What is Smooth Muscle?
- Smooth muscle, a distinct type of muscle tissue, plays a pivotal role in the function of various organ systems by exerting pressure on vessels and organs. Characterized by its unique cellular structure, smooth muscle is composed of sheets or strands of specialized cells.
- Within these cells, actin and myosin fibers are present, supported by an intricate framework of auxiliary proteins. The contraction of smooth muscle is a regulated process, initiated when adenosine triphosphate (ATP) is liberated for myosin utilization. The release of ATP is contingent upon the intensity of the stimuli, which enables smooth muscle to exhibit graded contractions, a stark contrast to the binary contraction mechanism observed in skeletal muscle.
- Morphologically, smooth muscle is an involuntary non-striated muscle. The absence of sarcomeres in its structure results in a lack of striations, distinguishing it from other muscle types. Smooth muscle can be further categorized into two subtypes: single-unit and multiunit. In the single-unit subtype, an entire bundle or sheet of smooth muscle cells contracts in a coordinated manner, functioning as a syncytium.
- Anatomically, smooth muscle is predominantly located in the walls of hollow organs, such as the stomach, intestines, bladder, and uterus. In the circulatory system, it forms the vascular smooth muscle, present in the walls of blood and lymph vessels, with the exception of capillaries. The respiratory, urinary, and reproductive systems also contain tracts lined with smooth muscle.
- Ocular structures, specifically the ciliary muscles and the muscles of the iris (iris dilator and sphincter muscles), are composed of smooth muscle. These ocular muscles play crucial roles in visual accommodation and pupil modulation. Additionally, in the integumentary system, the arrector pili smooth muscle cells are responsible for the erection of hair in response to stimuli such as cold or emotional states.
- Among the three primary muscle tissues in the human body—skeletal, cardiac, and smooth—smooth muscle is unique. It is non-striated, meaning it lacks the visible bands of contractile proteins observed in skeletal muscles. Its involuntary nature signifies that its contractions are not governed by conscious intent but are instead modulated by chemical or electrical signals.
- These signals are transmitted via autonomic nerves and specific hormones, including epinephrine and vasopressin. Structurally, smooth muscle tissues often form circular layers around organs like the gut or are arranged as sheets interspersed with connective tissue. A notable cellular characteristic of smooth muscle is the presence of a singular nucleus per cell.
- In conclusion, smooth muscle, with its specialized structure and function, is integral to the physiological processes of various organ systems. Its unique characteristics, ranging from its non-striated appearance to its involuntary contractions, underscore its importance in maintaining homeostasis within the human body.
Definition of Smooth Muscle
Smooth muscle is an involuntary, non-striated muscle tissue found primarily in the walls of hollow organs and blood vessels, responsible for functions such as regulating blood flow and propelling substances through organs.
Characteristics of smooth muscle
Smooth muscle tissue, distinct from its skeletal and cardiac counterparts, exhibits a set of unique characteristics that enable it to perform specialized functions in various organ systems. Here, we delve into the defining features of smooth muscle tissue:
- Cellular Morphology: Smooth muscle cells are fusiform or spindle-shaped, characterized by a broad central region that tapers towards both ends. This unique shape facilitates the muscle’s ability to contract and relax efficiently, especially in organs like the stomach.
- Filamentous Structure: Under microscopic examination, actin filaments can be observed traversing the smooth muscle cell. These filaments, depicted in red in certain microscopic images, connect dense bodies within the cell, providing structural integrity during contraction.
- Nuclear Presence: Each smooth muscle cell contains a singular, centrally located nucleus. This nucleus orchestrates various cellular activities, ensuring the muscle’s proper functioning.
- Elastic Properties: One of the hallmark features of smooth muscle is its inherent elasticity. After being stretched, these muscles can revert to their original size, a property crucial for organs that undergo periodic expansion and contraction, such as the bladder.
- Contractility: Smooth muscle tissue operates involuntarily, contrasting with the voluntary control exhibited by skeletal muscles. This involuntary nature ensures the continuous functioning of vital organ systems without conscious effort.
- Absence of Sarcomeres: Unlike skeletal and cardiac muscles, which possess repeating units called sarcomeres, smooth muscle lacks these structures. Sarcomeres, comprising alternating dark and light bands, contribute to the striated appearance of skeletal and cardiac muscles. The absence of sarcomeres in smooth muscle results in its non-striated appearance.
- Unique Protein Complexes: Smooth muscle does not contain the troponin-tropomyosin complex typical of other muscle types. Instead, it possesses calmodulin, a protein that binds calcium ions. The resultant Ca^2+-calmodulin complex activates myosin kinase, an enzyme essential for muscle contraction.
- Muscular System Context: Smooth muscle is one of the three primary muscle types in the animal body, the others being skeletal and cardiac muscles. While skeletal and cardiac muscles exhibit striations due to the organized arrangement of myosin filaments, smooth muscle’s uniform myosin distribution renders it non-striated. Furthermore, smooth muscle cells have a higher actin-to-myosin ratio compared to skeletal muscle cells, allowing them to contract to a much smaller fraction of their resting length. Their contractions, though slower than skeletal muscles, can be sustained over extended periods, making them indispensable for the rhythmic, involuntary movements of various organs.
In summary, smooth muscle tissue, with its distinct characteristics, plays a pivotal role in the involuntary functioning of numerous organ systems. Its unique morphology and protein composition enable it to execute rhythmic contractions, ensuring the seamless operation of vital physiological processes.
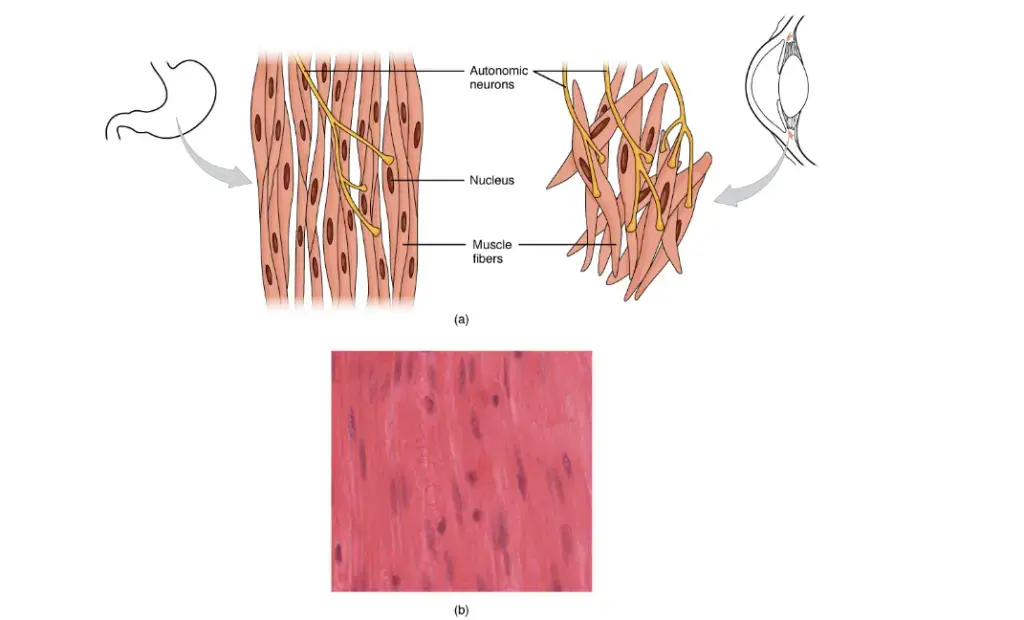
Organ system involved in smooth muscles
Smooth muscles, characterized by their involuntary and non-striated nature, are integral components of various organ systems within the body. Their presence and function in these systems underscore their significance in maintaining physiological homeostasis. Here, we elucidate the organ systems that incorporate smooth muscles and their respective roles:
- Integumentary System: Within the skin, smooth muscles known as erector pili are associated with hair follicles. Their contraction in response to external stimuli, such as cold temperatures, results in the elevation of hair, a phenomenon commonly referred to as “goosebumps.” This action aids in thermoregulation by reducing heat loss.
- Urinary System: The urinary system, particularly the urinary bladder, houses layers of smooth muscle. These muscles play a pivotal role in the storage and expulsion of urine, facilitating the body’s waste elimination process.
- Reproductive System: Smooth muscles are integral to both male and female reproductive tracts. In males, they are present in the vas deferens, aiding in sperm transport and the ejection of glandular secretions. In females, the uterus’s myometrium predominantly consists of smooth muscle, which contracts during labor, facilitating the birthing process.
- Gastrointestinal Tract (GIT): The gastrointestinal tract, encompassing organs like the stomach and intestines, contains extensive layers of smooth muscle. These muscles facilitate the movement of ingested food through the digestive system via coordinated contractions called peristalsis. Additionally, they play a role in the mixing and mechanical breakdown of food, aiding in digestion.
- Sensory System: Within the eye, smooth muscles are present in the iris and the ciliary body. The iris’s smooth muscles control the size of the pupil, regulating the amount of light entering the eye. The ciliary muscle, on the other hand, adjusts the eye’s lens shape, enabling focus on objects at varying distances.
- Cardiovascular System (CVS): Smooth muscles are integral to the cardiovascular system, particularly in the walls of blood vessels and lymphatic vessels. Vascular smooth muscles in the tunica media layer of arteries and veins regulate vessel diameter through contraction (vasoconstriction) and relaxation (vasodilation). This modulation directly influences blood flow, pressure, and distribution to various body tissues.
In summary, smooth muscles are intricately woven into multiple organ systems, playing diverse and essential roles. Their ability to contract and relax in response to various stimuli ensures the efficient and coordinated functioning of these systems, contributing to overall physiological well-being.
Smooth Muscle Structure
Gross anatomy
- Smooth muscle tissue, a fundamental component of the musculature system, is classified into two primary categories: single-unit (visceral) smooth muscle and multiunit smooth muscle.
- The predominant type, single-unit smooth muscle, is extensively distributed in the walls of most visceral organs, lining blood vessels (excluding large elastic arteries), the urinary system, and the digestive tract. Notably, the heart is devoid of this muscle type, being exclusively composed of cardiac muscle.
- In the single-unit variant, individual cells within a bundle receive innervation from an autonomic nerve fiber, exhibiting myogenic properties. The presence of numerous gap junctions between these cells facilitates the propagation of action potentials across adjacent muscle cells.
- This interconnectedness results in the formation of a syncytium, allowing the entire muscle to contract or relax in a coordinated manner, exemplified by uterine contractions during childbirth.
- The intrinsic nature of single-unit visceral smooth muscle is myogenic, enabling it to undergo regular contractions without necessitating motor neuron input. This contrasts with multiunit smooth muscle, which is neurogenic, requiring initiation by an autonomic nervous system neuron for contraction.
- Within a single-unit muscle, certain cells may function as pacemakers, producing rhythmic action potentials due to their inherent electrical activity. Given its myogenic attributes, this muscle type remains active even in the absence of neural stimulation. Multiunit smooth muscle is localized in regions such as the trachea, the iris, and the linings of large elastic arteries.
- However, the dichotomy of single- and multi-unit smooth muscle may be an oversimplified representation. In reality, smooth muscle activity is typically modulated by a myriad of neural elements. Furthermore, intercellular communication and the local production of activators or inhibitors often result in a coordinated response, even in multiunit smooth muscle.
- Distinct from skeletal and cardiac muscles, smooth muscle showcases differences in structure, functional regulation, contraction modulation, and excitation-contraction coupling.
- A salient feature of smooth muscle tissue is its enhanced elasticity and the ability to function across an expansive length-tension curve compared to striated muscle. This adaptability, crucial for organs like the intestines and bladder, allows them to stretch while retaining contractility.
- In the gastrointestinal tract, smooth muscle activation is orchestrated by a triad of cell types: smooth muscle cells (SMCs), interstitial cells of Cajal (ICCs), and platelet-derived growth factor receptor alpha (PDGFRα) cells. These cells are electrically interconnected, collaboratively functioning as an SIP functional syncytium.
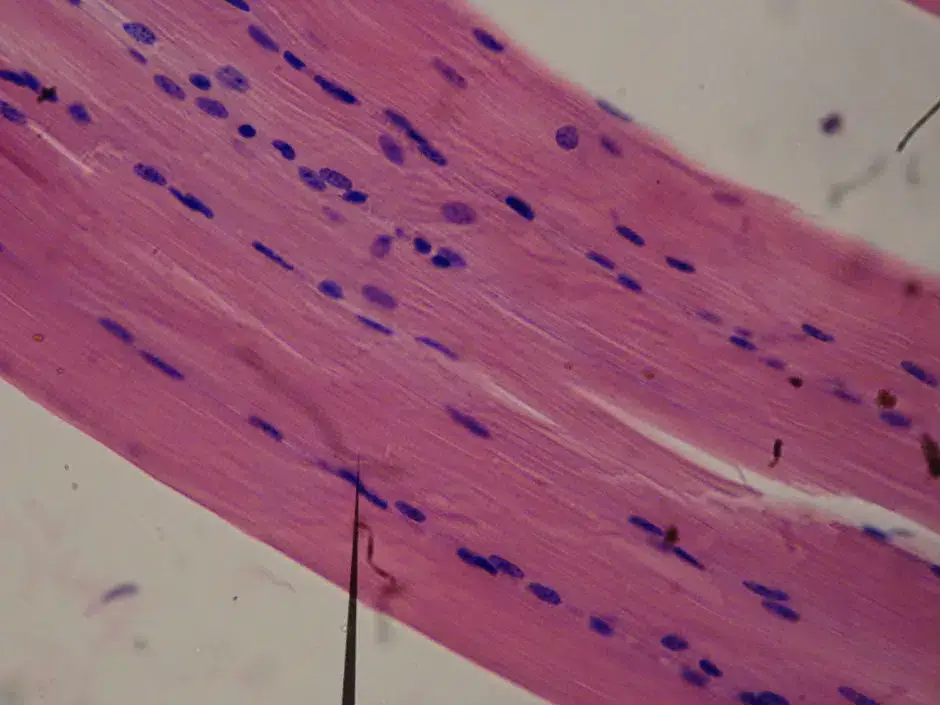
- Classification
- Single-unit (Visceral) Smooth Muscle
- Predominantly found in walls of visceral organs, blood vessels (excluding large elastic arteries), urinary, and digestive systems.
- Excludes the heart, which contains cardiac muscle.
- Myogenic in nature: can contract without motor neuron input.
- Contains numerous gap junctions, allowing coordinated contractions.
- Some cells function as pacemakers, producing rhythmic action potentials.
- Multiunit Smooth Muscle
- Located in the trachea, iris, and linings of large elastic arteries.
- Requires initiation by an autonomic nervous system neuron (neurogenic).
- Single-unit (Visceral) Smooth Muscle
- Functional Characteristics
- Single-unit muscle remains active even without neural stimulation.
- The distinction between single- and multi-unit is somewhat oversimplified.
- Most smooth muscles are influenced by various neural elements.
- Intercellular communication often results in coordinated responses.
- Comparative Features
- Differs from skeletal and cardiac muscles in structure, function, and regulation.
- Demonstrates greater elasticity and operates within a broader length-tension curve than striated muscle.
- Activation in the Gastrointestinal Tract
- Orchestrated by a combination of:
- Smooth muscle cells (SMCs)
- Interstitial cells of Cajal (ICCs)
- Platelet-derived growth factor receptor alpha (PDGFRα) cells.
- These cells work in tandem, forming an SIP functional syncytium.
- Orchestrated by a combination of:
Microanatomy of Smooth Muscle
Smooth muscle cells, distinct from their striated counterparts, are spindle-shaped myocytes characterized by a broad center that tapers at both ends, housing a singular nucleus. These cells, ranging from 30–200 micrometers in length, are significantly shorter than skeletal muscle cells. Despite the absence of myofibrils, a substantial portion of their cytoplasm is occupied by the proteins myosin and actin, which confer the ability to contract.
- Myosin in Smooth Muscle: Myosin, predominantly of class II in smooth muscle, comprises two heavy chains (MHC) that form the head and tail domains. Each heavy chain possesses an N-terminal head domain, while the C-terminal tails adopt a coiled-coil structure, intertwining the two heavy chains. Consequently, myosin II exhibits two heads. The gene MYH11 encodes the heavy chains of myosin II in smooth muscle, but splice variants of this gene yield four distinct isoforms. Additionally, some MHC in smooth muscle may not contribute to contraction. Myosin II also incorporates four light chains, with two allocated per head. These light chains, weighing 20 (MLC20) and 17 (MLC17) kDa, bind to the heavy chains in the “neck” region. The MLC20, or regulatory light chain, is instrumental in muscle contraction, while the exact role of MLC17 remains elusive.
- Actin Dynamics: The contractile machinery’s thin filaments are primarily composed of alpha-actin and gamma-actin. Smooth muscle alpha-actin is the dominant isoform, while a significant amount of actin, mainly beta-actin, polymerizes beneath the plasma membrane upon contractile stimulation, possibly aiding in mechanical tension. The actin to myosin ratio in smooth muscle varies between 2:1 and 10:1, contrasting with striated skeletal muscle where myosin is more dominant.
- Associated Proteins: Smooth muscle lacks troponin but expresses significant amounts of calmodulin, caldesmon, and calponin. Tropomyosin, present in smooth muscle, spans seven actin monomers, covering the entire length of the thin filaments. While its function in striated muscle is well-understood, its role in smooth muscle remains ambiguous. Both calponin and caldesmon have proposed roles in tension maintenance and actin-myosin-tropomyosin tethering, respectively. All three proteins might inhibit the ATPase activity of the myosin complex, which otherwise powers muscle contraction.
- Dense Bodies and Intermediate Filaments: Dense bodies, analogous to Z-discs in striated muscle, anchor actin filaments. Rich in alpha-actinin, they also connect intermediate filaments, primarily composed of vimentin and desmin. These structures seem to serve as anchoring points for thin filaments to exert force. The intermediate filaments further connect to adherens junctions on the smooth muscle cell’s sarcolemma, facilitating force transduction.
- Contraction Mechanism: During contraction, the contractile machinery undergoes spatial reorganization to optimize force generation. This involves phosphorylation of vimentin and potential changes in the number and length of myosin filaments. Single smooth muscle cells have been observed to contract in a spiral or corkscrew manner.
- Elasticity and Extracellular Matrix: Smooth muscle tissues require frequent stretching, emphasizing the importance of elasticity. These cells may produce an intricate extracellular matrix comprising collagen, elastin, glycoproteins, and proteoglycans. The interaction between smooth muscle and these extracellular matrix components contributes to the tissue’s viscoelasticity.
- Caveolae: The sarcolemma of smooth muscle cells features caveolae, lipid raft microdomains specialized for cell signaling and ion channel functions. Positioned close to the sarcoplasmic reticulum or mitochondria, these invaginations house a plethora of receptors, second messenger generators, G proteins, kinases, and ion channels. They are believed to organize signaling molecules within the membrane.
In conclusion, the microanatomy of smooth muscle is a complex interplay of proteins, filaments, and cellular structures that enable its unique contractile and elastic properties. This intricate design ensures the smooth muscle’s ability to function efficiently in various physiological contexts.
Types of Smooth Muscle
Smooth muscle, integral to the function of various organs and systems within the body, can be categorized into two primary types based on their functional and structural characteristics. These are:
- Single-unit (Visceral) Smooth Muscle: This type of smooth muscle is characterized by its synchronized and collective functionality. In single-unit smooth muscle, the cells operate in unison, behaving as a cohesive unit. Often referred to as “unitary,” these muscles exhibit coordinated contractions due to the presence of gap junctions that facilitate the rapid transmission of electrical impulses between adjacent cells. As a result, when one cell is stimulated, the impulse spreads to neighboring cells, ensuring a unified contraction. This type is commonly found in organs such as the intestines, uterus, and bladder.
- Multiunit Smooth Muscle: Contrasting with the single-unit type, multiunit smooth muscles consist of individual muscle fibers that operate independently of one another. In this type, the cells lack the extensive network of gap junctions seen in the single-unit variety, leading to independent and discrete contractions. Each muscle fiber requires its own nerve impulse for activation. Due to this characteristic, multiunit smooth muscles offer finer control and are typically found in locations where precision is paramount, such as the iris of the eye, airways of the lungs, and the walls of blood vessels.
In summary, the classification of smooth muscles into single-unit and multiunit types is based on their functional coordination and structural interconnections. While the single-unit type operates as a cohesive entity, the multiunit type provides more localized and precise contractions. Understanding these distinctions is crucial for comprehending the diverse roles smooth muscles play in various physiological processes.
Single-unit smooth muscle
Single-unit smooth muscles, often referred to as visceral muscles, predominantly constitute the walls of the body’s internal hollow organs. These muscles play a pivotal role in facilitating the movement and passage of substances within various systems, ensuring the smooth functioning of physiological processes.
1. Functionality: Single-unit smooth muscles are adept at producing prolonged and consistent contractions. Such contractions are instrumental in processes like the digestion of food within the gastrointestinal tract (GIT). The rhythmic and coordinated contractions aid in the propulsion of ingested food, ensuring efficient digestion and absorption.
2. Vascular Role: A significant presence of single-unit smooth muscles is observed in blood vessels. These muscles are intricately woven around the vessel structure, enabling them to regulate the vessel’s diameter. By contracting and relaxing, these muscles modulate blood flow and pressure, ensuring optimal distribution of blood to various body parts.
3. Anatomical Distribution: As the term “visceral” suggests, these muscles are predominantly associated with organs situated within the body, especially those housed in the abdominal cavity. This includes organs of the reproductive system, the urinary bladder, and the liver. Furthermore, in the circulatory system, vascular smooth muscles are organized in concentric layers encircling the vascular lumen, providing structural integrity and functional adaptability.
In the ocular system, single-unit smooth muscles play a crucial role in adjusting the iris’s size and altering the lens’s shape, thereby aiding in vision adaptation to varying light conditions. Additionally, these muscles are present in the skin, where they are responsible for piloerection, a phenomenon where hair stands erect in response to cold temperatures or emotional stimuli.
Single-unit smooth muscles, with their widespread distribution across various organ systems, play a fundamental role in maintaining homeostasis and ensuring the efficient functioning of physiological processes. Their ability to produce coordinated contractions makes them indispensable in processes ranging from digestion to blood flow regulation. Understanding their function and distribution is essential for a comprehensive grasp of human physiology.
Multi-unit smooth muscle
Multi-unit smooth muscles represent a distinct category within the realm of smooth muscle tissue. Unlike their single-unit counterparts, which operate in a coordinated and collective manner, multi-unit smooth muscles are characterized by their independent functionality.
1. Functional Independence: The hallmark feature of multi-unit smooth muscles is the autonomy of individual muscle fibers. Each muscle cell or fiber in this category operates independently, devoid of the extensive intercellular connections that typify single-unit smooth muscles. This independence allows for more precise and localized contractions, tailored to the specific needs of the organ or tissue they inhabit.
2. Anatomical Distribution: Multi-unit smooth muscles are strategically located in various parts of the body, serving specialized functions:
- Lungs: Within the respiratory system, these muscles play a pivotal role in modulating airway diameter, thereby regulating airflow and ensuring optimal oxygenation.
- Arrector Pili Muscles: These are tiny muscles associated with hair follicles. In response to certain stimuli, such as cold or emotional triggers, these muscles contract, leading to the erection of hair, a phenomenon commonly referred to as “goosebumps.”
- Large Arteries: In the circulatory system, multi-unit smooth muscles in large arteries contribute to the regulation of blood flow and pressure by adjusting the arterial diameter.
- Internal Eye Muscles: These muscles are integral to vision. They adjust the size of the pupil, controlling the amount of light entering the eye. Additionally, they play a role in reshaping the lens, facilitating focus on objects at varying distances.
Multi-unit smooth muscles, with their unique independent functionality, cater to the specific and nuanced needs of the organs and systems they are part of. Their precise and localized contractions enable a range of vital physiological processes, from vision adaptation to blood flow regulation. A comprehensive understanding of their role and distribution offers valuable insights into the intricate workings of the human body.
Smooth Muscle Location
Smooth muscle, distinguished by its ability to sustain contractions over prolonged periods, is strategically located in various regions of the body, facilitating a myriad of physiological functions. Here, we explore the primary locations of smooth muscle and its associated roles:
- Circulatory System: Smooth muscle is integral to the circulatory system, playing a pivotal role in regulating blood pressure and ensuring the efficient distribution of oxygen. While the heart is the primary force behind blood circulation, every artery and vein is lined with smooth muscle. These muscles can constrict, increasing vascular resistance, or relax, enhancing blood flow. Intriguingly, the presence or absence of oxygen stimulates these muscles, allowing them to adjust vascular dynamics to ensure optimal oxygen delivery.
- Digestive System: The digestive system extensively incorporates smooth muscle. Unlike the circulatory system’s smooth muscles, which respond to oxygen levels, those in the digestive tract react to mechanical stimuli. For instance, the act of swallowing exerts tension on one side of the smooth muscle sheet lining the gut. This tension triggers a contraction, initiating a wave-like motion known as peristalsis. Peristalsis propels ingested food through the intricate pathways of the digestive tract, ensuring efficient digestion and nutrient absorption.
- Integumentary System: Smooth muscle is also present in the integumentary system, specifically in the form of tiny muscles called arrector pili. These muscles attach to hair follicles and contract in response to cold temperatures or emotional stimuli, causing hair to stand erect—a phenomenon colloquially termed “goosebumps.”
- Ophthalmic System: In the eyes, smooth muscles are responsible for adjusting the diameter of the irises, thereby controlling the amount of light entering the eye. This regulation is crucial for vision, especially in varying light conditions.
- Other Locations: Smooth muscle’s unique ability to provide sustained, elastic tension has led to its incorporation in various other bodily functions. It is involved in the contraction of numerous sphincters, regulating the passage of substances through tubular structures. Additionally, it facilitates the movement of fluids within organs by exerting pressure, ensuring the efficient functioning of bodily systems.
In conclusion, smooth muscle, with its specialized contractile properties, is strategically located across various organ systems. While it may not exhibit the rapid contraction and relaxation seen in skeletal or cardiac muscles, its capacity for prolonged, consistent tension makes it indispensable for numerous physiological processes.
Cross-Bridge Formation and Smooth Muscle Contraction
Smooth muscle contraction, distinct from that of skeletal muscle, is orchestrated by a series of intricate molecular events. Unlike skeletal muscles, which rely on the troponin-tropomyosin complex for cross-bridge formation, smooth muscles employ a calcium-modulated protein known as calmodulin. Here’s a detailed breakdown of the process:
- Initiation by Membrane Depolarization: A change in membrane potential triggers the opening of L-type voltage-gated calcium channels. This allows extracellular calcium ions to flood into the cell, moving down their concentration gradient.
- Formation of Calcium-Calmodulin Complex: The intracellular calcium ions bind to calmodulin, resulting in the formation of the calcium-calmodulin complex. This complex subsequently activates an enzyme called myosin light chain kinase (MLC kinase).
- Activation of Myosin Heads: MLC kinase facilitates the phosphorylation of myosin heads by hydrolyzing ATP. Once phosphorylated, these myosin heads can bind to actin-binding sites, initiating the cross-bridge formation.
- Muscle Contraction: The actin filaments, anchored to dense bodies dispersed throughout the sarcoplasm, are pulled upon by the myosin heads. As the thin filaments slide past the thick ones, the dense bodies are drawn together, leading to the contraction of the entire muscle fiber.
- Muscle Relaxation: For the muscle to relax, the myosin heads must be dephosphorylated. This dephosphorylation is mediated by an enzyme called myosin light chain phosphatase.
- Calcium Ion Regulation: The contraction can persist until ATP-dependent calcium pumps actively transport calcium ions back into the sarcoplasmic reticulum and out of the cell. A basal level of calcium remains in the sarcoplasm, ensuring the maintenance of muscle tone and a slight, continuous contraction.
- Latch-Bridges: A specialized subtype of cross-bridge, known as latch-bridges, allows the myosin heads to remain attached to actin. This attachment sustains the linkage between thick filaments even in the absence of ATP and calcium. This mechanism is crucial for maintaining muscle tone, especially evident in the smooth muscle lining of arterioles.

In summary, the contraction and relaxation of smooth muscle are governed by a unique set of molecular interactions, primarily centered around calcium ions and calmodulin. This mechanism ensures the efficient and sustained functioning of smooth muscles in various physiological contexts.
Control of Contraction
Smooth muscle contraction operates autonomously, devoid of conscious intervention, classifying it as involuntary muscle. The initiation and regulation of these contractions are influenced by a myriad of factors, including hormones, autonomic nervous system-mediated neuronal stimulation, and localized conditions.
- Neuronal Stimulation: Unlike the structured neuromuscular junctions in skeletal muscles, the autonomic nervous system’s neurons interact with smooth muscle in a distinct manner. Axonal swellings, termed varicosities, laden with neurotransmitters, are situated in proximity to the sarcolemma, facilitating the transmission of signals.
- Pacemaker Cells: Certain smooth muscles possess inherent rhythmicity due to the presence of pacemaker cells. These cells can autonomously initiate action potentials without external stimuli. A notable example of such cells is the interstitial cells of Cajal, located in the gastrointestinal (GI) tract. These cells orchestrate the rhythmic contractions essential for propelling digestive contents.
- Stress-Relaxation Response: In specific anatomical regions, the act of muscle stretching can autonomously induce its contraction. This phenomenon, termed the stress-relaxation response, involves an initial mechanical stress-induced contraction, promptly followed by relaxation. This mechanism is paramount in hollow organs like the stomach or urinary bladder. As these organs fill and expand, the stress-relaxation response ensures that the surrounding smooth muscle maintains its tone. Consequently, when the organ contracts post-emptying, it avoids premature emptying and retains its structural integrity, preventing it from becoming flaccid.
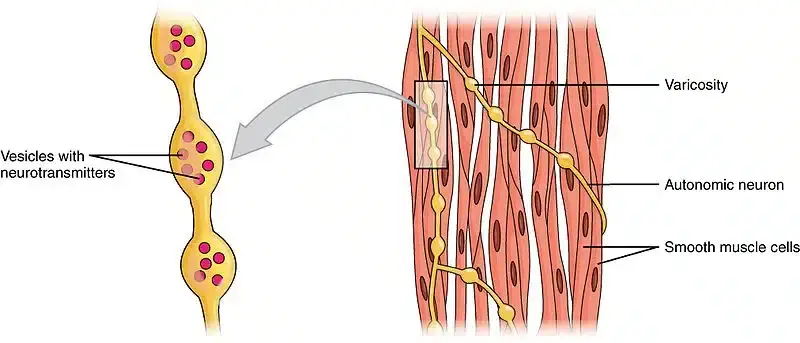
In essence, the control of contraction in smooth muscle is a multifaceted process, influenced by both intrinsic and extrinsic factors. This intricate regulation ensures the efficient functioning of various physiological systems, from digestion to blood circulation.
Mechanism of Smooth Muscle
Smooth muscle contraction is a complex process, intricately regulated by calcium dynamics. Unlike skeletal muscles, smooth muscle contraction is not governed by the troponin-tropomyosin complex. Instead, it relies on the interaction between calcium and a protein called calmodulin.
- Calcium Dynamics: The initiation of smooth muscle contraction is contingent upon an increase in intracellular calcium. This surge is achieved through two primary mechanisms:
- Activation by depolarization, hormones, or neurotransmitters prompts calcium entry via L-type channels in the cell membrane’s caveolae.
- Intracellular calcium stimulates the sarcoplasmic reticulum (SR) to release additional calcium through ryanodine receptors and IP3, a process termed calcium-induced calcium release.
- Calmodulin Activation: The elevated intracellular calcium binds to calmodulin, transforming it into its activated state. This activated calmodulin subsequently activates myosin light chain kinase (MLCK).
- Myosin Activation: MLCK phosphorylates the myosin light chain, enhancing its ATPase activity. This phosphorylation facilitates the binding of myosin to actin, initiating cross-bridge cycling and generating muscle tension.
- Role of Dense Bodies: The thin filaments in smooth muscle are anchored to dense bodies distributed throughout the sarcoplasm. As the thin filaments slide past the thick filaments during contraction, they exert a pull on these dense bodies, leading to a collective contraction of the muscle fiber.
- Relaxation Mechanism: The cessation of smooth muscle contraction is achieved by the dephosphorylation of myosin light chains, a process mediated by myosin light chain phosphatase (MLCP).
- Nitric Oxide in Relaxation: Nitric oxide, synthesized in endothelial cells, diffuses into smooth muscle cells and promotes the conversion of guanosine triphosphate (GTP) to cyclic guanosine monophosphate (cGMP) by activating guanylyl cyclase. Elevated cGMP levels stimulate cGMP-dependent protein kinase, which in turn activates MLCP, leading to muscle relaxation.
- Unique Electrical Properties: Smooth muscle cells exhibit distinct electrical properties. They can generate action potentials that are slower and longer-lasting than those in skeletal muscles. Some smooth muscle cells, like the interstitial cells of Cajal in the intestines, can produce spontaneous pacemaker currents, leading to rhythmic contractions. These oscillations in membrane potential, termed slow waves, are not directly responsible for contraction but can trigger it if they reach a certain amplitude.
- Latch State: Smooth muscles have a unique ability to maintain contraction over extended periods without significant energy expenditure, known as the latch state. This mechanism allows smooth muscles to sustain tension even as levels of phosphorylated myosin light chain kinase diminish.
- Neurotransmitter-Induced Contraction: In certain smooth muscles, neurotransmitters can induce contraction without the need for action potentials. For instance, in the eye’s iris, neurotransmitters like norepinephrine and acetylcholine can produce a depolarization termed a junctional potential, leading to muscle contraction.
In summary, smooth muscle contraction is a multifaceted process, relying on calcium dynamics, protein interactions, and unique electrical properties. This intricate mechanism ensures the efficient and sustained functioning of various physiological systems, from vascular tone regulation to gastrointestinal motility.
Invertebrate smooth muscle
Invertebrates, a diverse group of animals lacking a vertebral column, possess a unique smooth muscle mechanism distinct from their vertebrate counterparts. The intricacies of invertebrate smooth muscle contraction and its energy-efficient nature are pivotal for the survival and functionality of these organisms.
- Initiation of Contraction: In invertebrate smooth muscle, the contraction process is instigated when calcium ions directly bind to myosin. This binding facilitates the rapid cycling of cross-bridges, which in turn produces force. This mechanism is reminiscent of the contraction process in vertebrate smooth muscles, albeit with some distinct differences.
- Catch Phase: A notable feature of invertebrate smooth muscle contraction is the presence of a sustained, low-energy utilization phase known as the catch phase. During this phase, the muscle can maintain its contracted state for extended periods without significant energy expenditure. This energy-efficient mechanism is particularly advantageous for invertebrates, allowing them to perform vital functions without depleting their energy reserves rapidly.
- Role of Twitchin: The catch phase’s prolonged contraction is attributed to a specialized protein named twitchin. Twitchin exhibits similarities to both the myosin light-chain kinase, which plays a role in vertebrate smooth muscle contraction, and the elastic protein titin, which provides structural stability and elasticity to muscle fibers. The presence of twitchin in invertebrate smooth muscle is a testament to the evolutionary adaptations these organisms have developed to optimize their muscle functionality.
- Functional Significance in Bivalve Mollusks: Bivalve mollusks, such as clams, have harnessed the catch phase’s benefits to fulfill a crucial ecological role. These organisms can keep their shells securely closed for extended durations using the catch phase of their smooth muscles. This ability not only conserves energy but also offers protection against potential predators and environmental stressors.
In conclusion, invertebrate smooth muscles, through their unique contraction mechanisms and the incorporation of specialized proteins like twitchin, showcase the evolutionary adaptability of muscle systems across diverse animal taxa. These adaptations ensure that invertebrates can efficiently perform essential functions in their respective ecological niches.
Examples of Smooth Muscle
Smooth muscle tissue, characterized by its non-striated appearance and involuntary control, is ubiquitously distributed throughout the human body. It plays a pivotal role in facilitating various physiological processes by enabling the movement and modulation of internal organs and structures. Here are specific examples of where smooth muscle can be found:
- Blood Vessels: Smooth muscle layers in blood vessels, particularly in the tunica media, regulate vessel diameter, thereby controlling blood flow and pressure.
- Gallbladder: The presence of smooth muscle in the gallbladder aids in the release of bile, facilitating digestion.
- Intestines: Smooth muscle layers in the intestines, both in the small and large intestines, produce coordinated contractions known as peristalsis, propelling digested food and waste products.
- Stomach: The walls of the stomach contain smooth muscle that aids in mixing and churning food with gastric juices, promoting digestion.
- Urinary System: Smooth muscle in structures like the urinary bladder allows for the storage and expulsion of urine.
- Iris of the Eye: The smooth muscle in the iris, known as the sphincter and dilator pupillae, controls pupil size, adjusting the amount of light entering the eye.
- Prostate: The prostate gland, predominantly composed of smooth muscle, plays a role in the reproductive system by aiding in the expulsion of seminal fluid.
- Respiratory System: Smooth muscle in the respiratory tract, especially in the bronchi and bronchioles, modulates airway diameter, influencing airflow.
- Reproductive System: Smooth muscle is integral to the reproductive organs, facilitating processes like the transport of sperm in males and the movement of the ova in females.
- Uterus: The uterine wall is rich in smooth muscle, known as the myometrium, which contracts during childbirth to facilitate the delivery of the baby.
- Arrector Pili in the Skin: These tiny smooth muscles, associated with hair follicles, contract in response to cold or emotional stimuli, causing hair to stand erect, a phenomenon colloquially termed “goosebumps.”
In conclusion, smooth muscle’s widespread distribution underscores its significance in maintaining homeostasis and ensuring the efficient functioning of various physiological processes. Recognizing its presence in diverse organs and systems provides a comprehensive understanding of its multifaceted role in human physiology.
Smooth Muscles Functions
Smooth muscle tissue, characterized by its involuntary and non-striated nature, is intricately woven into various body systems, playing a pivotal role in maintaining physiological homeostasis. Its functions, determined by its specific location, are multifaceted and crucial for the body’s proper functioning.
- Cardiovascular System: Within the cardiovascular system, vascular smooth muscle cells are embedded in the tunica media layer of most vascular segments, with the exception of capillaries. Their ability to contract and dilate, known as vasoconstriction and vasodilation respectively, is paramount in modulating blood vessel diameter. This regulation directly influences blood distribution and blood pressure maintenance.
- Respiratory System: In the respiratory system, the walls of bronchi and bronchioles are lined with smooth muscle layers. These muscles play a crucial role in adjusting airflow into the lungs, ensuring optimal oxygenation and carbon dioxide removal.
- Gastrointestinal Tract: The gastrointestinal tract houses extensive layers of smooth muscle. These muscles facilitate the movement of food through the digestive system via coordinated contractions called peristalsis. Additionally, the gallbladder’s smooth muscle aids in the release of bile into the digestive tract, assisting in digestion.
- Urinary System: Smooth muscle layers in the urinary system, specifically in the ureters and bladder, facilitate the transportation and expulsion of urine, a key process in waste elimination.
- Male Reproductive Tract: In the male reproductive system, the vas deferens contains smooth muscle layers that assist in sperm transport. Additionally, these muscles play a role in the ejection of glandular secretions from the prostate, seminal vesicle, and bulbourethral glands.
- Female Reproductive Tract: The uterus’s myometrium is predominantly composed of smooth muscle. During labor, under the influence of oxytocin, these muscles contract, aiding in the delivery of the fetus.
- Ophthalmic System: In the eye, the ciliary muscle’s contractions and relaxations adjust the lens’s size and shape, modulating the amount of light entering the eye and ensuring clear vision.
- Integumentary System: Associated with hair follicles in the skin are the erector pili muscles. These smooth muscles contract in response to cold temperatures, causing hair to stand erect, a mechanism that aids in heat conservation.
- Contraction: Smooth muscles, akin to other muscle types, possess the inherent ability to contract. This contraction facilitates movement, such as the propulsion of food through the digestive tract.
- Autonomous Functioning: Smooth muscle tissue operates autonomously, governed by the autonomic nervous system. This ensures that vital functions, like breathing, occur seamlessly without conscious effort. For instance, in response to varying CO2 and oxygen levels, blood vessels’ smooth muscles undergo vasodilation and vasoconstriction, respectively.
- 11. Homeostasis Regulation: Smooth muscles play a pivotal role in maintaining homeostasis. An example is the arrector pili muscles attached to skin hair follicles. In cold conditions, these muscles contract, raising the skin hair, which in turn aids in heat generation and retention.
In conclusion, smooth muscles, with their diverse distribution and functions, are indispensable for the human body. Their roles range from facilitating movement to regulating homeostasis, underscoring their significance in ensuring physiological equilibrium.
Differences Between Single unit (visceral) smooth muscle and Multiunit smooth muscle
Smooth muscles, integral to various physiological processes, are organized in two distinct arrangements based on their structural and functional attributes. Here, we delineate the differences between single-unit (visceral) smooth muscles and multiunit smooth muscles:
Criteria | Single-unit (Visceral) Smooth Muscle | Multiunit Smooth Muscle |
---|---|---|
Frequency | More prevalent in the body. | Less commonly found. |
Fibre Coupling | Fibres are interconnected by gap junctions, facilitating electrical coupling. As a result, when one fibre contracts, the entire unit contracts synchronously. | Lacks gap junctions, meaning fibers operate independently. A stimulus affects only the specific fiber it targets, without influencing neighboring cells. |
Stimulation Mechanisms | – Autonomic nerves – Hormones – Exhibits a stress-relaxation response. | – Autonomic nerves – Hormones |
Location | Predominantly found in the walls of visceral organs, excluding the heart. | Located in large blood vessels, eyes, and respiratory airways. |
Contraction Characteristics | Engenders slow, sustained contractions, facilitating the movement of substances within the body, such as food propulsion in the gastrointestinal tract. | Produces asynchronous contractions, allowing for more localized and specific muscle actions. |
In essence, while both single-unit and multiunit smooth muscles play pivotal roles in various physiological processes, their structural and functional distinctions make them uniquely suited to their respective locations and functions within the body.
Quiz
Which of the following is NOT a characteristic of smooth muscle?
a) Striated appearance
b) Involuntary control
c) Single nucleus
d) Spindle-shaped cells
Smooth muscles are primarily found in which of the following?
a) Limbs
b) Heart
c) Hollow organs
d) Head
Which protein binds to calcium in smooth muscle cells to initiate contraction?
a) Troponin
b) Tropomyosin
c) Calmodulin
d) Actin
Which type of smooth muscle contracts as a single unit due to the presence of gap junctions?
a) Skeletal muscle
b) Cardiac muscle
c) Single-unit smooth muscle
d) Multiunit smooth muscle
Which of the following systems does NOT contain smooth muscle?
a) Digestive system
b) Respiratory system
c) Skeletal system
d) Urinary system
The contraction of smooth muscle is regulated by which of the following ions?
a) Sodium
b) Potassium
c) Calcium
d) Magnesium
Which type of smooth muscle is found in large blood vessels and the eyes?
a) Cardiac muscle
b) Skeletal muscle
c) Single-unit smooth muscle
d) Multiunit smooth muscle
Which of the following is responsible for moving food through the digestive tract?
a) Peristalsis
b) Osmosis
c) Diffusion
d) Filtration
In smooth muscle, the dense bodies are equivalent to which structure in skeletal muscle?
a) Sarcomeres
b) Z-lines
c) M-lines
d) H-zone
Which enzyme is activated by the calcium-calmodulin complex in smooth muscle cells?
a) ATPase
b) Myosin light chain kinase
c) Actin polymerase
d) Troponin kinase
FAQ
What is smooth muscle?
Smooth muscle is a type of involuntary muscle found in the walls of hollow organs and structures, characterized by its non-striated appearance and ability to contract and relax without conscious control.
Where is smooth muscle located in the body?
Smooth muscle is primarily located in the walls of hollow organs such as the stomach, intestines, urinary bladder, uterus, blood vessels, and respiratory airways.
How does smooth muscle differ from skeletal and cardiac muscles?
Unlike skeletal and cardiac muscles, smooth muscle does not have striations. It operates involuntarily, and its cells are spindle-shaped with a single nucleus.
What controls the contraction of smooth muscle?
The contraction of smooth muscle is controlled by the autonomic nervous system, hormones, and local factors. Calcium plays a crucial role in the contraction mechanism.
What is the significance of peristalsis in smooth muscle function?
Peristalsis is a rhythmic, wave-like contraction of smooth muscles, especially in the gastrointestinal tract, that helps move substances like food through the body.
Why is smooth muscle called “involuntary”?
Smooth muscle is termed “involuntary” because its contractions are not under conscious control. Instead, they are automatically regulated by the body based on various physiological needs.
How does smooth muscle maintain prolonged contraction with minimal energy?
Smooth muscle can maintain prolonged contraction through a mechanism known as the “latch state,” allowing it to sustain tension with reduced ATP consumption.
What is the role of smooth muscle in blood vessels?
In blood vessels, smooth muscle helps regulate blood flow and pressure by contracting (vasoconstriction) or relaxing (vasodilation), thereby changing the diameter of the vessel.
Can smooth muscle regenerate?
Yes, smooth muscle cells have a limited ability to regenerate after injury, primarily through the proliferation of existing smooth muscle cells.
What are the primary stimuli for smooth muscle contraction?
The primary stimuli for smooth muscle contraction include neuronal signals from the autonomic nervous system, hormones, and local factors like changes in pH or oxygen levels.
References
- Hafen BB, Shook M, Burns B. Anatomy, Smooth Muscle. [Updated 2023 Jul 17]. In: StatPearls [Internet]. Treasure Island (FL): StatPearls Publishing; 2023 Jan-. Available from: https://www.ncbi.nlm.nih.gov/books/NBK532857/
- Gunner, J. (2020). Smooth Muscle Examples and Function. YourDictionary. https://examples.yourdictionary.com/smooth-muscle-examples.html
- Smooth muscle | anatomy. (2020). Britannica. https://www.britannica.com/science/smooth-muscle
- Metz, R., Patterson, J., & Wilson, E. (2011). Vascular Smooth Muscle Cells: Isolation, Culture, and Characterization. Methods in Molecular Biology, 169-176. https://doi.org/10.1007/978-1-61779-523-7_16
- Taylor, T. (2015). Visceral Muscle Tissue – Anatomy Pictures and Information. Innerbody. https://www.innerbody.com/image_musc01/musc02.html#:~:text=Visceral%20muscle%20tissue%2C%20or%20smooth,skeletal%2C%20smooth%2C%20and%20cardiac.
- Sinha, S., Iyer, D., & Granata, A. (2014). Embryonic origins of human vascular smooth muscle cells: implications for in vitro modeling and clinical application. Cell Molecular Life Sciences, 71(12), 2271-88.
- Chen, S. N., Wang, Y. Q., Hao, C. L., Lu, Y. H., Jiang, W. J., Gao, C. Y., & Wu, M. (2019). Multisystem smooth muscle dysfunction syndrome in a Chinese girl: A case report and review of the literature. World Journal of Clinical Cases, 7(24), 4355–4365. https://doi.org/10.12998/wjcc.v7.i24.4355
- Bargehr, J., Low, L., Cheung, C., Bernard, W. G., Iyer, D., Bennett, M. R., Gambardella, L., & Sinha, S. (2016). Embryological Origin of Human Smooth Muscle Cells Influences Their Ability to Support Endothelial Network Formation. STEM CELLS Translational Medicine, 5(7), 946–959. https://doi.org/10.5966/sctm.2015-0282
- Hafen BB, Burns B. Physiology, Smooth Muscle. [Updated 2023 Aug 14]. In: StatPearls [Internet]. Treasure Island (FL): StatPearls Publishing; 2023 Jan-. Available from: https://www.ncbi.nlm.nih.gov/books/NBK526125/
- https://www.kenhub.com/en/library/anatomy/smooth-musculature
- https://open.oregonstate.education/aandp/chapter/10-7-smooth-muscle-tissue/
- https://www.biologyonline.com/dictionary/smooth-muscle
- https://teachmephysiology.com/histology/tissue-structure/muscle-histology/smooth-muscle/
- https://www.vedantu.com/biology/smooth-muscle
- https://biologydictionary.net/smooth-muscle/