovirus as a Vector:
- Retroviruses possess RNA genomes and rely on an enzyme known as reverse transcriptase to convert their RNA into DNA within a host cell. Once this conversion occurs, the viral DNA is integrated into the host genome, making retroviruses effective vectors for inserting transgenes into target organisms.
- Modification of the Retrovirus:
- The retrovirus is genetically modified by incorporating the desired transgene into its genome. This results in a recombinant virus that can deliver the transgene to the host cells.
- Infection of Embryonic Cells:
- The modified retrovirus is introduced at the eight-cell stage of the developing embryo. Infection at this stage allows the virus to integrate its genetic material into the embryo’s cells, including future germline cells. The infected embryos are then cultured to reach the blastocyst stage.
- Implantation into Foster Mothers:
- After reaching the blastocyst stage, the embryos are transferred into the uterus of a pseudopregnant female, which has been hormonally prepared for embryo implantation. If successful, the foster mother will give birth to transgenic offspring.
- Screening and Confirmation:
- After birth, the progeny are screened to verify the presence of the transgene. Techniques such as Polymerase Chain Reaction (PCR) are commonly used to confirm successful gene transfer. Additionally, phenotypic analysis can be conducted to observe any changes caused by the transgene.
- If the transgene is present in the offspring’s germline cells, these animals can be bred to produce homozygous transgenic lines, ensuring stable inheritance of the introduced gene.
Applications and Successes
- The retroviral vector method has been successfully applied to various species, including mice and pigs. It is particularly useful in species where rapid gene transfer is desired.
- Lentiviruses, a type of retrovirus, are often used because they can infect both dividing and non-dividing cells, such as neuronal and muscle cells, broadening the scope of research involving non-replicating cell types.
Advantages
- Efficient Gene Integration: Retroviruses are highly effective at integrating genetic material into host cells, making them a reliable method for producing transgenic animals.
- Targeting Germline Cells: The infection of early-stage embryos increases the likelihood that the transgene will be incorporated into the germline, enabling stable transmission to future generations.
Limitations of the Retroviral Method
- Transgene Size:
- Retroviruses have a limited capacity for carrying genetic material, typically restricting the size of the transgene to around 8 kilobases. This constraint can limit the complexity of genetic modifications that can be introduced.
- Risk of Contamination:
- There is a potential risk of contamination by helper viruses during the process. These helper viruses can integrate into the host genome alongside the transgene, particularly concerning for applications in food-producing animals.
- Transgene Silencing:
- In certain instances, the transgene introduced via a retroviral vector may become silenced. This is especially common in mouse embryos, where transgenes integrated into inactive regions of the genome may not be expressed.
- Chimerism:
- Initially, the progeny produced through this method may be chimeric, meaning that not all cells carry the transgene. This requires additional breeding over several generations to establish stable transgenic lines where all cells carry the modification.
Example of Use
The retroviral vector method was first used to create transgenic mice in 1974, marking a significant milestone in genetic research. Since then, it has been applied to various other species, including sheep, pigs, and cattle, allowing researchers to explore gene function and develop transgenic animals for agricultural and pharmaceutical purposes.
3. Embryonic Stem Cell Method
The embryonic stem (ES) cell method is a key technique used in genetic engineering, particularly for producing transgenic animals. It leverages the unique properties of pluripotent stem cells, which can differentiate into almost any cell type, including those contributing to the germline. This ability makes the method highly valuable for studying genetic diseases, developmental biology, and gene function. Below is an exploration of the key steps, applications, and limitations of this technique.
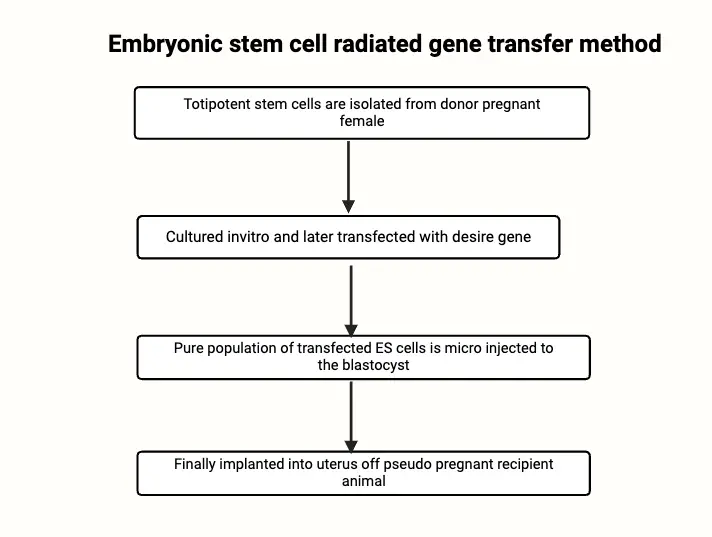
Key Steps in the Embryonic Stem Cell Method
- Isolation of Embryonic Stem Cells:
- The process begins by collecting fertilized ova from a donor animal, typically after inducing superovulation. The fertilized ova are cultured until they reach the blastocyst stage, which is an early stage of embryonic development. From the blastocyst, the inner cell mass (ICM) is harvested. The ICM contains pluripotent ES cells, which are then isolated and cultured in vitro.
- Transgene Introduction into ES Cells:
- Once the ES cells are cultured, they are transfected with a desired transgene. This transgene is introduced using a DNA vector, designed to ensure the gene integrates at a specific, non-disruptive site in the genome. The goal is to avoid integration in regions that could interfere with essential genes, especially those involved in development or vital cellular functions.
- Selection and Culture of Transfected ES Cells:
- Following the transfection, the ES cells undergo selection to isolate those that have successfully incorporated the transgene. The selected transfected ES cells are then expanded in culture to produce a larger population of genetically modified ES cells for further experimentation.
- Microinjection into Blastocysts:
- The genetically modified ES cells are injected into blastocysts from another strain of animals, often one that has a distinguishable coat color to facilitate identification later on. These injected blastocysts are then implanted into the uterus of a pseudopregnant foster mother to allow the embryo to develop.
- Chimeric Offspring and Breeding:
- The progeny born from this procedure are often chimeric, meaning they contain a mixture of normal and genetically modified cells. Some of the modified ES cells contribute to the development of adult tissues, including the germline. By breeding these chimeras, researchers can ensure that the transgene is passed to the next generation, producing stable transgenic lines in the offspring (G1).
Applications of the ES Cell Method
- Modeling Human Diseases:
- One of the most significant applications of the ES cell method is creating animal models that mimic human diseases. Researchers can introduce disease-related genes into an animal’s genome, providing a platform for studying the molecular mechanisms underlying various conditions, such as cancer, diabetes, and neurodegenerative diseases.
- Gene Knockout Studies:
- The method is also critical in gene knockout studies, where specific genes are deliberately inactivated or “knocked out.” This approach allows scientists to observe the effects of losing a particular gene function, which is essential for understanding the role of specific genes in development, physiology, and disease.
Limitations
- Chimerism:
- A significant limitation of this technique is the production of chimeric offspring. These animals consist of both genetically modified and unmodified cells, making it necessary to breed them further to achieve homozygous transgenic lines in which all cells carry the desired genetic modification.
- Low Efficiency:
- The efficiency of generating fully transgenic animals using this method is often low. Chimerism and the randomness of transgene incorporation mean that additional breeding and screening are required to produce animals with stable germline transmission of the transgene.
Example Species
- Mice:
- The ES cell method is most frequently used in mice, where it has transformed genetic research by enabling the production of gene-targeted transgenic models for studying a wide range of biological phenomena.
- Rats:
- Though less common, the method has also been applied to rats, with successes in creating transgenic models to study behavior, physiology, and genetic regulation.
4. Other Methods of Transgenesis
In addition to the more widely known techniques like the embryonic stem cell method and retroviral vector method, several other methods for genetic modification have been developed. These approaches offer alternatives for introducing or manipulating genes within animal models and are employed based on the desired outcome, species, and limitations of other methods. Below are some of these alternative transgenesis techniques:
a. Transposon-Mediated Gene Transfer
- Description: Transposons are small DNA sequences that have the ability to move within the genome using a “cut-and-paste” mechanism. When used as vectors, transposons carry a desired gene and can be microinjected into embryos of animals to integrate the gene into the progeny’s genome.
- Function: The primary function of transposons is to facilitate gene transfer by hopping from one genomic location to another.
- Limitations: One significant limitation of this method is that transposons do not integrate completely into the genome, which can result in incomplete or unstable gene transfer.
- Examples:
- Zebra fish
- Chicken
- Cattle
b. Somatic Cell Nuclear Transfer (SCNT)
- Description: In somatic cell nuclear transfer, an oocyte is used, which has been arrested in meiosis II of the cell cycle, specifically at metaphase or telophase. The oocyte is reprogrammed with the nucleus of a somatic cell, and an electric shock is applied to stimulate the cell cycle and initiate embryonic development.
- Function: This method allows the nucleus of a differentiated somatic cell to direct the development of an embryo, creating a genetically identical clone.
- Limitations: SCNT has significant drawbacks, primarily due to the stress on both the egg cell and the somatic nucleus. This stress results in a low efficiency of successful cell reprogramming and development.
- Examples:
- Dolly the sheep (the first mammal cloned through SCNT)
c. Sperm-Mediated Gene Transfer (SMGT)
- Description: In this technique, mature spermatozoa are allowed to bind with exogenous transfected DNA. The DNA-bound sperm is then injected into the cytoplasm of the oocyte through a process called intracytoplasmic sperm injection (ICSI).
- Function: SMGT offers a means for introducing a transgene into an organism via sperm-mediated delivery.
- Limitations: The success of this technique is heavily reliant on the sperm’s ability to bind to the DNA without losing its viability, which can be a limiting factor.
- Examples:
- Cattle
- Pigs
d. Testis Cell Implantation
- Description: In this method, the testis is removed from a donor male and is transfected with the desired gene. The transfected cells are cultured and then microinjected into the lumen of the seminiferous tubules in the recipient animal.
- Function: This technique is used to introduce a transgene directly into the spermatogenic cells of the recipient male, potentially passing the modified gene to future generations.
- Limitations: The method involves complex procedures and is dependent on the proper integration and functioning of the gene in the recipient’s germline cells.
- Examples: No specific animal examples are highlighted, but this method has been explored in various species for genetic research.
e. RNA Interference (RNAi)
- Description: RNA interference (RNAi) is a technique that uses small interfering RNA (siRNA) molecules to silence specific genes. The siRNA has a complementary sequence to the target mRNA, allowing it to bind covalently. This complex is recognized by the Argonaute protein, which then destroys the mRNA, thereby preventing the gene from being expressed.
- Function: The main function of RNAi is to silence specific genes in order to study their function or reduce the expression of harmful genes.
- Examples:
- Salt-sensitive rats, where RNAi has been used to investigate gene function in hypertension studies.
Examples of Transgenic Animals
The following examples illustrate the diverse applications of transgenic animals across different species.
- Transgenic Fish
- Superfish (Transgenic Salmon):
- Purpose: Engineered for accelerated growth.
- Method: A gene responsible for producing growth hormones is inserted into fertilized eggs.
- Outcome: Transgenic salmon can achieve growth rates approximately 10 to 11 times faster than non-modified salmon.
- Transgenic Zebra Fish (Danio rerio):
- Purpose: Utilized for research and as aquarium pets.
- Method: A fluorescent protein gene derived from jellyfish is integrated into the embryos.
- Function: These fish exhibit fluorescence, facilitating studies in developmental biology.
- Superfish (Transgenic Salmon):
- Transgenic Mice
- Alzheimer’s Mouse:
- Purpose: Employed to investigate the mechanisms of Alzheimer’s disease.
- Method: An amyloid precursor gene is introduced into fertilized mouse eggs.
- Outcome: These mice develop symptoms akin to human Alzheimer’s patients, including amyloid plaques in the brain.
- Oncomouse:
- Purpose: Serves as a model for cancer research.
- Method: Oncogenes are activated through genetic modification to predispose the mice to cancer.
- Function: This model is pivotal for studying cancer biology and testing potential therapies.
- Smart Mouse:
- Purpose: Engineered to enhance cognitive abilities.
- Method: Overexpression of the NR2B receptor occurs in synaptic pathways.
- Outcome: These mice exhibit improved learning capabilities throughout their lifespan.
- Alzheimer’s Mouse:
- Transgenic Pigs
- Phytase-Expressing Pig:
- Purpose: Improves the digestion of phytate, a common feed component.
- Method: The phytase gene from E. coli is introduced, allowing for the production of phytase in salivary glands.
- Function: This enzyme facilitates the breakdown of indigestible phytate, enhancing nutrient absorption.
- Pigs for Organ Transplant:
- Purpose: Aimed at reducing organ rejection in human transplant scenarios.
- Method: Human genes are integrated into the pig genome.
- Outcome: These pigs may produce organs that are less likely to be rejected by the human immune system.
- Phytase-Expressing Pig:
- Transgenic Livestock
- Cattle:
- Purpose: Designed to produce therapeutic proteins in milk.
- Method: Genes for lactoferrin and interferons are inserted into the genome.
- Function: This enhances the medicinal properties of milk, providing a cost-effective production method.
- Sheep:
- Purpose: To improve wool quality and production.
- Notable Example: Dolly, the first cloned mammal, was created through somatic cell nuclear transfer in 1996, illustrating the potential of cloning in biotechnology.
- Goats:
- Purpose: Express tissue plasminogen activator and antithrombin III in milk.
- Function: These proteins have significant therapeutic applications, making goat milk a valuable source of pharmaceuticals.
- Cattle:
- Transgenic Rabbits
- Alba:
- Purpose: Created as a transgenic artwork.
- Method: The Enhanced Green Fluorescent Protein (EGFP) gene is introduced.
- Function: Alba serves as a visual representation of the possibilities of genetic engineering.
- Alba:
- Transgenic Monkeys
- Andi:
- Purpose: The first transgenic monkey, born in 2000.
- Method: A harmless gene for green fluorescent protein (GFP) is inserted into the genome using an engineered virus.
- Outcome: Andi’s creation signifies that transgenic primates can be produced, contributing to the understanding of gene expression in higher mammals.
- Andi:
Assays Used for Screening Transgenic Animals
Below is a detailed examination of the primary techniques utilized in this screening process.
- Southern Blot Test
- Purpose: This assay analyzes genetic patterns in DNA to assess the presence, integrity, and arrangement of transgenes within the animal’s genome.
- Functionality:
- Determines the number of copies of the transgene integrated into the genome.
- Identifies the chromosomal locations of transgene insertions.
- Validates the structural integrity of the transgene.
- Procedure:
- The assay is conducted before the transgenic offspring reach six weeks of age.
- A restriction enzyme is selected to cut the transgene at specific sites, producing DNA fragments.
- These fragments undergo size separation via gel electrophoresis, where DNA migrates towards the positive electrode.
- Heating or chemical treatment denatures the DNA, enabling hybridization with a labeled probe specific to the transgene.
- Detection of a hybridized band confirms successful integration of the transgene.
- Western Blot Test
- Purpose: This technique is utilized to detect proteins produced from the transgene, thereby confirming expression at the protein level.
- Functionality:
- Employs antibodies specific to the transgenic protein to identify its presence in tissue extracts.
- Procedure:
- Protein extracts from transgenic animals are subjected to gel electrophoresis and subsequently transferred to a membrane.
- An antibody specific to the trans-protein is introduced, binding to any proteins present.
- The appearance of a band on the blot indicates that the trans-protein is being synthesized in the analyzed tissues.
- Real-Time (Reverse Transcriptase) PCR
- Purpose: This method screens for the expression of the transgene by quantifying mRNA levels, providing insight into gene transcription.
- Functionality:
- Measures the transcription of the transgene by detecting its corresponding mRNA.
- Procedure:
- Tissue samples, such as brain, pancreas, or liver, are collected to extract cytoplasmic mRNA.
- The mRNA is reverse-transcribed into complementary DNA (cDNA), followed by the degradation of residual mRNA.
- Fluorescent primers amplify the cDNA, enabling real-time quantification of transcription levels.
- Results are analyzed using agarose gel visualization under UV light, confirming the presence of the transgene transcript.
- Enzyme-Linked Immunosorbent Assay (ELISA)
- Purpose: This quantitative assay detects transgenic proteins in biological samples, such as blood or serum, allowing for measurement of protein expression levels.
- Functionality:
- Measures the concentration of trans-proteins, providing a quantitative assessment of their expression.
- Procedure:
- Wells of a plastic tray are coated with antibodies specific to the trans-protein.
- Samples are introduced, allowing the trans-protein to bind to the coated antibodies.
- A secondary antibody, conjugated with an enzyme (e.g., alkaline phosphatase or horseradish peroxidase), is added to detect the captured proteins.
- The formation of color in the wells correlates with the concentration of trans-protein; greater color intensity indicates higher amounts of the protein present.
Classification of Transgenic Animals
Transgenic animals can be classified into five distinct categories based on their intended purposes. Each classification reflects specific functional attributes and applications in scientific and agricultural contexts. Below is a detailed exploration of these categories:
- Disease Models
- Definition: Transgenic animals in this category are engineered to replicate human diseases.
- Purpose: They serve as models for studying the pathophysiology of diseases and evaluating potential treatments.
- Examples: Mouse models are frequently used to study cancer, Alzheimer’s disease, and other genetic disorders. These animals often express human genes that are implicated in specific conditions, enabling researchers to observe disease progression and test therapeutic interventions.
- Transpharmers
- Definition: This group comprises animals designed to produce pharmaceutical compounds.
- Purpose: Transpharmers are utilized in the production of proteins, antibodies, and other therapeutics that can be harvested for medical use.
- Examples: Genetically modified goats, sheep, or cows may produce milk containing valuable therapeutic proteins, such as antithrombin, which is used to treat blood clotting disorders.
- Xenotransplanters
- Definition: Xenotransplanters are transgenic animals specifically bred for organ transplantation.
- Purpose: They address the shortage of human organs available for transplant by providing genetically compatible organs from animal sources.
- Examples: Transgenic pigs are commonly researched for their potential to provide organs such as hearts and kidneys for human recipients. Genetic modifications aim to reduce immunogenicity and enhance compatibility.
- Food Sources
- Definition: This category includes transgenic animals optimized for food production.
- Purpose: These animals are engineered for traits such as enhanced growth rates, improved feed efficiency, or increased disease resistance, ultimately leading to more sustainable agriculture.
- Examples: Genetically modified salmon that grow faster than their non-transgenic counterparts represent an advancement in aquaculture, potentially meeting growing food demands while reducing environmental impacts.
- Biological Models
- Definition: Biological models are transgenic animals used for basic biological research.
- Purpose: They help elucidate fundamental biological processes, gene functions, and genetic regulation.
- Examples: Zebrafish and fruit flies are frequently modified to study gene expression, developmental biology, and genetic interactions, contributing to a greater understanding of biological systems.
Disease Models
Transgenic animals designed as disease models serve a crucial role in biomedical research. These organisms are genetically modified to express specific traits associated with human diseases, thereby facilitating the study of disease mechanisms and potential treatments without involving human subjects. Below is a detailed exploration of this category:
- Purpose and Functionality
- Study of Disease Mechanisms: Transgenic disease models enable researchers to investigate the etiology of complex diseases. By mimicking human conditions, these models provide insights into the underlying biological processes.
- Development of Therapies: Researchers can test potential therapeutic interventions in these models before proceeding to clinical trials, allowing for a safer and more ethical approach to drug development.
- Examples of Disease Models
- AIDS Mouse Models: Engineered to express genes associated with HIV, these models help in studying viral interactions and potential antiretroviral therapies.
- Alzheimer’s Disease Models: These models typically express human amyloid precursor protein (APP) mutations, allowing researchers to explore the accumulation of amyloid plaques and their effects on neurodegeneration.
- Oncomouse: This model is designed to study cancer by expressing oncogenes that lead to tumor formation, making it valuable for understanding cancer biology and testing anticancer drugs.
- Hypertension Models:
- Transgenic Mouse with Rat Renin Gene: This model expresses the rat Ren-2 gene, leading to hypertension due to overactivation of the renin-angiotensin-aldosterone system (RAAS).
- Transgenic Rat Models: By microinjecting the mouse Ren-2 gene into the oocytes of normotensive rats, researchers created heterozygous transgenic rats (TGRm Ren-2) exhibiting fulminant hypertension with systolic blood pressure exceeding 200 mmHg. These models are responsive to antihypertensive drugs such as angiotensin receptor blockers (ARBs) and ACE inhibitors.
- Diabetes Models:
- Insulin Action Studies: Transgenic models facilitate the investigation of genes involved in peripheral insulin action and insulin secretion mechanisms.
- Insulin-Dependent Diabetes Mellitus (IDDM) Model: Utilizing retroviral vectors, this model helps in understanding autoimmune diabetes and the role of genetic factors in insulin production.
- Atherosclerosis Models:
- High Susceptibility Models: These include LDL gene knockout mice and ApoE knockout mice, both of which demonstrate increased plasma cholesterol levels and are valuable for studying cholesterol metabolism and cardiovascular diseases.
- Low Susceptibility Models: Models such as LDL overexpression and ApoA knockout mice help in understanding conditions that lead to lower plasma cholesterol levels.
- Impact on Research and Medicine
- Transgenic disease models have significantly advanced our understanding of complex conditions, including hypertension, diabetes, and atherosclerosis.
- They offer a platform for testing novel therapeutic approaches and contribute to the identification of genetic risk factors associated with various diseases.
Transpharmer
A Transpharmer refers to a genetically engineered animal designed to produce human pharmaceutical proteins in their bodily fluids, such as milk, saliva, urine, or blood. This process, known as “pharming,” has transformed the field of biotechnology by offering a method to produce complex therapeutic proteins on a large scale. Below is a detailed explanation of the concept and its applications.
- Pharming and Its Evolution
- Initial Development: The history of pharming dates back to the early 1920s when insulin was extracted from pig pancreas to treat diabetes. By the 1980s, scientists began using recombinant DNA technology to produce human insulin in bacteria, marking the beginning of biopharmaceutical production through genetic engineering.
- Current Applications: Transgenic animals have since become a key tool in producing more complex proteins that require post-translational modifications, which bacteria and simpler systems cannot perform. These modifications are crucial for the stability and functionality of therapeutic proteins.
- Mechanism of Pharming
- Genetic Engineering: Transgenic animals are created by introducing human genes into their DNA through methods like microinjection or nuclear transfer. These genes encode specific proteins that the animal will then produce in their milk, egg whites, or other bodily fluids.
- Production of Recombinant Proteins (RPs): The animals express these human proteins as part of their normal biological processes. For example:
- Milk: Transgenic animals such as goats and cows can produce human antithrombin III in their milk. This protein is used as an anticoagulant to prevent blood clotting disorders.
- Egg Whites: Chickens have been engineered to produce monoclonal antibodies (mABs) and human interferons in their egg whites. These proteins are valuable in the treatment of immune disorders and viral infections.
- Examples of Pharming
- Insulin Production: Transgenic animals are used for producing insulin to treat diabetes mellitus. This method complements earlier techniques of insulin extraction and bacterial production, allowing for larger-scale production with fewer complications.
- Vaccines: Transpharmers are involved in creating vaccines by producing the necessary antigens, which help in preventing diseases.
- Growth Hormone: Animals engineered to produce human growth hormone provide an essential treatment for individuals with growth hormone deficiencies.
- Alpha-1 Antitrypsin (AAT): This protein, produced by transgenic animals, is used to treat AAT deficiency, a genetic disorder that can lead to lung and liver disease.
- Coagulation Factors: Transgenic animals produce coagulation factors essential for patients with hemophilia, offering a reliable and scalable source of these life-saving proteins.
- Lactoferrin: Produced in the milk of transgenic animals, lactoferrin is used as a nutritional supplement in infant formulas to enhance immune function.
- Challenges and Future Directions
- Regulatory Concerns: As pharming involves genetically modifying animals, it raises ethical and regulatory questions about the safety of the products and the welfare of the animals involved.
- Technological Advances: Ongoing research is focused on improving the efficiency of protein production and reducing the potential for adverse effects on the animals.
Xenotransplanters
Xenotransplanters refer to transgenic animals that are genetically modified to serve as sources for organ and tissue transplants into humans. As the demand for organ transplants far exceeds the availability of human donors, xenotransplantation offers a promising solution by using animals as organ donors. Below is a detailed exploration of xenotransplanters, their function, and the technological advancements that make them viable for medical applications.
- Definition and Function
- Genetic Modification: Xenotransplanters are animals that have undergone genetic modifications to make their organs more compatible with the human immune system. These modifications are primarily aimed at eliminating foreign antigens that could trigger immune rejection when the organs are transplanted into human recipients.
- Goal: The primary function of these transgenic animals is to serve as a sustainable and efficient alternative to human organ donors, particularly as the shortage of human organs for transplantation continues to be a critical issue in medicine.
- Pig as the Preferred Xenotransplanter
- Physiological Compatibility: Pigs are the only animals currently used for xenotransplantation. Their physiology closely resembles that of humans, particularly in terms of organ size and function, making them suitable candidates for transplanting organs like the heart, kidneys, liver, and lungs.
- Cost Efficiency: Pigs are much more cost-effective compared to other primates like monkeys. This economic advantage, combined with their faster reproduction rates, makes pigs a practical choice for ongoing research and potential clinical applications.
- Challenges with Antigen Expression
- Alpha-1,3 Galactose: One of the major barriers to xenotransplantation is the presence of a specific sugar molecule, Alpha-1,3 galactose, on the surface of pig cells. This molecule is produced by the enzyme Alpha-1,3-galactosyltransferase and is absent in humans. When pig organs are transplanted into humans, this antigen can trigger a severe immune response, leading to organ rejection.
- Genetic Solutions: To overcome this, researchers have developed GGAT1-knockout pigs, which are genetically engineered to lack the enzyme responsible for producing Alpha-1,3 galactose. These pigs show a much lower incidence of organ rejection, especially in preclinical trials involving monkeys, thus offering a significant advancement in xenotransplantation research.
- Applications in Transplantation
- Heart Valves: Porcine heart valves have already been successfully transplanted into human patients, and they are routinely used in cardiac surgery. This success has encouraged researchers to explore other organs for xenotransplantation.
- Other Organs: Organs like the liver, lungs, and kidneys from transgenic pigs are currently being tested in clinical research, with the goal of making these transplants a viable solution for patients suffering from organ failure.
- Risk Management
- Viral Transmission: One of the concerns associated with xenotransplantation is the risk of transmitting porcine viruses to human recipients. To mitigate this, advanced screening processes are employed to reduce the risk of viral transmission before the transplantation occurs. This pre-scanning ensures that the organs used are safe and free from infectious agents.
Food Sources
Transgenic animals have been explored as potential food sources with the goal of increasing efficiency and cost-effectiveness in agricultural production. By modifying the genetics of these animals, researchers aim to create livestock that grow larger, require less food, and are more sustainable. Below is a detailed explanation of transgenic animals as food sources, along with the associated challenges and current status.
- Enhanced Growth and Efficiency
- Objective: The primary aim of creating transgenic animals for food production is to develop livestock that can grow larger and faster without needing significant amounts of food. This would improve the overall efficiency of food production, reducing costs for farmers and increasing food supply, especially in regions facing food insecurity.
- Mechanism: Through genetic engineering, specific genes that control growth rate, metabolism, or nutrient absorption are modified or introduced into animals. These genetic alterations lead to improved feed conversion ratios, meaning the animals produce more meat or other food products while consuming less feed.
- Potential Benefits
- Increased Production: By producing animals that grow larger in a shorter period, the quantity of food derived from livestock can be significantly increased. This could help meet the rising global demand for animal-based food products, particularly in a world with a growing population.
- Cost-Effectiveness: With reduced feed requirements, the cost of raising transgenic livestock would decrease. Farmers could produce more meat, milk, or eggs with lower input costs, making animal products more affordable for consumers.
- Ethical and Safety Concerns
- Ethical Considerations: The creation of genetically modified animals for food has sparked ethical debates. Concerns include the welfare of the animals, potential environmental impacts, and the long-term effects of consuming genetically modified organisms (GMOs).
- Food Safety: Another critical issue is the safety of transgenic animals for human consumption. Although these animals are designed to be more efficient, rigorous testing is necessary to ensure that the modifications do not negatively affect the nutritional quality or introduce harmful substances into the food chain.
- Current Status
- Research Stage: As of now, transgenic animals designed for food production have not been commercially produced or marketed for human consumption. These animals remain in the research phase, where scientists are studying the potential benefits and addressing the ethical and safety concerns associated with genetically modified food sources.
- Regulatory Challenges: Before these animals can be used as commercial food sources, they must undergo extensive regulatory review to ensure they meet safety standards. Governments and international bodies continue to evaluate the implications of introducing transgenic livestock into the food market.
Transgenic Biological Models
Transgenic biological models play a crucial role in advancing our understanding of genetics and protein function. These models are genetically engineered animals that help researchers explore complex biological processes by manipulating specific genes. The modifications often involve overexpressing a gene or knocking it out, thereby revealing the role that the corresponding protein plays in the organism. Below is a detailed explanation of how transgenic biological models are used and their importance in scientific research.
- Purpose and Function
- Studying Protein Function: The primary purpose of transgenic biological models is to provide insight into the functions of specific proteins. This is achieved by altering the gene responsible for the production of that protein, either by increasing its expression (overexpression) or disabling it (knockout). These modifications allow researchers to observe the biological outcomes, thereby determining the role of the protein in various cellular and physiological processes.
- Genetic and Biological Studies: Transgenic models are essential tools in genetic research, where they help scientists investigate the effects of particular genes on development, behavior, disease progression, and more. By manipulating genetic pathways, researchers can simulate conditions or traits that may be difficult to study in humans directly.
- Techniques Used
- Overexpression: In this approach, the gene encoding the target protein is inserted or modified in such a way that the animal produces more of that protein than usual. This can reveal what happens when there is an excess of the protein, providing clues to its normal function and what might go wrong when its regulation is disrupted.
- Gene Knockout: A gene knockout involves disabling or “knocking out” a specific gene, rendering it non-functional. This method is used to study what happens when the organism cannot produce a certain protein, helping to identify its essential roles in biological systems.
- Examples of Transgenic Biological Models
- Andi: One of the early transgenic biological models, Andi the monkey, was genetically engineered to carry a jellyfish gene that produces a fluorescent protein. This helped researchers study gene expression and integration in primates.
- Smartmouse: This transgenic mouse model was designed to have enhanced memory and learning abilities by overexpressing genes involved in cognitive function. It has been used to study memory formation, learning processes, and related neurological conditions.
- Youth Mouse: Another example involves transgenic mice modified to delay aging processes by manipulating genes related to longevity and cellular repair mechanisms. These models are critical for studying the genetic basis of aging and potential therapies to mitigate age-related decline.
- Applications
- Understanding Diseases: Transgenic biological models are invaluable for studying the genetic basis of diseases. By replicating human disease conditions in animals through genetic manipulation, researchers can better understand how diseases develop, which in turn aids in identifying potential treatments.
- Drug Testing and Development: These models are also used to test the efficacy and safety of new drugs. Since they can be engineered to mimic human disease conditions, they provide a more accurate testing environment before clinical trials in humans.
Applications of Transgenic Animals
- Biomedical Research: Transgenic animals are used to study gene function, disease mechanisms, and drug development. For instance, mouse models can mimic human diseases to test new treatments.
- Pharmaceutical Production: Some transgenic animals produce proteins or antibodies that can be harvested for therapeutic use. For example, goats and cows have been engineered to produce proteins for drugs like antithrombin.
- Agriculture: Transgenic animals can be modified for traits like disease resistance, improved growth rates, or enhanced nutritional content. For instance, pigs have been genetically engineered to be more resistant to certain diseases.
- Tissue and Organ Transplantation: Researchers are exploring transgenic pigs as potential organ donors for humans, aiming to reduce the risk of rejection.
- Environmental Research: Transgenic animals can help in studying environmental effects on ecosystems or in bioremediation processes.
- Food Production: Genetic modifications can lead to improved traits in livestock, such as faster growth or higher quality meat and milk.
Advantages of Transgenic Animals
Transgenic animals, which are genetically modified to express specific traits, offer numerous advantages that can enhance various sectors, including agriculture, medicine, and research. Below are several key advantages of transgenic animals:
- Increased Growth Rate:
- Transgenic animals can be engineered to grow at an accelerated rate. This enhancement leads to faster production cycles, allowing for more efficient use of resources and potentially increasing profitability in livestock farming.
- Improved Disease Resistance:
- Genetic modifications can enhance the immune responses of animals, making them more resistant to diseases. This resistance reduces the need for antibiotics and vaccines, promoting healthier animal populations and lowering healthcare costs in animal husbandry.
- Enhanced Food Conversion Rates:
- Transgenic animals can be developed to improve food conversion efficiency, meaning they can convert feed into body mass more effectively. This trait results in lower feed costs and reduced environmental impact associated with feed production.
- Increased Muscle Mass:
- Certain genetic modifications can lead to an increase in muscle mass, particularly in livestock species. This advantage not only boosts meat production but also contributes to a more sustainable meat supply, meeting consumer demand efficiently.
- Improved Nutritional Quality:
- Transgenic animals can be designed to enhance the nutritional content of their products. For instance, modifications may increase the levels of essential fatty acids or vitamins in meat or milk, thus contributing to better human nutrition.
- Enhanced Wool Quality:
- In species such as sheep, transgenic approaches can lead to improved wool characteristics, such as fiber strength and quality. This enhancement can benefit the textile industry by providing higher-quality raw materials.
Disadvantages of Transgenic Animals
Below are key disadvantages associated with transgenic animals:
- Multifunctionality of Inserted Genes:
- When a gene is inserted into a transgenic animal, it may not only affect the desired trait but could also influence multiple biological functions. This unpredictability can lead to unintended consequences that complicate the understanding of the animal’s physiology.
- Breathing Problems:
- Certain genetic modifications may lead to respiratory issues in transgenic animals. These complications can arise from alterations in genetic pathways essential for normal respiratory function, resulting in reduced quality of life or increased veterinary care.
- Risk of Mutagenesis:
- The insertion of foreign genes can sometimes trigger mutagenesis, where additional mutations occur within the genome. Such mutations can affect the functionality of the animal’s genes, potentially leading to health issues or abnormal development.
- Low Survival Rates:
- Transgenic animals often experience lower survival rates compared to their non-modified counterparts. This reduction can stem from various factors, including developmental complications or genetic instability, which may hinder the animal’s overall viability.
- Ethical and Welfare Concerns:
- The process of creating transgenic animals raises ethical questions regarding animal welfare. Concerns about the well-being of genetically modified animals and the implications of such modifications on their natural behaviors and health must be addressed.
- https://krishi.icar.gov.in/jspui/bitstream/123456789/24651/1/Transgenic%20Animals%20and%20its%20application.pdf
- https://gyansanchay.csjmu.ac.in/wp-content/uploads/2021/12/UNIT-1-TRANSGENIC-ANIMALS-1.pdf
- https://epgp.inflibnet.ac.in/epgpdata/uploads/epgp_content/S000035ZO/P001806/M027427/ET/1519105740M16TransgenicanimalsMethodsofProductionQuad1.pdf
- https://ijcrt.org/papers/IJCRT2203348.pdf
- https://www.davuniversity.org/images/files/study-material/transgenic%20animal.pdf
- https://www.ijcmas.com/7-10-2018/Sheikh%20Firdous%20Ahmad,%20et%20al.pdf
- https://www.geeksforgeeks.org/transgenic-animals-biotechnology-and-its-application/