What are Sense Organs?
- Sense organs are specialized structures that allow living organisms to interact with their environment by detecting various stimuli and converting them into signals the brain can interpret. These organs are essential for survival, as they help individuals respond to changes in their surroundings. Humans have five primary sense organs, each designed to process specific types of sensory information.
- The first of these is the eye, which is responsible for the sense of sight or vision. The eye contains specialized cells called photoreceptors, including rods and cones, which are sensitive to light. Rods detect light intensity and allow vision in dim lighting, while cones are responsible for color perception. When light enters the eye, it is focused onto the retina, where photoreceptors convert light into electrical signals. These signals are sent to the brain via the optic nerve, allowing us to perceive images and colors.
- The second sense organ, the ear, is associated with hearing or audition. The ear detects sound through tiny hair cells located in the cochlea, a spiral-shaped structure within the inner ear. Sound waves cause these hair cells to vibrate, and these vibrations are translated into electrical signals that are transmitted to the brain by the auditory nerve. Additionally, the ear also plays a crucial role in balance, as fluid-filled canals in the inner ear detect changes in head position and movement.
- The nose is the third sense organ, responsible for the sense of smell or olfaction. Inside the nose, there are specialized olfactory receptors located in the upper nasal cavity. These receptors detect chemical molecules from odors in the air. Once the olfactory receptors bind to odor molecules, they send signals to the brain’s olfactory bulb, where smells are identified and processed.
- Next is the tongue, which governs the sense of taste or gustation. The surface of the tongue is covered with tiny bumps called papillae, which house the taste buds. These taste buds contain receptors that detect five basic tastes: sweet, sour, salty, bitter, and umami. Each type of receptor is sensitive to different molecules in food, and these signals are sent to the brain to create the perception of taste.
- Lastly, the skin functions as the sense organ for touch. It is the body’s largest organ and contains a variety of sensory receptors, including mechanoreceptors, thermoreceptors, and nociceptors. These receptors respond to physical sensations such as pressure, temperature, and pain. The sensory information is transmitted to the brain, where it is interpreted, helping us perceive different textures, temperatures, and levels of discomfort or pain.
Types of Sensory Receptors
Sensory receptors in vertebrates are specialized structures that detect various types of stimuli from both the external and internal environments. These receptors convert sensory input into electrical signals, which are then transmitted to the brain for processing. Sensory receptors are classified based on their distribution, location, and the type of energy they detect. Understanding these classifications is critical to comprehending how organisms perceive and interact with their surroundings.
- Classification of Sensory Receptors Based on Distribution:
- General Sensory Receptors:
- These receptors are widely distributed throughout the body and are involved in detecting sensations like touch, temperature, and proprioception (awareness of body position and movement).
- They can be divided into three categories: a. Free Sensory Receptors:
- The sensory nerve ending lacks any specialized structure and is referred to as a free nerve ending. These receptors are primarily involved in detecting pain but can also respond to extreme temperatures. When tissue damage occurs, it can directly stimulate these receptors, leading to sensations of pain. b. Encapsulated Sensory Receptors:
- In these receptors, the terminal end of the sensory neuron is enclosed in a specialized structure formed of mesodermal cells. They are located just beneath the epidermis in the skin and are responsive to touch, vibration, and heat. An example is the Ruffini sensory receptor, which detects touch, pressure, and temperature. c. Associated Sensory Receptors:
- Here, the sensory neuron’s terminal end wraps around another organ. For instance, nerve endings associated with hair follicles are stimulated when the hair is moved, providing sensory input related to touch.
- General Sensory Receptors:
- Classification Based on Location:
- Somatic Receptors:
- These are found on the body’s surface, skin, or skeletal muscles. They detect external stimuli such as touch and temperature.
- Visceral Sensory Receptors:
- Located within the body’s viscera (internal organs), these receptors detect sensations such as internal pressure or pain originating from organs.
- Somatic Receptors:
- Classification Based on the Type of Energy Detected:
- Sensory receptors are categorized according to the type of energy or stimuli they can perceive, which includes mechanical, chemical, light, or thermal stimuli. These are further divided into:
- Exteroreceptors (External Senses):
- These receptors respond to external environmental stimuli and include:
- Photoreceptors for sight.
- Phonoreceptors for hearing.
- Olfactoreceptors for smell.
- Gustatoreceptors for taste.
- Tangoreceptors for touch.
- Mechanoreceptors for pressure and mechanical forces.
- Thermoreceptors for temperature.
- Nociceptors for pain.
- Rheoreceptors for detecting water currents.
- These receptors respond to external environmental stimuli and include:
- Interoreceptors (Internal Senses):
- These receptors detect stimuli from within the body, including:
- Proprioreceptors, located in muscles, joints, and tendons, which provide information about body position and movement.
- These receptors detect stimuli from within the body, including:
- Exteroreceptors (External Senses):
- Sensory receptors are categorized according to the type of energy or stimuli they can perceive, which includes mechanical, chemical, light, or thermal stimuli. These are further divided into:
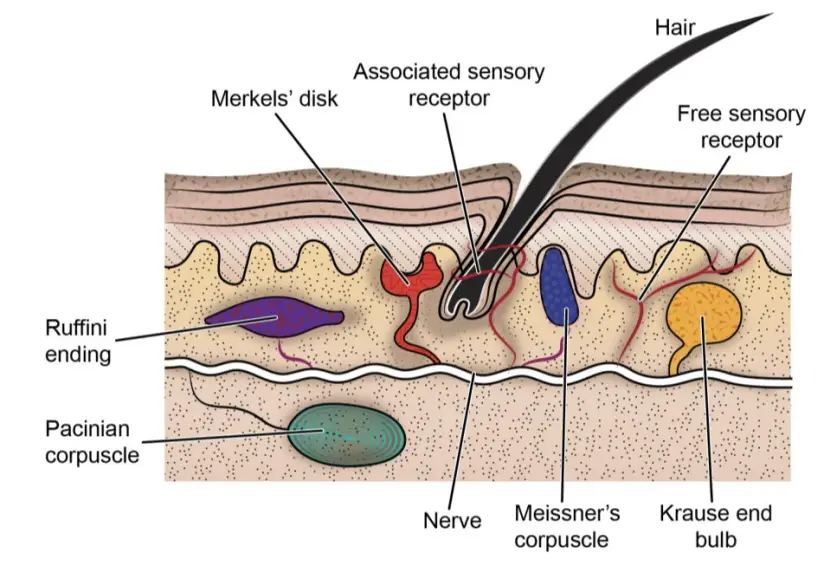
Photoreceptor organs of vertebrates
Photoreceptor organs in vertebrates are specialized structures designed to detect and process light, enabling organisms to perceive their surroundings. Among vertebrates, the most advanced and complex photoreceptor organs are the eyes. These organs originate from the brain and are essential for various biological functions, including vision and light regulation. Vertebrate photoreceptors can be divided into two types: the parietal (median) eye and the paired lateral eyes, each serving different purposes.
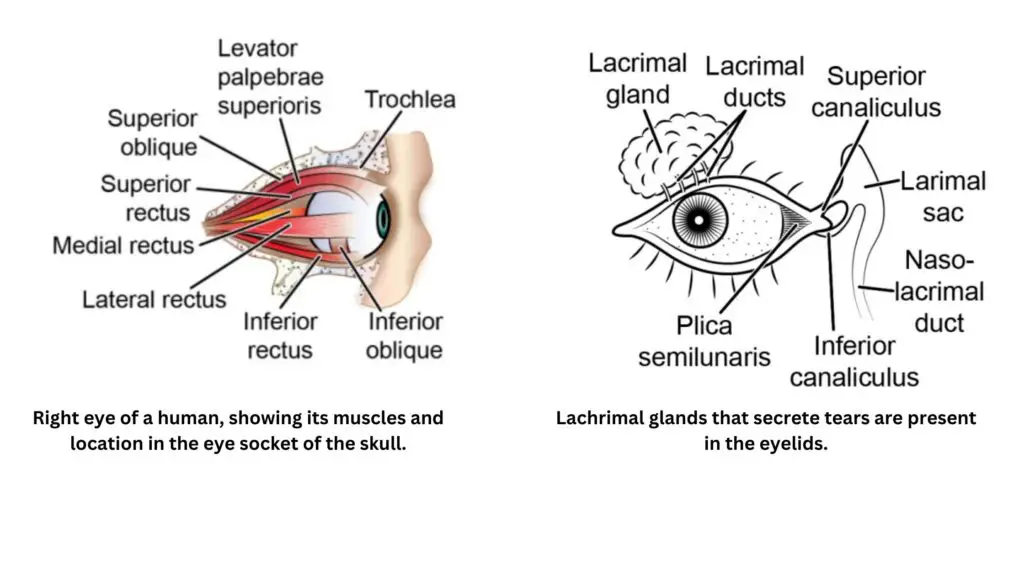
- Parietal Eye:
- The parietal, also known as the median or pineal eye, is the simplest photoreceptor organ found in some vertebrates, such as certain fish and lizards. This eye originates from the diencephalon of the brain and is positioned at the top of the head.
- It is a small sensory structure consisting of thousands of sensory cells that send light-based information to the brain. A transparent lens overlays the sensory layer, focusing incoming light on the sensory cells.
- The parietal eye acts as a dosimeter of light exposure, helping regulate biological processes such as circadian rhythms and thermoregulation. Unlike the paired lateral eyes, it does not form images but instead senses the intensity and duration of light.
- Paired Lateral Eyes (Vertebrate Eye):
- All vertebrates possess paired lateral eyes, which are more complex and highly developed in structure and function than the parietal eye. These eyes share a basic construction plan across vertebrate species.
- The vertebrate eye originates from three embryonic sources: the diencephalon (forming the retina and optic nerve), the ectoderm (forming the lens and part of the cornea), and the mesoderm (contributing to the sclera, muscles, and surrounding tissues).
- A detailed look at the human eye, representative of mammalian eyes, reveals a sophisticated structure that ensures effective vision. Before describing the core components, it’s essential to understand the auxiliary structures that protect and support the eye.
- Auxiliary Structures of the Eye:
- Bony Eye Orbit: Each eye is housed in a protective bony socket called the orbit, which shields the eyes from mechanical injuries.
- Extraocular Eye Muscles: Six muscles are attached to each eye, facilitating precise eye movements.
- Layer of Orbital Fat: Surrounding the eye and its muscles is a cushion of orbital fat, enabling smooth and stable rotation of the eye around a fixed point known as the center of rotation.
- Eyebrows: Positioned above the eyes, eyebrows are composed of skin and hair, which protect the eyes from sweat, dust, and foreign particles.
- Eyelids and Eyelashes: The eyelids are two movable folds of skin (upper and lower) that protect the eyes by closing over them. The eyelids are lined with eyelashes that further guard against dust and foreign objects. Additionally, the third eyelid, or plica semilunaris, is a vestigial structure that, in some vertebrates, plays a role in protecting and moistening the eyes.
- Lachrymal Glands (Tear Glands): The eyelids contain lachrymal glands, which secrete lachrymal fluid (tears) that keep the cornea moist and remove debris from the eye’s surface.
- Conjunctiva: The conjunctiva is a clear mucous membrane that lines the inner eyelid and extends over the anterior surface of the eye. It serves to lubricate and protect the eye, ensuring smooth eye movement and shielding the eye from irritants.
Structure of the human eye
The human eye, like that of all vertebrates, is an intricate structure composed of multiple layers, chambers, and specialized components that work together to enable vision. This organ is vital in translating light into images, and its complex design reflects its critical function. The eye is encased within three coats, and these layers surround the aqueous humor, lens, and vitreous body containing the vitreous humor.
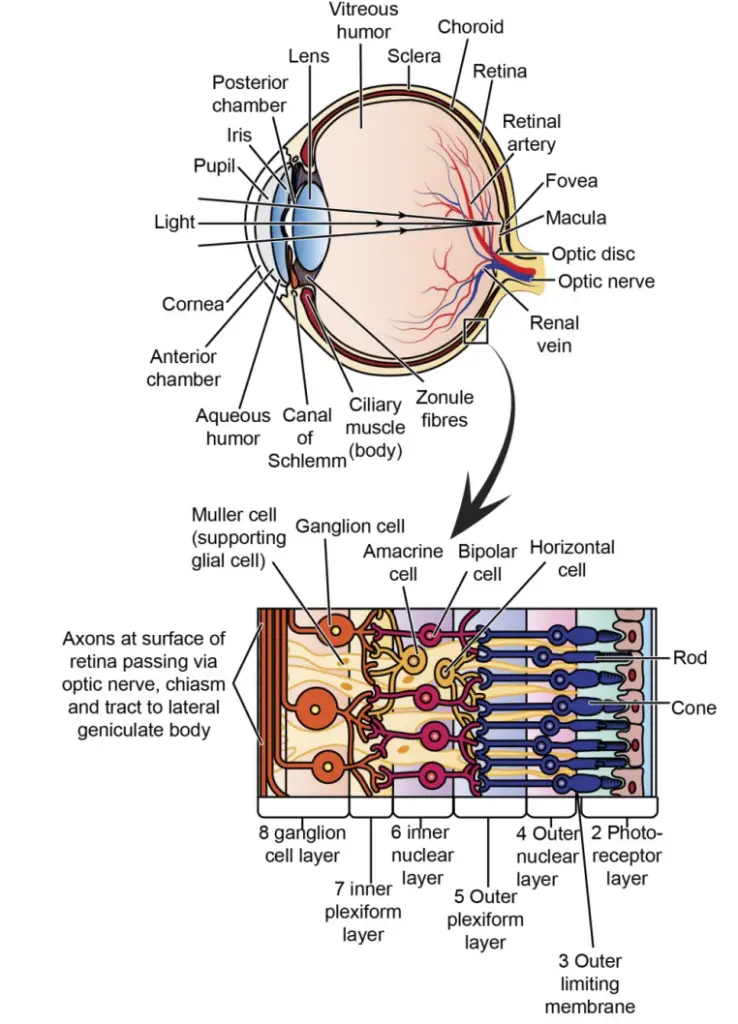
- Sclera: The outermost layer of the eye, also known as the white of the eye, is a tough connective tissue that maintains the shape of the eyeball. It also provides attachment points for the muscles that control eye movement.
- Conjunctiva: A membrane that lines the inside of the eyelids and attaches to the sclera, providing a protective covering.
- Cornea: The transparent, front-facing part of the sclera. It functions as a window that allows light to enter the eye and works alongside the lens to focus light on the retina.
- Anterior Chamber: The space between the cornea and the pupil, filled with aqueous humor.
- Aqueous Humor: A clear fluid located behind the cornea, which fills the anterior chamber. It helps maintain the shape of the cornea, provides nutrients to the cornea and lens, and facilitates waste removal from the front of the eye.
- Uvea: The middle layer of the eye, located between the sclera and the retina. It contains numerous blood vessels and includes the choroid, ciliary body, and iris.
- Choroid: A pigmented layer within the uvea, located toward the back of the eye. Its primary function is to absorb excess light, preventing it from scattering inside the eye.
- Ciliary Body: Composed of ciliary muscles and processes, the ciliary body is responsible for secreting aqueous humor and adjusting the lens to focus light, a process known as accommodation.
- Iris: The anterior part of the uvea, situated just behind the cornea. This pigmented muscular diaphragm controls the size of the pupil and thus the amount of light entering the eye. The iris also determines eye color, with its pigmentation varying among individuals.
- Pupil: The central opening in the iris that regulates the amount of light entering the eye. It changes size depending on the light intensity—constricting in bright light and dilating in dim conditions.
- Lens: Located immediately behind the pupil, the lens is a transparent, flexible structure that focuses light onto the retina. It changes shape, becoming more curved for near vision and flatter for distant vision, adjusting to ensure a clear image.
- Retina: The innermost layer of the eye, the retina is a sensory tissue that lines the back of the eye and contains photoreceptor cells—rods and cones. These cells convert light into electrical signals, which are transmitted to the brain through the optic nerve.
- Optic Nerve: Comprised of the axons of retinal ganglion cells, the optic nerve transmits visual information from the retina to the brain.
Retina
- The retina extends from the rear of the eye to the ciliary body. It functions as a complex neural and sensory layer responsible for detecting light and converting it into chemical energy.
- Photoreceptor Cells: The two types of photoreceptors, rods and cones, are crucial for vision. Rods are highly sensitive to dim light but cannot detect color, while cones are less sensitive to low light but are responsible for color vision and detecting fine detail.
- Retinal Function: The retina converts light energy into electrical impulses, which are processed by neurons, including bipolar and ganglion cells. These impulses are transmitted through the optic nerve to the brain for interpretation.
- Fovea: A small central area in the retina, densely packed with cones and devoid of rods, responsible for sharp, detailed vision.
Lens and Image Formation
- Light entering the eye is refracted by both the cornea and the lens. The cornea handles most of the refraction, while the lens fine-tunes the focus by adjusting its shape. This process ensures that images are sharply focused on the retina.
- The zonule fibers, also known as suspensory ligaments, connect the lens to the ciliary body, facilitating the lens’ movement and shape adjustments.
Chambers of the Eye
- The eye contains three chambers filled with fluid:
- Anterior Chamber: Between the cornea and iris, filled with aqueous humor.
- Posterior Chamber: Between the iris and the lens, also filled with aqueous humor.
- Vitreous Chamber: The largest chamber, located between the lens and the retina. It is filled with a gel-like substance called vitreous humor, which helps maintain the shape of the eyeball and ensures the retina remains pressed against the choroid.
Binocular Vision
- Humans possess binocular vision, meaning both eyes focus on a single object simultaneously. This occurs due to the crossing of the optic nerves at the optic chiasma. The left and right optic nerves decussate, allowing visual information from both eyes to be processed together, resulting in a single, cohesive image. This contrasts with monocular vision seen in some animals, where each eye focuses independently on different objects.
Comparative Anatomy of the Vertebrate Eye
The comparative anatomy of the vertebrate eye reveals significant variations in structure and function across different classes, driven by their distinct habitats and lifestyles. While the general pattern of the vertebrate eye is consistent, the specific adaptations reflect the evolutionary pressures of the environment, influencing aspects like vision type, accommodation, retinal development, and pupil shape.
- Other Mammals
The structure of the human eye is typical of most mammals. However, variations exist between aquatic, terrestrial, and aerial mammals. In nocturnal species, a tapetum lucidum is present, enhancing vision in low-light conditions. The pupil is generally round, although variations can occur. Most mammals possess a retina comprising both rods and cones, though color vision is predominantly limited to higher primates. Optic nerve decussation in mammals supports binocular vision, which enables depth perception and a three-dimensional view of the environment. - Birds
Birds are primarily adapted for aerial vision. Most species exhibit uniocular vision, although some, like owls and birds of prey (hawks and eagles), have binocular vision. The avian eyeball is large relative to body size, aiding their aerial lifestyle. A distinctive feature in birds is the presence of an ossified sclerotic ring around the ciliary region. Additionally, a well-developed nictitating membrane offers eye protection during flight. Birds’ eyes show advanced accommodation mechanisms, with ciliary muscles altering the shape of the cornea for precise vision. Diurnal birds possess more cones, while nocturnal species have more rods, supporting both types of vision. Birds have foveas, with some species having two, enhancing vision resolution. A unique structure, pectin, believed to aid in movement perception and retinal nourishment, extends into the posterior chamber from the optic nerve. - Reptiles
Reptilian eyes are adapted to terrestrial environments. Unlike other vertebrates, many reptiles (except snakes) possess movable eyelids and a true nictitating membrane. Most reptiles also have Harderian and lacrimal glands, though these glands are poorly developed in certain species like snakes, chameleons, and Sphenodon. The reptilian retina has a higher number of cones, and a central area for acute vision is common. Color vision is likely in turtles and lizards but less certain in crocodiles and snakes. Snakes, having fused eyelids, develop a transparent skin called a spectacle over their eyes. A notable feature in reptiles is their specialized ciliary apparatus that can alter the shape of both the lens and cornea, allowing accommodation for both near and distant vision. - Amphibians
Amphibian eyes are structurally similar to those of other vertebrates but include some specific features. Amphibians use retractor bulbi muscles to retract their eyes into the socket and levator bulbi muscles to protrude them outward. Their eyes have ciliary bodies and poorly developed lacrimal glands. However, their eyes are kept moist by glandular secretions. Amphibians are the first vertebrates to possess movable eyelids and secretions that moisten the eyes. The lack of a tapetum lucidum means their eyes do not reflect light, despite their shiny appearance. It is believed that amphibians lack color vision. Among amphibians, anurans (frogs and toads) have the best-developed eyes, while caudate amphibians (salamanders) have smaller eyes, often degenerated in cave-dwelling species. - Fishes
Fish eyes vary widely between species. In cartilaginous fishes, eyes are large and held in place by six eye muscles, with an optic pedicel supporting the eye in some species. The sclera is cartilaginous, and ciliary muscles are absent. Notably, in elasmobranchs (sharks and rays), the choroid coat contains light-reflecting guanine crystals, enhancing vision in dim conditions. While cones are absent in elasmobranchs, bony fishes display widespread color vision. In most teleosts (advanced bony fishes), the eye is adapted for near vision, with the lens being moved closer to the retina for distant vision. Special adaptations also exist for vision in air and water. Deep-sea fishes have particularly large eyes, aiding vision in low-light environments. Some species, like flatfishes, have both eyes on one side of the head, while cave-dwelling species may have degenerated eyes due to their dark habitats. - Cyclostomes
Cyclostomes, such as hagfish and lampreys, display primitive eye structures. In hagfish, the eyes are small, degenerated, and non-functional, with the cornea, sclera, and choroid undifferentiated. In contrast, lampreys possess more developed eyes, though still primitive. Their eyeballs are flattened, and the sclera and cornea are separate from the skin. Lampreys lack a suspensory ligament and ciliary apparatus, have fixed-size pupils, and do not have eyelids. Rods outnumber cones, emphasizing their limited visual capacity.
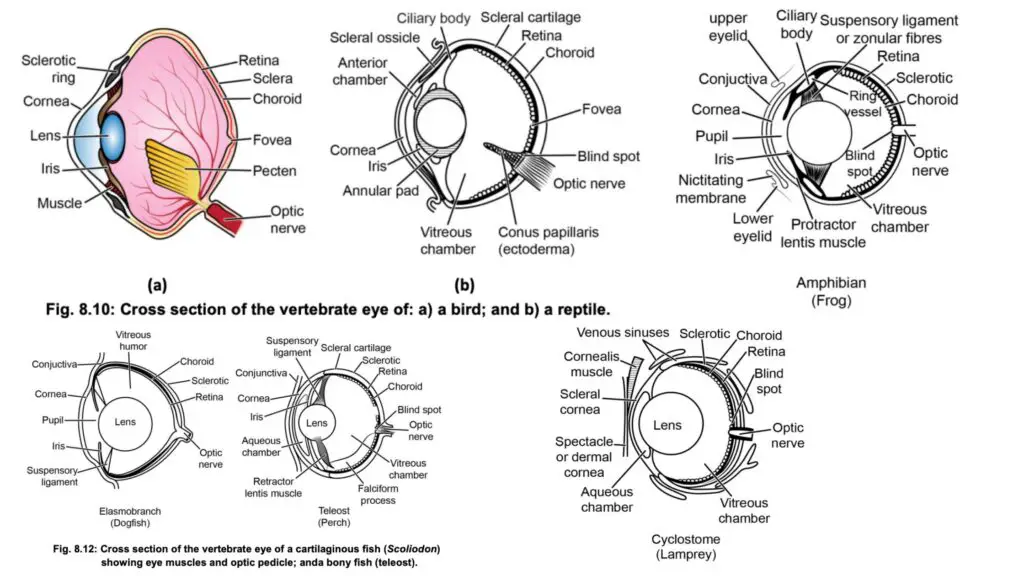
The vertebrate ear
The vertebrate ear is a specialized sensory organ responsible for detecting sound waves in the environment. In higher forms, it functions in both hearing and equilibrium. Although what we commonly see as the ear in mammals is the external ear, the actual processes of hearing and balance are carried out by the internal ear. This internal structure, protected by the bony skull, is highly conserved across vertebrates and evolved primarily as a mechanism for balance before adapting to detect sound.
- Development of the Vertebrate Ear
- The vertebrate internal ear originated from the labyrinth or vestibular apparatus, which initially evolved as a structure dedicated to maintaining balance and equilibrium.
- Hearing in vertebrates likely developed as a survival mechanism, alerting animals to nearby dangers.
- Eventually, hearing also became crucial for other functions such as locating food, finding mates, and communication with other individuals.
- Internal Ear Structure and Function
- The vertebrate internal ear, enclosed within the skull for protection, is the primary organ responsible for both hearing and equilibrium.
- Over evolutionary time, part of the inner ear became adapted to receive and process sound waves.
- Certain hair cells in the inner ear specialized to detect vibrations caused by sound, thus enabling the perception of auditory stimuli.
- Dual Function: Hearing and Balance
- While hearing allows vertebrates to detect sound, the ear also plays a critical role in maintaining balance and spatial orientation.
- The structures involved in equilibrium, derived from the original labyrinth, remain consistent across vertebrate species, emphasizing the ear’s foundational role in balance before its adaptation to include auditory functions.
Auditory System of Mammals
The auditory system of mammals, exemplified by the human ear, is a complex organ responsible for detecting sound and maintaining balance. As a sensory organ, it functions through the conversion of sound waves into electrochemical signals while also playing a role in equilibrium. The mammalian ear is anatomically divided into three main sections: the outer ear, the middle ear, and the inner ear, each contributing to the process of hearing and balance.
- Outer Ear Structure and Function
- The outer ear consists of the pinna (auricle) and the external auditory canal (external auditory meatus).
- The pinna is the visible, external part of the ear, which collects sound waves from the environment.
- The external auditory canal channels these sound waves toward the tympanic membrane (eardrum), a thin, semi-transparent membrane that closes the canal at its internal end.
- The canal is lined with fine outward-pointing hairs and modified sweat glands that produce earwax (cerumen), which helps protect the ear by discouraging insects from entering.
- The tympanic membrane separates the outer ear from the middle ear and is responsible for transmitting sound waves into the middle ear.
- The outer ear consists of the pinna (auricle) and the external auditory canal (external auditory meatus).
- Middle Ear Anatomy and Role
- The middle ear is an air-filled cavity within the temporal bone of the skull. It contains three small bones, known as ossicles, which link the tympanic membrane to the oval window of the inner ear.
- The ossicles include the malleus (hammer), incus (anvil), and stapes (stirrup). These bones are suspended by ligaments and vibrate in response to sound, transmitting vibrations from the tympanic membrane to the inner ear.
- The eustachian tube, part of the middle ear, connects to the nasopharynx and functions to equalize air pressure on both sides of the tympanic membrane.
- The middle ear’s primary role is to transmit and amplify sound waves from the outer ear to the inner ear.
- The middle ear is an air-filled cavity within the temporal bone of the skull. It contains three small bones, known as ossicles, which link the tympanic membrane to the oval window of the inner ear.
- Inner Ear Structure and Function
- The inner ear connects to the middle ear through the oval window, which is covered by the footplate of the stapes. It consists of a bony labyrinth that houses the membranous labyrinth, filled with endolymph, a potassium-rich fluid.
- The bony labyrinth is divided into the vestibule, semicircular canals, and the cochlea.
- The vestibule and semicircular canals are involved in balance and equilibrium, containing sensory organs that help with postural coordination.
- The cochlea, a spirally coiled structure, is responsible for hearing. It contains the scala vestibuli, scala tympani, and scala media, with the scala vestibuli and scala tympani filled with perilymph.
- The inner ear connects to the middle ear through the oval window, which is covered by the footplate of the stapes. It consists of a bony labyrinth that houses the membranous labyrinth, filled with endolymph, a potassium-rich fluid.
- Cochlea and Hearing Mechanism
- The cochlea is divided into three canals: scala vestibuli, scala tympani, and scala media, with the helicotrema at the apex allowing communication between the first two.
- Sound waves enter the cochlea through the oval window into the scala vestibuli and travel through the cochlea, ultimately exiting via the round window through the scala tympani.
- The Organ of Corti, located on the basilar membrane within the scala media, is the primary sensory organ for hearing.
- The Organ of Corti contains rows of inner hair cells and outer hair cells, which are mechanoreceptors that convert mechanical sound vibrations into electrical signals.
- The inner hair cells, numbering around 3,500, are the primary auditory receptors, while the outer hair cells receive neural input from the brain.
- Signal Transmission Pathway
- When sound vibrations reach the cochlea, they cause movement in the basilar membrane and hair cells within the Organ of Corti.
- The hair cells are topped with stereocilia, arranged in a graded manner with shorter stereocilia on the outer rows and longer ones in the center.
- As the stereocilia bend in response to the pressure changes in the cochlear fluids, they open ion channels, leading to depolarization of the hair cells.
- Depolarization triggers an electrical signal, which is then transmitted via the cochlear nerve to the brain for processing.
- The base of the cochlea is sensitive to higher frequency sounds, while the apical portion detects lower frequencies.
- When sound vibrations reach the cochlea, they cause movement in the basilar membrane and hair cells within the Organ of Corti.
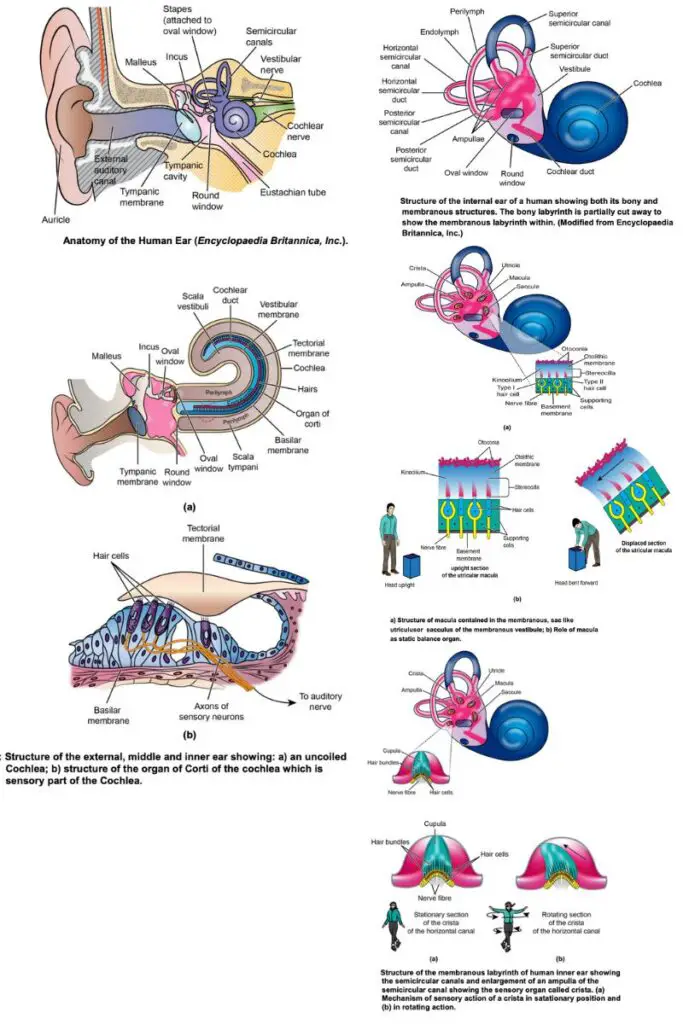
Vestibular Apparatus
The vestibular apparatus, located within the membranous labyrinth of the inner ear, plays a crucial role in maintaining balance and spatial orientation. This structure, filled with endolymph fluid, detects changes in head position and motion, transmitting information to the brain to regulate equilibrium. The vestibular apparatus consists of two main components: the utriculus and sacculus for detecting static balance, and the semicircular ducts for detecting motion or kinetic balance.
- Membranous Vestibular Apparatus
- The membranous vestibular apparatus is housed within the bony vestibular apparatus and consists of two sac-like chambers:
- The utriculus (upper sac), meaning “little bottle.”
- The sacculus (lower sac), meaning “little sac.”
- These two sacs are connected by a duct known as the sacculoutricular duct and filled with endolymph, a viscous fluid derived from cerebrospinal fluid.
- A narrow endolymphatic duct joins the sacculus or the sacculoutricular duct, allowing fluid exchange.
- The membranous vestibular apparatus is housed within the bony vestibular apparatus and consists of two sac-like chambers:
- Macula and Sensory Hair Cells
- Inside the utriculus and sacculus, a patch of sensory cells, called a macula, monitors the position of the head relative to gravity.
- Each macula consists of a neuroepithelium, a layer containing supporting cells, sensory hair cells, nerve fibers, and endings, all placed on a basement membrane.
- The sensory cells have two types of cilia:
- Stereocilia – stiff, non-motile, hair-like structures.
- Kinocilia – flexible, motile structures.
- These cilia project from the apical ends of the sensory cells, and the macula is covered by the otolithic membrane (also known as the statolithic membrane).
- The otolithic membrane is coated with otoconia or statoconia, which are calcium carbonate crystals. When the head changes position, these crystals move, stimulating the sensory hair cells to send nerve impulses to the brain, informing it of the change in position.
- Static Balance Organs: Utriculus and Sacculus
- The utriculus and sacculus are static balance organs, responsible for detecting the position of the head and body in relation to gravity.
- When the head moves, the otoconia shift over the hair cells of the macula, producing nerve impulses that are transmitted to the brain. This allows the brain to receive real-time information about the head’s position, ensuring balance and spatial orientation.
- Semicircular Ducts (Canals)
- The vestibular apparatus also includes three semicircular ducts, positioned at right angles to each other:
- Superior canal – vertically oriented.
- Horizontal canal – oriented horizontally.
- Posterior canal – vertically oriented but directed backward.
- Each canal is connected to the utriculus at both ends and has an expanded region called the ampulla.
- Inside the ampulla, a sense organ known as the crista detects motion (kinetic sense).
- The crista contains sensory cells with hair-like projections, embedded in a gelatinous mass called the cupula terminalis. Calcium carbonate crystals (otoconia) are also present, resting just above the sensory hairs.
- The vestibular apparatus also includes three semicircular ducts, positioned at right angles to each other:
- Function of Semicircular Canals in Motion Detection
- The semicircular canals are designed to respond to rotational movements rather than linear accelerations.
- As the head rotates, inertia prevents the fluid within the semicircular ducts from moving immediately. This creates a relative motion between the fluid and the cupula, causing the cupula to move in the opposite direction to the head’s motion.
- This movement stimulates the sensory hair cells within the crista, generating nerve impulses that are transmitted to the brain via branches of the auditory nerve. These signals allow the brain to detect rotational movements, helping maintain dynamic balance.
Comparative Anatomy of the Ear
The comparative anatomy of the ear across various vertebrates reveals distinct adaptations that reflect each group’s evolutionary needs and environmental interactions. All tetrapods (amphibians, reptiles, birds, and mammals) share some basic structures, such as the middle ear and tympanic membrane, but significant differences arise in external, middle, and inner ear anatomy. Each group displays specialized adaptations that allow for differing modes of sound detection and balance, corresponding to their ecological requirements and evolutionary history.
- Tetrapods
- All tetrapods possess a middle ear that includes a tympanic membrane, an important structure for transmitting sound waves from the external environment into the middle ear.
- Among reptiles, birds, and mammals, an external auditory meatus (ear canal) connects the tympanic membrane to the external surface of the head. However, fishes and amphibians lack this external structure.
- Mammals also have an external ear structure called the pinna, which aids in collecting and directing sound into the ear canal.
- Mammals
- Mammals exhibit a highly specialized external, middle, and inner ear structure, with the pinna varying greatly in shape and size across species.
- In some mammals, the pinna can be movable, allowing them to orient it toward a sound source.
- The middle ear of mammals is more complex than that of other tetrapods, containing three small bones, or ossicles: the malleus, incus, and stapes.
- Different mammals have unique auditory ranges; for example, humans can hear sound frequencies between 20 and 20,000 hertz, while whales and dolphins can detect ultrasonic sounds up to 150,000 hertz, and elephants can perceive infrasonic sounds as low as 10-14 hertz.
- The inner ear’s vestibular apparatus in mammals is similar to humans, aiding in balance, while the cochlea is notably elongated and coiled, except in monotremes, where it is less coiled.
- Birds and Reptiles
- The external ear appears for the first time in some reptiles, like lizards and crocodiles. It consists of a meatus (passage) that opens externally, leading to an internal tympanic membrane located at the end of the external auditory meatus.
- In both birds and reptiles, the middle ear consists of a single ossicle called the columella (or stapes in mammals), connected to the eardrum via a cartilaginous structure called the extracolumella and to the inner ear through the fenestra ovalis.
- The inner ears of birds and reptiles include sensory structures like the sacculus, utriculus, and lagena. The lagena is elongated and houses the basilar papilla, a structure that contains sensory hair cells for detecting sound. In reptiles, the basilar papilla is generally shorter than in birds, except in crocodiles, where the lagena is coiled like the mammalian cochlea.
- In birds, the basilar papilla has differentiated short and tall sensory hair cells, enhancing hearing sensitivity.
- While birds have highly sensitive hearing, snakes, lacking external and middle ear cavities, rely on sound vibrations traveling through the ground, which are transmitted from their lower jaw to the quadrate bone and then to the stapes.
- Amphibians
- Amphibians, like fishes, lack a true external ear, and their middle ear is often degenerate. Members of Urodela and Apoda groups lack a middle ear, leaving the inner ear to primarily maintain balance and posture.
- In anurans (frogs and toads), the middle ear contains a tympanic membrane that transmits sound vibrations to the inner ear via a columella bone. This adaptation allows amphibians to hear airborne sounds, a significant evolutionary development.
- The inner ear’s lagena in anurans has evolved to form a basilar papilla, though its structure differs from that found in birds and reptiles, both in its function and evolutionary origin.
- Fishes
- Unlike tetrapods, fishes do not possess an external or middle ear. However, they do have an inner ear with sensory chambers lined with hair cells that detect sound through vibrations. Each chamber contains an otolith (ear stone), and sound vibrations passing through the fish create differences in vibration between the dense otoliths and the fine hair cells, allowing them to perceive sound.
- In certain bony fishes, such as those in the Superorder Ostariophysia, a specialized structure called the Weberian apparatus connects the inner ear to the swim bladder, amplifying sound vibrations. This apparatus consists of small bones, called Weberian ossicles, that enhance the fish’s ability to detect sound.
- Cyclostomes (Jawless Fishes)
- Cyclostomes, including hagfish and lampreys, have a simplified ear structure with a reduced number of semicircular canals. Hagfish have a single canal, while lampreys possess two. These canals help maintain balance and spatial orientation, though their auditory capacity is limited compared to other vertebrates.
Olfactory organs of vertebrates
The olfactory organs of vertebrates play a crucial role in the detection of odors, a process known as olfaction. This ability is primarily facilitated by chemoreceptors, which are specialized cells responsive to chemical stimuli. Despite variations across species, the fundamental characteristics of the olfactory system remain consistent among vertebrates. In higher vertebrates, such as mammals, the olfactory system is well-defined with specific structures that enhance their capacity to identify and process smells. Conversely, lower vertebrates, including fish and amphibians, utilize chemosensory receptors located throughout their bodies to detect environmental chemicals.
- Olfactory System in Humans
- In vertebrates, including humans, the olfactory system comprises three main components:
- Olfactory Epithelium: This specialized epithelium is located within the nasal cavity. It contains:
- Basal cells
- Supporting sustentacular cells, which secrete mucus and support olfactory sensory cells (the actual chemoreceptors).
- Each sensory cell has a tuft of cilia at its apical end, embedded in mucus secreted by sustentacular cells. These sensory cells communicate directly with the olfactory bulb via their axons, which pass through the bony cribriform plates.
- Olfactory Bulb: This structure processes the incoming olfactory information.
- Olfactory Tract: This pathway transmits signals from the olfactory bulb to various brain regions.
- Olfactory Epithelium: This specialized epithelium is located within the nasal cavity. It contains:
- In vertebrates, including humans, the olfactory system comprises three main components:
- Comparative Olfactory System of Vertebrates
- Tetrapods: In tetrapods, each nasal passage is accessed via a small external opening, or naris. The back of the nasal passage opens into the mouth through an internal naris. The upper part contains the mucous-covered sensory epithelium, while the lower part serves as a respiratory passage.
- Mammals:
- The mammalian nose is complex, serving multiple functions, including olfaction, humidifying, warming, and filtering inhaled air.
- In humans, olfactory receptors are located high in the nasal cavity.
- Most mammals are macrosmatic, meaning they possess a strong sense of smell, characterized by a large area of olfactory mucous membrane.
- Certain mammals, such as seals, baleen whales, and most primates, are microsmatic, exhibiting a reduced sense of smell. In adult toothed whales, the olfactory region is practically suppressed, indicating a diminished olfactory capability.
- Birds:
- The avian olfactory system shares similarities with that of mammals, reptiles, and amphibians. Most birds have paired external nares located near the base of their upper mandible.
- Air enters through these nares, leading to a series of three internal nasal cavities:
- The first two chambers likely function to warm and moisten incoming air.
- The second chamber opens into the buccal cavity, allowing some air to reach the lungs directly.
- The third chamber contains the olfactory epithelium, which is histologically similar to that in mammals. Olfactory receptors in the epithelium connect, via paired olfactory nerves, with olfactory bulbs located at the brain’s rostral end.
- Reptiles:
- Each reptilian nasal organ consists of an external naris, a vestibule, a nasal cavity proper, a nasopharyngeal duct, and an internal naris, serving as air passages lined with nonsensory epithelium.
- The olfactory epithelium primarily occupies the roof and anterodorsal walls of the nasal cavity.
- The vestibule varies in length and curvature across species, and a concha, covered with sensory epithelium, projects into the nasal cavity.
- The vomeronasal organ in reptiles detects nonaerial, particulate odors that are transported to the vicinity of the duct by the tongue.
- Amphibians:
- In salamanders, the nasal organs consist of a large main cavity partially divided by a ventrolateral fold. Aquatic salamanders have simpler, smaller nasal cavities but larger vomeronasal organs.
- Frogs possess a complex nasal cavity with three chambers and a prominent vomeronasal organ.
- Caecilians exhibit simple nasal cavities similar to salamanders, but they have a distinct sensory tentacle that varies in size, position, and structure among species. This tentacle arises from a combination of nasal and orbital tissues and transports odor particles via the nasolacrimal duct to the vomeronasal organ.
- Fishes:
- Fish possess two blind-ended olfactory lateral pits, called nasal sacs, where olfactory receptors are embedded. As fish swim, water carrying chemicals flows in and out of these sacs.
- Vomeronasal Organ:
- Many vertebrates, including most mammals and reptiles (excluding humans), possess a dual olfactory system: a main olfactory system for volatile stimuli and an accessory olfactory system, or vomeronasal organ, located in the vomer bone between the nose and mouth.
- The vomeronasal organ contains sensory cells with microvilli projecting into its lumen. Its neural circuitry runs parallel to the olfactory neural system.
- This organ is absent in most turtles, crocodiles, birds, certain bats, primates, and aquatic mammals.
- In some amphibians, reptiles (especially snakes), and certain mammals, a distinct region of olfactory epithelium forms the vomeronasal epithelium, also known as Jacobson’s organ. Snakes utilize their vomeronasal organ to detect prey, extending their tongues to sample chemicals in the environment, a method also observed in snails.
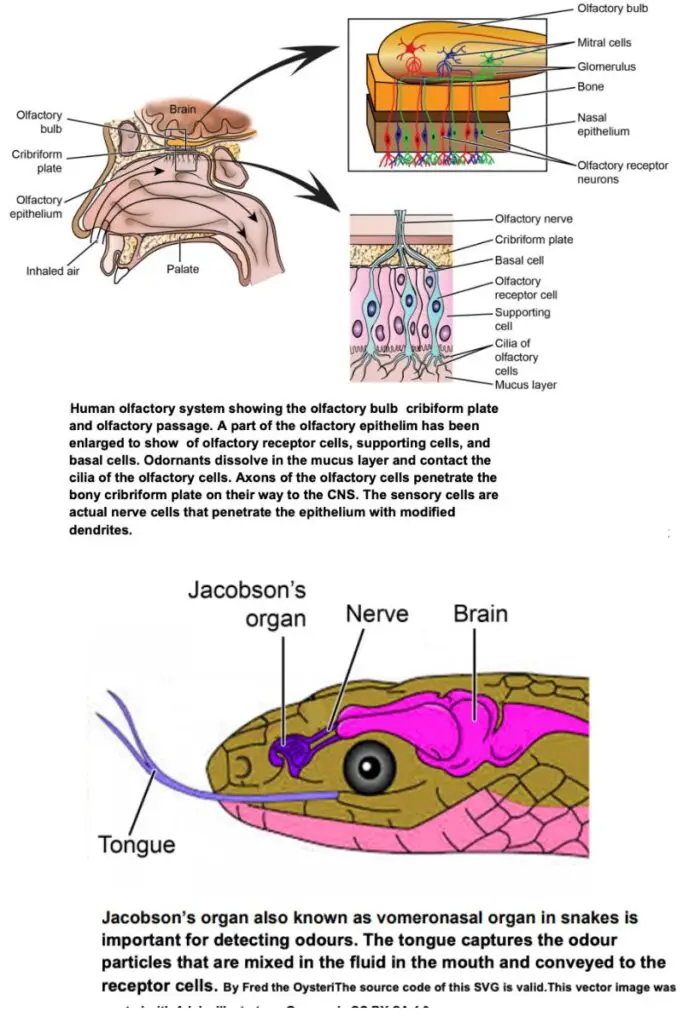
Specialised sensory organs
Specialized sensory organs play a crucial role in the survival of various organisms by enabling them to interact effectively with their environments. These sensory structures, often unique or complex, include electroreceptors, the lateral line system, pit organs in snakes, and systems for echolocation. This overview explores these specialized sensory systems, highlighting their functions and anatomical features.
- Lateral Line System in Fishes:
- The lateral line system (LLS) is a tactile sensory system found exclusively in aquatic vertebrates, including cyclostomes, fishes, and amphibians. It is absent in terrestrial vertebrates.
- The LLS detects movements, currents, vibrations, and pressure gradients in the surrounding water, allowing fish to gauge their own movement and the movements of nearby predators or prey.
- This system is visually identifiable as faint lines of pores extending along each side of a fish’s body from near the gill covers to the tail’s base. It consists of mechanoreceptors known as neuromasts or lateral line organs, which enable fish to sense mechanical changes in the water.
- Neuromasts are categorized into two types:
- Superficial or free-standing neuromasts: Located on the surface of the body.
- Canal neuromasts: Found beneath the skin within fluid-filled canals of the lateral line, forming an interconnected network along the head and body.
- In amphibians, the lateral line system is present only in larval forms and aquatic adults. Various fish species exhibit different canal structures; for instance, in Holocephali fishes, the canals are fully exposed, while in elasmobranchs and teleosts, they are closed and filled with mucus.
- Neuromasts consist of clusters of sensory and support cells encased in a jelly-like sheath called the cupula. These hair cells have cilia sensitive to water movement. The neuromasts send a continuous stream of bioelectric signals, altering the pulse rhythm upon stimulation.
- Disruption or removal of the lateral line system can cause disorientation and impair schooling behavior in fish.
- Electroreception in Fishes:
- Beyond detecting water currents, the neuromast system also responds to weak electrical potentials, aiding fish in orientation, predator avoidance, and schooling behavior.
- Most fish generate electric potentials through muscle contractions. Some species possess specialized electric organs that create a stronger electric field, allowing them to monitor disturbances caused by objects within this field.
- Sharks and rays have evolved neuromasts into electroreceptors known as the ampullae of Lorenzini, which can detect minute electrical signals from prey and Earth’s electromagnetic field, aiding in navigation and migration.
- Electric organs may also facilitate communication or self-defense among fishes. However, electroreceptors are ineffective in terrestrial animals due to poor electric conduction in air.
- Pit Organs in Snakes:
- The pit organs of certain snake families, such as Viperidae and Boidae, serve as specialized heat sensors. Morphologically resembling external neuromasts of fish, pit organs consist of epidermal pits housing free nerve endings responsive to infrared radiation, relaying information to the optic tectum of the midbrain.
- Apical pits are primarily located on the trunk, while labial pits are found in boa constrictors and pythons along the lips. In pit vipers (sub-family Crotalinae), loreal pits are situated between the external nares and the eye.
- Each pit has an inner and outer chamber separated by a membrane innervated by the trigeminal nerve, allowing snakes to detect temperature fluctuations as minor as 0.003°C, which is vital for locating warm-blooded prey.
- Echolocation in Aquatic and Aerial Mammals:
- Echolocation is a navigation technique employed by bats, dolphins, and other animals, enabling them to locate objects through reflected sound. This capability is crucial for navigation, hunting, and obstacle avoidance in low-visibility environments.
- Dolphins and whales emit high-pitched clicking sounds by forcing air through nasal passages near the blowhole, directing sound waves into the forehead’s fat structure known as the melon, which focuses them into a beam.
- The reflected sounds are detected through the lower jaw and conveyed to the ears. This method allows these animals to ascertain an object’s distance, direction, speed, density, and size.
- Among the 900 known bat species, over half rely on echolocation for navigation and food detection. These bats produce ultrasonic sound waves that are inaudible to humans. Echoes returned to the bats’ ears provide critical information about their environment.
- Bats utilize a combination of constant frequency (CF) and frequency-modulated (FM) calls, allowing them to detect objects based on varying echo arrival times and loudness levels. This acoustic information is processed by a specialized auditory cortex in their brains.
- Unique adaptations in bat anatomy enhance their echolocation abilities. For instance, the stapedius muscle in the middle ear contracts before sound emission to prevent deafness from the loud calls, and the external ear structure assists in receiving echoes effectively.
- https://www.notesonzoology.com/animals/nervous-system/receptors-and-sense-organs-in-animals-zoology/5611
- https://www.notesonzoology.com/vertebrates/receptor-organs-in-vertebrates-with-diagram-chordata-zoology/8668
- https://link.springer.com/chapter/10.1007/978-94-017-0619-3_6#:~:text=Practically%20all%20vertebrates%20have%20functional,broad%20classes%2C%20rods%20and%20cones.
- https://gcwgandhinagar.com/econtent/document/15878895601ZOOTC0201-%20Photoreceptors.pdf
- https://profiles.umassmed.edu/display/122398
- file:///Users/souravpan/Desktop/Unit%208%20Sense%20Organs.pdf