Pyrimidines and Pyrimidine Nucleotides
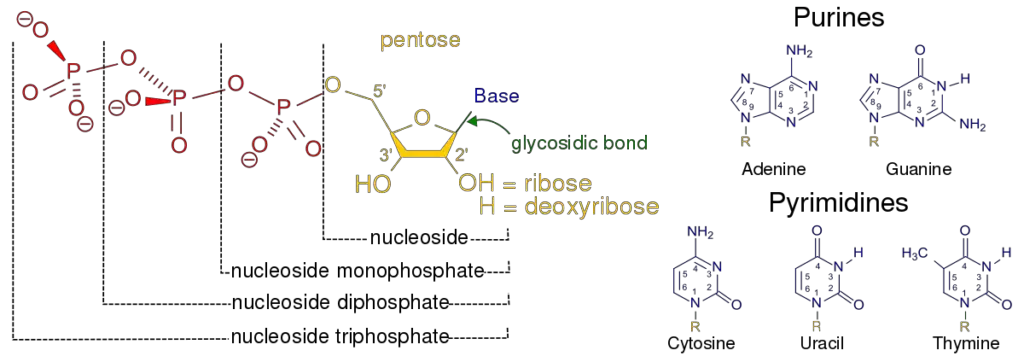
Structure of Pyrimidines and Pyrimidine Nucleotides
- Cytosine, thymine, uracil, and orotate are the most common pyrimidines in nucleotides.
- In comparison to the typical pyrimidine ring structure, cytosine has oxo (keto) and amino groups at positions 2 and 4, while orotate has oxo groups at positions 2 and 4 and a carboxyl group at position 6, and uracil has oxo groups at positions 2 and 4.
- Thymine is the same as adding a methyl group to the fifth spot of uracil.
- All of the pyrimidine nucleotides have an N-glycosidic linkage between the N1 of the base and the C1′ of the sugar. This linkage can only take on a small number of different shapes.
- For pyrimidine nucleosides and nucleotides, the phosphate ester bond is most stable in the synclinical (+sc) shape.
Occurrence of Pyrimidines and Pyrimidine Nucleotides
- Most pyrimidine nucleotides in a cell are in their polymeric form because they are part of DNA and RNA molecules.
- Both DNA and RNA have cytosine, but uracil is usually only found in RNA and thymine is usually only found in DNA. (However, thymine can sometimes be found in certain RNA molecules, and uracil can form in DNA when cytosine is broken down.)
- The other pyrimidine nucleotides are made by making orotate first.
- Because certain bases pair up (Thy with Ade and Cyt with Gua), 50% of double-stranded DNA is made up of pyrimidine nucleotides.
- Within a single species, the number of cytosines to thymines is always the same. However, this number changes between species.
- This is especially clear in species that live in harsh conditions, like high temperatures, where CG base pairs are used a lot to make the DNA molecule more stable.
Properties of Pyrimidines and Pyrimidine Nucleotides
- Pyrimidine nucleotides help make DNA and RNA, which are molecules that store and process genetic information. This is their main job in all cells.
- But in some biosynthetic reactions, pyrimidine nucleotides are also used as activated intermediates. For instance, UDP-glucose and CDP-diacylglycerol are precursors of glycogen and phosphoglycerides, respectively.
Biosynthesis of Pyrimidine
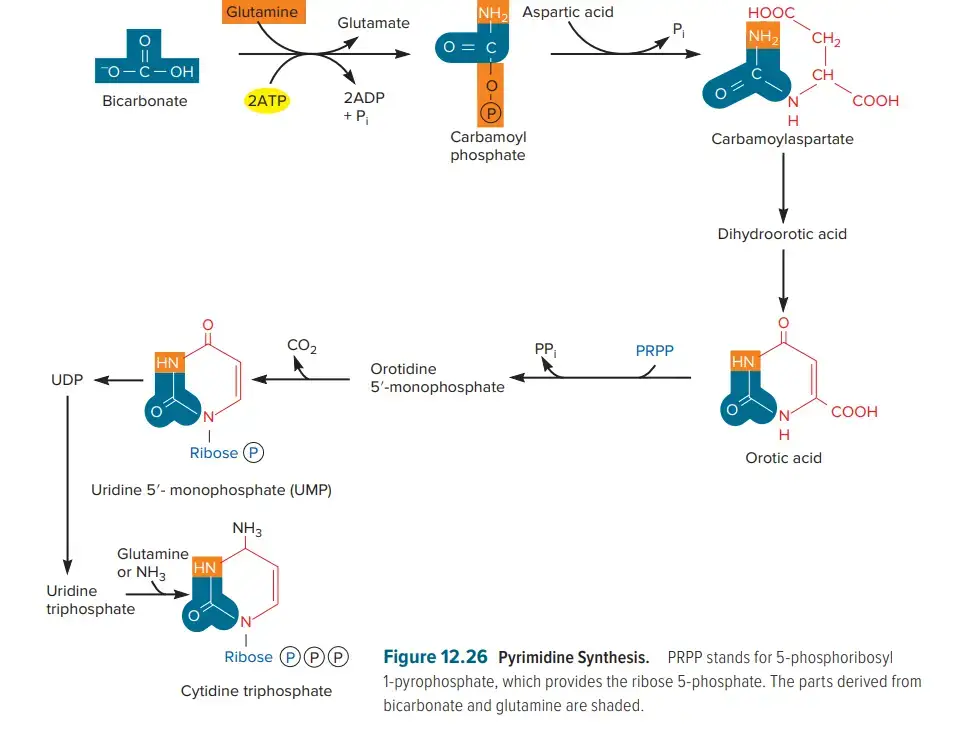
- Purine synthesis is just as difficult as pyrimidine biosynthesis. Nevertheless, the two processes differ significantly: Purines are formed by adding chemical moieties to ribose 5-phosphate and never exist as free bases; pyrimidine rings are constructed prior to the attachment of ribose.
- Biosynthesis of pyrimidine begins with aspartic acid and carbamoyl phosphate, a high-energy compound generated from glutamine-derived bicarbonate and ammonia.
- Aspartate carbamoyltransferase catalyses the condensation (joining) of these two substrates to produce carbamoyl aspartate, which is then transformed to orotic acid, the initial pyrimidine product.
- 5-phosphoribosyl 1-pyrophosphate (PRPP) is a high-energy molecule that supplies the ribose 5-phosphate that is linked to orotic acid to form a nucleotide.
- Orotidine monophosphate is transformed into uridine monophosphate, uridine triphosphate, and cytidine triphosphate in a sequential manner.
- These ribonucleotides undergo the same reduction to deoxy forms as purine ribonucleotides.
- This leaves the cell with one remaining deoxynucleotide: deoxythimidine monophosphate. It is produced by methylating deoxyuridine monophosphate with a folic acid derivative.
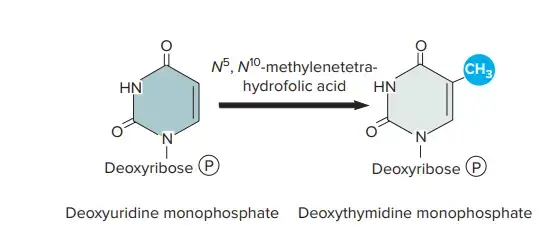
Pyrimidine Nucleotide Examples
Cytosine
- The other type of nucleotide is made up of pyrimidines.
- Cytosine is a pyrimidine nucleotide, and it is made up of only one ring.
- In both DNA and RNA, cytosine bonds to guanine.
- They work well together because of the nucleotide guanine.
Thymine
- Thymine is a pyrimidine nucleotide, like cytosine, and has one ring.
- It sticks to the DNA adenine.
- RNA does not have thymine.
- Only two hydrogen bonds connect it to adenine in DNA, making them the weaker pair.
Uracil
- Pyrimidines also include uracil.
- During the process of turning DNA into RNA, uracil goes where thymine would usually go.
- We don’t fully understand why this is, but we do know that uracil has both good and bad points.
- Most living things don’t use uracil in their DNA because it doesn’t last long and can break down into cytosine.
- But because RNA is also a short-lived molecule, uracil is the preferred nucleotide.
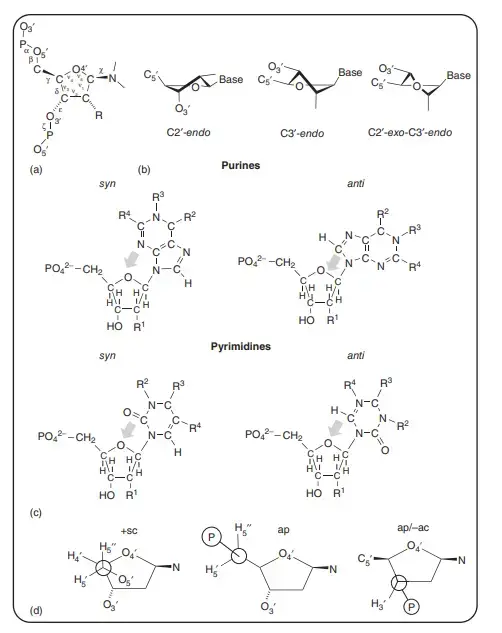
Purines and Purine Nucleotides
Structure of Purines and Purine Nucleotides
- In Table 1, the names of four common purine nucleotides used in cellular metabolism are given.
- In addition to the basic purine ring structure, the bases have the following extra groups: an amino group at position 6 for adenine, oxo and amino groups at positions 6 and 2 for guanine, an oxo group at position 6 for hypoxanthine, and oxo groups at positions 2 and 6 for xanthine.
- In contrast to pyrimidines, purines have a wide range of conformations at their N-glycosidic bond, with most nucleotides being in the anti conformation.
- Notably, purines can also form a syn N-glycosidic bond, which can be seen in some nucleic acid sequences that form a specific DNA secondary structure called Z-DNA.
- For purine nucleosides, the preferred shape of the phosphate ester bond is either +sc or ap.
- But in nucleotides, the 5′-phosphate limits the number of possible shapes, and the +sc shape is the most common one.
- For different parts of cellular metabolism, different kinds of purine nucleotides are made. For example, 3′,5′-cyclic AMP (cAMP) is made when the 3′-hydroxyl group of the ribose ring moves away from the phosphorus atom at the centre of ATP.
- There are several types of coenzymes that contain adenine nucleotides: nicotinamide–adenine dinucleotides (NAD+, NADH, NADP+, NADPH) have a modified version of the vitamin nicotinate attached to a ribose that is bound to AMP; flavin–adenine dinucleotide (FAD) has AMP attached to the coenzyme riboflavin 5′-phosphate; coenzyme A (CoA) is made up of AMP
Occurrence of Purines and Purine Nucleotides
- Nucleotides with adenine and guanine are used in many ways in the biochemistry of cells. Nucleotides with hypoxanthine and xanthine are made when adenylate and guanylate are made.
- Like the pyrimidine nucleotides, many of the major purine nucleotides are found in DNA and RNA polymers.
- Unlike pyrimidine nucleotides, ribo and deoxyribo versions of the same nucleotides are used.
- Another difference between pyrimidine nucleotides and purine ribonucleotides is that cells have a lot of purine ribonucleotides because they are used in many energy-intensive processes.
- Modified versions of purine nucleotides, like cAMP, different forms of NAD+ and FAD, and coenzyme A, are also found in all living things.
- The amounts of these nucleotides can vary a lot. For example, cyclic nucleotides are pretty stable, but cAMP has a short half-life inside cells because enzymes break it down quickly.
Properties of Purines and Purine Nucleotides
- Purine-based nucleotides play different roles in DNA and RNA, but perhaps the most important thing about them is that many enzymes use them as a source of energy.
- GTP gives macromolecules the energy they need to move, such as when nascent peptide chains on ribosomes move or when signal-coupling proteins are turned on.
- ATP is the universal currency of energy in biological systems. This is because it has a high potential for phosphate transfer due to the instability caused by the electrostatic repulsion of phosphate moieties in the pyrophosphate.
- The products of hydrolysis are more stable than ATP, but this depends a lot on pH and Mg2+ concentration.
- In addition to playing a big role in the energy of cells, nucleotides that contain adenine can take on a number of very different biochemical roles. cAMP is a “second messenger” that controls and mediates the actions of peptide hormones; bacterial cells use guanosine polyphosphates (such as ppGpp and pppGpp) to link growth rate to nutrient availability; puromycin is a powerful inhibitor of protein biosynthesis; arabino or 8-aza derivatives of adenosine have antibiotic properties; different forms of NAD+ and FAD are also used in a variety of oxid
Purine Nucleotides Examples
Adenine
- Adenine is a purine, which is a nitrogenous base from one of two families.
- Purines are made up of two linked rings. Adenine bonds with thymine in DNA. In RNA, uracil bonds to adenine.
- As we’ve already talked about, adenine is used as a base in adenosine triphosphate. Three phosphate groups can be added from there.
- This means that the bonds can store a lot of energy.
- The bonds in ATP are strong for the same reason that the sugar-phosphate backbone is strong.
- It can be transferred to other reactions and molecules when it is mixed with special enzymes that have formed to release the energy.
Guanine
- Guanine is also a purine nucleotide, like adenine. It has two rings, just like adenine.
- It sticks to both DNA and RNA cytosine. As you can see in the picture above, there are three hydrogen bonds between guanine and cytosine.
- This makes the bond between cytosine and guanine a little bit stronger than the bond between thymine and adenine, which only forms two hydrogen bonds.
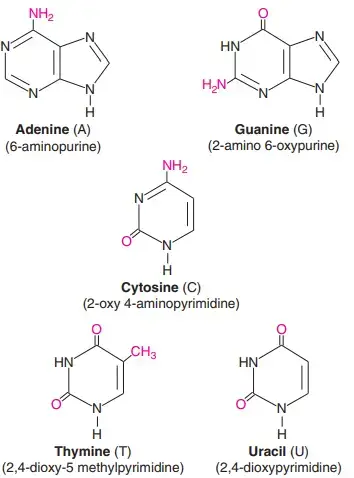
Biosynthesis of Purine
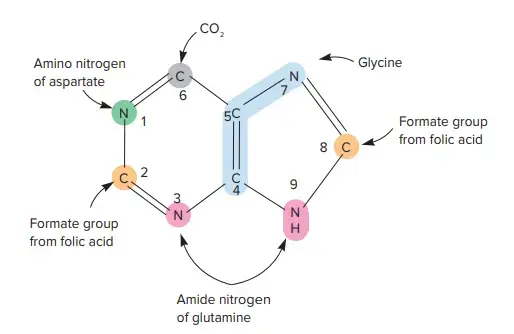
- The biosynthetic pathway for purines is a complex, 11-step procedure in which seven separate molecules contribute to the final skeleton of purines.
- The sugar ribose 5-phosphate is used to form the purine skeleton at the beginning of the process. Therefore, the initial purine product of the route is not a free purine base but rather the nucleotide inosinic acid.
- Folic acid is a crucial cofactor for purine biosynthesis, as folic acid derivatives contribute two carbons to the purine skeleton.
- After the formation of inosinic acid, relatively short pathways produce adenosine monophosphate and guanosine monophosphate, as well as nucleoside diphosphates and triphosphates via phosphate transfers from ATP.
- At this phase, ribonucleotides have been produced by the cell. However, deoxynucleotides are required for DNA synthesis.
- On carbon two of the sugar in deoxyribonucleotides, a H atom rather than a hydroxyl group is present. This carbon must be reduced to turn a ribonucleotide into a deoxyribonucleotide.
- Two distinct pathways result in the formation of deoxyribonucleotides from the reduction of nucleoside diphosphates or nucleoside triphosphates.
- Certain microbes degrade ribose in nucleoside triphosphates using a method that requires vitamin B12.
- Others decrease the amount of ribose in nucleoside diphosphates. As their reducing agent, both systems use a tiny sulfur-containing protein called thioredoxin.
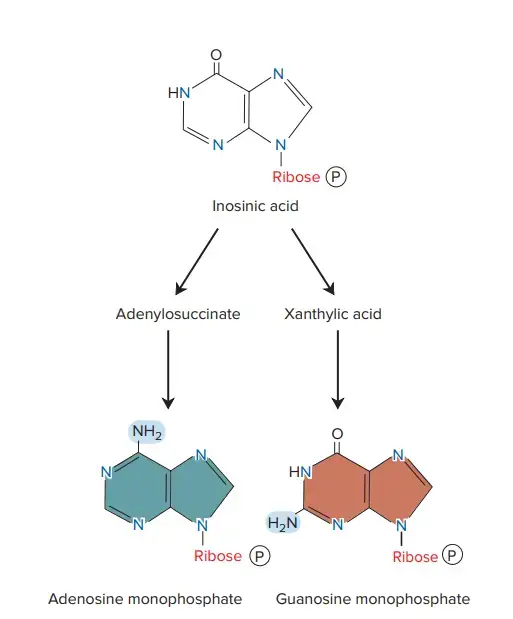