Streptococcus pneumoniae Overview
Streptococcus pneumoniae, commonly known as pneumococcus, is a Gram-positive, spherical bacterium. It is alpha-hemolytic and belongs to the genus Streptococcus. Discovered by Louis Pasteur and George Sternberg in the late 19th century, it is a significant human pathogen, especially noted for causing pneumonia.
S. pneumoniae typically appears as lancet-shaped diplococci, meaning it occurs in pairs. It is non-motile and does not form spores. This bacterium resides asymptomatically in healthy individuals, primarily colonizing the respiratory tract, sinuses, and nasal cavity. However, it can become pathogenic in people with weakened immune systems, such as the elderly and young children. Transmission occurs through direct contact via respiratory droplets.
Pneumococcus is the leading cause of community-acquired pneumonia and meningitis in children and the elderly. It is also responsible for sepsis in individuals with HIV. Other pneumococcal infections include bronchitis, rhinitis, acute sinusitis, otitis media, conjunctivitis, septic arthritis, endocarditis, peritonitis, pericarditis, cellulitis, and brain abscess.
The bacterium can be distinguished from other alpha-hemolytic streptococci by its sensitivity to optochin and lysis by bile, known as the bile solubility test. A critical feature of S. pneumoniae is its polysaccharide capsule, which acts as a virulence factor. This capsule helps the bacterium evade the host’s immune system by inhibiting phagocytosis.
Over 100 different serotypes of S. pneumoniae exist, each varying in virulence and resistance to antibiotics. The capsular polysaccharide (CPS) serves as a primary defense mechanism against the host’s immune response. It forms the outermost layer of encapsulated strains and prevents granulocytes from accessing the cell wall.
Historically, S. pneumoniae played a pivotal role in genetic research. In 1928, Frederick Griffith’s experiment demonstrated the transformation capability of pneumococcus, leading to the discovery that DNA is the genetic material. This groundbreaking work laid the foundation for modern molecular genetics.
Vaccination efforts against S. pneumoniae began in the early 20th century, with the first pneumococcal vaccine produced in the United States in 1977. The introduction of the conjugate vaccine in 2000 significantly improved protection against various serotypes.
Characteristics of Streptococcus pneumoniae
- Cell Morphology
- Shape: Streptococcus pneumoniae cells are lancet-shaped, elongated cocci with a slightly pointed outer curvature.
- Arrangement: Commonly observed as pairs of cocci (diplococci), they can also appear singly or in short chains.
- Size: The diameter of each cell ranges between 0.5 and 1.25 micrometers.
- Growth and Hemolysis
- Blood Agar: When grown on blood agar, S. pneumoniae exhibits alpha hemolysis, which is partial hemolysis resulting in a greenish discoloration around colonies.
- Non-Motile and Non-Spore Forming
- Mobility: These bacteria do not produce spores and are immobile.
- Catalase Negative: They lack catalase, an enzyme that breaks down hydrogen peroxide.
- Metabolic Traits
- Fermentation: Like other streptococci, S. pneumoniae ferments glucose into lactic acid.
- Inulin Hydrolysis: Unlike other streptococci, they can hydrolyze inulin.
- Cell Wall Composition
- Peptidoglycan and Teichoic Acid: S. pneumoniae has a unique peptidoglycan and teichoic acid composition in its cell wall.
- Capsule: The polysaccharide capsule acts as a critical virulence factor, inhibiting phagocytosis by the host immune system.
- Lack of M Protein
- M Protein: Unlike other streptococci, S. pneumoniae lacks M protein, a surface protein that prevents immune recognition.
- Habitat
- Upper Respiratory Tract: They are part of the normal flora of the upper respiratory tract in humans, typically located in the throat and nasal passages.
- Seasonal Infection Patterns
- Winter Infections: S. pneumoniae infections are more common in children during the winter months.
- Optimal Growth Temperature
- Temperature Range: The optimal growth temperature for S. pneumoniae ranges from 30 to 35°C.
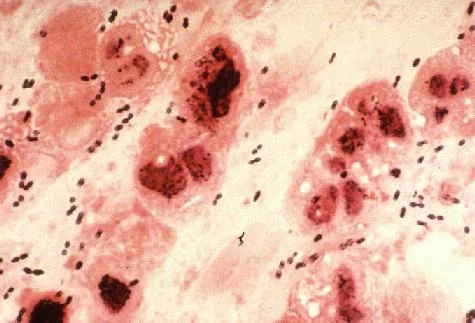
Streptococcus pneumoniae, also known as pneumococcus, is a type of bacteria that typically inhabits the upper respiratory tract of humans, including the nose and throat. It can also be found in other parts of the body, such as the lungs and ears. The bacteria can be transmitted through respiratory droplets, and can cause a variety of infections, including pneumonia, meningitis, and sepsis.
Where is streptococcus pneumoniae found?- Habitat of Streptococcus pneumoniae
- Global Distribution
- Prevalence: Streptococcus pneumoniae is found worldwide, affecting various species.
- Hosts
- Humans: It is part of the normal flora of the upper respiratory tract in humans.
- Location: Primarily colonizes the throat and nasal passages.
- Animals: Also found in primates, livestock, and felines.
- Humans: It is part of the normal flora of the upper respiratory tract in humans.
- Seasonal Patterns
- Infections: The bacterium tends to cause infections more frequently in children during the winter months.
- Temperature Preferences
- Mesophilic Nature: S. pneumoniae thrives at temperatures ranging from 30 to 35°C, indicating its preference for moderate temperatures.
How is streptococcus pneumoniae primarily transmitted?
Streptococcus pneumoniae (S. pneumoniae) is primarily transmitted through:
- Respiratory droplets: S. pneumoniae is spread through respiratory droplets that contain the bacteria, which are generated when an infected person talks, coughs, or sneezes.
- Close contact: Transmission occurs through close contact with an infected person, such as touching, shaking hands, or sharing utensils.
- Fomites: The bacteria can survive on surfaces and objects (fomites) for a short period, allowing transmission through indirect contact.
- Vertical transmission: Mother-to-child transmission during childbirth or through breast milk.
- Asymptomatic carriers: Healthy individuals can carry S. pneumoniae in their nasopharynx without showing symptoms, serving as reservoirs for transmission.
High-risk groups:
- Young children
- Older adults
- People with weakened immune systems (e.g., HIV/AIDS, cancer)
- Those with chronic medical conditions (e.g., heart disease, diabetes)
Prevention measures:
- Vaccination (PCV and PPSV)
- Good hygiene practices (handwashing, proper disposal of respiratory secretions)
- Avoiding close contact with infected individuals
- Using masks in crowded areas or during outbreaks
Morphology of Streptococcus pneumoniae
- Gram Stain Characteristics
- Gram-Positive: Streptococcus pneumoniae is classified as a Gram-positive bacterium.
- Staining Variation: In older cultures, it may lose its Gram-positive characteristic and appear Gram-negative.
- Gram-Positive: Streptococcus pneumoniae is classified as a Gram-positive bacterium.
- Cell Structure
- Non-Sporing: The bacteria do not produce spores.
- Non-Motile: S. pneumoniae lacks motility.
- Shape and Arrangement
- Size: The cells are approximately 0.5 x 1.25 micrometers in diameter.
- Shape: They exhibit a lancet-shaped or bullet-shaped morphology.
- Arrangement: S. pneumoniae is commonly observed as pairs (diplococci), but can also appear singly or in short chains when isolated from body fluids.
- Surface Features
- Hemolysis and Surface Proteins
- Hemolysis: S. pneumoniae is classified as alpha (α) hemolytic, meaning it partially lyses red blood cells.
- Surface Proteins: The bacterium contains more than 500 different surface proteins.
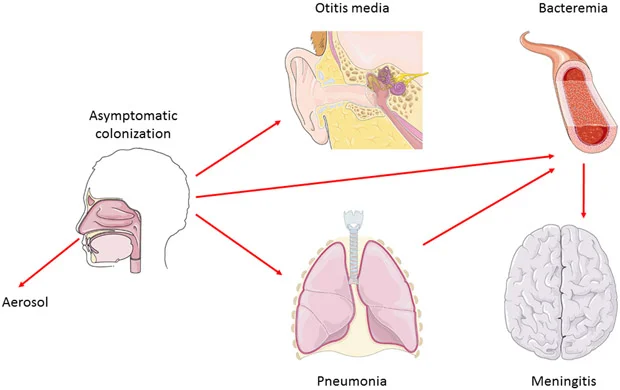
Genomes of Streptococcus pneumoniae
- Chromosome Structure
- Type: Streptococcus pneumoniae has a single, circular chromosome.
- Size: The chromosome is approximately 2 million base pairs in length.
- Genomic Composition
- GC Content: The genome has a GC content of 39.7%, reflecting the proportion of guanine and cytosine nucleotides.
- Genetic Coding
- Proteins: The genome encodes for 1,914 proteins.
- Functions: These proteins include various enzymes, structural components, and virulence factors essential for bacterial survival and pathogenicity.
- RNA: It also includes 73 RNA genes.
- Types: This RNA consists of ribosomal RNA (rRNA) and transfer RNA (tRNA), which are critical for protein synthesis and other cellular processes.
- Proteins: The genome encodes for 1,914 proteins.
Epidemiology of Streptococcus pneumoniae
- Global Distribution
- Prevalence: Streptococcus pneumoniae infections are widespread globally. They are particularly common during winter and early spring.
- Colonization: The bacterium frequently colonizes the nasopharynx, contributing to its global distribution.
- Carriage Rates
- Healthy Carriers: In healthy populations, 40%-50% of children and 20%-30% of adults carry the bacterium asymptomatically.
- Impact of Vaccination: The introduction of childhood conjugate vaccines has reduced colonization rates.
- Healthy Carriers: In healthy populations, 40%-50% of children and 20%-30% of adults carry the bacterium asymptomatically.
- Risk Groups
- Age: The bacterium affects all age groups, but is more prevalent in individuals over 65 years and those under 2 years.
- Additional Risks: Higher incidence is observed among smokers, individuals who abuse alcohol, those with asthma or chronic obstructive pulmonary disease (COPD), and asplenic individuals.
- Incidence Rates
- United States: The rate of confirmed Streptococcus pneumoniae infections ranges from 5.16 to 6.11 cases per 100,000 adults.
- Specific Rates: For those older than 65 years, the rate is 36.4 per 100,000, while for infants younger than 1 year, it is 34.2 per 100,000.
- United States: The rate of confirmed Streptococcus pneumoniae infections ranges from 5.16 to 6.11 cases per 100,000 adults.
- Global Impact
- Mortality: In 2005, the World Health Organization estimated that 1.6 million deaths were attributable to Streptococcus pneumoniae, including 1 million children under 5 years.
- Co-infection: Streptococcus pneumoniae is a frequent co-infection in patients with influenza, significantly impacting morbidity and mortality rates.
Cultivation of Streptococcus pneumoniae
- Streptococcus pneumoniae is a fussy bacteria that prefers 5% carbon dioxide for optimal growth. Approximately 20% of fresh clinical isolates necessitate anaerobic conditions.
- In all instances, growth requires a source of catalase (such as blood) to neutralise the significant amount of hydrogen peroxide created by the bacteria. At 37 degrees Celsius, the doubling time of the bacterium in complex media including blood is 20 to 30 minutes.
- On agar, pneumococci grow as 1 mm in diameter, sparkling colonies. Types 3 and 37 are mucoid serotypes. At a rate of 1 in 105, pneumococci undergo a genetically regulated phase transition from opaque to transparent colonies.
- The transparent variety is adapted for colonisation of the nasopharynx, whereas the opaque variant is adapted for blood survival. The molecular reason for the difference in colony appearance is unknown, however the expression of surface proteins differs significantly between the two types.
- Streptococcus pneumoniae is an aerotolerant fermenting anaerobe. It is typically grown in medium containing blood. On blood agar, colonies create a zone of alpha (green) hemolysis, which distinguishes S. pneumoniae from the group A (beta hemolytic) streptococcus, but not from commensal alpha hemolytic (viridans) streptococci that occupy the upper respiratory tract.
- To distinguish pneumococcus from Streptococcus viridans, specialised assays, such as inulin fermentation, bile solubility, and optochin (an antibiotic) sensitivity, must be used routinely.
- Streptococcus pneumoniae is an exceptionally delicate bacteria that possesses the enzymatic capacity to break and disintegrate cells. The relevant enzyme is known as an autolysin. This autolysin’s physiological function is to trigger a distinctive autolysis that kills the entire culture when grown to stationary phase.
- Almost all clinical isolates of pneumococci contain this autolysin and undergo lysis between 18 and 24 hours following growth initiation under optimum circumstances.
- Autolysis is associated with colony morphological alterations. Colonies initially have a plateau-like morphology, but when autolysis occurs, the centres begin to collapse.
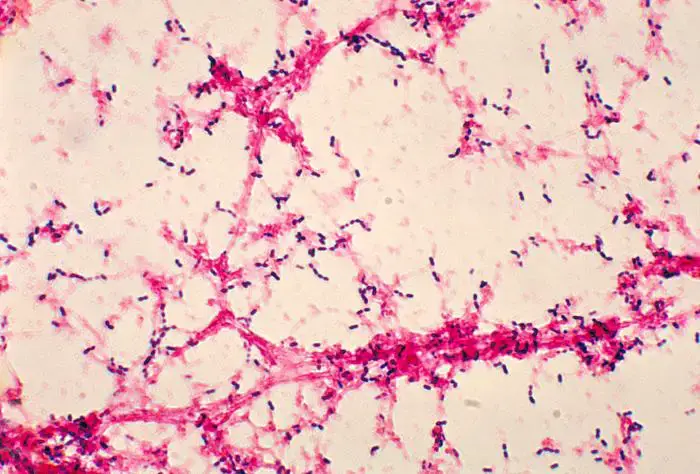
Cell Surface Structure of Streptococcus pneumoniae
1. Capsule
- Pneumococcal cells are entirely enveloped by a polysaccharide capsule. During invasion, the capsule is an important virulence factor.
- By blocking complement C3b from opsonizing bacterial cells, the capsule inhibits phagocytosis. The antigenic serotyping of the organism is based on the identification of 90 different types of pneumococcal capsules.
- Based on formulations of several capsular (polysaccharide) antigens taken from highly prevalent strains, anti-pneumococcal vaccines are created.
2. Cell Wall
- The cell wall of S. pneumoniae is made of peptidoglycan with teichoic acid connected to approximately every third N-acetylmuramic acid and is approximately six layers thick.
- Lipoteichoic acid is chemically identical to teichoic acid, but has a lipid moiety linked to the cell membrane.
- Both teichoic acid and lipoteichoic acid contain phosphorylcholine; two choline residues can be covalently attached to each repeating carbohydrate.
- Choline binds particularly to choline-binding receptors that are present on practically all human cells, making this a crucial component of S. pneumoniae’s biology.
3. Pili
- Recent research has revealed that several strains of S. pneumoniae possess hair-like structures that extend from the surface.
- It has been established that they contribute to colonisation of the upper respiratory tract and boost the immune system’s production of TNF during invasive infection.
4. Surface Proteins
- The pneumococcus is believed to have more than 500 surface proteins based on functional genomic research. Some are physically connected with the cell wall, whereas others are membrane-associated lipoproteins.
- Five penicillin-binding proteins (PBPs), two neuraminidases, and an IgA protease are present in the latter. The family of choline-binding proteins is a distinct group of proteins on the pneumococcal surface (CBPs).
- Twelve CBPs are noncovalently attached to the choline portion of the bacterial cell wall and are utilised to “snap” several functional components onto the bacterial surface.
- CBPs have a C-terminal choline-binding domain, but their N-termini are unique, indicating that their activities are separate. Important virulence factors within the CBP family include PspA (protective antigen), LytA, B, and C (three autolysins), and CbpA. (an adhesin).
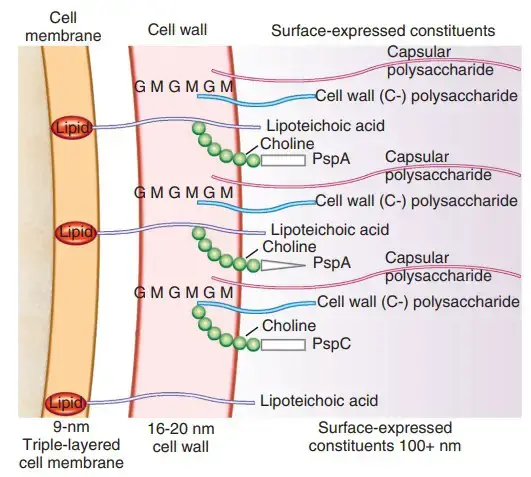
Identification of Streptococcus pneumoniae
- Pneumococci can be identified and distinguished from other streptococci based on their sensitivity to bile or optochin, Gram-positive staining, and hemolytic activity.
- On agar containing equine, human, rabbit, and ovine erythrocytes, pneumococci cause alpha hemolysis.
- Under anaerobic conditions, they switch to beta hemolysis, which is produced by an oxygen-sensitive hemolysin.
- Pneumococci typically form a 16-mm zone of inhibition around a 5 mg disc of optochin and are lysed by bile salts (e.g. deoxycholate).
- A few drops of 10% deoxycholate added at 37 degrees Celsius lyses the entire colony within minutes.
- Deoxycholate’s capacity to breakdown the cell wall is contingent on the presence of the autolytic enzyme LytA. Practically all pneumococci clinical isolates include autolysin and undergo deoxycholate lysis.
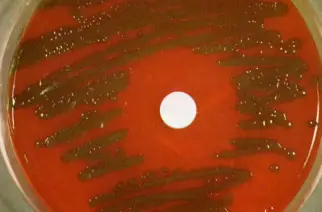
Diseases caused by Streptococcus pneumoniae
Streptococcus pneumoniae is a significant pathogen responsible for a range of diseases, primarily affecting various age groups and presenting in several forms. The key diseases caused by this bacterium include:
1. Bacterial Pneumonia
- Overview: S. pneumoniae is the leading bacterial cause of pneumonia. It primarily affects infants and the elderly, who are more vulnerable.
- Mechanism: When pneumococci invade the alveoli, they trigger an inflammatory response. This results in the release of fluid, red blood cells, and white blood cells into the alveolar spaces.
- Symptoms: Patients typically present with “rusty sputum,” a characteristic brownish-red color of expectorated mucus.
- Diagnosis: Diagnosis is often confirmed through sputum analysis and imaging studies like chest X-rays.
2. Meningitis
- Overview: S. pneumoniae is a leading cause of bacterial meningitis, particularly in adults and young children.
- Characteristics: The cerebrospinal fluid (CSF) in affected individuals usually shows elevated white blood cells (WBCs) and reduced glucose levels.
- Symptoms: Symptoms may include fever, headache, neck stiffness, and altered mental status.
- Diagnosis: CSF analysis and culture are crucial for diagnosis.
3. Otitis Media
- Overview: This condition, an infection of the middle ear, is prevalent among children.
- Symptoms: Common signs include ear pain, fever, and irritability.
- Diagnosis: Diagnosis is typically based on clinical examination and otoscopic findings.
4. Sinusitis
- Overview: S. pneumoniae can cause sinusitis, particularly in children.
- Symptoms: Symptoms include nasal congestion, facial pain, and purulent nasal discharge.
- Diagnosis: Diagnosis is generally confirmed through clinical evaluation and imaging studies.
5. Bronchitis
- Overview: Though less common than pneumonia, S. pneumoniae can also cause bronchitis.
- Symptoms: Patients may experience cough, mucus production, and wheezing.
- Diagnosis: Diagnosis involves clinical assessment and, if necessary, sputum cultures.
6. Bacteremia and Other Infectious Processes
- Overview: S. pneumoniae can enter the bloodstream, leading to bacteremia and septicemia.
- Symptoms: Symptoms vary widely but may include fever, chills, and signs of systemic infection.
- Diagnosis: Blood cultures and clinical evaluation are essential for diagnosis.
Virulence factors and Pathogenesis of Streptococcus pneumoniae
Streptococcus pneumoniae exhibits several key virulence factors that contribute to its pathogenicity. Understanding these factors is crucial for comprehending how this bacterium causes disease.
1. Polysaccharide Capsule
- Major Virulence Factor: The polysaccharide capsule is the primary virulence factor of S. pneumoniae. It acts as an antiphagocytic barrier, preventing phagocytosis by immune cells.
- Serotype Diversity: Over 80 serotypes exist, each with a distinct polysaccharide capsule. This diversity enables the bacterium to evade immune detection and provides type-specific immunity through antibodies.
- Function: By inhibiting phagocytosis, the capsule facilitates the bacterium’s survival and proliferation within the host.
2. Peptidoglycan and Teichoic Acids
- Inflammatory Response: Peptidoglycan and teichoic acids, components of the bacterial cell wall, elicit strong inflammatory responses in the host. This is particularly notable in the central nervous system during infections like meningitis.
- Function: These components contribute to the pathogen’s ability to provoke inflammation, which can exacerbate tissue damage and disease severity.
3. IgA Protease
- Colonization: IgA protease aids in colonization by degrading immunoglobulin A (IgA) present on mucosal surfaces. This enzyme helps the bacterium persist in the upper respiratory tract.
- Function: By neutralizing IgA, the protease reduces the immune system’s ability to prevent colonization.
4. Teichoic Acids
- Adherence: Teichoic acids facilitate adherence to host tissues, particularly within the nasopharynx and respiratory tract.
- Function: These acids help the bacterium establish itself on mucosal surfaces, an essential step in colonization and infection.
5. Pneumolysin O
- Hemolysin and Cytolysin: Pneumolysin O is a potent hemolysin and cytolysin that damages respiratory epithelial cells.
- Mechanism: It is similar to streptolysin O in its ability to damage eukaryotic cells. Pneumolysin O inhibits leukocyte respiratory burst and interferes with classical complement pathway activation.
- Function: This damage contributes to tissue destruction and enhances bacterial survival by evading immune responses.
Reservoirs and Transmission
- Reservoirs: S. pneumoniae harmlessly resides in the upper respiratory tract of humans. It often colonizes asymptomatically without causing disease.
- Transmission: Transmission occurs via respiratory droplets. However, it is not considered highly communicable compared to other pathogens. The bacterium can be present in the throat without necessarily causing infection.
Genetics or Genome of Streptococcus pneumoniae
- S. pneumoniae utilises a natural transformation pathway for genetic exchange. This process is medically significant since it clearly explains the explosive growth of antibiotic resistance in bacteria during the last two decades.
- For instance, penicillin resistance is a result of modified penicillin-binding proteins (PBPs) with reduced affinity for beta lactam antibiotics. Comparing the nucleotide sequences encoding PBPs in S. pneumoniae and S. mitis reveals horizontal gene transfer between these two bacteria.
- S. pneumoniae can be changed in the laboratory utilising genes from related and unrelated bacteria.
- In addition, horizontal exchanges of genetic information may occur in the upper respiratory tract of the host between strains of pneumococci that coexist or struggle for dominance with normal flora.
- Streptococcus pneumoniae can also gain resistance to antibiotics through the timeless process of mutation and natural selection.
- The bacterium has a relatively rapid growth rate and attains high cell densities in an infectious environment. These conditions not only enhance the occurrence of natural transformation, but also the creation of antibiotic-resistant mutants that arise spontaneously.
- The binding, absorption, and assimilation of exogenous DNA during transformation occur as a sequence of regulated actions during a physiologically defined condition called competence.
- Competent bacteria self-aggregate, readily generate protoplasts, are susceptible to autolysis, and have a higher concentration of H+ and Na+, all of which contribute to an increase in glycolysis and ATP reserves.
- During competence, a distinct set of at least 11 proteins is preferentially expressed. Growing bacteria release a 17-amino acid peptide known as competence-stimulating peptide (CSP) early in the competent stage.
- Consistent with a quorum-sensing hypothesis, CSP produces competence when it reaches a critical concentration that relies on the cell density.
Pathogenesis of Streptococcus pneumoniae
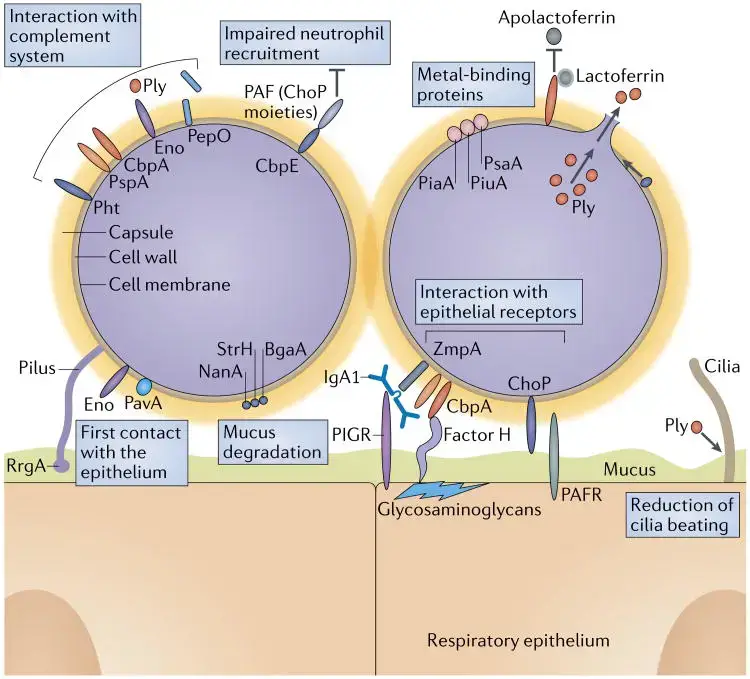
The pathogenesis of Streptococcus pneumoniae involves a series of well-orchestrated events that facilitate colonization, invasion, and disease development. Here is a detailed examination of these processes:
1. Colonization
- Initial Attachment: S. pneumoniae adheres strongly to the nasopharyngeal epithelium through various mechanisms. This attachment is often type-specific, meaning immunity can be generated but is specific to particular serotypes.
- Neuraminidase Activity: The bacterium utilizes neuraminidase to alter surface receptors on epithelial cells, aiding in adherence and colonization.
- Surface Receptor Alteration: Changes in receptor structure facilitate the bacteria’s attachment and persistence in the nasopharynx.
- Spread to Middle Ear: Pneumococci can migrate from the nasopharynx to the middle ear through the Eustachian tube.
- Inflammatory Response: The bacteria induce inflammation in the middle ear by promoting alterations in epithelial surface receptors and causing cytotoxicity to cochlear ciliated cells.
- Movement to Lower Respiratory Tract: If the bacteria bypass the ciliated upper respiratory epithelium, they enter the lower respiratory tract.
- Alveolar Interaction: Pneumococci attach to alveolar cells that produce surfactant. This attachment often requires a significant bacterial load (around 100,000 bacteria/ml) to trigger an inflammatory response, although a lower number (about 10 bacteria) can induce inflammation if a proinflammatory signal, such as a cytokine or viral infection, is present.
2. Invasion
- Resistance to Phagocytosis: S. pneumoniae evades phagocytic clearance through its virulence factors, including its polysaccharide capsule.
- Inflammatory Cascades: The bacterium’s cell wall components activate various inflammatory pathways, including the complement system, coagulation cascade, and cytokine production. Key cytokines such as interleukin-1, interleukin-6, and tumor necrosis factor (TNF) are released.
- Cytotoxic Effects: Pneumolysin and hydrogen peroxide are released by lysed pneumococci, which exacerbate tissue damage and inflammation.
- Nitric Oxide Production: The synthesis of nitric oxide during this process may contribute to septic shock.
- Altered Vascular Permeability: Pneumococcal interaction with the PAF receptor G-protein on endothelial cells leads to increased vascular permeability.
- Exudate Formation: Initially, a serous exudate forms in the lungs, which transitions to a purulent exudate as leukocytes infiltrate the site. This progression indicates the severity of the infection.
3. Direct Endothelial Cell Interaction
- Binding and Endocytosis: Pneumococci bind directly to endothelial cells through choline on cell wall teichoic acids and choline-binding protein CbpA.
- Receptor-Mediated Endocytosis: The bacteria are internalized into vacuoles and released on the opposite side of the endothelial barrier, facilitating their passage through the barrier.
- Transmigration to the Brain: In cases of bacteremia, S. pneumoniae can adhere to brain capillaries using the same choline-mediated binding mechanisms.
- Cerebrospinal Fluid Invasion: Once in the cerebrospinal fluid, pneumococcal cell wall components provoke a strong inflammatory response, leading to meningitis.
Bacterial Determinants of Virulence
Understanding the bacterial determinants of virulence in Streptococcus pneumoniae is essential for grasping how this pathogen causes disease. These determinants include pili, capsules, cell wall components, choline-binding proteins, hemolysins, hydrogen peroxide, and various exoenzymes.
1. Pili
- Function: Pili enhance initial bacterial adherence to epithelial cells in the upper respiratory tract.
- Pili Structure: Encoded by the rlrA islet, which is present in some clinical isolates but not all. The rlrA islet enables the expression of pili.
- Impact on Virulence: Pilus-expressing strains show improved adhesion to lung epithelial cells and increased virulence in animal models compared to nonpiliated strains.
- Inflammatory Response: Piliated pneumococci elicit a more robust tumor necrosis factor (TNF) response during systemic infection, indicating their role in driving the host’s inflammatory response.
2. Capsule
- Function: The capsule of S. pneumoniae interferes with phagocytosis by leukocytes.
- Composition: The capsule’s polysaccharide structure helps evade immune detection and is crucial for resistance to phagocytosis.
- Virulence: Encapsulated strains are significantly more virulent than unencapsulated ones, being 100,000 times more effective in causing disease.
- Immunity: The capsule does not act as an antigenic disguise but prevents opsonization by C-reactive protein or antibodies to teichoic acid.
3. Cell Wall Components
- Function: Pneumococcal cell wall components are strong inflammatory triggers.
- Phosphorylcholine: Located on teichoic acid and lipoteichoic acid, it facilitates bacterial adhesion and invasion. Phosphorylcholine also serves as a docking site for choline-binding proteins (CBPs).
- Inflammatory Pathways: The cell wall activates the alternative complement pathway, coagulation cascade, and cytokine production, leading to inflammation and tissue damage.
- C-Reactive Protein Interaction: Pneumococci subvert the C-reactive protein response to evade immune detection.
4. Choline-Binding Proteins (CBPs)
- Key Proteins:
- PspA (Protective Antigen): A 6 kDa protein that suppresses complement-mediated opsonization, enhancing bacterial survival.
- LytA, LytB, and LytC: Autolysins involved in pneumococcal lysis and cell separation.
- CbpA: An adhesin with eight choline-binding repeats, crucial for binding to pulmonary epithelial cells and other human cells. CbpA-deficient mutants show reduced colonization and binding abilities.
- Functions: CBPs play roles in bacterial adherence, immune evasion, and influencing host immune responses.
5. Hemolysins
- Pneumolysin:
- Function: Pneumolysin is a potent hemolysin that can activate complement and lyse host cells. It forms oligomers that create transmembrane pores, leading to cell death.
- Effects: Pneumolysin promotes inflammation, disrupts epithelial cell function, inhibits lymphocyte proliferation, and reduces neutrophil activity.
- Other Hemolysins: An additional hemolysin activity has been identified, though its exact identity remains unknown. Pneumococci also produce high levels of hydrogen peroxide, contributing to bacterial and host cell damage.
6. Hydrogen Peroxide
- Function: Produced in greater amounts than by human leukocytes, hydrogen peroxide damages host cells and has bactericidal effects on competing bacteria, such as Staphylococcus aureus.
7. Exoenzymes
- Neuraminidase and IgA Protease: These enzymes assist in bacterial pathogenicity by modifying host cell surfaces and evading immune responses, similar to other pathogens.
How is streptococcus pneumoniae able to avoid destruction by a phagocyte?
Streptococcus pneumoniae (S. pneumoniae) employs several strategies to avoid destruction by phagocytes:
- Capsule formation: S. pneumoniae produces a polysaccharide capsule, which inhibits phagocytosis by preventing the activation of complement proteins and blocking the binding of phagocytic receptors.
- Immunoglobulin A1 (IgA1) protease production: S. pneumoniae secretes an enzyme that cleaves IgA1, an antibody that plays a key role in mucosal immunity, reducing its effectiveness.
- Complement evasion: S. pneumoniae can bind to complement regulatory proteins, such as factor H, to prevent the activation of the complement cascade.
- Phagocyte membrane disruption: S. pneumoniae can insert its cholesterol-binding toxin, pneumolysin, into the phagocyte membrane, causing membrane disruption and inhibiting phagocytosis.
- Inhibition of phagolysosome fusion: S. pneumoniae can prevent the fusion of phagosomes with lysosomes, which contain digestive enzymes, thereby avoiding degradation.
- Modulation of cytokine responses: S. pneumoniae can influence cytokine production, suppressing the activation of immune cells and reducing the effectiveness of the immune response.
These mechanisms enable S. pneumoniae to evade phagocytic destruction and establish infection.
Vaccines for Streptococcus pneumoniae
Pneumococcal vaccines are essential tools in preventing infections caused by Streptococcus pneumoniae. Due to the complexity of pneumococcal capsular diversity, vaccines have evolved to address the most common and virulent strains.
1. Polysaccharide Vaccines
- Formulation: Early pneumococcal vaccines were based on polysaccharides from various serotypes. Initially, the vaccines included four serotypes in 1945, expanded to fourteen by the 1970s, and now cover 23 serotypes.
- Current Vaccine: The 23-valent polysaccharide vaccine includes serotypes such as 1, 2, 3, 4, 5, 6B, 7F, 8, 9N, 9V, 10A, 11A, 12F, 14, 15B, 17F, 18C, 19A, 19F, 20, 22F, 23F, and 33F.
- Coverage: These serotypes account for 85 to 90 percent of invasive pneumococcal infections.
- Efficacy: The vaccine’s estimated efficiency is about 60%. Despite its benefits, underuse has allowed pneumococcus to remain a major cause of hospitalization.
- Limitations:
- Immunogenicity: Polysaccharide vaccines are not effective in children under 2 years of age.
- Age Recommendations: Adults aged 65 and older are advised to receive a single dose for lifetime protection.
2. Conjugate Vaccines
- Introduction: Since 2000, pneumococcal conjugate vaccines (PCVs) have been available, improving protection for younger populations.
- PCV7: This heptavalent vaccine targets seven serotypes and is recommended for children aged 2 to 23 months, and for at-risk children aged 24 to 59 months.
- Schedule: The vaccine is administered in a four-dose series at 2, 4, 6, and 12-14 months of age.
- Protection:
- Effectiveness: The PCV7 provides adequate protection against invasive pneumococcal infections, including septicemia and meningitis.
- Limitations: The vaccine does not cover all serotypes, which may leave some children vulnerable if exposed to non-included serotypes.
- Ongoing Research:
- Broad-Spectrum Vaccines: To address the limitations of current vaccines, research continues into developing vaccines that offer protection against a wider range of pneumococcal serotypes.
How to treat streptococcus pneumoniae?
The management of infections caused by Streptococcus pneumoniae primarily involves antibiotic therapy and supportive care. Treatment strategies may vary based on the severity of the infection and the patient’s risk profile.
1. Antibiotic Therapy
- Initial Treatment:
- Timing: Antibiotics should be administered as soon as possible after the diagnosis. Immediate treatment helps to improve patient outcomes. According to the American College of Emergency Physicians (ACEP), there is insufficient evidence to suggest a specific time frame for initiating antibiotics that impacts mortality or morbidity significantly. Therefore, treatment should begin promptly once the diagnosis is confirmed.
- Outpatient Therapy:
- Low-Risk CAP: Patients with low-risk community-acquired pneumonia (CAP) are often treated on an outpatient basis with macrolide monotherapy.
- High-Risk CAP: For outpatient individuals at higher risk, respiratory fluoroquinolones are used.
- Inpatient Therapy:
- Non-ICU Patients: Those admitted to non-ICU settings receive dual therapy consisting of a β-lactam plus a macrolide, or alternatively, respiratory fluoroquinolone monotherapy.
- ICU Patients: Patients requiring ICU care are treated with a more intensive regimen, either a β-lactam plus a macrolide, or a β-lactam plus a respiratory fluoroquinolone.
- Antibiotic Resistance:
- Resistance: Certain strains of S. pneumoniae may exhibit resistance to specific antibiotics. Thus, susceptibility testing is critical for tailoring effective treatment. Resistance patterns can impact the choice of antibiotic and necessitate adjustments based on the strain’s profile.
2. Supportive Care
- Mechanical Ventilation:
- Severe Cases: In cases of severe pneumococcal infections, mechanical ventilation may be required to support respiratory function.
- Complicated Pneumonia:
- Parapneumonic Effusion: For complicated pneumonia with parapneumonic effusion, tube thoracostomy may be necessary to drain fluid. If the condition progresses to empyema and is resistant to drainage, video-assisted thoracoscopic surgery (VATS) with decortication may be performed.
3. Vaccination and Prevention
- Vaccines:
- Types: Two pneumococcal vaccines are available, both designed to stimulate an immune response by delivering antigens that elicit protective immunity.
- Effectiveness: Vaccination is considered preferable to treatment due to its preventive nature. However, some vaccine strains may not cover all circulating serotypes, leading to a need for continuous evaluation of vaccine efficacy and the development of new vaccines targeting conserved antigens.
- Ongoing Research:
- New Vaccines: Research is focused on developing vaccines that include more conserved antigens, improving their ability to protect against a broader range of S. pneumoniae strains.
Diagnosis of Streptococcus pneumoniae Infection
- By cultivating bacterial samples on blood agar, S. pneumoniae is readily identifiable. Due of their hemolytic properties, bacteria will transform blood agar into a dark green colour. However, cultivating bacterial cultures can be time-consuming. Consequently, it is more effective to diagnose an illness utilising contemporary methods.
- The first method involves the identification of antigens in urine, which are components of the streptococcus bacterium. Several body fluids include C polysaccharide, a major component of the bacterial cell wall. Immunochromatography is used to detect the presence of certain antigens and is preferable to bacterial culture for diagnosis.
- The second method utilises a test that detects S. pneumoniae-specific DNA sequences. The tremendous specificity afforded by using DNA as a target decreases the possibility of error, and these procedures can be executed incredibly rapidly.
Laboratory diagnosis of streptococcus pneumoniae
The laboratory diagnosis of Streptococcus pneumoniae infection involves several key methods to identify the presence of this pathogen. The process includes sample collection, transport, and culture techniques, as well as specific tests to confirm the diagnosis.
1. Sample Collection
- Respiratory Tract Specimens:
- Sputum: Collect greater than 1.0 ml of expectorated sputum in a sterile screw-capped container.
- Lung Aspirate/Pleural Fluid: Obtain more than 1.0 ml by percutaneous needle aspiration into a sterile screw-capped tube.
- Body Fluids:
- Blood: After disinfecting the venipuncture site with 70% alcohol and iodine, collect blood aseptically in a culture broth containing an anticoagulant. For adults, 5-10 ml of blood is collected, while for children under 12 years, 1.5-2.0 ml is sufficient. Mix the blood with the broth gently to prevent clotting.
- Cerebrospinal Fluid (CSF): Clean the skin over the L3-L4 interspace with 70% alcohol and iodine, and collect over 1.0 ml of CSF in a sterile screw-capped tube. If not processed immediately, store the CSF in an incubator at 35-37°C.
- Exudates:
- Joints/Middle Ear: Collect more than 1.0 ml by aspiration into a sterile screw-capped tube or add directly to a culture broth.
Note: Avoid refrigerating the sample, as S. pneumoniae is a fastidious organism.
2. Sample Transport
- Transport Guidelines:
- Timeliness: Transport specimens promptly, within 1-2 hours of collection, to maintain sample integrity.
- Temperature: Blood samples can be held at room temperature (20°C–25°C) for 4-6 hours before incubation. Protect samples from extreme temperatures (less than 18°C or more than 30°C) and direct sunlight.
3. Culture and Identification
- Gram Staining:
- Procedure: Perform Gram staining of sputum or CSF samples.
- Observation: Look for Gram-positive, lanceolate-shaped diplococci.
- Culture Techniques:
- Media: Inoculate the sample onto blood agar and chocolate agar plates.
- Incubation: Incubate at 37°C with 5-10% CO2 for 24-48 hours.
- Colony Morphology:
- Blood Agar Plate:
- Appearance: Colonies are small (0.5 mm), round, translucent, or mucoid with alpha-hemolysis, which is a green discoloration of the agar around the colonies.
- Draughtsman Colony: Young alpha-hemolytic colonies appear raised. After 24-48 hours, they flatten with a depressed center, known as a draughtsman colony, due to partial autolysis. This characteristic is indicative of S. pneumoniae.
- Distinction: Alpha-hemolytic colonies from Streptococcus viridans do not produce draughtsman colonies and are thus differentiated from S. pneumoniae.
- Blood Agar Plate:
Biochemical Tests for S. pneumoniae
Biochemical tests are crucial for the accurate identification of Streptococcus pneumoniae. These tests include the catalase test, optochin sensitivity test, bile solubility test, and others. Each test provides specific information to confirm the presence of this pathogen.
1. Catalase Test
- Procedure: Emulsify colonies in hydrogen peroxide solution.
- Result: S. pneumoniae is catalase-negative. No gas bubbles are observed, indicating a negative result.
2. Optochin Sensitivity Test
- Procedure:
- Inoculate a blood agar plate with suspected alpha-hemolytic isolates.
- Place a commercially available optochin disc (6 mm, 5 µg) on the streaked plate.
- Incubate at 37°C with 5-10% CO2 for 18-24 hours.
- Result Interpretation:
- Susceptible: A zone of inhibition greater than 14 mm around the disc indicates sensitivity to optochin, confirming S. pneumoniae.
- Intermediate: A zone size between 9-13 mm requires further testing for bile solubility.
3. Bile Solubility Test
- Procedure:
- Prepare a 1.0 ml saline suspension of the organism, adjusting to the 0.5 McFarland standard.
- Inoculate 0.5 ml of the suspension into two tubes.
- Add 0.5 ml of 2% sodium deoxycholate to the test tube and 0.5 ml of saline to the control tube.
- Incubate at 37°C for 2 hours.
- Result Interpretation:
- Positive Reaction: Clearing or reduction in turbidity in the test tube indicates that the bile salts lyse the colonies of S. pneumoniae.
- Negative Reaction: Persistence of turbidity in the test tube signifies a negative result.
Note: The bile solubility test can also be performed directly on the colony by adding the bile solution.
4. Oxidase Test
- Procedure: Assess the presence of cytochrome c oxidase in the organism.
- Result: S. pneumoniae is oxidase-negative, meaning no color change is observed.
5. Hemolysis
- Procedure: Observe the type of hemolysis on blood agar.
- Result: S. pneumoniae exhibits alpha-hemolysis, characterized by partial hemolysis and a green discoloration around colonies.
6. Oxidative/Fermentative (O/F) Test
- Procedure: Determine the metabolic process used by the bacteria.
- Result: S. pneumoniae is fermentative, utilizing fermentation pathways for energy.
7. Motility
- Procedure: Assess the organism’s movement in semi-solid media.
- Result: S. pneumoniae is non-motile.
Test | Procedure | Result |
---|---|---|
Catalase Test | Emulsify colonies in hydrogen peroxide solution. | S. pneumoniae is catalase-negative. No gas bubbles are observed. |
Optochin Sensitivity Test | – Inoculate a blood agar plate with suspected alpha-hemolytic isolates.<br>- Place optochin disc (6 mm, 5 µg) on the plate.<br>- Incubate at 37°C with 5-10% CO2 for 18-24 hours. | – Susceptible: Zone of inhibition > 14 mm indicates S. pneumoniae.<br>- Intermediate: Zone size between 9-13 mm requires bile solubility testing. |
Bile Solubility Test | – Prepare a 1.0 ml saline suspension of the organism.<br>- Inoculate 0.5 ml into two tubes.<br>- Add 0.5 ml of 2% sodium deoxycholate to the test tube and 0.5 ml saline to the control.<br>- Incubate at 37°C for 2 hours. | – Positive Reaction: Clearing or reduction in turbidity in the test tube indicates lysis by bile.<br>- Negative Reaction: Persistence of turbidity in the test tube. |
Oxidase Test | Assess the presence of cytochrome c oxidase. | S. pneumoniae is oxidase-negative. No color change is observed. |
Hemolysis | Observe type of hemolysis on blood agar. | S. pneumoniae exhibits alpha-hemolysis, with green discoloration around colonies. |
Oxidative/Fermentative (O/F) Test | Determine the metabolic process used by the bacteria. | S. pneumoniae is fermentative. |
Motility | Assess movement in semi-solid media. | S. pneumoniae is non-motile. |
streptococcus pneumoniae under microscope
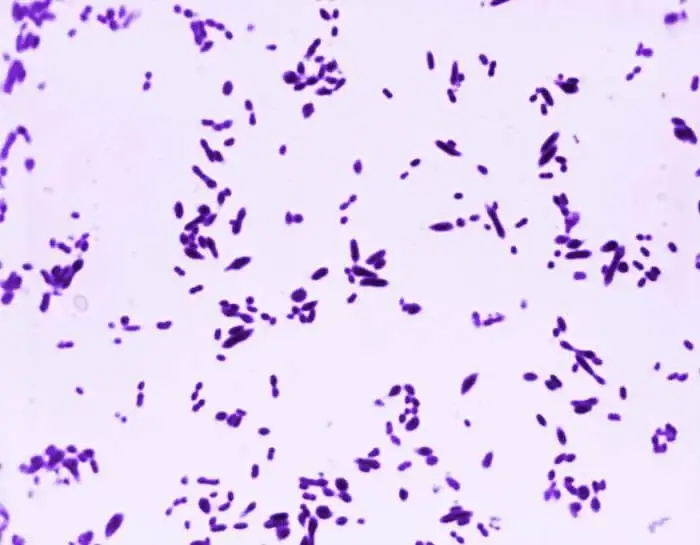
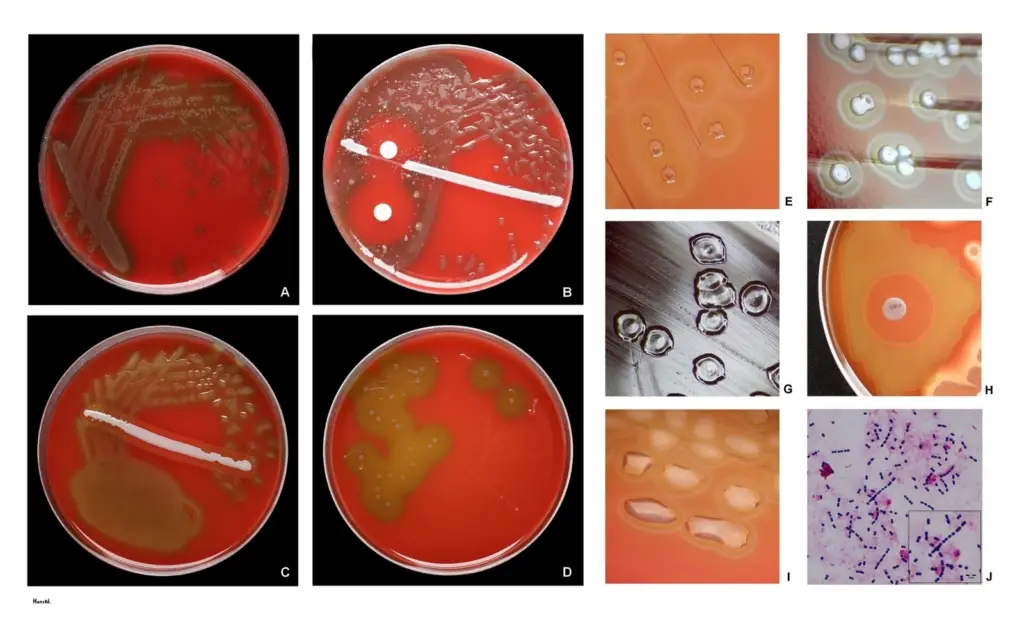
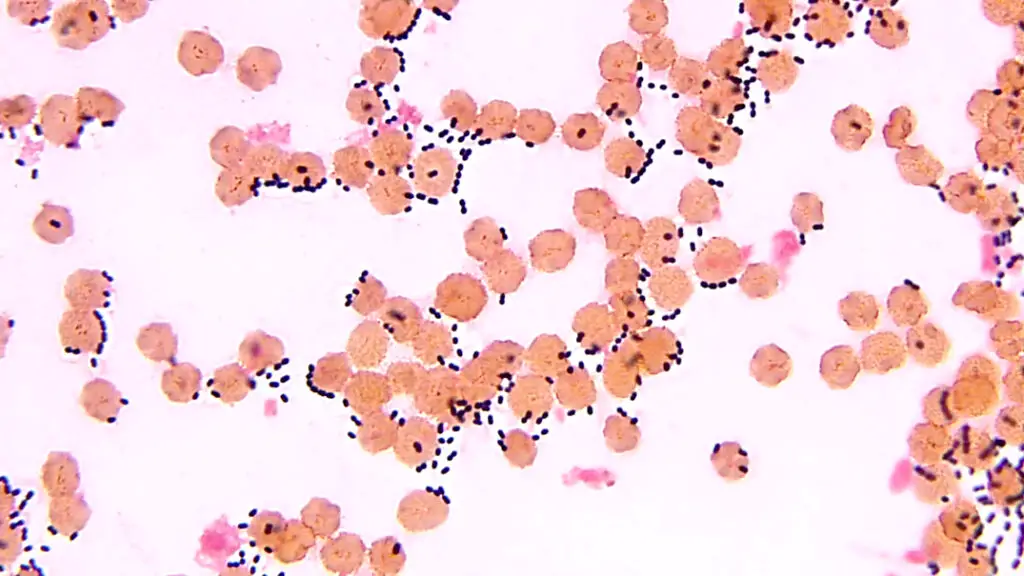
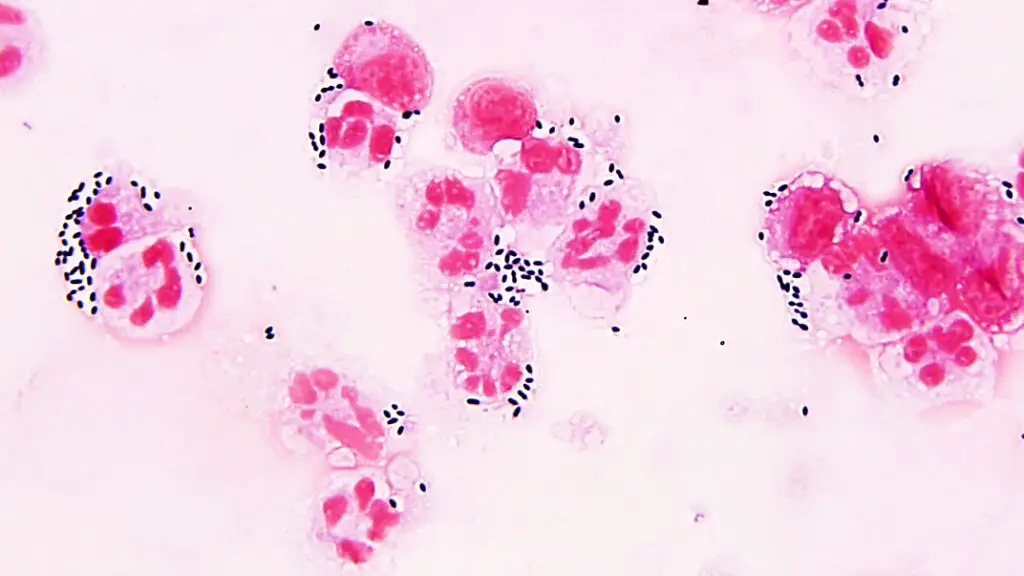
FAQ
What is an invasive Streptococcus pneumoniae infection?
Streptococcus pneumoniae is a common bacterium found in the nose and throat of both children and adults. S. pneumoniae can infect the lungs (pneumonia) or the middle ear (otitis media), but it is called “invasive” when it is detected in the blood, spinal fluid (e.g., meningitis), or another place where germs are ordinarily absent.
Who gets S. pneumoniae infections?
Numerous individuals harbour S. pneumoniae without becoming unwell. Children who are not vaccinated, the elderly, and those with compromised immune systems are at the greatest risk for invasive infection; nonetheless, some persons acquire an invasive infection for no obvious cause.
How is S. pneumoniae spread?
The bacteria are passed from person to person by the breathing in droplets produced by an infected person while coughing or sneezing.
What are the symptoms of S. pneumoniae infection?
Signs and symptoms depend on the part of the body affected. Invasive infection often includes fever, chills, and irritability. Headache, stiff neck, confusion, sleepiness, vomiting, and poor feeding can occur with meningitis.
How soon after exposure do symptoms appear?
The incubation period varies. Signs and symptoms of infection can occur within 1-3 days after exposure, but may occur long after exposure.
How is S. pneumoniae diagnosed?
Laboratory testing on blood, pleural fluid, joint fluid, or cerebrospinal fluid (CSF) are needed to confirm the diagnosis of invasive illness.
What is the treatment for S. pneumoniae infection?
The main treatment for invasive S. pneumoniae infection is antibiotics.
What can be done to prevent the spread of S. pneumoniae?
Vaccines can prevent S. pneumoniae infections from becoming invasive. The 13-valent pneumococcal conjugate vaccine (PCV-13) is recommended for all children between the ages of two months and 59 months. The 23-valent pneumococcal polysaccharide vaccine (PPSV-23) is administered to high-risk individuals at least two years old. Both PCV-13 and PPSV-23 are indicated for adults; their use is determined by lifestyle, age, and health status. Consult your physician or local health department to determine if you should receive the vaccine.
Frequent handwashing with soap and water (or, in the absence of soap and water, with alcohol-based hand sanitizers or gels) can help prevent the transmission of numerous viruses and germs. Not sharing food, beverages, or eating utensils with others can also aid in preventing the transmission of infections.
Exposure to a person with a S. pneumoniae infection does not typically necessitate preventative treatment (e.g., antibiotics).
When and for how long is a person able to spread S. pneumoniae?
The infectious period is variable and might extend as long as the pathogen is present in the nose and throat. After taking the appropriate medicines for 1-2 days, a person can no longer transmit S. pneumoniae.
Does past infection with S. pneumoniae make a person immune?
No. Individuals who have previously contracted S. pneumoniae can contract it again.
References
- Ramirez, M., Carriço, J. A., van der Linden, M., & Melo-Cristino, J. (2015). Molecular Epidemiology of Streptococcus pneumoniae. Streptococcus Pneumoniae, 3–19. doi:10.1016/b978-0-12-410530-0.00001-6
- Ramirez, M. (2015). Streptococcus pneumoniae. Molecular Medical Microbiology, 1529–1546. doi:10.1016/b978-0-12-397169-2.00086-x
- Janoff, E. N., & Musher, D. M. (2015). Streptococcus pneumoniae. Mandell, Douglas, and Bennett’s Principles and Practice of Infectious Diseases, 2310–2327.e5. doi:10.1016/b978-1-4557-4801-3.00201-0
- Sá-Leão, R., & Tomasz, A. (2009). Streptococcus Pneumoniae. Encyclopedia of Microbiology, 304–317. doi:10.1016/b978-012373944-5.00207-8
- Weiser, J.N., Ferreira, D.M. & Paton, J.C. Streptococcus pneumoniae: transmission, colonization and invasion. Nat Rev Microbiol 16, 355–367 (2018). https://doi.org/10.1038/s41579-018-0001-8
- Dion CF, Ashurst JV. Streptococcus Pneumoniae. [Updated 2022 Aug 8]. In: StatPearls [Internet]. Treasure Island (FL): StatPearls Publishing; 2022 Jan-. Available from: https://www.ncbi.nlm.nih.gov/books/NBK470537/
- Ramirez, M., Carriço, J. A., van der Linden, M., & Melo-Cristino, J. (2015). Molecular Epidemiology of Streptococcus pneumoniae. Streptococcus Pneumoniae, 3–19. doi:10.1016/b978-0-12-410530-0.00001-6
- https://www.frontiersin.org/articles/10.3389/fimmu.2018.01366/full
- https://www.who.int/teams/health-product-policy-and-standards/standards-and-specifications/vaccine-standardization/pneumococcal-disease
- https://www.vdh.virginia.gov/epidemiology/epidemiology-fact-sheets/streptococcus-pneumoniae-invasive-infection/
- https://www.uptodate.com/contents/invasive-pneumococcal-streptococcus-pneumoniae-infections-and-bacteremia
- https://www.dhs.wisconsin.gov/immunization/pneumo.htm
- https://www.dshs.texas.gov/vaccine-preventable-diseases/streptococcus-pneumoniae-infection-pneumococcal-disease
- https://www.creative-diagnostics.com/tag-streptococcus-pneumoniae-antigens-73.htm
- https://www.nhsinform.scot/illnesses-and-conditions/infections-and-poisoning/pneumococcal-infections
- https://pubmlst.org/organisms/streptococcus-pneumoniae
- https://hhs.iowa.gov/cade/disease-information/streptococcus-pneumoniae
- https://www.cdc.gov/pneumococcal/clinicians/streptococcus-pneumoniae.html#:~:text=Streptococcus%20pneumoniae%20are%20lancet%2Dshaped,the%20majority%20of%20pneumococcal%20infections.
- https://microbenotes.com/habitat-and-morphology-of-streptococcus-pneumoniae/
- https://www.ncbi.nlm.nih.gov/books/NBK470537/
- https://www.news-medical.net/health/Streptococcus-pneumoniae-(pneumococcus)-Overview.aspx
- https://emedicine.medscape.com/article/225811-overview