What is Transfer-messenger RNA (tmRNA)?
- Transfer-messenger RNA (tmRNA), also known as 10Sa or SsrA RNA, is exceptional among bifunctional RNAs in that it possesses both tRNA and mRNA characteristics.
- tmRNA employs these two activities to liberate translationally stalled ribosomes and to target nascent polypeptides for destruction. This coordinated reaction, known as trans-translation, helps to bacterial translational quality control and gene expression regulation.
- Mutants lacking tmRNA activity generally exhibit severe abnormalities, including problems in viability, pathogenicity, and environmental stress responses.
- tmRNA ends fold into a structure similar to tRNA (Ala).
- In place of the anticodon loop, however, tmRNA contains numerous pseudoknots and a specific open reading frame.
- This peculiar shape enables tmRNA to bind with certain ribosomes during the trans-translation reaction.
- During trans-translation, tmRNA serves as both a tRNA and a message, inducing the ribosome to attach a peptide tag to the C terminus of the nascent polypeptide. This process degrades the precursor polypeptide and releases the ribosome.
- Although the physiological function of trans-translation is still being studied, tmRNA has been identified in every bacterial genome sequence and is one of the most abundant RNAs in the cell, indicating that it confers a significant evolutionary advantage in all environments that support bacterial life.
History
- After a mixed “10S” electrophoretic fraction of Escherichia coli RNA was separated into tmRNA and the similarly-sized RNase P RNA, tmRNA was initially termed 10Sa RNA (10Sb).
- The presence of pseudouridine in the 10S RNA mixture suggested that tmRNA shares modified nucleotides with tRNA.
- Sequencing ssrA from Mycobacterium tuberculosis revealed the similarity between the 3′ end of tmRNA and the T stem-loop of tRNA for the first time.
- The subsequent comparison of sequences revealed the whole tRNA-like domain (TLD) formed by the 5′ and 3′ ends of tmRNA, including the acceptor stem with features similar to those in alanine tRNA that facilitate its aminoacylation by alanine-tRNA ligase.
- It also highlighted distinctions between tRNA and tmRNA: the anticodon arm is absent from tmRNA, and the D arm region consists of a baseless loop.
Properties of tmRNA
- tmRNA’s 5′ and 3′ ends fold into a structure containing an acceptor stem and a TΨC arm.
- The acceptor stem has a G:U wobble base pair, which permits it to be identified and charged with alanine by alanyl-tRNA synthetase.
- The tRNA-like domain is bound by two proteins that are needed for trans-translation activity: SmpB, a specialised component of the trans-translation system, and EF-Tu, which is essential for ribosome-ribosome interactions.
- Depending on the species, the tag reading frame encodes a short peptide and is housed within an extensive (>250 nt) sequence containing 2-4 pseudoknots.
- Despite the fact that this region is translated during trans-translation, it lacks a conventional start codon and there is no evidence that ribosomes can launch translation on the tag reading frame.
- It is therefore not a genuine open reading frame. The length of annotated tag reading frames ranges from 8 to 35 codons and ends with a stop codon.
- However, the tag reading frame can be truncated to a single codon or lengthened to code for entire proteins without interfering with trans-translation activity. Uncertain is the function of pseudoknots in this location.
- If any of the pseudoknots on the tmRNA of E. coli are substituted, the tmRNA is still functional, hence none of these elements are needed for activity. However, the evolutionary conservation of pseudoknots, specifically PK1, shows that they have a function in living organisms.
tmRNA Structure
tmRNA contain the following domains;
tRNA-Mimic Domain
- The tRNA-like structure (TLS) of tmRNA is retained in all known eubacterial genomes, often forming a two-component complex.
- The primary transcript of tmRNA is processed by tRNA maturation enzymes, such as RNase P, and the 5′ and 3′ ends of tmRNA fold into a structure consisting of an acceptor stem and a TC arm.
- A G:U wobble base pair in the acceptor stem permits tmRNA to be identified and charged with alanine by alanyl-tRNAsynthetase.
- Additionally, the tRNA-mimic domain contains binding sites for protein components such as alanyl-tRNA synthetase, SmpB, and EF-Tu.
Pseudoknots
- tmRNA is significantly larger than regular tRNA and lacks the anticodon stem-loop structure.
- Rather, they are composed of multiple pseudoknots (2 to 4, depending on the species), and the mRNA-like domain is typically located between pseudoknots 1 and 2. The function of pseudoknots in tmRNA is still contested.
- The fact that all tmRNAs contain pseudoknots suggests that they play a crucial function in the tmRNA’s biological activity.
- Mutations in pseudoknot 1, whose structure is known, can render tmRNA useless, whereas mutations in other pseudoknots can drastically reduce tmRNA activity.
- In contrast, E. coli tmRNA variants devoid of pseudoknots 1, 2, 3, and/or 4 exhibit in vitro transpeptidation activity.
- In addition, recent research has demonstrated that substituting pseudoknots with basic hairpin structures enhances the biological activity of tmRNA.
- Results indicate that replacing single-stranded RNA with a pseudoknot or disturbing its structure is substantially more dangerous than replacing it with a folded element.
- In actuality, the structure of the pseudoknots is significantly more important than their sequence.
- Pseudoknots reportedly assist tmRNA folding, which is essential for tmRNA stability, the binding of tmRNA-associated protein factors, and the maintenance of the proper translation geometry.
mRNA-Mimic Domain
- The mRNA-like domain comprises a short open reading frame (ORF) that encodes a hydrophobic signal peptide (referred to as “tag”).
- The tag is added to the nascent polypeptide when the ribosome changes from the original mRNA reading frame to the tmRNA reading frame.
- Different bacterial species encode peptides ranging in length from 8 to 35 residues. Even though the tag reading frame concludes with a stop codon, it lacks a translation initiation sequence and a typical start codon.
- There is a list of over 600 tag sequences on the tmRNA website http://www.indiana.edu/tmrna/ at now.
- A comparison of 610 peptide tags from various bacteria has revealed an evident bias in the amino acid content of the ORFs: Ala and Asp are the most common amino acids in ORFs, while Trp and Cys are uncommon.
- Because Trp and Cys are vulnerable to oxidation, this amino acid composition may indicate that tmRNA can function normally even under extreme conditions such as oxidation.
- In addition, His and Met, two rare residues in the ssrA tag, are susceptible to oxidation and other changes.
- However, certain codons are uncommon in the tmRNA structure, such as frame-shifting susceptible codons and sense codons vulnerable to misreading as nonsense codons.
- The 3 codons of the ORF region of the tmRNA comprise a stem-loop structure. Although mutations that impair the stem-loop structure do not prevent ribosome rescue or tagging, new research reveal that degradation of rescued mRNAs mediated by tmRNA requires on the 3 bases of ORF.
- When the ribosome encounters a stop codon, translation is terminated; the polypeptide is then released and destroyed by proteases that recognise the C-terminal signal peptide.
- Recent reports indicate that the 3′ end of ORF is needed for the degradation of nonstop mRNAs. This area also facilitates recycling of ribosomal subunits for reuse in protein synthesis.
Protein Partners
- tmRNA, like many other RNAs, requires protein cofactors for its action.
- Numerous factors, such as EF-Tu, SmpB, ribosomal protein S1, and alanyl-tRNA synthetase, have been discovered as tmRNA-binding proteins with a direct and specialised role in tmRNA.
- For example, the synthetase-catalyzed charging step is functionally important since uncharged tmRNAs are inactive.
- In vitro tagging mediated by tmRNA requires alanyl-tRNA synthetase or precharged alanyl-tRNA.
- Despite major differences in size and structure, tRNAAla and tmRNA are both charged by the same alanyl- tRNA synthetase because their acceptor stems include simple determinants.
- tmRNA can be charged by alanyl- tRNA synthetase alone, although aminoacylation is enhanced in the presence of SmpB.
- SmpB, a tiny protein that specifically and tightly attaches to tmRNA, is a crucial molecule for the structure, stability, and activity of tmRNA.
- tmRNA binding to ribosomes is one of the most essential roles of SmpB in this regard.
- Despite the fact that many SmpB molecules can attach to a single tmRNA molecule, there is a high-affinity region in the tRNA-mimic domain that appears to be crucial for interaction.
- The C-terminal tail of SmpB is likely important for tmRNA activity after ribosome binding but prior to the completion of transpeptidation, according to structural analyses.
- EF- Tu (a translation elongation factor) binds to tmRNA and promotes a fruitful interaction with the ribosome.
- EF-Tu transports Ala-tmRNA to the ribosome, similar to aminoacyl-tRNA in translation, such that the aa-tRNA•EF-Tu•GTP complex can completely connect to the A-site of the ribosome.
- When GTP.EF-Tu binds to an amino-acylated tRNA (aa-tRNA), the ester bond between a charged amino acid and the tRNA is decreased.
- Cognate codon-anticodon pairing induces GTP hydrolysis, causing conformational changes, EF-Tu•GDP dissociation, and migration of the acceptor end of tRNA into the ribosome’s peptidyl-transfer centre.
- Although tmRNA-mediated addition of alanine to a stalled peptide can occur slowly in the absence of EF-Tu in vitro, EF-Tu is absolutely required for normal tmRNA-mediated tagging in vivo.
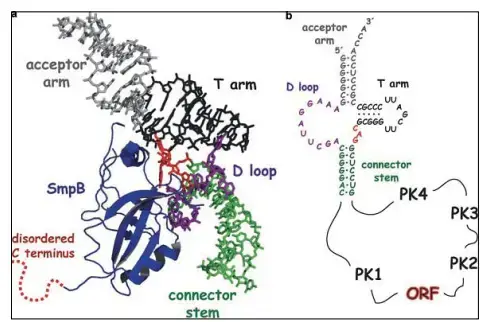
tmRNA Function ― Trans-Translation
- The tRNA-like and mRNA-like functions of tmRNA are used in a single, concerted reaction, as opposed to other bifunctional RNAs which can use their different roles independently.
- Transfer-messenger RNA (tmRNA) is a crucial component of the quality control mechanism of eubacteria. This system monitors protein synthesis and reactivates ribosomes halted by defective mRNA.
- Normal translation in bacteria ceases when the ribosome encounters one of three stop codons on the mRNA template.
- The nascent peptide chain is then released, and ribosome subunits are separated.
- The ribosome, however, pauses at the 3′ end of uninterrupted mRNA when the template mRNA lacks a stop codon.
- Each bacterial generation often has a high frequency of translational stalling. At this rate, if there were no methods to eliminate translational blocks, the whole pool of ribosomes in a cell would be eliminated in less than one generation.
- This mechanism is called “trans-translation” because two RNAs operating in trans create the tagged protein.
- Only in the instance of trans-translation is a protein generated from two different RNA signals. Clearly, the tmRNA pathway does not interact with ribosomes during normal translation; rather, a stopped ribosome at the 3′-end of an uninterrupted mRNA triggers trans-translation.
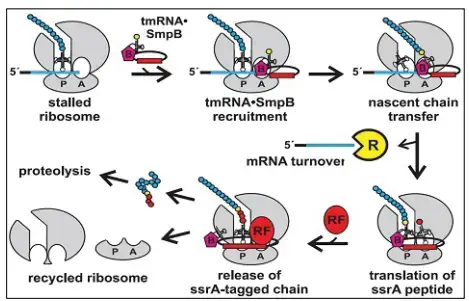
Trans-translation of tmRNA activity Steps
- ribosome arrest is caused by nonstop mRNA translation.
- The complex tmRNA•SmpB attaches to the A site of stalled ribosomes and accepts the nascent chain.
- The non-stop mRNA is then released and destroyed preferentially by RNase R, allowing translation to restart using the open reading frame found in tmRNA.
- After the production of the ssrA peptide, translation is terminated by release factors (RF), and the ribosome is recycled into large and small subunits.
- A number of proteases break down the ssrA-tagged chain.
Regulation of tmRNA activity
- tmRNA is abundant in developing cells, but stress factors such as heat shock, cold shock, and biofilm development increase its production.
- C. crescentus exhibits more extensive regulation of tmRNA, as the abundances of tmRNA and SmpB vary as a function of the cell cycle.
- tmRNA and SmpB levels rise during the late G1 phase and are rapidly depleted once DNA replication begins.
- tmRNA is stable in G1-phase cells, but is destroyed by the exonuclease RNase R in early S phase.
- The structure of tmRNA may boost C. crescentus’ capacity to regulate tmRNA stability. The gene encoding tmRNA in C. crescentus is circularly permuted, resulting in tmRNA with two RNA chains.
- RNase R identifies the non-tRNA-like 3′ end of tmRNA; therefore, the two-part structure of tmRNA is essential for its controlled breakdown.
- In addition to -proteobacteria, at least two more bacterial lineages possess circularly permuted tmRNA. C. crescentus’ tmRNA and SmpB are also regulated by subcellular localization.
- The results of fluorescence in situ hybridization and immunofluorescence indicate that tmRNA and SmpB are helical within the cell.
- RNase R is localised in a non-overlapping manner, indicating that localization may be utilised in part to restrict RNase R’s access to tmRNA and avoid its improper destruction.
- Localization’s function in trans-translation is yet unclear.
- Despite the conservation of tmRNA in bacteria and the requirement for trans-translation in numerous bacterial systems, no bifunctional RNA identical to tmRNA has been identified in the nuclear genome of a eukaryote.
- Due to the above-described mRNA proofreading capabilities, the necessity for translation quality control may be minimal in eukaryotes.
- In yeast, however, a protein-based method comparable to trans-translation has been established.
- In Saccharomyces cerevisiae, proteins translated from aberrant mRNA are ubiquitinated by a specific E3 ligase to stimulate proteasomal destruction.
- Therefore, it is possible that eukaryotes have evolved proteins to replace bifunctional tmRNA.
Facts
- Transfer-messenger RNA (tmRNA) possesses characteristics of both tRNA and mRNA.
- In trans-translation, these functions are used to release stalled ribosomes and direct nascent polypeptides toward destruction.
- trans-translation aids in translational quality control and gene expression regulation.
- tmRNA is widespread in bacteria and one of the most abundant RNAs in the cell.
- The phenotypes of mutations lacking tmRNA activity are severe.
References
- Kazemi Nezhad, Seyed Reza & Montazeri, F. & Hoseini, Seyed Mehdi. (2015). Role of the transfer-messenger RNA pathway _ trans-translation _ in bacterial physiology. Genetics in the Third Millennium. 13. 4014-4021.
- Keiler KC, Ramadoss NS. Bifunctional transfer-messenger RNA. Biochimie. 2011 Nov;93(11):1993-7. doi: 10.1016/j.biochi.2011.05.029. Epub 2011 Jun 1. PMID: 21664408; PMCID: PMC3175250.
- Doherty, J., & Guo, M. (2016). Transfer RNA. Encyclopedia of Cell Biology, 309–340. doi:10.1016/b978-0-12-394447-4.10039-2
- https://mcmanuslab.ucsf.edu/node/272
- https://www.frontiersin.org/articles/10.3389/fgene.2014.00066/full
- https://rnajournal.cshlp.org/content/11/5/668.full
- https://www.pnas.org/doi/10.1073/pnas.0607438103