What is DNA Transcription (RNA Synthesis)?
- DNA transcription is a crucial biological process that involves the synthesis of RNA molecules from a DNA template. It plays a fundamental role in gene expression and protein synthesis within cells. The main focus of DNA transcription is the production of messenger RNA (mRNA), which carries the genetic information from DNA to the cellular machinery responsible for protein synthesis.
- During transcription, an enzyme called RNA polymerase binds to a specific region on the DNA molecule called the promoter. The promoter acts as a signaling sequence that tells the RNA polymerase where to start transcribing. The RNA polymerase then unwinds a segment of the DNA double helix, creating a transcription bubble. This separates the two DNA strands, exposing the template strand.
- Using the template strand as a guide, the RNA polymerase adds complementary RNA nucleotides to synthesize an RNA molecule. The nucleotides in RNA are similar to those in DNA, except that RNA contains uracil (U) instead of thymine (T). The RNA polymerase matches the RNA nucleotides to the corresponding DNA nucleotides on the template strand, following the base pairing rules (adenine with uracil, cytosine with guanine, and vice versa).
- As the RNA polymerase adds RNA nucleotides, a sugar-phosphate backbone forms, creating a single-stranded RNA molecule. This process continues until the RNA polymerase reaches a termination signal on the DNA template, indicating the end of transcription.
- After transcription, the RNA molecule undergoes additional processing steps, especially in eukaryotic cells. These steps include polyadenylation, where a string of adenine nucleotides, called a poly(A) tail, is added to the end of the RNA molecule. The RNA molecule may also undergo capping, where a modified nucleotide is added to the beginning of the molecule. Additionally, in eukaryotes, the RNA molecule may undergo splicing, where non-coding regions, called introns, are removed, and the remaining coding regions, called exons, are joined together.
- Once the RNA molecule is fully processed, it can perform its various functions depending on its type. If it is an mRNA molecule, it carries the genetic information to the ribosomes in the cytoplasm, where it serves as a template for protein synthesis through a process called translation. Other types of RNA molecules, such as microRNAs, transfer RNAs, and ribosomal RNAs, have distinct roles in regulating gene expression, protein synthesis, and cellular processes.
- In summary, DNA transcription is a complex process that involves the synthesis of RNA molecules from a DNA template. It plays a crucial role in gene expression and protein synthesis by generating the necessary instructions for cellular functions. Understanding DNA transcription is essential for unraveling the mechanisms of genetic regulation and molecular biology.
Definition of DNA Transcription
DNA transcription is the process of copying genetic information from DNA to RNA. It involves the synthesis of RNA molecules using a DNA template and is an essential step in gene expression and protein synthesis.
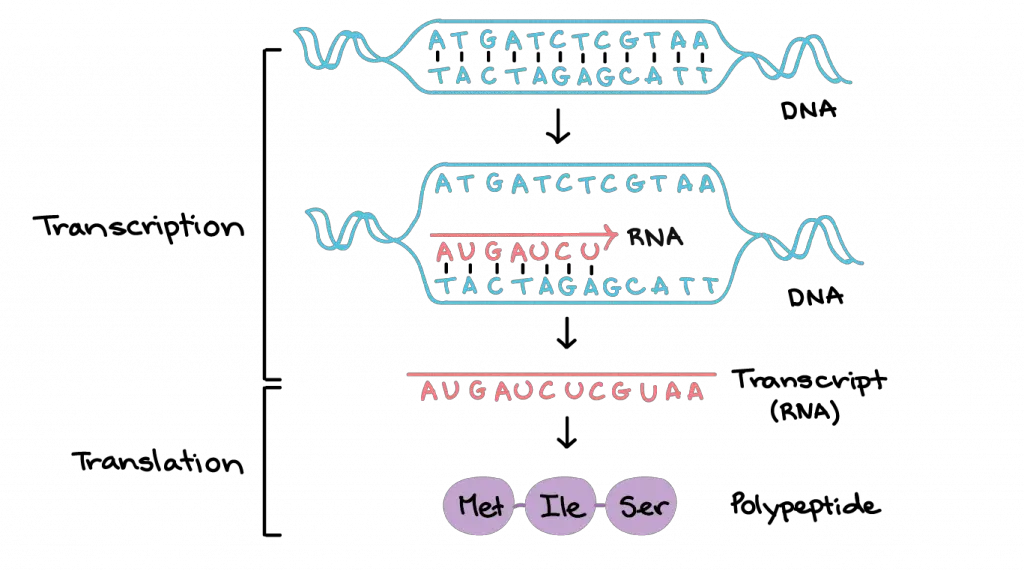
Enzyme involve in DNA Transcription and Their Function
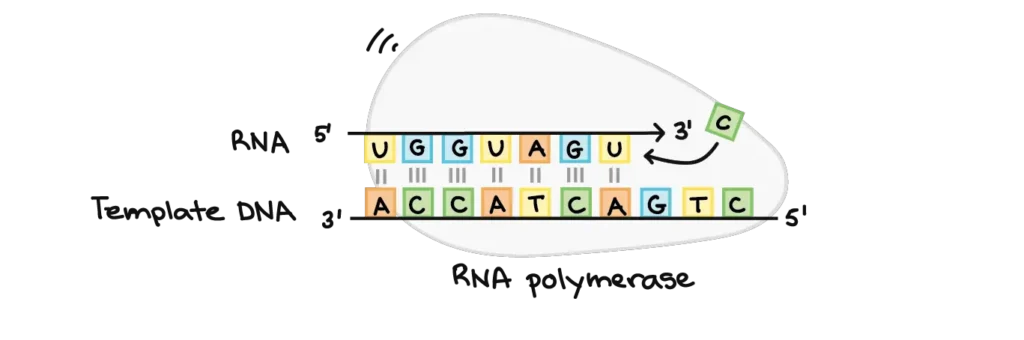
During DNA transcription, several enzymes are involved in the process, with the primary enzyme being RNA polymerase. The specific types of RNA polymerase used depend on whether the transcription occurs in prokaryotes or eukaryotes. Prokaryotes utilize a single type of RNA polymerase, while eukaryotes employ three distinct types: RNA polymerase I, II, and III.
RNA polymerase carries out various functions throughout DNA transcription, including:
- Formation of the initiator complex: RNA polymerase, along with other transcription factors, binds to the promoter region of the DNA molecule. This complex aids in unwinding the double helix structure of DNA, preparing it for transcription.
- Synthesis and elongation of the RNA transcript: RNA polymerase adds complementary RNA nucleotides to the growing RNA chain. These nucleotides include Adenine (A), Cytosine (C), Guanine (G), and Uracil (U) instead of Thymine (T). It follows the base pairing rules by matching RNA nucleotides with the complementary DNA nucleotides on the template strand, thereby synthesizing an RNA transcript.
- Termination of transcription: RNA polymerase also plays a role in the termination of transcription. It recognizes specific termination sequences on the DNA template, signaling the completion of transcription and causing the detachment of RNA polymerase from the DNA molecule.
Additionally, other enzymes and proteins are involved in DNA transcription, such as transcription factors that help RNA polymerase bind to the promoter region and unwind the DNA. Some enzymes also aid in the processing of the transcribed RNA molecule, including the addition of a poly(A) tail, capping, and splicing of introns in eukaryotes.
Overall, RNA polymerase is the central enzyme responsible for DNA transcription, working in conjunction with other enzymes and factors to initiate, elongate, and terminate the synthesis of RNA molecules from DNA templates.
Factors that regulate transcription
Transcription, the process of synthesizing RNA from a DNA template, is regulated by a complex interplay of factors. These factors can enhance or inhibit transcription and determine when and to what extent a gene is expressed. Here are some key factors that regulate transcription:
- Transcription Factors: Transcription factors are proteins that bind to specific DNA sequences called enhancers or promoters. They can activate or repress transcription by interacting with RNA polymerase and other regulatory proteins. Transcription factors can be general or specific to certain genes or cell types.
- Promoters and Enhancers: Promoters are DNA sequences located near the transcription start site that provide a binding site for RNA polymerase and transcription factors. Enhancers are regulatory DNA sequences that can be located far from the promoter and can modulate gene expression by interacting with transcription factors and the promoter region.
- Chromatin Structure: DNA in the nucleus is packaged into a complex structure called chromatin. The accessibility of DNA for transcription is influenced by the degree of chromatin condensation. Chromatin remodeling complexes and histone modifications, such as acetylation and methylation, can alter chromatin structure and regulate transcriptional activity.
- DNA Methylation: DNA methylation is a chemical modification where a methyl group is added to DNA. Methylation of certain regions, such as CpG islands near promoters, can inhibit transcription by blocking the binding of transcription factors or recruiting proteins that repress gene expression.
- Non-Coding RNAs: Non-coding RNAs, such as microRNAs (miRNAs) and long non-coding RNAs (lncRNAs), can regulate transcription by binding to mRNA molecules and either promoting their degradation or inhibiting their translation into proteins. They can also interact with transcription factors and chromatin modifiers to influence gene expression.
- Signal Transduction Pathways: Various extracellular signals, such as growth factors or hormones, can activate intracellular signaling pathways that ultimately regulate gene transcription. These pathways often involve the activation or repression of transcription factors through phosphorylation or other post-translational modifications.
- Epigenetic Modifications: Epigenetic modifications, including DNA methylation, histone modifications, and non-coding RNAs, can be heritable and can influence gene expression patterns over generations. They provide an additional layer of regulation on top of the DNA sequence itself.
- Cellular Environment and Developmental Stage: Transcriptional regulation can vary depending on the cell type, tissue, or developmental stage. Different combinations of transcription factors, chromatin modifiers, and signaling pathways are active in different contexts, allowing for cell-specific gene expression patterns.
Summarized Steps of DNA Transcription – Steps of transcription
The process of DNA transcription can be summarized as follows:
- Transcription factor binding: Around 50 different protein transcription factors bind to specific promoter sites on the 5′ side of the gene to be transcribed. These factors play a role in regulating gene expression.
- RNA polymerase binding: The RNA polymerase enzyme binds to the transcription factor complex, facilitating the opening up of the DNA double helix.
- Direction of transcription: The RNA polymerase reads one strand of the DNA molecule in the 3′ to 5′ direction, known as the template strand.
- Nucleosome displacement (in eukaryotes): In eukaryotic cells, for protein-coding genes, the nucleosome structure is temporarily displaced as RNA polymerase (Pol II) advances along the DNA strand.
- Ribonucleotide assembly: As the RNA polymerase moves along the DNA strand, it assembles ribonucleotides into a growing RNA strand. This process utilizes triphosphates (ATP).
- Base-pairing: Each ribonucleotide is inserted into the growing RNA strand through base-pairing. Cytosine (C) is linked to guanine (G), while uracil (U) is linked to adenine (A). This process ensures the complementary nature of the RNA sequence to the DNA template.
- Direction of synthesis: RNA synthesis occurs in the 5′-3′ direction, with new ribonucleotides being added to the 3′ end of the growing RNA strand.
- Removal of terminal phosphates: As each nucleoside triphosphate is added to the growing RNA strand, the two terminal phosphates are removed, resulting in a single phosphate linkage.
- Termination and release: When transcription reaches the end of the gene, the transcript is released from the RNA polymerase. At this point, the polymerase enzyme also detaches from the DNA.
These steps summarize the main stages of DNA transcription, where the DNA template is read by RNA polymerase, leading to the synthesis of an RNA molecule that carries the genetic information encoded in the gene.
DNA Transcription Process
In prokaryotic cells the whole process of transcription is described in three phases: Initiation Elongation, and Ending. When the process comes to closing process in prokaryotes the mRNA that is formed is now in a position to be translated. As opposed to eukaryotes termination, an immature version of mRNA is created, and as such it is necessary to go through additional processes to make an mRNA that can then be transformed into proteins.
In general, the process of transcription converts DNA into mRNA, the type of RNA which contains the information required for the production of proteins. In eukaryotes there are two main stages that happen during transcription:
- Pre-messenger RNA formation using an RNA polymerase enzyme.
- Editing of pre-messenger RNA by splicing
Pre-messengerger RNA creation involves beginning, elongation and terminating phases, which are completed with the formation of an mRNA. The mRNA goes through various phases of splicing before forming mature mRNA.
Formation of pre-messenger RNA
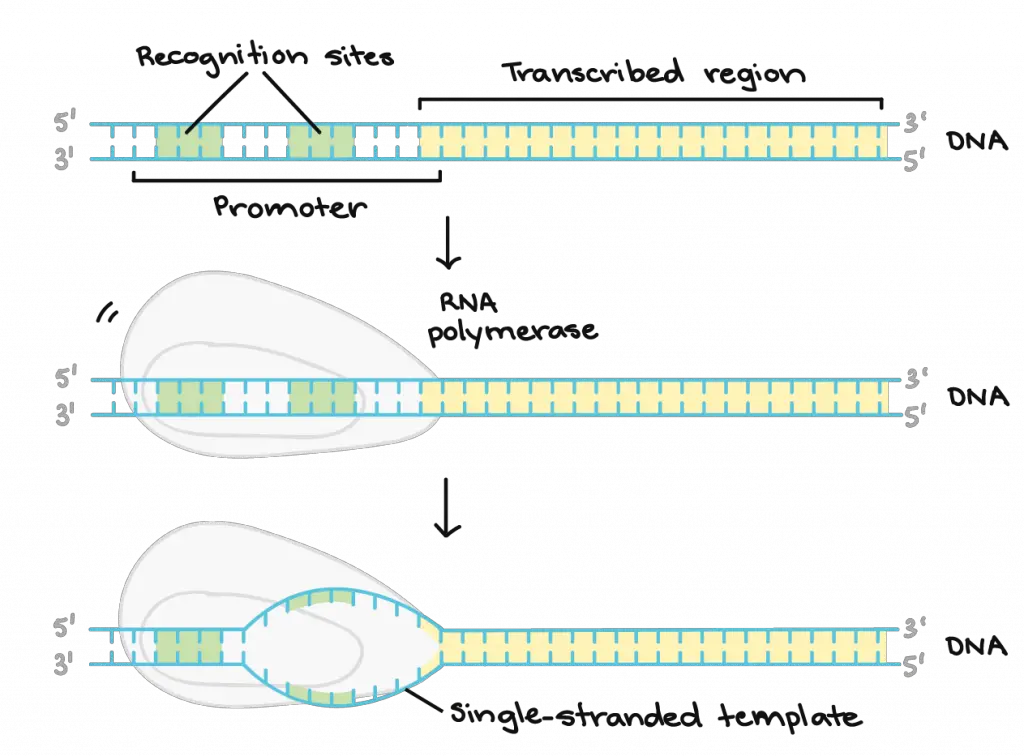
The formation of pre-messenger RNA (pre-mRNA) occurs during the process of transcription, which is catalyzed by RNA polymerase. Here are the key steps involved:
- Unwinding of DNA: At the beginning of transcription, the DNA molecule must unwind to expose the template strand. RNA polymerase assists in this process by breaking the hydrogen bonds between the DNA strands.
- Sense and antisense strands: In transcription, only one DNA strand is transcribed, known as the antisense strand. The complementary DNA strand is called the sense strand. Although the mRNA is a copy of the sense strand, it is the antisense strand that is transcribed.
- Base pairing and RNA synthesis: Ribonucleoside triphosphates (NTPs) align along the antisense DNA strand through base pairing. RNA polymerase joins these ribonucleotides together to form a pre-mRNA molecule. The pre-mRNA is complementary to a specific region on the antisense strand of the DNA.
- Termination: Transcription continues until the RNA polymerase reaches a triplet of bases known as a stop signal. At this point, the RNA polymerase enzyme dissociates from the DNA template, and the pre-mRNA molecule is complete. The DNA molecule then rewinds to reform the double helix structure.
Formation of the pre-mRNA is an essential step in gene expression. It represents the initial transcription of the genetic information encoded in the DNA into an RNA molecule. This pre-mRNA will undergo further processing, including the removal of introns and addition of a poly(A) tail, before it becomes mature messenger RNA (mRNA). The mRNA molecule will then proceed to translation, where it serves as a template for protein synthesis. The stages of pre-mRNA formation include initiation, elongation, and termination, which collectively contribute to the accurate transcription of the DNA sequence.
Initiation
Promoter and initiation Mechanism in prokaryotes
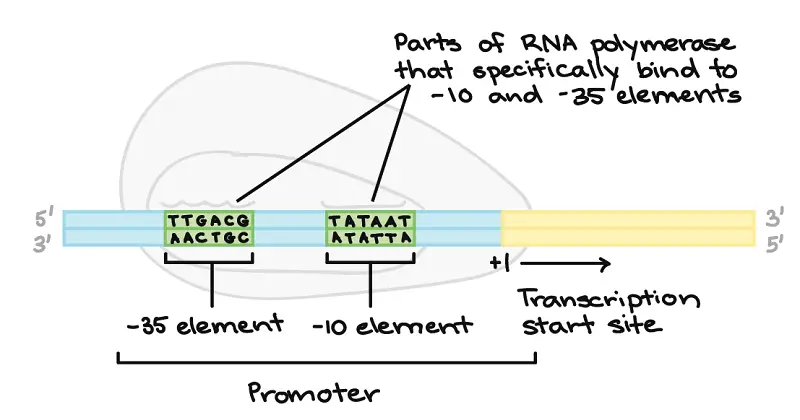
In prokaryotes, the promoter region plays a crucial role in the initiation of transcription. Here’s an explanation of the promoter and initiation process in prokaryotic DNA transcription:
- Promoter recognition: The initiation of transcription is guided by a specific DNA region called the promoter. The promoter serves as a binding site for RNA polymerase, which is the enzyme responsible for catalyzing transcription.
- Sigma factor and initiation complex: RNA polymerase in prokaryotes consists of several subunits, including a dissociative unit called the sigma (σ) factor. The sigma factor allows the RNA polymerase to recognize and bind to the promoter sequence. The promoter sequence is typically located between -35 and -10 regions relative to the transcription start site.
- Closed promoter complex: When the sigma factor of RNA polymerase recognizes the promoter sequence, it binds to the DNA template molecule. This binding forms a closed promoter complex, where the DNA duplex remains intact but is locally denatured in the region of the promoter.
- Transcription factors: Transcription factors are regulatory proteins that can bind to the promoter region along with the RNA polymerase. These factors control the rate of transcription by influencing the binding of RNA polymerase to the promoter.
- Open promoter complex: Once the closed promoter complex is formed, RNA polymerase proceeds to denature the DNA duplex locally, resulting in the formation of an open promoter complex. In this complex, the DNA strands separate, exposing the bases on each of the two DNA strands.
The formation of the open promoter complex allows RNA polymerase to initiate transcription by synthesizing an RNA molecule using the DNA template strand. The transcription process continues with elongation and termination, resulting in the production of an RNA transcript that corresponds to the DNA sequence being transcribed.
Promoters in prokaryotes can vary in sequence and strength, influencing the efficiency and regulation of transcription. The specific interactions between the RNA polymerase, sigma factor, transcription factors, and the promoter sequence determine the initiation of transcription and subsequent gene expression in prokaryotic organisms.
Promoter and initiation Mechanism in Eukaryotes
In eukaryotes, the process of promoter recognition and initiation of transcription differs from that of prokaryotes. Here’s an explanation of the promoter and initiation process in eukaryotic DNA transcription:
- Basal transcription factors: Unlike prokaryotes, eukaryotic RNA polymerase does not directly attach to the promoter sequence. Instead, a group of proteins called basal transcription factors bind to the promoter first. These factors are responsible for recruiting and positioning the RNA polymerase at the transcription start site.
- Promoter sequence elements: Eukaryotic promoters contain various sequence elements that are recognized by transcription factors. One of the most common promoter elements in eukaryotes is the TATA box. The TATA box is a short DNA sequence rich in adenine (A) and thymine (T) bases. It is recognized by specific transcription factors, including the TATA-binding protein (TBP).
- Transcription factor binding: Transcription factors, including TBP, recognize and bind to specific sequence elements within the promoter region. These factors help initiate the assembly of the transcription pre-initiation complex.
- Assembly of the pre-initiation complex: The binding of transcription factors at the promoter recruits additional proteins, forming the pre-initiation complex. This complex includes RNA polymerase and other factors necessary for transcription initiation.
- DNA unwinding: The TATA box, with its high A and T content, facilitates the unwinding of the DNA double helix in the vicinity of the promoter. This unwinding exposes the template strand, allowing RNA polymerase to access it for transcription.
Once the pre-initiation complex is assembled and the DNA is unwound, the RNA polymerase proceeds with the initiation of transcription. It synthesizes an RNA molecule complementary to the DNA template strand, starting from the transcription start site.
Eukaryotic promoters can contain additional regulatory elements and have more complex structures compared to prokaryotic promoters. The precise interactions between transcription factors, basal transcription factors, and the promoter sequence govern the initiation of transcription and the regulation of gene expression in eukaryotes.
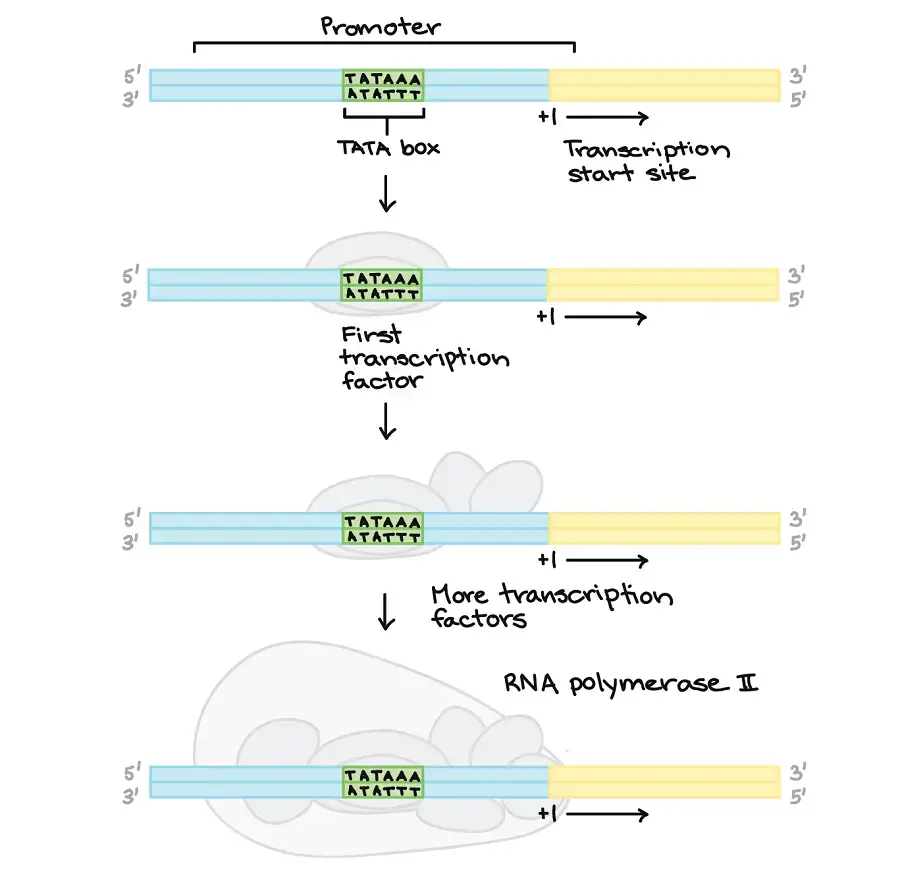
Elongation
Elongation is the phase of DNA transcription where RNA synthesis occurs, resulting in the elongation of the RNA molecule. Here are the key points related to elongation:
- Dissociation of the sigma factor: After initiation, the sigma (σ) factor, which helps RNA polymerase recognize the promoter sequence, dissociates from the RNA polymerase complex.
- Template strand and coding strand: The template strand of DNA, also known as the noncoding strand, is used as a template for RNA synthesis. The RNA polymerase reads the template strand in the 3′ to 5′ direction. The other strand of DNA, known as the coding strand, has the same base sequence as the newly formed mRNA, except that thymine (T) is replaced by uracil (U).
- Phosphodiester bond formation: The RNA polymerase catalyzes the formation of phosphodiester bonds between adjacent ribonucleotides. As the enzyme moves along the DNA template, it adds ribonucleotides to the growing RNA strand.
- Energy utilization: The energy required for RNA polymerization comes from the hydrolysis of high-energy triphosphate molecules (NTPs) to monophosphate, releasing inorganic diphosphates (PPi).
- Transcription bubble: A transcription bubble is formed and maintained during elongation. This bubble represents the region where the DNA strands are separated to allow access for RNA synthesis. The bubble moves along the DNA duplex as elongation progresses.
- Pausing and stalling: Pausing and stalling can occur during elongation, which can be important for transcription termination and regulation. These pauses allow for proper RNA editing and processing factors to bind, ensuring accurate RNA production.
- Proofreading mechanism: Elongation involves a proofreading mechanism that can correct any incorrectly incorporated bases. In eukaryotes, pauses during transcription elongation provide opportunities for appropriate RNA editing factors to bind and replace incorrect bases.
During elongation, multiple rounds of transcription can occur on a single DNA template, leading to the rapid production of multiple mRNA molecules from a single gene. The rate of elongation varies between prokaryotes and eukaryotes, with factors like nucleosomes in eukaryotes posing barriers to transcription elongation. The speed of elongation in both prokaryotes and eukaryotes is approximately 10-100 nucleotides per second.
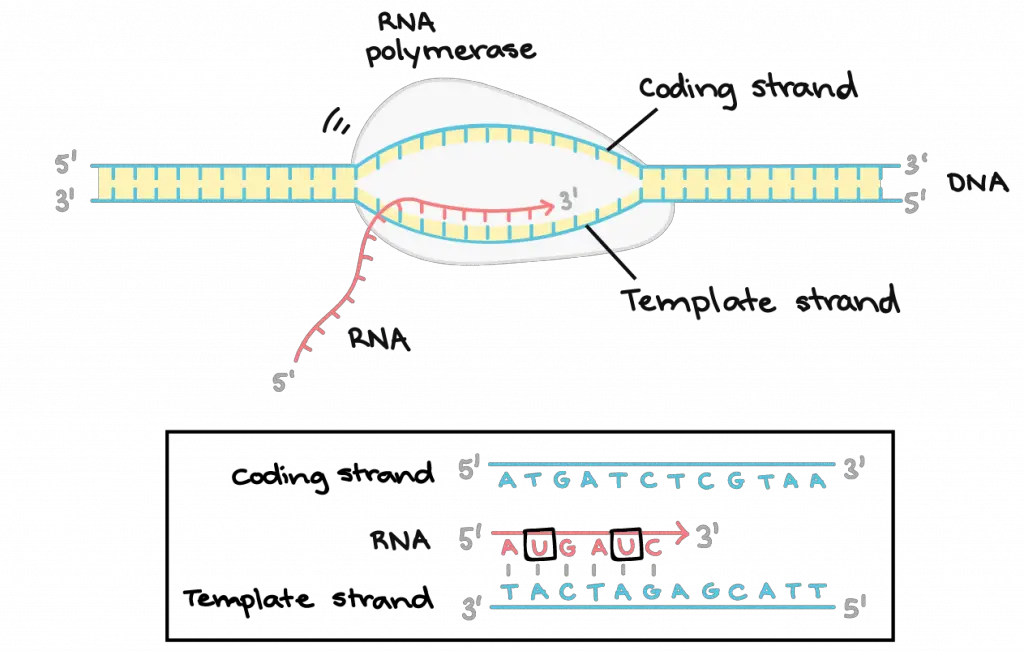
Termination
Termination is the final phase of DNA transcription, where the process of synthesizing RNA comes to an end. Here are the main points related to termination:
- Terminator sequence: Termination is signaled by a specific DNA sequence known as the terminator sequence. When the RNA polymerase encounters this sequence, it initiates the termination process.
- Release of the DNA template: Once the RNA polymerase transcribes the terminator sequence, it releases the DNA template strand. The DNA molecule then rewinds back into its double-helical structure.
In bacteria, there are two main methods of termination:
a. Rho-dependent termination: In this process, the RNA molecule contains a binding site for a protein called the Rho factor. The Rho factor binds to the DNA sequence and starts moving along the RNA transcript towards the RNA polymerase. When it reaches the transcription bubble, it pulls the RNA transcript and the DNA template strand apart. This releases the RNA molecule and terminates the transcription process. A specific DNA sequence located downstream of the termination site causes the RNA polymerase to pause, allowing the Rho factor to catch up and facilitate termination.
b. Rho-independent termination: This process relies on a specific sequence on the DNA template strand. As the RNA polymerase approaches the end of the gene being transcribed, it encounters a region rich in cytosine (C) and guanine (G) nucleotides. The RNA transcript from this region folds back on itself, forming a stable hairpin structure. The complementary C-G base pairing stabilizes the hairpin structure. Following the hairpin, there is a uracil (U) in the RNA terminator sequence that pairs with adenine (A) in the DNA template. The weak interaction between the U-A region and the stalled RNA polymerase leads to instability, causing the enzyme to dissociate from the DNA template and terminate transcription.
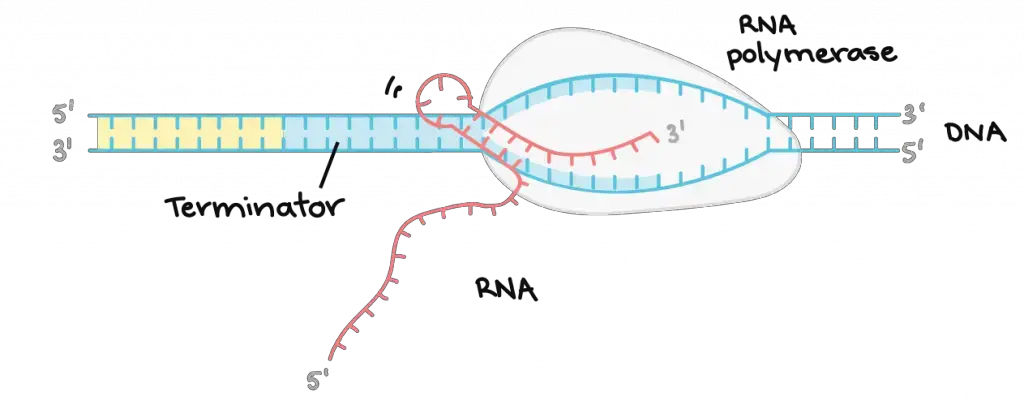
These termination mechanisms ensure the accurate and efficient completion of transcription, allowing for the production of functional RNA molecules.
Pre-translational mRNA processing
In eukaryotes the mRNA which has been translated is known as pre-mRNA. Therefore it needs to undergo additional procedures to develop into mature MRNA. These are referred to as pre-translational mRNA process. They are:
5′ Capping
- 5′ capping is a critical step in the pre-translational mRNA processing that occurs in eukaryotic cells. During this process, a methylated guanine cap is added to the 5′ end of the mRNA transcript, which plays important roles in mRNA recognition and stability.
- The addition of a methylated guanine cap involves the enzymatic modification of the mRNA molecule. Specifically, a modified guanine nucleotide, known as 7-methylguanosine, is added in a reverse orientation to the 5′ end of the mRNA transcript. This modification is carried out by a series of enzymatic reactions involving specialized enzymes known as capping enzymes.
- One of the primary functions of the 5′ cap is to facilitate the recognition of mRNA by ribosomes, the cellular machinery responsible for protein synthesis. The cap acts as a binding site for the ribosomes, allowing them to recognize and attach to the mRNA molecule. This recognition is crucial for the initiation of translation, where the ribosomes read the mRNA sequence and synthesize proteins based on the genetic information encoded within.
- In addition to its role in ribosome recognition, the 5′ cap also plays a crucial role in protecting the immature mRNA molecule from degradation by ribonucleases (RNases). RNases are enzymes that can break down RNA molecules, and they are present in the cellular environment. The presence of the 5′ cap helps shield the mRNA from the activity of these RNases, enhancing the stability of the molecule.
- By protecting the mRNA from degradation, the 5′ cap ensures that the mRNA remains intact and functional for a sufficient duration within the cell. This stability is vital for the efficient translation of the mRNA into proteins, allowing the cellular machinery to produce the proteins necessary for various cellular processes.
- Furthermore, the 5′ cap also participates in other regulatory processes. It influences mRNA export from the nucleus to the cytoplasm, where translation occurs. The cap interacts with specific proteins involved in mRNA transport, facilitating the export of the mRNA molecule to the cytoplasm.
Polyadenylation
- Polyadenylation is a critical process in pre-translational mRNA processing that occurs in eukaryotic cells. During polyadenylation, a poly(A) tail is added to the 3′ end of the mRNA molecule. This tail is composed of several molecules of adenosine monophosphate (AMP) and serves important functions in stabilizing the RNA molecule and protecting it from degradation.
- The addition of the poly(A) tail involves a series of enzymatic reactions. Initially, endonucleases cleave the mRNA molecule at a specific sequence downstream of the coding region. This cleavage site is determined by specific signals within the mRNA sequence. The cleavage reaction results in the formation of a free 3′ end.
- Subsequently, the enzyme poly(A) polymerase comes into action. It catalyzes the addition of multiple adenine nucleotides to the free 3′ end of the mRNA molecule. This enzymatic process continues until a poly(A) tail of the desired length is synthesized. The length of the poly(A) tail can vary, but it typically ranges from about 100 to 250 adenosine residues.
- The addition of the poly(A) tail is essential for stabilizing the mRNA molecule. RNA is inherently more unstable than DNA due to its susceptibility to degradation by various cellular enzymes. The presence of the poly(A) tail significantly enhances the stability of the mRNA molecule, allowing it to persist in the cell for a longer duration. The poly(A) tail acts as a protective shield, preventing the rapid degradation of the mRNA molecule by exonucleases.
- Moreover, the poly(A) tail plays important roles in mRNA processing and regulation. It is involved in the nuclear export of mRNA from the nucleus to the cytoplasm, where translation occurs. The poly(A) tail interacts with specific proteins that facilitate mRNA transport, ensuring the efficient transfer of the mRNA molecule to the site of protein synthesis.
- The poly(A) tail also participates in the regulation of mRNA stability and translation efficiency. Certain proteins that bind to the poly(A) tail can influence the rate of mRNA decay or enhance the efficiency of translation. Additionally, the length of the poly(A) tail can be dynamically regulated in response to cellular signals, affecting mRNA stability and translation.
Splicing
- Splicing is a crucial process in pre-translational mRNA processing that allows for the coding of multiple proteins from a single pre-mRNA molecule, thus conserving genetic material. It involves the removal of non-coding sequences called introns and the joining of coding sequences known as exons, ultimately leading to the production of mature mRNA.
- Splicing is carried out by a large molecular complex called the spliceosome. The spliceosome recognizes specific nucleotide sequences at the boundaries between introns and exons within the pre-mRNA transcript. It excises the introns through a series of enzymatic reactions, including cleavage and ligation. The introns are removed, and the exons are joined together to form a continuous coding sequence.
- This process of intron removal and exon joining is sequence-dependent, meaning it relies on specific nucleotide sequences within the transcript. The spliceosome accurately identifies the intron-exon boundaries based on these sequences, ensuring the correct removal of introns and the precise joining of exons. By removing introns and rejoining exons in different combinations, splicing allows for the generation of multiple protein variants from a single pre-mRNA molecule, thereby expanding the proteome diversity.
- Once the splicing process is complete, the mature mRNA molecule is formed. It carries the genetic information necessary for protein synthesis. The mature mRNA molecule possesses open reading frames (ORFs), which are regions that can be translated into proteins. Translation occurs in the ribosomes, where the mRNA sequence is read in three-nucleotide units known as codons. Each codon corresponds to a specific amino acid, and the ribosomes link these amino acids together to synthesize proteins.
- At the ends of the mature mRNA molecule, there are untranslated regions (UTRs). The 5′ UTR is located upstream of the coding sequence, while the 3′ UTR is located downstream. These UTRs do not participate in protein synthesis; instead, they play important regulatory roles. The UTRs can interact with various factors involved in mRNA stability, localization, and translation efficiency, influencing the overall protein production. They contribute to the fine-tuning of gene expression and can impact the protein’s function and cellular localization.
Differences Between Eukaryotic DNA Transcription and Prokaryotic DNA Transcription
Transcription in eukaryotes as well as prokaryotes are similar, but with some differentiating features.
Similarities
Common similarities include;
- DNA serves as the template for both organisms.
- The RNA polymerase is a key enzyme that is responsible for the whole process within both organisms
- The RNA molecule is the ultimate product of both organisms.
- The chemical structure for the transcript identical in both the organisms.
Differences
Differences in Eukaryotic DNA Transcription:
- Promoter:
- Multiple promoter elements such as TATA box, initiator elements, downstream core promoter, CAAT box, and CG box.
- RNA Polymerase:
- Three types of RNA polymerases (I, II, III) involved in transcription.
- Initiation:
- Formation of an initiation complex with various transcription factors that dissociate after initiation.
- Transcription and Translation Concurrence:
- Transcription must be completed before translation can begin.
- Post-Transcriptional Modifications:
- RNA undergoes post-transcriptional modifications like capping, polyadenylation, and splicing to form mature mRNA.
- RNA Genetics:
- Monocistronic mRNA, meaning a single gene is present on one mRNA molecule.
Differences in Prokaryotic DNA Transcription:
- Promoter:
- Three promoter elements (-10, -35 promoters) and upstream elements.
- RNA Polymerase:
- Single RNA polymerase involved in transcription.
- Initiation:
- No formation of an initiation complex.
- Transcription and Translation Concurrence:
- Transcription and translation occur simultaneously.
- Post-Transcriptional Modifications:
- No post-transcriptional modifications occur.
- RNA Genetics:
- Polycistronic mRNA, meaning multiple genes can be present on a single mRNA molecule.
- Termination:
- Termination aided by either Rho-dependent or Rho-independent factors.
These differences highlight the contrasting mechanisms and regulatory features of DNA transcription between eukaryotes and prokaryotes.
Aspect | Eukaryotic DNA Transcription | Prokaryotic DNA Transcription |
---|---|---|
Promoter | Multiple promoter elements (TATA box, initiator elements, downstream core promoter, CAAT box, CG box, etc.) | Three promoter elements (-10, -35 promoters) and upstream elements |
RNA Polymerase | Three types of RNA polymerases (I, II, III) involved in transcription | Single RNA polymerase involved in transcription |
Initiation | Formation of initiation complex with transcription factors that dissociate after initiation | No formation of an initiation complex |
Transcription and Translation Concurrence | Transcription must be complete before translation begins | Transcription and translation occur simultaneously |
Post-Transcriptional Modifications | RNA undergoes capping, polyadenylation, and splicing to form mature mRNA | No post-transcriptional modifications |
RNA Genetics | Monocistronic mRNA (single gene per mRNA molecule) | Polycistronic mRNA (multiple genes on a single mRNA molecule) |
Termination | Terminated by poly-adenylated (A) signal and downstream terminator sequence | Termination aided by Rho-dependent or Rho-independent factors |
What is Reverse Transcription?
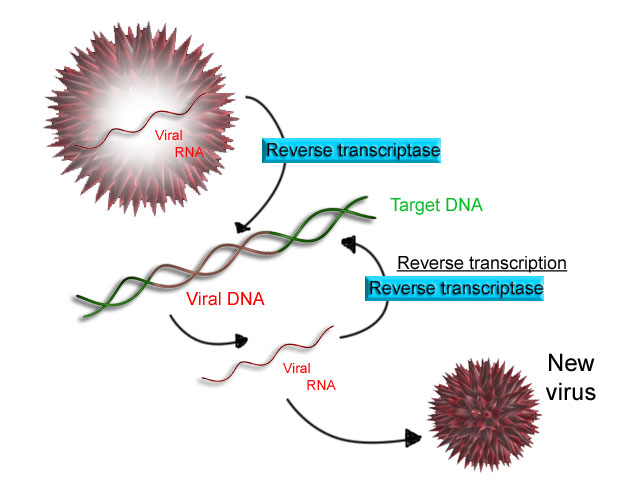
Reverse transcription is a process in which RNA is converted into DNA, resulting in the synthesis of a complementary DNA (cDNA) strand. It plays a significant role in various biological processes and is particularly important in the life cycle of certain viruses, such as HIV. Here are key points about reverse transcription:
- RNA to DNA Conversion: Reverse transcription involves the conversion of RNA molecules into DNA molecules. This process utilizes an enzyme called reverse transcriptase, which synthesizes a complementary DNA strand based on the RNA template.
- Retroviruses: RNA-encoded viruses, known as retroviruses, employ reverse transcription as a fundamental mechanism in their replication cycle. For example, HIV utilizes reverse transcriptase to convert its RNA genome into DNA.
- cDNA Synthesis: Reverse transcriptase synthesizes a complementary DNA strand, known as cDNA, by using the RNA template. This cDNA can then integrate into the host cell’s genome and direct the production of viral proteins, leading to the assembly of new viral particles.
- Telomerase: In addition to viral replication, reverse transcription is also involved in cellular processes. Telomerase, an enzyme found in some eukaryotic cells, exhibits reverse transcription activity. It extends the ends of linear chromosomes by synthesizing repetitive DNA sequences called telomeres. Telomerase helps maintain the integrity of chromosomes during replication.
- Telomere Lengthening and Cancer: Telomerase activation plays a crucial role in cancer cells. By lengthening the telomeres, telomerase enables cancer cells to continuously duplicate their genomes without losing essential DNA sequences. This activation of telomerase is associated with immortalization and the ability of cancer cells to proliferate indefinitely.
- Alternative Lengthening of Telomeres (ALT): In addition to telomerase, a small proportion of carcinogenic tumors use an alternative mechanism called Alternative Lengthening of Telomeres (ALT) to maintain telomere length. ALT provides an alternative pathway for telomere maintenance in the absence of telomerase activity.
Reverse transcription is not only essential for the replication of retroviruses like HIV but also plays a role in cellular processes like telomere maintenance. Understanding reverse transcription has important implications for both virology and cancer research. Furthermore, in the laboratory setting, reverse transcription is employed as a diagnostic tool for detecting RNA viruses, including HIV, hepatitis, influenza, and coronaviruses.
Inhibitors for Transcription
Transcription inhibitors are substances or compounds that impede the activity of the RNA polymerase enzyme, thereby interfering with the process of transcription. These inhibitors are often utilized to disrupt bacterial transcription in disease-causing pathogens. Here are some key points about transcription inhibitors:
- α-Amanitin: This inhibitor, derived from yeast, selectively targets RNA polymerase II and RNA polymerase III, hindering their activity.
- Rifampicin: A well-known transcription inhibitor, rifampicin specifically inhibits bacterial transcription by binding to the beta-subunit of DNA-dependent RNA polymerase.
- 8-Hydroxyquinoline: This compound acts as an antifungal transcription inhibitor, impeding the transcription process in fungi.
- Actinomycin D: Another commonly used transcription inhibitor, actinomycin D interferes with the action of RNA polymerase by binding to DNA, preventing its progression.
- CDK9 inhibitors: Examples include DRB (5,6-dichloro-1-beta-D-ribofuranosylbenzimidazole) and flavopiridol. These inhibitors target cyclin-dependent kinase 9 (CDK9), which plays a role in transcription regulation.
- Histone Methylation: Histone methylation is an inhibitory mechanism that can prevent transcription. Modifications to histone proteins can affect chromatin structure, making it more compact and less accessible for transcription factors and RNA polymerase.
Transcription inhibitors are not only useful as research tools but also have applications as antibiotics against bacteria and fungi. Rifampicin, for example, is an antibacterial transcription inhibitor that hampers bacterial transcription and is used in the treatment of tuberculosis. Similarly, compounds like 8-hydroxyquinoline act as antifungal agents by inhibiting fungal transcription.
Moreover, recent studies have explored natural products such as triptolide, which inhibit mammalian transcription by targeting specific components of the transcription machinery. These findings open up possibilities for the development of transcription inhibitors with therapeutic potential, particularly in targeting specific types of cancer cells.
How to Measure and detect DNA Transcription (RNA Synthesis)?
Transcription can be measured and detected using various techniques and assays. Here are some common methods for measuring and detecting transcription:
- G-Less Cassette Transcription Assay: This assay is used to measure the strength of a promoter. It involves inserting a G-less cassette into the promoter region and measuring the transcription activity of the cassette.
- Run-Off Transcription Assay: This assay helps identify the transcription start sites (TSS) by allowing RNA polymerase to initiate transcription from a specific promoter, followed by the detection of the synthesized RNA fragments.
- Nuclear Run-On Assay: This assay measures the relative abundance of newly formed transcripts by isolating nuclei from cells and incubating them with radiolabeled nucleotides, which are incorporated into newly synthesized RNA. The labeled RNA is then analyzed to determine the transcriptional activity.
- KAS-seq: This method measures single-stranded DNA generated by RNA polymerases. It enables the detection of transcription activity even with a small number of cells (e.g., 1,000 cells).
- RNase Protection Assay and ChIP-Chip of RNAP: These techniques are used to detect active transcription sites. The RNase Protection Assay involves hybridizing a labeled RNA probe to the target RNA, followed by RNase digestion to protect the hybridized regions. ChIP-Chip of RNAP (RNA Polymerase) combines chromatin immunoprecipitation (ChIP) with microarray analysis to identify regions associated with active transcription.
- RT-PCR: Reverse Transcription Polymerase Chain Reaction (RT-PCR) is a widely used method to measure the absolute abundance of total or nuclear RNA levels. It can provide quantitative information about RNA expression but may not directly reflect transcription rates.
- DNA Microarrays: DNA microarrays, also known as gene chips, measure the relative abundance of global RNA levels. They allow for the simultaneous detection of the expression levels of thousands of genes. However, the RNA levels measured by microarrays may not precisely reflect transcription rates.
- In Situ Hybridization: This technique detects the presence of a specific transcript within cells or tissues by hybridizing a labeled probe complementary to the target RNA sequence. It provides spatial information about RNA localization.
- MS2 Tagging: This method involves incorporating RNA stem loops, such as MS2, into a gene of interest. The stem loops can be detected using a fusion protein of GFP and the MS2 coat protein, which binds specifically to the MS2 stem loops. This approach allows visualization of transcription sites as fluorescent spots and has revealed the occurrence of transcriptional bursting.
- Northern Blot: This traditional method involves separating RNA samples by gel electrophoresis, transferring them to a membrane, and hybridizing with a labeled probe specific to the target RNA. It provides quantitative information about RNA abundance.
- RNA-Seq: RNA-Seq utilizes next-generation sequencing techniques to sequence the entire transcriptome. It allows the measurement of relative RNA abundance, detection of variations such as fusion genes and post-transcriptional edits, and identification of novel splice sites.
- Single-Cell RNA-Seq: This technique amplifies and reads partial transcriptomes from isolated single cells. It enables detailed analyses of RNA expression patterns in individual cells, providing insights into tissue heterogeneity, embryonic development, and cancer biology.
These various methods and assays offer researchers a range of options for measuring and detecting transcription, allowing them to study gene expression and regulatory processes in different biological contexts.
What happens to dna once transcription is done?
Once transcription is complete, the newly synthesized RNA molecule dissociates from the DNA template. The DNA molecule resumes its double-stranded structure, and the RNA molecule, called the primary transcript or pre-mRNA, undergoes several modifications and processing steps before it becomes a mature mRNA ready for translation into proteins. Here are the main events that occur after transcription:
- RNA Processing: In eukaryotic cells, pre-mRNA undergoes several modifications to generate a mature mRNA molecule. These modifications include:
- Addition of a 5′ cap: A modified guanine nucleotide is added to the 5′ end of the pre-mRNA. The cap structure helps protect the mRNA from degradation and assists in its export from the nucleus.
- Polyadenylation: A stretch of adenine nucleotides, called a poly(A) tail, is added to the 3′ end of the pre-mRNA. The poly(A) tail provides stability to the mRNA and plays a role in its translation.
- RNA splicing: Introns, non-coding regions within the pre-mRNA, are removed by a process called splicing. The exons, the coding regions, are joined together to form a continuous sequence that represents the mature mRNA.
- mRNA Export: Mature mRNA molecules are transported from the nucleus to the cytoplasm, where they will undergo translation to produce proteins. Export is facilitated by specific protein complexes that recognize the mRNA’s modified ends and transport it through nuclear pores.
- mRNA Degradation: In the cytoplasm, mRNA molecules have a finite lifespan. They can be degraded by cellular machinery, such as exonucleases and endonucleases, to control gene expression and remove unwanted or damaged transcripts.
- Translation: The mature mRNA is now ready for translation, the process by which the information in the mRNA sequence is used to synthesize proteins. Translation occurs on ribosomes, where transfer RNAs (tRNAs) bring the corresponding amino acids based on the mRNA codons, leading to protein synthesis.
It’s important to note that in prokaryotic cells, which lack a nuclear membrane, transcription and translation can occur simultaneously. In these cells, the mRNA synthesized by transcription is immediately available for translation without the need for additional processing steps seen in eukaryotic cells.
DNA replication vs transcription
Aspect | DNA Replication | Transcription |
---|---|---|
Process | Duplication of the entire DNA molecule | Synthesis of RNA molecules using a DNA template |
Enzyme involved | DNA polymerase | RNA polymerase |
Location | Nucleus (in eukaryotes) | Nucleus (in eukaryotes) or cytoplasm (in prokaryotes) |
Template | Both DNA strands serve as templates | One DNA strand (template strand) serves as a template |
Product | Two identical DNA molecules | RNA molecule |
Complementary bases | Adenine (A) pairs with Thymine (T) | Adenine (A) pairs with Uracil (U) |
Initiation | Initiated at specific origin sites | Initiated at specific promoter regions |
Elongation | DNA polymerase adds nucleotides to the growing DNA strand | RNA polymerase adds nucleotides to the growing RNA strand |
Termination | Replication forks meet at termination sites | Specific termination signals mark the end of transcription |
Occurrence | During the S phase of the cell cycle | Throughout the cell cycle |
Purpose | To produce an exact copy of the DNA molecule | To synthesize RNA molecules for protein synthesis |
Importance of DNA Transcription (RNA Synthesis)
DNA transcription, also known as RNA synthesis, plays a crucial role in the central dogma of molecular biology, which describes the flow of genetic information from DNA to RNA to protein. It is a fundamental process that is essential for gene expression and the synthesis of functional proteins. Here are some key reasons highlighting the importance of DNA transcription:
- Gene Expression: Transcription allows the transfer of genetic information encoded in DNA to RNA molecules, which can then be used to direct protein synthesis. It is a key step in gene expression, enabling the translation of genetic information into functional proteins.
- Protein Synthesis: Transcription produces different types of RNA molecules, including messenger RNA (mRNA), transfer RNA (tRNA), and ribosomal RNA (rRNA). mRNA serves as the template for protein synthesis during translation, while tRNA and rRNA are involved in the assembly of the ribosome and the proper positioning of amino acids for protein synthesis.
- Regulation of Gene Expression: Transcriptional control mechanisms play a vital role in regulating gene expression. Through the activation or repression of specific genes, cells can respond to internal and external signals, developmental cues, and environmental changes. Transcription factors, regulatory proteins, and various signaling pathways are involved in modulating transcriptional activity.
- Alternative Splicing: Pre-mRNA undergoes splicing, a process in which non-coding introns are removed, and coding exons are joined together. This mechanism enables the generation of multiple protein isoforms from a single gene, thereby increasing proteome diversity and functional complexity.
- Post-Transcriptional Modifications: RNA molecules undergo various post-transcriptional modifications, including capping, polyadenylation, and RNA editing. These modifications contribute to the stability, processing, and functionality of RNA molecules, ensuring their proper function in gene regulation and protein synthesis.
- Disease Mechanisms: Dysregulation of DNA transcription can have significant implications for human health. Mutations or alterations in transcription factors, promoter regions, or regulatory elements can lead to aberrant gene expression patterns, contributing to the development of genetic disorders, cancer, and various diseases.
Understanding the importance of DNA transcription is crucial for unraveling the complexities of gene regulation, protein synthesis, and the underlying mechanisms of various biological processes. It provides insights into cellular functions, development, disease mechanisms, and potential targets for therapeutic interventions.
Is dna directly involved in transcription?
Yes, DNA is directly involved in transcription. Transcription is the process by which an RNA molecule is synthesized using a DNA template. It takes place in the nucleus of eukaryotic cells or in the cytoplasm of prokaryotic cells.
During transcription, an enzyme called RNA polymerase binds to a specific region of the DNA known as the promoter. The DNA double helix is unwound, and the RNA polymerase starts moving along one strand of the DNA, known as the template strand. As it moves, the RNA polymerase adds complementary RNA nucleotides to the growing RNA molecule, based on the sequence of the DNA template.
The DNA molecule acts as a template for the synthesis of the RNA molecule. The DNA sequence determines the sequence of nucleotides in the RNA molecule, with the exception that RNA uses uracil (U) instead of thymine (T) as one of its bases. The RNA molecule synthesized during transcription is complementary to the DNA template strand and is referred to as the primary transcript or pre-mRNA.
So, DNA provides the template and the genetic information for the synthesis of RNA during the process of transcription.
FAQ
What is DNA transcription?
DNA transcription is the process by which an RNA molecule is synthesized from a DNA template. It involves the production of an RNA strand that is complementary to the DNA sequence.
What is the enzyme responsible for DNA transcription?
The enzyme responsible for DNA transcription is called RNA polymerase. It binds to the DNA template and catalyzes the synthesis of RNA by adding complementary nucleotides.
How does DNA transcription start?
DNA transcription begins with the binding of RNA polymerase to the promoter region of the DNA. The promoter sequence acts as a signal for the start of transcription.
What are the types of RNA produced during transcription?
Three main types of RNA are produced during transcription: messenger RNA (mRNA), transfer RNA (tRNA), and ribosomal RNA (rRNA). Each type has a specific role in protein synthesis.
What is the role of transcription factors in DNA transcription?
Transcription factors are proteins that bind to specific DNA sequences and regulate the activity of RNA polymerase. They help initiate or enhance transcription by interacting with the promoter region.
What is the significance of RNA splicing in transcription?
RNA splicing is the process of removing non-coding sequences (introns) from pre-mRNA and joining together the coding sequences (exons). It increases protein diversity by allowing different combinations of exons to be included in the final mRNA.
How is transcription different in prokaryotes and eukaryotes?
In prokaryotes, transcription occurs in the cytoplasm and is coupled with translation. In eukaryotes, transcription occurs in the nucleus and is followed by RNA processing before the mRNA is transported to the cytoplasm for translation.
What are the factors that regulate transcription?
Transcriptional regulation is controlled by various factors, including transcription factors, enhancers, repressors, chromatin structure, DNA methylation, and signaling pathways. They influence the activity of RNA polymerase and the accessibility of DNA for transcription.
Can DNA transcription be influenced by environmental factors?
Yes, environmental factors such as temperature, pH, chemicals, and stress can affect the regulation of transcription. They can alter the binding of transcription factors or modify the chromatin structure, leading to changes in gene expression.
How is DNA transcription studied in the laboratory?
Scientists use various techniques to study DNA transcription, such as RT-PCR (Reverse Transcription Polymerase Chain Reaction), RNA sequencing (RNA-Seq), microarrays, and reporter gene assays. These methods provide insights into gene expression patterns, transcriptional regulation, and the functional roles of specific genes.
Where does DNA transcription occur?
DNA transcription occurs in the nucleus of eukaryotic cells and in the cytoplasm of prokaryotic cells. In eukaryotes, the process of transcription begins in the nucleus, where the DNA is located. The DNA is transcribed into RNA by RNA polymerase enzymes. The resulting RNA molecule, known as pre-mRNA, undergoes further processing, such as splicing, capping, and polyadenylation, before it is transported out of the nucleus to the cytoplasm.
In prokaryotes, which lack a nucleus, transcription occurs in the cytoplasm. The DNA is present in the cytoplasm in the form of a single circular chromosome. RNA polymerase binds to the DNA at specific promoter regions and synthesizes RNA directly from the DNA template. The resulting RNA molecule, called messenger RNA (mRNA), can be immediately used for protein synthesis within the cytoplasm.
Overall, while the location of transcription differs between eukaryotes and prokaryotes, both processes are essential for gene expression and protein synthesis.
References
- Alberts, B., Johnson, A., Lewis, J., Raff, M., Roberts, K., & Walter, P. (2002). Molecular Biology of the Cell (4th edition). Garland Science.
- Lodish, H., Berk, A., Zipursky, S. L., Matsudaira, P., Baltimore, D., & Darnell, J. (2000). Molecular Cell Biology (4th edition). W. H. Freeman.
- Watson, J. D., Baker, T. A., Bell, S. P., Gann, A., Levine, M., & Losick, R. (2014). Molecular Biology of the Gene (7th edition). Pearson.
- Lewin, B. (2007). Genes IX. Jones & Bartlett Publishers.
- Berg, J. M., Tymoczko, J. L., & Gatto, G. J. (2015). Stryer’s Biochemistry (8th edition). W. H. Freeman.