What is Reactive Oxygen Species (ROS)?
- Reactive oxygen species (ROS) are a group of highly reactive molecules derived from molecular oxygen (O₂), water, and hydrogen peroxide. Prominent members of the ROS family include hydroperoxide (H₂O₂), superoxide (O₂⁻), hydroxyl radicals (•OH), and singlet oxygen. Their ubiquity in biological systems is due to the abundance of oxygen, from which they are readily generated.
- ROS play multifaceted roles in biological systems, acting as both signaling molecules and intermediates in critical biochemical processes. They are integral to cell signaling pathways, influencing various cellular functions such as cell growth, differentiation, and apoptosis. For example, ROS are involved in the redox behavior of oxygen, which is pivotal in energy production within fuel cells. Additionally, ROS contribute to the photodegradation of organic pollutants, aiding in the environmental detoxification processes.
- Under normal physiological conditions, the concentration of ROS is tightly regulated to maintain cellular homeostasis. At controlled levels, ROS serve as important messengers that modulate signal transduction, gene expression, and overall cellular functions. However, when ROS levels exceed physiological thresholds, they can lead to oxidative stress, a condition that disrupts cellular homeostasis.
- Excessive ROS can inflict damage on essential cellular components, including nucleic acids, membrane lipids, and proteins. This oxidative damage has profound implications, triggering cell apoptosis and contributing to the aging process. Furthermore, ROS have been implicated in the development of various pathological conditions, including cancers, neurodegenerative diseases, diabetes, and inflammatory disorders.
- To mitigate the harmful effects of ROS, cells have evolved sophisticated antioxidant defense systems. The glutathione-ascorbate cycle is one of the most extensively studied mechanisms, facilitating the conversion of hydrogen peroxide (H₂O₂) into water (H₂O) by utilizing electron donors such as NADH and NADPH. In addition, enzymatic systems play a crucial role in ROS management. For instance, superoxide dismutase (SOD) catalyzes the dismutation of superoxide anions (O₂⁻) into molecular oxygen (O₂) or hydrogen peroxide (H₂O₂). Similarly, catalase is an enzyme that decomposes hydrogen peroxide into water and oxygen, thereby preventing oxidative damage.
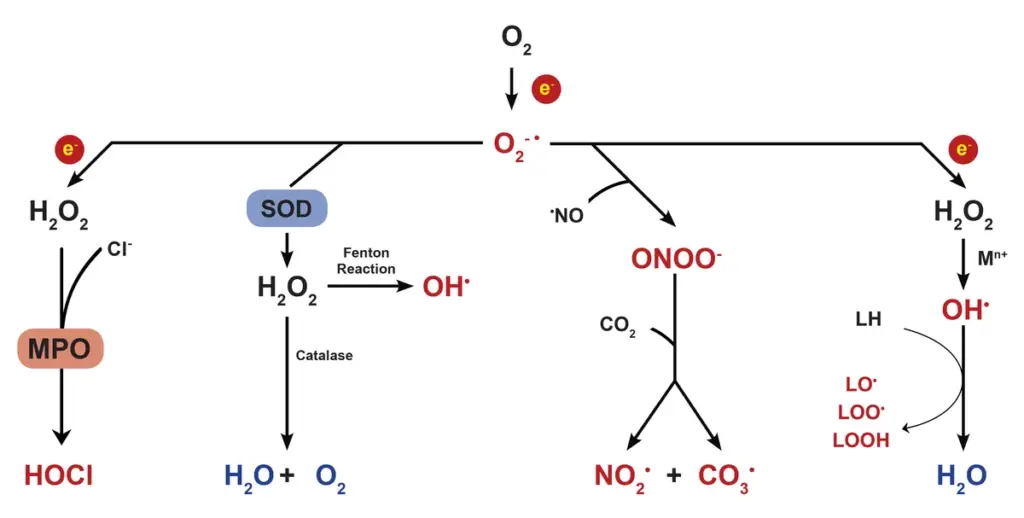
Types of reactive oxygen species (ROS)
Reactive oxygen species (ROS) encompass a diverse range of oxygen-containing molecules that exhibit significant chemical reactivity. These species are primarily generated as byproducts of cellular metabolism, particularly through mitochondrial oxidative phosphorylation, as well as through various enzymatic processes. Understanding the types of ROS is essential for comprehending their roles in biological systems. The following points delineate the major types of ROS, highlighting their formation and functions.
- Superoxide Anion (O₂⁻): This is one of the first products formed during the reduction of oxygen. It is generated through various enzymatic reactions, particularly by the enzyme NADPH oxidase during cellular respiration. The superoxide anion is a relatively stable free radical that can undergo further reactions to produce more reactive species. Its role in signaling is crucial, although it can also contribute to oxidative stress when in excess.
- Hydrogen Peroxide (H₂O₂): Formed from the dismutation of superoxide anions, hydrogen peroxide is less reactive than superoxide but can penetrate biological membranes, leading to oxidative damage. It serves as an important signaling molecule and is involved in various physiological processes, including cell proliferation and immune response. H₂O₂ can be further broken down into water and oxygen by enzymes like catalase.
- Hydroxyl Radical (•OH): This is one of the most reactive ROS, generated primarily from the Fenton reaction involving transition metals. Hydroxyl radicals can inflict severe damage on cellular components, including lipids, proteins, and DNA, contributing to cellular aging and the pathogenesis of various diseases.
- Hypochlorous Acid (HOCl): Produced by the enzyme myeloperoxidase in neutrophils during the immune response, hypochlorous acid is a powerful oxidizing agent. It plays a key role in the destruction of pathogens and is also involved in inflammatory processes. While effective for immune defense, its overproduction can lead to tissue damage.
- Peroxynitrite Anion (ONOO⁻): This ROS is formed by the reaction of superoxide with nitric oxide (NO). Peroxynitrite is a potent oxidant that can modify lipids, proteins, and DNA, contributing to cellular dysfunction and inflammation. Its formation is associated with various pathological conditions, including neurodegenerative diseases.
- Nitric Oxide (NO): While primarily a signaling molecule, nitric oxide can also contribute to the formation of reactive nitrogen species. NO plays critical roles in vascular regulation, neurotransmission, and immune responses. However, when produced in excess, it can lead to nitrosative stress, similar to oxidative stress.
Chemistry of reactive oxygen species (ROS)
Reactive oxygen species (ROS) are highly reactive molecules that play significant roles in various biochemical processes, including signaling pathways and oxidative stress responses. Their reactivity is largely derived from their electron configuration, particularly the unique properties of oxygen in different spin states. Understanding the chemistry of ROS is essential for appreciating their biological implications and potential roles in health and disease.
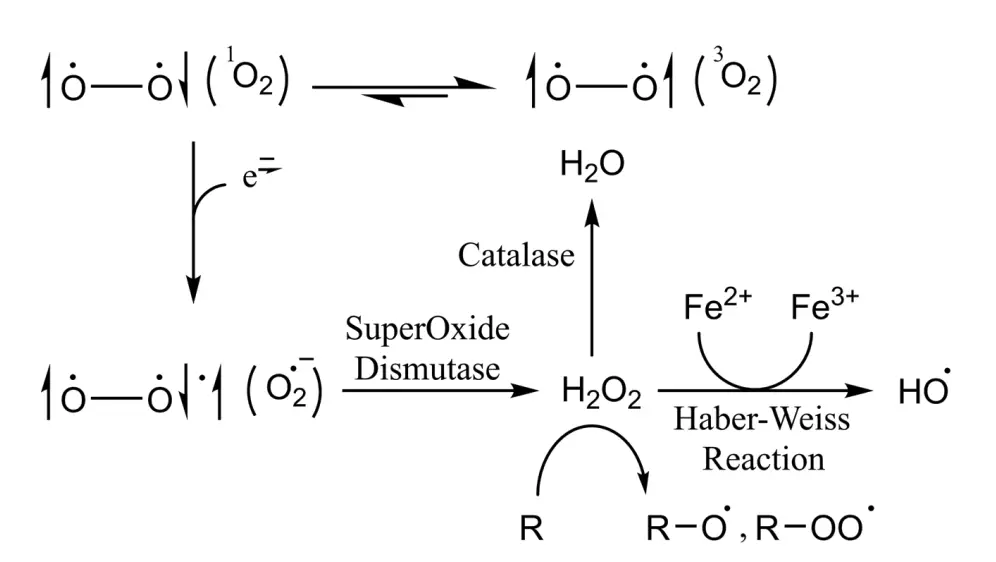
- Electron Spin States in Molecular Chemistry
- All known bi-atomic molecules, except for oxygen (O2), exhibit electrons spinning in an antiparallel manner. This configuration allows electrons from two atoms to share a single orbital, forming a stable covalent bond.
- Antiparallel spinning minimizes energy according to the Pauli exclusion principle, resulting in a total spin energy of zero in the orbital.
- In contrast, biradical molecules with parallel electron spins cannot dissipate energy by pairing in an orbital. Each electron remains as a radical, contributing to a total spin of one (2×(1/2)).
- The triplet state, characterized by unpaired electrons, is generally more energetic than the singlet state. However, oxygen exhibits a unique stability in its unpaired electron state.
- Reactivity of Oxygen and Formation of ROS
- The triplet state of molecular oxygen (3O2) is more stable than the singlet state (1O2), which is essential for understanding the oxidative properties of ROS.
- In its unpaired biradical form, oxygen is relatively stable; however, its reactivity increases when one of the antiparallel electrons pairs with an external electron. This process can generate superoxide radicals (O·-2).
- Superoxide radicals are characterized by their inability to easily cross cellular membranes, rendering them brief and localized in action. However, they can be converted into hydrogen peroxide (H2O2) by the enzyme superoxide dismutase (SOD), which is more stable and membrane-permeable.
- Pathways for ROS Generation
- Superoxide radicals can engage in reactions with metal ions in the body, particularly through the Haber–Weiss reaction. This process utilizes iron catalysis to produce highly reactive hydroxyl radicals (OH·) from superoxide and H2O2.
- Other relevant ROS can arise from peroxides, including alkoxy radicals (RO·) and peroxyl radicals (ROO·). The short lifespan of hydroxyl radicals, typically less than 1 nanosecond, limits the cell’s ability to defend against them, emphasizing the importance of preventing their formation in the first place.
- Nitric Oxide as a Reactive Nitrogen Species
- Nitric oxide (NO·) is a critical second messenger in cellular signaling and is produced through poorly understood mechanisms involving nitrogen-containing compounds like amino acids.
- The same biochemical pathways that generate nitric oxide can also produce reactive nitrogen species, including peroxynitrite (ONOO−) and nitrogen dioxide (NO2). These reactive nitrogen species can interact with various cellular components, influencing signaling pathways and potentially contributing to oxidative stress.
- Regulation of ROS Levels
- The endoplasmic reticulum (ER) plays a pivotal role in regulating the redox environment within cells, thereby influencing the levels of generated ROS.
- Changes in the redox status of the ER can modulate redox signaling pathways that affect protein folding, maturation, and overall cellular function. This interplay between ROS levels and the redox environment underscores the importance of maintaining a balance between oxidative and reductive processes within cells.
Cellular defence against ROS
Cellular defense against reactive oxygen species (ROS) is essential for maintaining cellular integrity and function, especially in aerobic organisms. The production of ROS during metabolic processes necessitates the evolution of various mechanisms to counteract their potential harmful effects. When ROS levels exceed the capacity of cellular defenses, oxidative stress occurs, leading to cellular damage and contributing to numerous diseases. The following points outline the key mechanisms and components involved in the cellular defense against ROS.
- Superoxide Dismutase (SOD): This enzyme plays a critical role in the detoxification of superoxide anions (O₂⁻). It catalyzes the conversion of two superoxide radicals into hydrogen peroxide (H₂O₂) and molecular oxygen (O₂), thus initiating the removal of reactive species. This reaction is essential for reducing the levels of superoxide, which can cause significant cellular damage if left unchecked.
- Catalase: Located in peroxisomes, catalase complements the action of SOD by converting hydrogen peroxide into water and oxygen. This enzyme effectively neutralizes H₂O₂, thereby preventing its accumulation and mitigating oxidative stress. The detoxification process is vital for maintaining a balanced redox state within cells.
- Glutathione Peroxidase: This group of selenium-containing enzymes further degrades hydrogen peroxide and organic peroxides, converting them into alcohols. Glutathione peroxidase utilizes glutathione (GSH) as a substrate, linking the detoxification of ROS to the cellular antioxidant system.
- Glutathione: Often regarded as one of the most important intracellular antioxidants, glutathione is a tripeptide composed of glutamate, cysteine, and glycine. It contains a reactive sulfhydryl group that readily interacts with ROS, facilitating their detoxification. When glutathione is oxidized during these reactions, it can be regenerated to its reduced form through the action of NADPH-dependent reductases, thus maintaining its availability for ongoing antioxidant defense.
- Nonenzymatic Antioxidants: In addition to enzymatic defenses, several small molecules also contribute to the detoxification of ROS. Vitamin C (ascorbic acid) is a water-soluble antioxidant that can donate electrons to reduce reactive species, while vitamin E (α-tocopherol) serves a similar function in lipid membranes due to its lipid-soluble nature. These vitamins play crucial roles in protecting cellular components from oxidative damage.
- Oxidized vs. Reduced Glutathione Ratio: The dynamic balance between oxidized glutathione (GSSG) and reduced glutathione (GSH) serves as a crucial indicator of oxidative stress within the cell. A high ratio of GSSG to GSH suggests an increased level of oxidative stress, signaling the need for enhanced antioxidant responses.
Physiological Roles of ROS
The following points outline the key physiological roles of ROS, illustrating their dual nature as both signaling molecules and potential disruptors of homeostasis.
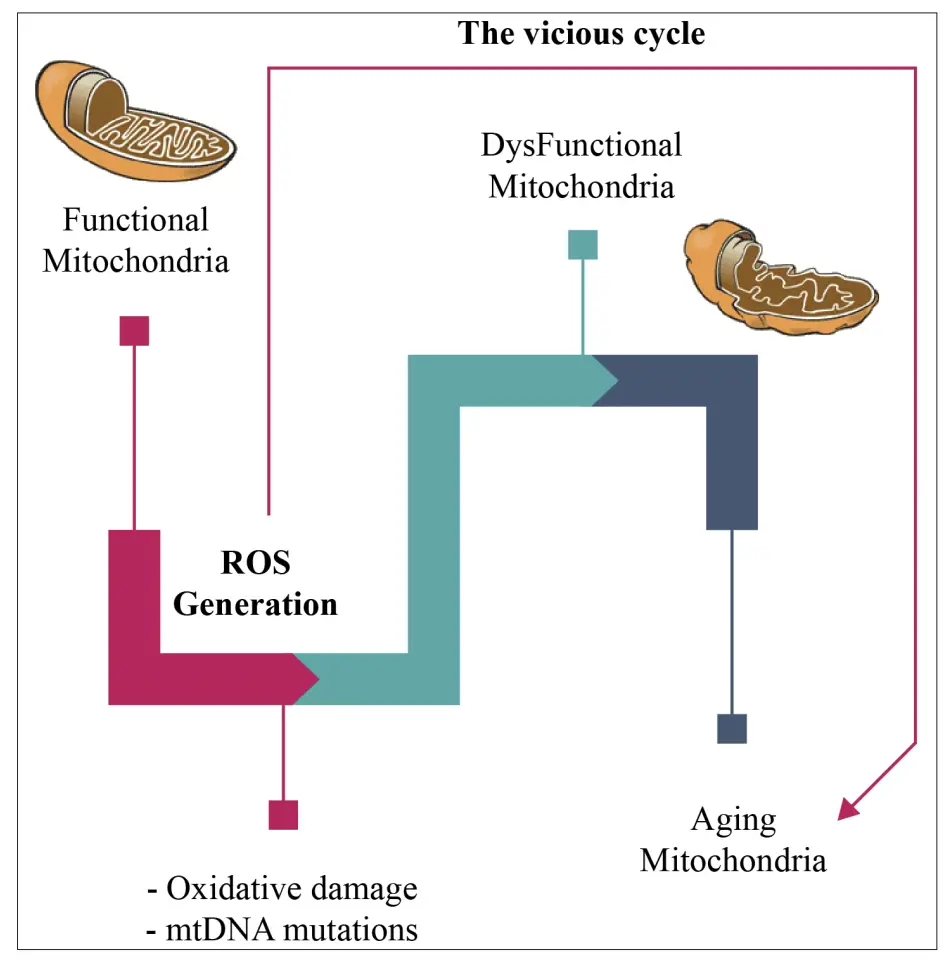
- Regulation of Aging: Aging is a multifaceted process characterized by the gradual deterioration of cellular and organismal functions. While the free radical theory of aging, proposed by Denham Harman in the 1950s, suggests that oxidative damage from ROS accumulates over time, leading to cellular dysfunction, more recent findings indicate that ROS play a more nuanced role. They modulate key signaling pathways, such as the “target of rapamycin” (TOR) and “AMP-activated kinase” (AMPK), which are critical in cellular responses to nutrients and energy status. ROS can influence the expression and activation of these pathways, thereby affecting the aging process and cellular stress responses.
- Enhancement of Immune Responses: The immune system relies heavily on ROS for effective defense against pathogens. Activated phagocytes produce ROS during an oxidative burst to destroy engulfed bacteria. This oxidative burst is part of the innate immune response, where superoxide and hydrogen peroxide are generated to kill microorganisms. Furthermore, ROS play a role in the activation of T lymphocytes, enhancing the adaptive immune response. They facilitate the amplification of signaling pathways that lead to the activation of immune cells, including macrophages, which respond to pathogens by varying their secretion of cytokines based on their intracellular redox state. Thus, ROS are essential for both innate and adaptive immunity.
- Cognitive Function: In the central nervous system (CNS), ROS have traditionally been viewed as detrimental due to their association with neurodegenerative diseases. However, recent studies suggest that mitochondrial ROS (mROS) serve as important signaling molecules that regulate neuronal function. For example, mROS can strengthen inhibitory synapses by coupling cellular metabolism to the activity of GABA receptors, essential for maintaining excitatory and inhibitory balance in the brain. This implies that ROS may play a role in facilitating synaptic plasticity and communication within neural networks.
- Role in Fertility: In the context of reproduction, ROS are produced by both sperm cells and leukocytes in semen. They are crucial for several aspects of sperm function, including chromatin condensation and capacitation, which are necessary for successful fertilization. Controlled levels of ROS stimulate processes that enhance sperm motility and facilitate the acrosome reaction, enabling sperm to penetrate the oocyte. However, excessive ROS production can overwhelm antioxidant defenses, leading to oxidative stress, DNA damage in sperm, and decreased fertility.
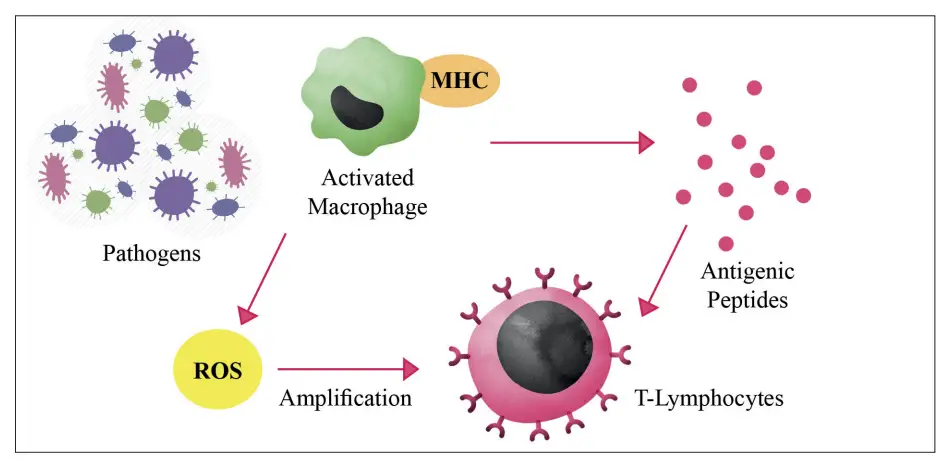
Pathological Roles of Reactive Oxygen Species (ROS)
Reactive oxygen species (ROS) play complex roles in biological systems, acting as both signaling molecules and damaging agents. Their pathological roles, particularly in infertility and cancer, underscore their dual nature in health and disease. This discussion delves into how ROS contribute to these conditions, highlighting their mechanisms and effects.
- ROS and Infertility
- Infertility affects up to 20% of couples globally, with male factors implicated in approximately half of these cases. The World Health Organization (WHO) defines infertility as the inability to achieve pregnancy after 12 months of regular sexual activity.
- Healthy semen parameters established by WHO include volume, pH, total sperm number, concentration, motility, and morphology.
- Oxidative stress (OS) is a major contributor to male infertility, with 30% to 40% of infertile men in the U.S. exhibiting elevated ROS levels in their seminal plasma.
- Endogenous sources of ROS in seminal plasma primarily include leukocytes (neutrophils and macrophages) and immature spermatozoa, while exogenous factors include excessive alcohol intake, smoking, environmental toxins, and radiation exposure.
- Infection and inflammation can cause peroxidase-positive leukocytes to produce ROS levels up to 100 times higher than normal. The presence of over 1 million peroxidase-positive cells per milliliter of semen is classified as leukocytospermia, significantly elevating ROS levels and damaging sperm.
- Spermatozoa are particularly vulnerable to ROS due to their high membrane content of polyunsaturated fatty acids (PUFAs), which, when oxidized, initiate lipid peroxidation (LPO). This process depletes ATP, damages axonemes, reduces sperm viability, and results in increased midpiece defects, ultimately impairing sperm motility.
- The mechanisms of ROS generation in sperm include the NADPH oxidase system at the plasma membrane and the mitochondria through NAD-dependent oxidoreductase reactions, primarily producing superoxide anions.
- Elevated ROS levels correlate with varicocele grades, a condition characterized by abnormal dilation of veins in the spermatic cord, found in about 40% of infertile males.
- Exogenous sources of ROS, such as radiation and exposure to heavy metals, contribute to reduced sperm quality and viability, while smoking significantly alters the balance between ROS and antioxidants in semen.
- The oxidative damage extends to sperm DNA, where incomplete chromatin compaction can lead to mutations and hinder fertilization potential. Although some DNA damage can be repaired, poor repair mechanisms in oocytes may prevent embryo development.
- High ROS levels in female reproductive systems are also implicated in infertility, with studies showing correlations between ROS in peritoneal fluid and idiopathic infertility. Notably, lower ROS levels in follicular fluid are associated with successful in vitro fertilization (IVF) outcomes.
- ROS have been linked to pregnancy complications, including spontaneous abortion and preeclampsia, highlighting the need for understanding ROS’s role in both male and female reproductive health.
- ROS in Cancer
- ROS are known to participate in cellular signal transduction through oxidation-reduction reactions, altering protein structure and function to elicit cellular responses.
- In cancer cells, increased mitochondrial metabolism results in elevated ROS concentrations, which further promote tumorigenesis and increase mutation rates, contributing to metastasis.
- Early studies indicated that overexpression of superoxide dismutase (SOD) in murine sarcoma cells provided protection against radiation but not against chemical transformation, emphasizing the complex relationship between ROS and tumor progression.
- Genetic modifications, such as the downregulation of the tumor suppressor p53, lead to increased ROS production, further contributing to cancer development. Studies show that antioxidants can sometimes exacerbate tumor growth, demonstrating the delicate balance of ROS in cancer biology.
- The Forkhead box O (FoxO) protein family plays a crucial role in regulating the intracellular redox environment, promoting antioxidant enzyme expression to counteract ROS effects. Genetic alterations in genes like Brca1 have been linked to increased ROS levels and higher rates of mammary tumors.
- While ROS promote cancer cell survival, angiogenesis, and proliferation, studies have also revealed that antioxidant supplementation, such as N-acetylcysteine (NAC) and vitamins A and E, can inadvertently accelerate tumor progression and mortality.
- Clinical trials have shown that antioxidant supplementation does not prevent cancer occurrence and may worsen outcomes in specific cancer types. This suggests that while ROS can promote tumorigenesis, antioxidants may provide cancer cells with a survival advantage under certain conditions.
- Interestingly, cancer cells that metastasize may undergo reversible metabolic changes, enhancing their resistance to oxidative stress, indicating that a nuanced understanding of ROS’s role in cancer is critical for developing therapeutic strategies.
- Jakubczyk, Karolina & Dec, Karolina & Antoniewicz, Justyna & Kawczuga, Dorota & Kochman, Joanna & Janda-Milczarek, Katarzyna. (2020). Reactive oxygen species – sources, functions, oxidative damage. Polski merkuriusz lekarski: organ Polskiego Towarzystwa Lekarskiego. XLVIII. 124-127.
- Sullivan, Lucas & Chandel, Navdeep. (2014). Mitochondrial reactive oxygen species and cancer. Cancer & metabolism. 2. 17. 10.1186/2049-3002-2-17.
- Bardaweel SK, Gul M, Alzweiri M, Ishaqat A, ALSalamat HA, Bashatwah RM. Reactive Oxygen Species: the Dual Role in Physiological and Pathological Conditions of the Human Body. Eurasian J Med. 2018 Oct;50(3):193-201. doi: 10.5152/eurasianjmed.2018.17397. PMID: 30515042; PMCID: PMC6263229.
- Ray PD, Huang BW, Tsuji Y. Reactive oxygen species (ROS) homeostasis and redox regulation in cellular signaling. Cell Signal. 2012 May;24(5):981-90. doi: 10.1016/j.cellsig.2012.01.008. Epub 2012 Jan 20. PMID: 22286106; PMCID: PMC3454471.
- https://www.cancer.gov/publications/dictionaries/cancer-terms/def/reactive-oxygen-species
- https://wou.edu/chemistry/files/2020/03/Reactive-Oxygen-Species.pdf
- https://www.agilent.com/cs/library/whitepaper/public/ROS%20White%20Paper_2015_5994-3454EN.pdf
- https://www.eajm.org/content/files/sayilar/210/buyuk/193-201.pdf
- https://www.bbau.ac.in/dept/dem/TM/Lecture%201.pdf