What is Nitrogen Cycle?
- The nitrogen cycle is a fundamental biogeochemical process that facilitates the conversion of atmospheric nitrogen, which is chemically inert and inaccessible to most living organisms, into forms that can be readily assimilated. This cycle plays a pivotal role in the ecosystem, ensuring the availability of essential nitrogen compounds for various biological functions.
- Atmospheric nitrogen (N2), which constitutes approximately 78% of Earth’s atmosphere, is the primary reservoir of nitrogen. However, this form of nitrogen is not directly usable by most plants and animals. To bridge this gap, a series of interconnected processes within the nitrogen cycle transform this inert gas into biologically available compounds.
- The first step in this cycle is nitrogen fixation. Specialized bacteria, known as nitrogen-fixing bacteria, have the unique ability to convert atmospheric nitrogen into ammonia (NH3) or ammonium ions (NH4+). This process provides plants with an initial source of usable nitrogen.
- Following fixation, ammonification occurs. During this phase, decomposing bacteria break down organic matter, releasing ammonium ions back into the soil. This ammonium then undergoes nitrification, a two-step bacterial process. Initially, ammonium is converted into nitrite (NO2-), and subsequently, into nitrate (NO3-), a compound that plants can efficiently absorb and utilize.
- Once absorbed by plants, nitrogen compounds are incorporated into amino acids and proteins. As plants are consumed by herbivores, and herbivores by carnivores, these essential nitrogenous compounds are transferred along the food chain, ensuring nitrogen availability to higher trophic levels.
- The final step in the nitrogen cycle is denitrification. In this process, specific bacteria in oxygen-deprived environments transform nitrates back into gaseous nitrogen, releasing it into the atmosphere and completing the cycle.
- It’s crucial to note that human activities, such as the combustion of fossil fuels, extensive use of nitrogen-based fertilizers, and wastewater discharge, have significantly impacted the natural balance of the nitrogen cycle. Such alterations can have detrimental effects on both the environment and human health.
- In conclusion, the nitrogen cycle is a sophisticated and vital process that ensures the continuous availability of an essential element for life on Earth. Through a series of biological and physical transformations, atmospheric nitrogen is converted into forms that can be assimilated by living organisms, supporting biodiversity and ecosystem functionality.
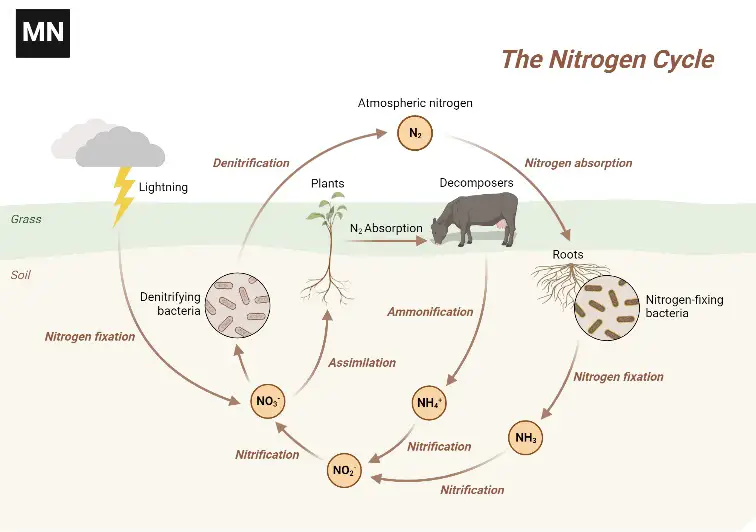
Nitrogen Cycle Definition
The nitrogen cycle is a biogeochemical process that transforms atmospheric nitrogen into usable forms for living organisms and then returns it to the atmosphere through a series of biological and chemical reactions.
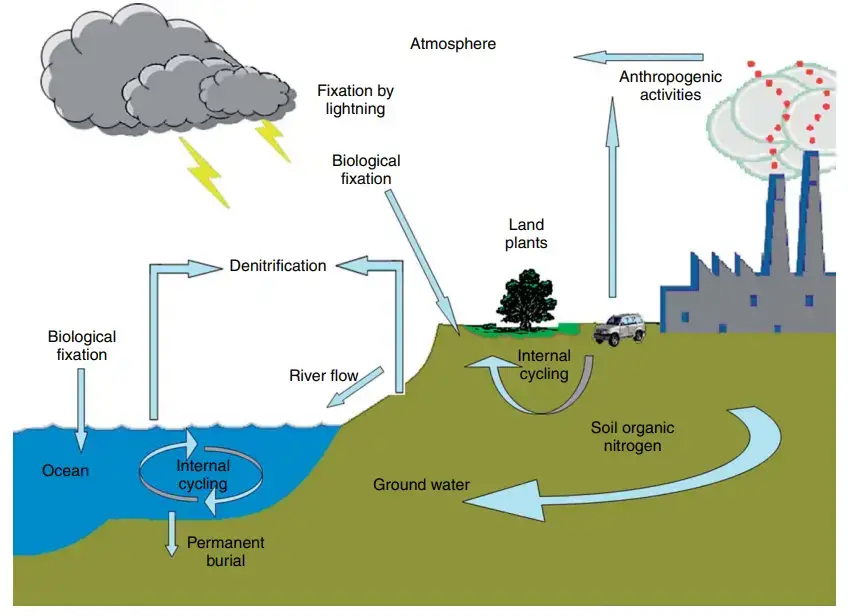
Stages of Nitrogen Cycle
Nitrogen fixation, nitrification, assimilation, ammonification, and denitrification are the steps in the Nitrogen Cycle. These processes occur in stages and are described in detail below:
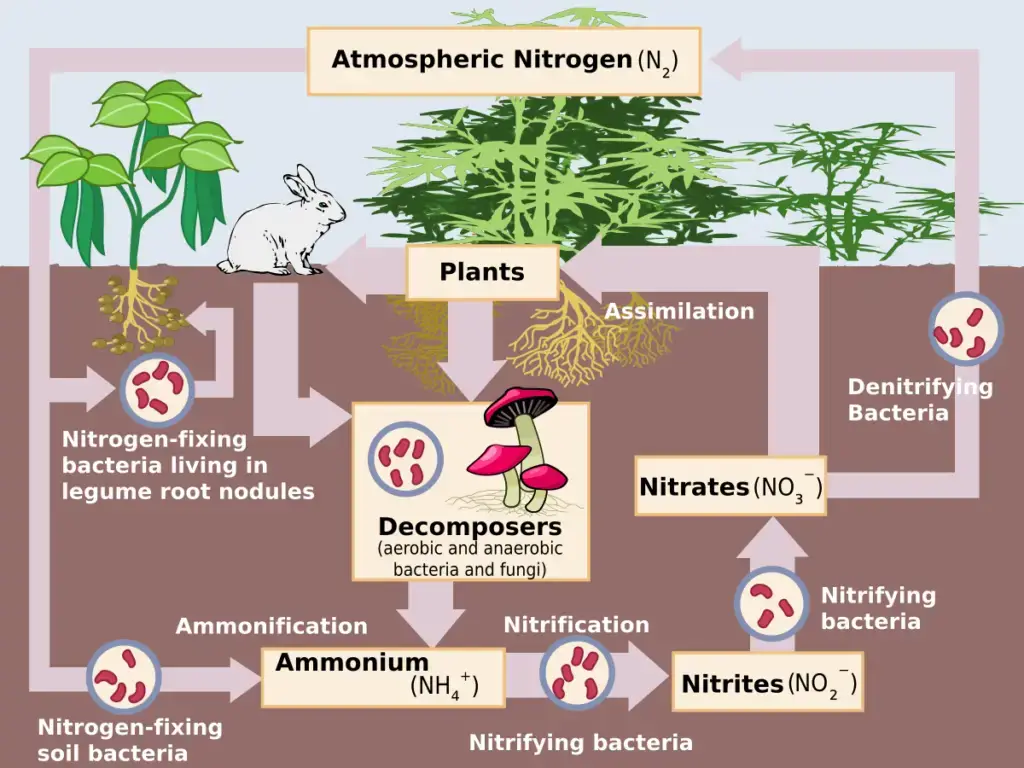
1. Nitrogen Fixation
- Nitrogen fixation represents the primary step in the nitrogen cycle, where the inert atmospheric nitrogen (N2) is transformed into a biologically usable form, specifically ammonia (NH3). This pivotal process is facilitated by a select group of microorganisms known as diazotrophs. These organisms possess a specialized enzyme, nitrogenase, which facilitates the combination of nitrogen atoms with hydrogen to produce ammonia.
- Among the diazotrophs, certain free-living soil bacteria, including Azotobacter and Klebsiella, as well as cyanobacteria or blue-green algae, play a significant role in this process. Additionally, symbiotic bacteria, predominantly Rhizobium, collaborate with plant roots to facilitate nitrogen fixation. It’s noteworthy that diazotrophic microorganisms contribute to approximately 60% of the Earth’s newly fixed nitrogen, estimated to be around 1011 kg annually. Other natural phenomena, such as lightning and ultraviolet radiation, account for an additional 15% of nitrogen fixation, with the residual portion attributed to industrial activities.
- The chemical reaction underpinning nitrogen fixation can be represented as: N2+3H2⇌2NH3 This reaction is mediated by the nitrogenase complex, which comprises a reductase and an iron–molybdenum-containing nitrogenase enzyme. The process is energy-intensive, necessitating the hydrolysis of at least 16 ATP molecules to produce two ammonia molecules. In symbiotic relationships, leghemoglobin acts as a protective agent, shielding the nitrogenase in Rhizobium from deactivation by oxygen.
- Furthermore, anthropogenic activities, including industrial combustion and agricultural fertilization, introduce additional fixed nitrogen into the environment. While industrial sources primarily release oxidized nitrogen gases like nitric oxide (NO) and nitrogen dioxide (NO2), agricultural activities contribute nitric oxide, nitrate (NO3–), and ammonia (NH3).
- In essence, nitrogen fixation serves as the gateway, ensuring the continuous replenishment of usable nitrogen in ecosystems, thereby sustaining life on Earth.
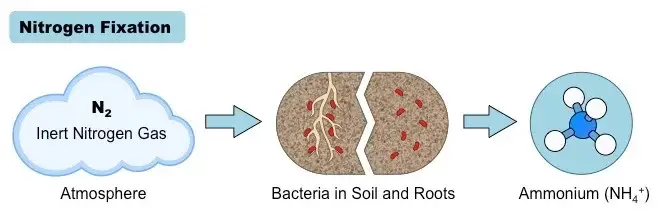
Nitrogen Fixation Steps/Process
Nitrogen fixation is a fundamental biochemical process that transforms the inert atmospheric nitrogen (N2) into forms that are biologically accessible to plants and other organisms. This conversion is pivotal for the sustenance of life, as nitrogen is a key component of amino acids, proteins, and nucleic acids. The process of nitrogen fixation can be categorized into several distinct pathways:
- Atmospheric Nitrogen Fixation: This process is predominantly driven by lightning. The intense heat generated during lightning strikes facilitates the conversion of atmospheric nitrogen into various nitrogen oxides, including nitrous oxide, nitrogen peroxide, and nitric oxide. These compounds, upon dissolution in rain, form diluted nitric acid. When this acidified rainwater reaches the terrestrial surface, it reacts with alkaline substances, producing nitrates that plants can readily assimilate.
- Biological Nitrogen Fixation: Certain specialized bacteria possess the capability to transform atmospheric nitrogen into ammonia. This biological conversion is mediated by the enzyme nitrogenase.
- Free-Living Bacteria: Organisms such as Azotobacter, Beijernickia, and Rhodospirillum, along with cyanobacteria, are examples of free-living bacteria that facilitate nitrogen fixation.
- Symbiotic Bacteria: Rhizobium, a symbiotic bacterium, forms associations with the roots of leguminous plants. Through a symbiotic relationship, it aids in the conversion of atmospheric nitrogen into forms that plants can utilize. The practice of crop rotation, where leguminous crops are alternated with other species, serves to naturally enrich soil nitrogen content.
- Industrial Nitrogen Fixation: The Haber process stands out as a pivotal industrial technique that transforms atmospheric nitrogen into ammonia. This synthesized ammonia is subsequently converted into various nitrates, which find extensive use in fertilizers to augment soil nutrient content.
- Nitrogen Fixation by Lightning: Beyond the primary atmospheric fixation process, lightning also plays a role, albeit minor, in nitrogen fixation. The energy from lightning strikes aids in the conversion of nitrogen into bioavailable forms, ensuring that plants have a consistent supply of this crucial nutrient.
Furthermore, anthropogenic activities, encompassing industrial processes, vehicular emissions, power generation, and even forest fires, release nitrogen oxides. These compounds, while not directly involved in the primary nitrogen fixation process, influence the overall nitrogen balance in the environment.
Types of Nitrogen Fixation
Nitrogen fixation is a pivotal process that facilitates the conversion of atmospheric nitrogen into forms that are biologically accessible. This transformation is essential for the sustenance of life on Earth, as nitrogen is a fundamental component of proteins, nucleic acids, and other vital biomolecules. The process of nitrogen fixation can be classified into three primary types:
- Atmospheric Fixation: This is a natural process wherein the immense energy from lightning strikes dissociates atmospheric nitrogen molecules, converting them into nitrogen oxides. These oxides subsequently dissolve in rainwater and are assimilated by plants. Through this mechanism, the ecosystem benefits from a direct influx of nitrogen, ensuring that terrestrial flora has access to this essential nutrient.
- Industrial Nitrogen Fixation: This method is anthropogenic in nature and involves the synthesis of ammonia through the direct combination of nitrogen and hydrogen gases. The resultant ammonia is a precursor for various nitrogenous fertilizers, such as urea. These fertilizers are then applied to agricultural lands, enhancing the nitrogen content of the soil and promoting plant growth.
- Biological Nitrogen Fixation: Contrary to popular belief, plants cannot directly assimilate atmospheric nitrogen. Instead, they rely on a symbiotic relationship with specific bacteria, such as Rhizobium and cyanobacteria (blue-green algae). These microorganisms possess the unique ability to convert atmospheric nitrogen into compounds that are readily usable by plants. Through this biological process, nitrogen is “fixed” in the soil in a form that plants can absorb and utilize for their growth and development.
2. Nitrification
Nitrification is a fundamental biochemical process in the nitrogen cycle, characterized by the sequential conversion of ammonia (NH3) or ammonium ions (NH4+) into nitrate (NO3–). This transformation is facilitated by specific soil bacteria in a two-tiered mechanism:
- Ammonia to Nitrite Conversion: In the initial phase, specialized bacteria, primarily Nitrosomonas and Nitrococcus, oxidize ammonia to form nitrite (NO2–). These bacteria are classified as “chemotrophs,” deriving their energy from chemical reactions rather than light or organic matter. The conversion of ammonia to nitrite is an energy-yielding process, and it predominantly occurs under aerobic conditions.Reaction: NH4+ (Ammonium) → NO2- (Nitrite) Catalyzed by: Nitrosomonas (Optimal pH range: 4 – 10)
- Nitrite to Nitrate Conversion: In the subsequent phase, the nitrite produced is further oxidized to nitrate (NO3–) by another group of bacteria, notably Nitrobacter. This reaction, too, is an aerobic process and is essential for the bacteria’s energy metabolism.Reaction: NO2- (Nitrite) → NO3- (Nitrate) Catalyzed by: Nitrobacter (Optimal pH range: 6 – 9)
Optimal Conditions for Nitrification:
- Aeration: Efficient oxygen supply is crucial as nitrification is an aerobic process.
- Temperature: The process is most efficient at temperatures ranging from 25°C to 35°C.
- Soil Moisture: Adequate moisture ensures the mobility of ions and facilitates bacterial activity.
- Exchangeable Bases: The presence of bases, especially calcium (Ca), enhances the nitrification process.
- Nutrient Availability: The presence of essential nutrients like NPK (Nitrogen, Phosphorus, and Potassium) supports bacterial growth and activity.
- Carbon to Nitrogen (C/N) Ratio: A lower C/N ratio is conducive for nitrification as it ensures adequate nitrogen availability.
Nitrification Steps/Process
Nitrification is a pivotal microbiological process in the nitrogen cycle, orchestrated by specific bacteria, including Nitrosomonas, Nitrosococcus, and Nitrosospira. This process encompasses the systematic oxidation of nitrogen compounds, transitioning from ammonia to nitrite and subsequently to nitrate.
Step 1: Conversion of Ammonia to Nitrite In the primary phase of nitrification, ammonia-oxidizing bacteria facilitate the transformation of ammonia (NH3) into nitrite (NO2-). The chemical reaction underpinning this conversion is delineated as: Ammonia (NH3) + Oxygen (O2) → Nitrite (NO2-) + 3 Hydrogen ions (3H+) + 2 electronsAmmonia (NH3) + Oxygen (O2) → Nitrite (NO2-) + 3 Hydrogen ions (3H+) + 2 electrons
Step 2: Transition from Nitrite to Nitrate Following the initial conversion, the nitrite produced undergoes a subsequent oxidation to form nitrate (NO3-). This reaction is mediated by nitrite-oxidizing bacteria. The chemical equation representing this transformation is: Nitrite (NO2-) + Water (H2O) → Nitrate (NO3-) + 2 Hydrogen ions (2H+) + 2 electronsNitrite (NO2-) + Water (H2O) → Nitrate (NO3-) + 2 Hydrogen ions (2H+) + 2 electrons
The genus Nitrobacter is instrumental in this secondary phase of nitrification. Furthermore, other bacterial genera, such as Nitrospina, Nitrospira, and Nitrococcus, also partake in autotrophic nitrite oxidation, reinforcing the conversion process from nitrite to nitrate.
To encapsulate, nitrification is a two-tiered process wherein autotrophic bacteria expedite the oxidation of ammonia first to nitrite and subsequently to nitrate. These sequential transformations are paramount in the nitrogen cycle, producing forms of nitrogen that are biologically assimilable. Through nitrification, the ecosystem is endowed with nitrates, an indispensable nutrient for flora and various ecological entities.
Factors Affecting the Nitrification Process
The nitrification process, carried out by specialized bacteria, is influenced by various environmental conditions that play a significant role in its rate and efficiency. Several key factors affect the nitrification process, including:
- pH: The pH level of the environment has a considerable impact on nitrification. Nitrifying bacteria function optimally within a specific pH range. Generally, a slightly acidic to neutral pH is preferred for efficient nitrification. Extreme pH levels can hinder the activity of nitrifying bacteria, leading to a slowdown or even inhibition of the process.
- Temperature: Temperature is a crucial factor affecting the rate of nitrification. Nitrification is a temperature-dependent process, with the activity of nitrifying bacteria increasing as the temperature rises within a suitable range. However, very high or low temperatures can disrupt the activity of these bacteria, affecting the overall nitrification process.
- Transfer Rate: Efficient nitrification also relies on the transfer rate of substrates, such as ammonia and nitrite, to the nitrifying bacterial colonies. A sufficient transfer rate ensures a steady supply of these substrates to the bacteria, promoting continuous nitrification.
- Type of Media: The type of media used in nitrification systems can influence the surface area available for bacterial colonization. A larger surface area allows more nitrifying bacteria to establish themselves, enhancing the overall nitrification capacity.
- Level of the Filter: In wastewater treatment systems, the level of the filter bed can affect nitrification. Properly designed filter systems ensure adequate contact time between the wastewater and nitrifying bacteria, facilitating efficient ammonia removal.
- Dissolved Oxygen: Nitrifying bacteria are aerobic microorganisms, meaning they require oxygen to carry out the nitrification process. Sufficient dissolved oxygen levels in the environment are essential for optimal nitrification rates. Inadequate oxygen levels can hamper the activity of nitrifying bacteria and lead to incomplete nitrification.
- Presence of Inhibiting Agents: Certain substances or chemicals can act as inhibiting agents for nitrifying bacteria. For example, high concentrations of toxic substances like heavy metals, chlorine, or specific organic compounds can negatively impact the nitrification process, reducing the effectiveness of nitrifying bacteria.
- Wastewater BOD (Biochemical Oxygen Demand): High levels of Biochemical Oxygen Demand (BOD) in wastewater can indicate the presence of organic matter that consumes oxygen during decomposition. This can reduce the available oxygen for nitrifying bacteria, affecting the nitrification process.
3. Nitrogen Assimilation
Nitrogen assimilation is a cardinal step in the nitrogen cycle, where inorganic nitrogen forms are integrated into organic nitrogenous compounds. This process is pivotal for both flora and fauna, enabling them to absorb and utilize the nitrate (NO3–) and ammonia produced through prior stages of nitrogen fixation and nitrification.
Mechanisms of Nitrogen Assimilation:
- Glutamate Dehydrogenase Pathway: Glutamate dehydrogenase is an enzyme that facilitates the reductive amination of α-ketoglutarate, an intermediate of the citric acid cycle. This reaction, although reversible, predominantly utilizes NADPH as the reductant in the biosynthetic direction. Apart from its role in nitrogen assimilation, this enzyme also plays a part in amino acid catabolism.
- Glutamine Synthetase Pathway: Glutamine synthetase is responsible for the assimilation of ammonia into glutamine. This reaction is driven by the energy derived from ATP hydrolysis. The nomenclature “synthetase” is attributed to this enzyme due to its ATP-dependent reaction mechanism, distinguishing it from “synthases” that operate without ATP.
- Incorporation into Amino Acids: The nitrogen assimilated in the form of glutamate and glutamine serves as a precursor for the synthesis of other amino acids. These amino acids are then integrated into proteins and nucleic acids within the organism.
Uptake and Reduction: Plants and certain microorganisms absorb nitrogen in the forms of nitrate, ammonium, and dissolved ammonia. These nitrogen sources are predominantly present in soils, marine sediments, or are procured from nitrogen-fixing entities. Nitrate undergoes a two-step reduction: initially to nitrite, catalyzed by nitrate reductase, and subsequently to ammonium, facilitated by nitrite reductase. The resultant ammonium is then incorporated into amino acids.
Central Enzymatic Players: The enzymes glutamine synthetase (GS) and glutamate synthase, also termed glutamine oxoglutarate aminotransferase (GOGAT), are central to nitrogen assimilation. GS mediates the integration of an amino group into glutamate, yielding glutamine. Concurrently, GOGAT reverses glutamine to glutamate, which acts as a substrate for synthesizing other amino acids. This process also involves the intermediate ɑ-ketoglutarate, a key component of the Krebs cycle.
Nitrogen Assimilation Process in Plants
Nitrogen assimilation in plants is a pivotal biochemical mechanism that facilitates the absorption and utilization of nitrogen, a vital element for plant growth and metabolism. This intricate process encompasses a series of enzymatic reactions that transform inorganic nitrogen sources from the soil into organic nitrogen compounds, which are integral to the synthesis of essential plant biomolecules.
- Mechanism of Nitrogen Uptake: Plants predominantly acquire nitrogen from the soil in two primary forms: nitrates (NO3-) and ammonium ions (NH4+). The plant root system is equipped with specialized transport proteins that facilitate the efficient uptake of these nitrogenous compounds, ensuring a consistent nitrogen supply for various metabolic activities.
- Nitrate Reduction Pathway: Upon absorption, nitrates are channeled to the plant leaves, where they undergo a sequential reduction process. The enzyme nitrate reductase, located in the cytoplasm, catalyzes the initial conversion of nitrate to nitrite (NO2-). Subsequently, within the chloroplasts, nitrite reductase facilitates the reduction of nitrite to ammonia (NH3). Notably, this reduction can also transpire in the root plastids.
- Ammonia Assimilation and Amino Acid Synthesis: The generated ammonia interacts with the amino acid glutamate, resulting in the formation of glutamine. This reaction is orchestrated by the enzyme glutamine synthetase. The glutamine synthetase-glutamate synthase (GS-GOGAT) pathway further enables the transamination of ammonia, leading to the production of other vital amino acids, including asparagine. Given the alkaline nature of plant cellular pH, the synthesized ammonia undergoes protonation, transforming into ammonium ions. However, elevated concentrations of ammonium ions can be detrimental to plant cells. To circumvent this, plants swiftly convert ammonium ions into amino acids, thereby integrating the nitrogen into proteins and other indispensable biomolecules.
- Significance of Nitrogen Assimilation: The process of nitrogen assimilation is of paramount importance for plants. It not only ensures the synthesis of crucial amino acids and proteins but also underpins various metabolic pathways. By efficiently converting inorganic nitrogen sources into organic forms, plants bolster their growth, development, and vitality. This mechanism underscores the role of plants in maintaining the nitrogen balance within ecosystems, contributing to ecological stability and sustainability.
Synthesis of amino acids from ammonium ion (NH4+) in plants
In plants, the assimilation of ammonium ions (NH4+) into amino acids is a fundamental biochemical process that underpins the synthesis of proteins and other vital biomolecules. This intricate mechanism encompasses two primary pathways: reductive amination and transamination, both of which play pivotal roles in nitrogen metabolism.
1. Reductive Amination:
Reductive amination stands as a cornerstone in the synthesis of amino acids from ammonium ions. In this process, NH4+ reacts with 𝛂-ketoglutaric acid, culminating in the formation of the amino acid glutamate. The enzyme glutamate dehydrogenase (GDH) orchestrates this reaction. The chemical representation of this reaction is as follows:
𝛂-ketoglutaric acid + NH4+ + NADPH → glutamate + H2O + NADP+
Glutamate, in this context, is not merely an end product but a central hub in nitrogen metabolism. It paves the way for the generation of other amino acids through a myriad of enzymatic transformations.
2. Transamination:
Transamination is a subsequent pathway integral to amino acid synthesis. Here, an amino group is shuttled from one amino acid (typically glutamic acid) to a keto acid, thereby synthesizing a new amino acid. The enzyme spearheading these reactions is termed transaminase.
This transamination process is versatile, facilitating the synthesis of a diverse array of amino acids by shuttling the amino group between different keto acids. Such a mechanism ensures a rich repertoire of amino acids, each serving distinct roles in plant physiology.
Moreover, specific amino acids, namely aspartic acid and glutamic acid, undergo further modifications. An additional amino group is appended to them, resulting in the formation of asparagine and glutamine, respectively. These compounds, termed amides, are pivotal for nitrogen storage and conveyance in plants, disseminating to various plant tissues via the xylem.
In certain plants, like the soybean, nitrogen fixation in nodules yields ureides. These compounds ascend with the sap due to transpiration and are instrumental in the plant’s internal nitrogen transport mechanism.
The conversion of ammonium ions into amino acids in plants is a meticulously orchestrated process, encompassing the pathways of reductive amination and transamination. These pathways are indispensable for the seamless integration of nitrogen into amino acids, which subsequently form the foundation for proteins and other quintessential biomolecules. Through a well-regulated nitrogen metabolism, plants ensure the optimal utilization and distribution of nitrogen, underpinning their growth, development, and overall vitality.
3. Ammonification
Ammonification, also referred to as mineralization, is a pivotal biochemical process in the nitrogen cycle. It encompasses the transformation of organic nitrogen forms, such as proteins, amino acids, and nucleic acids, into inorganic ammonia or ammonium (NH4+).
- Mechanism of Ammonification: The genesis of ammonification lies in the decomposition of organic matter. As plants, fungi, and animals die and decompose, the nitrogenous compounds within them, including proteins and genetic materials, undergo breakdown. This degradation is facilitated by proteolytic enzymes, which hydrolyze these compounds, releasing ammonia into the environment.
- Role of Soil Organisms: A myriad of soil-dwelling organisms, including bacteria and fungi, play a central role in ammonification. These microorganisms metabolize organic matter, harnessing the carbon and energy it provides. However, in this metabolic process, nitrogen is extricated and released in the form of ammonia. This ammonia, once liberated, stands ready for subsequent processes in the nitrogen cycle, such as nitrification or direct assimilation.
- Ecological Implications: Ammonification is ubiquitous, occurring in both terrestrial and marine ecosystems. In terrestrial realms, it is predominantly executed by plants, fungi, and small fauna. Conversely, in marine ecosystems, the baton is passed to non-photosynthetic microorganisms. The ammonia produced through ammonification can be assimilated to synthesize nitrogenous compounds, thereby augmenting biomass. Alternatively, it can embark on the path of nitrification, where it is oxidized to nitrate.
- Specialized Processes: In environments devoid of oxygen (anoxic conditions), a specialized process known as dissimilative nitrate/nitrite reduction to ammonia (DNRA) can transpire. Here, ammonium is derived from nitrate or nitrite. This process is a hallmark of certain nitrogen-reducing bacteria and fungi found in diverse habitats, ranging from ocean sediments and shores to human gastrointestinal tracts and wastewater treatment facilities.
Ammonification stands as a linchpin in the nitrogen cycle, bridging the gap between organic and inorganic nitrogen forms. By converting complex nitrogenous compounds into a more accessible form, ammonification ensures the perpetuation of the nitrogen cycle, sustaining life across ecosystems. This intricate process underscores the interconnectedness of life and the environment, highlighting the importance of each cog in the vast machinery of ecological processes.
Implications of Ammonification
Ammonification, the process of converting organic nitrogen into inorganic ammonia or ammonium ions, has several implications that are crucial for the cycling and availability of nitrogen in ecosystems.
- Recycling of Nitrogen: Ammonification plays a key role in the recycling of nitrogen. When plants or animals die and organic matter decomposes, the nitrogen contained in their tissues is released back into the soil in the form of ammonium ions. This recycling process ensures that nitrogen is continually available for uptake by plants and other organisms, supporting their growth and development.
- Nitrogen Utilization: Living organisms require nitrogen in various forms, such as ions, proteins, vitamins, and urea. Ammonification provides a pathway for converting organic nitrogen present in the remains of living organisms into inorganic forms that can be easily utilized by plants and other organisms. This availability of nitrogen is crucial for various biological processes, including protein synthesis and other essential cellular functions.
- Nutrient Cycling: Ammonification is an essential part of nutrient cycling in ecosystems. The release of ammonium ions into the soil makes nitrogen accessible to plants, which then take up this nitrogen and incorporate it into their tissues. As animals consume plants, they obtain nitrogen-containing compounds, and when these organisms die or excrete waste, the cycle continues through ammonification, completing the loop of nutrient cycling.
- Soil Fertility: Ammonification contributes to soil fertility by providing a continuous supply of nitrogen in an easily utilizable form. In ecosystems with decomposers that efficiently carry out ammonification, the soil becomes enriched with ammonium ions, promoting the growth of plants and supporting a diverse range of organisms within the ecosystem.
- Plant Adaptations: Certain plant species that thrive in acidic soils are particularly adapted to receive nitrogen most effectively through ammonification. The presence of ammonium ions in acidic soils favors the growth and development of these specialized plant species, allowing them to thrive in their specific environmental niches.
- Environmental Concerns: While ammonification is essential for nitrogen cycling, excessive levels of ammonia in the soil can have negative implications for ecosystems. For instance, when fertilizers are added to the soil to increase ammonia levels, it can lead to an overgrowth of algae in water bodies, causing eutrophication and disrupting aquatic ecosystems. Therefore, proper management of nitrogen-based fertilizers is essential to prevent environmental imbalances.
4. Denitrification
Denitrification is a microbial-driven process that facilitates the conversion of nitrate (NO3–) into gaseous nitrogen forms, primarily nitrogen gas (N2) and, to a lesser extent, nitrous oxide (N2O). This transformation, predominantly orchestrated by denitrifying bacteria, plays a pivotal role in the nitrogen cycle, ensuring the return of nitrogen to the atmosphere.
1. Environmental Context:
Denitrification predominantly occurs in environments characterized by limited oxygen availability, such as the deeper strata of soils proximate to the water table. Wetlands, with their saturated conditions, emerge as significant arenas for denitrification, aiding in the mitigation of surplus nitrogen levels.
2. Mechanistic Insights:
In the absence of sufficient oxygen, denitrifying bacteria employ nitrate as the terminal electron acceptor during cellular respiration. This initiates a cascade of enzymatic reactions, leading to the sequential reduction of nitrate to dinitrogen:
NO3– → NO2– → NO → N2O → N2
This progression involves a suite of enzymes, including nitrate reductase, nitrite reductase, nitric oxide reductase, and nitrous oxide reductase. However, not all denitrifying bacteria possess the complete set of these enzymes. As a result, some bacteria halt the reduction process prematurely, releasing intermediates like nitric oxide (NO) and nitrous oxide (N2O) into the atmosphere.
3. Conditions Favoring Denitrification:
- Oxygen scarcity
- Presence of oxidizable organic matter as an energy source
- Warm soil temperatures
- Slightly acidic soil pH
4. ANAMMOX: An Alternative Pathway:
Apart from denitrification, the nitrogen cycle also encompasses the anaerobic ammonium oxidation (ANAMMOX) process. Here, ammonium (NH4+) undergoes oxidation to yield dinitrogen gas (N2) in tandem with the reduction of nitrite (NO2–):
NH4+ + NO2– → N2 + 2H2O
This autotrophic process is executed by specific Planctomycetes species, predominantly located in marine sediments and wastewater treatment plant sludge.
Denitrification stands as a cornerstone of the nitrogen cycle, ensuring the cyclic return of nitrogen to the atmosphere. By transforming nitrates into gaseous nitrogen forms, it aids in maintaining the equilibrium of nitrogenous compounds in terrestrial and aquatic ecosystems. This process, in conjunction with ANAMMOX, underscores the intricate web of microbial interactions that underpin the sustainability of our planet’s nitrogen balance.
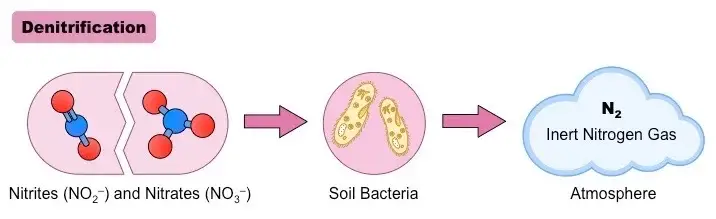
Half Reactions in Denitrification
Denitrification often occurs by a mixture of the following half-reactions, with the enzymes involved being listed in brackets:
- NO3− + 2 H+ + 2 e−→ NO₂− + H2O (Enzyme involved: Nitrate reductase)
- NO₂− + 2 H+ + e− → NO + H2O (Enzyme involved: Nitrite reductase)
- 2 NO + 2 H+ + 2 e− → N₂O + H2O (Enzyme involved: Nitric oxide reductase)
- N₂O + 2 H+ + 2 e− → N₂ + H2O (Enzyme involved: Nitrous oxide reductase)
A net balanced redox reaction where nitrate (NO3) is completely reduced to dinitrogen (N2) can be expressed as follows:
2 NO3− + 10 e− + 12 H+ → N2 + 6 H2O
Factors affecting the denitrification process
The denitrification process, a crucial part of the nitrogen cycle, is influenced by various factors that affect the activity and efficiency of denitrifying microorganisms in the soil. These factors play a significant role in regulating the rate and extent of denitrification, thereby impacting nitrogen dynamics in ecosystems. The main factors affecting the denitrification process are as follows:
- Soil Organic Matter: Soil organic matter is one of the most influential factors in the denitrification process. Denitrifying bacteria depend on organic matter present in the soil as their primary source of sustenance. This organic matter can originate from plant residues, decaying organic materials, or other sources within the soil. The availability of readily decomposable organic matter enhances denitrification rates, as it provides the necessary carbon sources for the denitrifying microorganisms.
- Soil pH: Soil pH plays a significant role in denitrification. Denitrification is favored in neutral to slightly acidic soils (pH 6-7), as extreme pH levels can inhibit the activity of denitrifying bacteria. Acidic conditions can suppress denitrification, while alkaline conditions may limit the availability of nitrogen sources for denitrification to occur efficiently.
- Soil Texture: Soil texture, referring to the proportions of sand, silt, and clay in the soil, can impact denitrification. Sandy soils with larger particle sizes tend to have better aeration and drainage, providing suitable conditions for denitrification to occur. In contrast, clayey soils with smaller particle sizes may have lower oxygen availability, which can affect denitrification rates.
- Temperature: Temperature is a crucial factor affecting denitrification rates. Denitrification is a microbial process, and the activity of denitrifying bacteria is influenced by temperature. Warmer temperatures generally enhance microbial activity, including denitrification, leading to higher rates of nitrogen loss as nitrogen gas.
- Soil Oxygen Concentration: Denitrification is an anaerobic process, meaning it occurs in the absence of oxygen. Therefore, the availability of oxygen in the soil can influence denitrification. Oxygenated soils tend to suppress denitrification, as the presence of oxygen competes with the denitrification process for microbial respiration.
- Soil Moisture Content: Soil moisture content is a critical factor affecting denitrification. Adequate soil moisture is required to support microbial activity, including denitrification. Waterlogged or excessively wet soils can promote anaerobic conditions, favoring denitrification.
- Nitrate Concentration in the Soil: The availability of nitrate (NO3-) in the soil is essential for denitrification to occur. Higher nitrate concentrations can support more denitrification activity. However, extremely high nitrate levels may lead to excessive denitrification, resulting in the loss of valuable nitrogen from the soil and negatively impacting nutrient availability for plants.
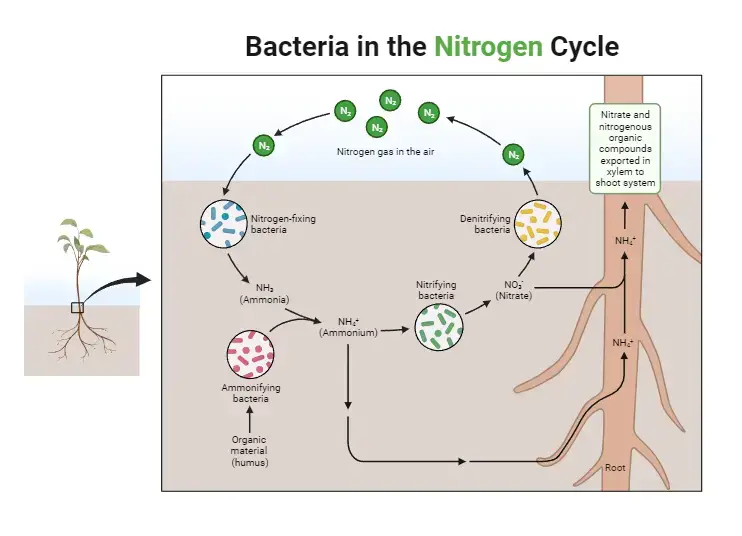
Nitrogen Cycle in Marine Ecosystem
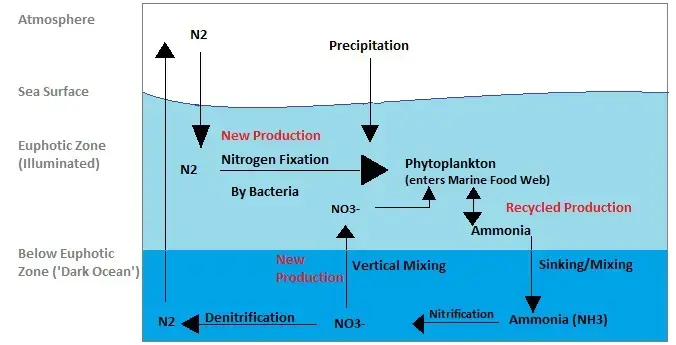
The nitrogen cycle in marine ecosystems shares many similarities with the nitrogen cycle in terrestrial ecosystems but also exhibits some unique features due to the presence of marine microorganisms. Let’s explore how the nitrogen cycle operates in marine environments:
- Nitrogen Input: Nitrogen enters marine ecosystems through various pathways, including precipitation, surface runoff, and atmospheric deposition. Atmospheric nitrogen, in the form of dinitrogen (N2), constitutes the largest portion of nitrogen in the atmosphere, and some of it dissolves in the water during rainfall and enters the oceans.
- Nitrogen Fixation: Nitrogen fixation is a crucial process in the marine nitrogen cycle, and it is primarily carried out by cyanobacteria, also known as blue-green algae. These specialized marine microorganisms have the ability to convert atmospheric dinitrogen into a usable form, such as ammonia (NH3) or nitrate (NO3-). Some marine bacteria, like Trichodesmium, play a significant role in fixing nitrogen in open ocean waters.
- Phytoplankton Consumption: Phytoplankton, microscopic marine algae, play a vital role in the marine nitrogen cycle. They consume the nitrogen-rich cyanobacteria and utilize the nitrogen-containing compounds obtained from them for their growth and metabolic processes.
- Ammonia and Urea Excretion: As phytoplankton consume cyanobacteria, they excrete ammonia (NH3) and urea (CO(NH2)2) into the water. These nitrogenous waste products are released into the marine environment, contributing to the pool of available nitrogen compounds.
- Ammonia Transport: Due to processes like waste mixing and sinking, the ammonia excreted by phytoplankton can be transported to lower depths in the ocean. This vertical transport helps to distribute nitrogen throughout different layers of the marine ecosystem.
- Nitrification: Bacteria in the marine environment convert ammonia into nitrites (NO2-) and nitrates (NO3-) through the process of nitrification. These nitrifying bacteria play a crucial role in converting ammonia, which is toxic at high concentrations, into less harmful nitrate forms.
- Phytoplankton Uptake: Nitrates are essential nutrients for marine organisms, especially phytoplankton. Nitrates in the water column are absorbed by phytoplankton, supporting their growth and photosynthetic activity.
- Denitrification: In some regions of the ocean, denitrifying bacteria perform denitrification, converting nitrate back into gaseous nitrogen (N2). This process releases dinitrogen into the atmosphere, completing the nitrogen cycle in the marine ecosystem.
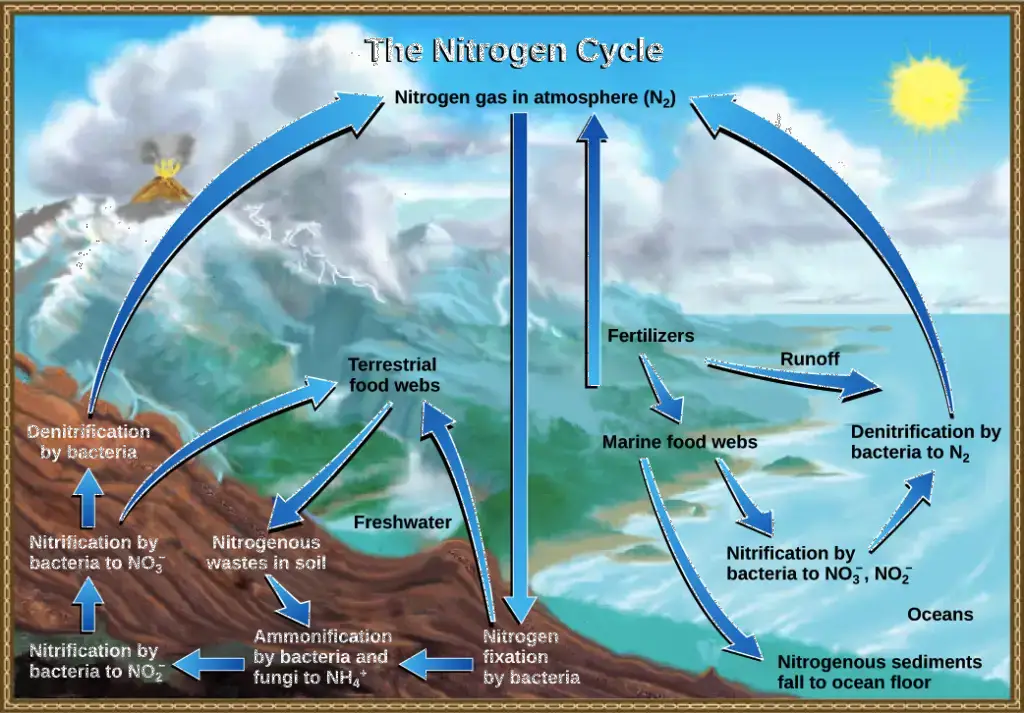
Nitrogen as a limiting nutrient
Many activities in natural ecosystems, including primary production and breakdown, are constrained by the availability of nitrogen. In other words, nitrogen is frequently the limiting nutrient, i.e., the nutrient that is in the smallest supply and so restricts the growth of organisms or populations.
How do we determine if a nutrient is scarce? This is frequently evaluated as follows:
- Adding more of a limiting nutrient will increase plant growth; for instance, it will lead plants to grow taller than if nothing were supplied.
- If a non-limiting nutrient is added, it will have no effect; for example, plants will grow to the same height regardless of the presence or absence of the nutrient.
Adding nitrogen to half of the bean plants in a garden and observing that they grew higher than untreated plants would indicate that nitrogen was limiting. If, however, we did not observe a difference in growth in our experiment, it would indicate that a nutrient other than nitrogen is limiting.
Nitrogen and phosphorus are the two most prevalent limiting elements in both natural and agricultural ecosystems. If you examine a bag of fertiliser, you will notice that it includes a great deal of nitrogen and phosphate.
Human activity affects cycling of nitrogen
Human activities have a significant impact on the cycling of nitrogen in the environment, leading to both positive and negative consequences. While humans cannot fix nitrogen organically, we do so industrially through the Haber-Bosch process, which converts atmospheric nitrogen (N2) into fixed nitrogen compounds, such as ammonia (NH3). This process is essential for the production of nitrogen-based fertilizers, which play a crucial role in modern agriculture.
- Industrial Nitrogen Fixation: Approximately 450 million metric tonnes of fixed nitrogen are produced annually through the industrial nitrogen fixation process. The majority of this fixed nitrogen is used to manufacture fertilizers that are widely used in agriculture to enhance crop yields. While this process has significantly contributed to global food production, it has also led to environmental concerns.
- Atmospheric Nitrogen Emissions: Human activities, such as the combustion of fossil fuels and the use of nitrogen-containing fertilizers, release nitrogen into the atmosphere. These processes increase the concentrations of nitrogen-containing molecules in the atmosphere, such as nitric oxide (NO) and nitrous oxide (N2O). Nitric oxide can react with other atmospheric compounds to form nitric acid (HNO3), contributing to the formation of acid rain, which can have detrimental effects on ecosystems and human infrastructure.
- Fertilizer Runoff and Eutrophication: One of the major environmental impacts of human nitrogen use is excess fertilizer runoff. When nitrogen and phosphorus-containing fertilizers are applied to agricultural fields, excess nutrients can be washed away by rainwater and enter nearby water bodies through surface runoff. This nutrient-rich runoff leads to eutrophication, a process where excessive nutrients cause algal blooms in lakes, rivers, and coastal waters.
- Algal Blooms and Dead Zones: Algal blooms occur when the excess nutrients from fertilizers stimulate the rapid growth of algae and other microorganisms in water bodies. As these organisms die and decompose, they consume large amounts of oxygen, leading to hypoxic or anoxic conditions in the water, known as “dead zones.” Dead zones can cause mass mortality of fish and other marine organisms, severely disrupting aquatic ecosystems.
- Greenhouse Gas Emissions: Nitrous oxide (N2O), a potent greenhouse gas, is released into the atmosphere as a byproduct of human activities like agricultural practices and industrial processes. It contributes to global warming and climate change.
Bacteria play a key role in the nitrogen cycle.
Bacteria play a crucial role in the nitrogen cycle, a biogeochemical process that regulates the flow of nitrogen through different ecosystems. Through various metabolic pathways, bacteria and other unicellular prokaryotes transform atmospheric nitrogen (N2) into physiologically useful forms, enabling the incorporation of nitrogen into living organisms.
- Nitrogen Fixation: Some bacteria have the remarkable ability to fix atmospheric nitrogen into a usable form. Nitrogen-fixing bacteria convert atmospheric nitrogen gas (N2) into ammonia (NH3), a physiologically useful form of nitrogen. These bacteria are either free-living in soil or water or form symbiotic relationships with plants. Rhizobium and Bradyrhizobium are examples of symbiotic bacteria that form nodules on the roots of leguminous plants, providing them with fixed nitrogen.
- Plant-Microbe Symbiosis: Nitrogen-fixing bacteria form a mutually beneficial relationship with plants. In plant roots, these bacteria reside in specialized structures called nodules, where they convert atmospheric nitrogen into ammonia for the plant’s use. In return, the plant provides the bacteria with carbohydrates and other nutrients.
- Nitrogen Assimilation: Once fixed by nitrogen-fixing bacteria, ammonia (NH3) is utilized by plants to produce organic molecules, including amino acids, proteins, and nucleic acids. When animals consume plants, they obtain these nitrogen-containing molecules, incorporating them into their bodies.
- Nitrogen Recycling: Nitrogen does not persist indefinitely in living organisms. When plants and animals die or excrete waste, organic nitrogen is returned to the environment. This is where bacteria play another vital role in the nitrogen cycle. Decomposers, mainly bacteria, break down organic nitrogen compounds from dead organisms and waste materials.
- Ammonification: In the process of ammonification, bacteria convert nitrogenous substances from dead organisms and waste into ammonia (NH3). This ammonia can then be used by other microorganisms or taken up by plants, continuing the nitrogen cycle.
- Nitrification: Bacteria further convert ammonia (NH3) into nitrites (NO2-) and nitrates (NO3-). This step in the nitrogen cycle is called nitrification and is essential for making nitrogen available to plants and other organisms in a form they can utilize.
- Denitrification: Denitrifying bacteria complete the nitrogen cycle by converting nitrates (NO3-) back into atmospheric nitrogen (N2) gas. This process occurs mainly in anaerobic conditions where oxygen is limited, such as waterlogged soils or sediments.
The Danger of Too Much Nitrogen
While nitrogen is essential for plant and animal life, too much nitrogen can pose significant dangers to both human health and ecosystems. Excessive nitrates in the soil, water, and food can lead to various adverse effects, highlighting the importance of managing nitrogen use sustainably.
- Toxicity in Humans: High levels of nitrates in food or water can be directly toxic to humans. When consumed in large quantities, nitrates can increase the risk of cancer and interfere with blood chemistry, hindering the proper oxygen-carrying capacity of blood. “Blue baby syndrome” is a known side effect of excessive nitrate consumption in infants, where nitrates interfere with the blood’s ability to carry oxygen, leading to cyanosis or bluish skin discoloration.
- Ecosystem Imbalance: Excessive nitrogen can disrupt natural ecosystems. Some organisms, like certain algae and plants, can thrive and grow rapidly when there is an abundance of nitrogen. This rapid growth can outcompete other organisms, leading to imbalances within ecosystems. For example, excessive nitrogen in water bodies can trigger the overgrowth of algae, resulting in algal blooms. These blooms can block sunlight and oxygen from reaching other aquatic organisms, leading to habitat degradation and harmful consequences for aquatic life.
- Red Tide and Harmful Algal Blooms: Nitrate fertilizer runoff into water bodies has been linked to the occurrence of harmful algal blooms, such as “red tides” and “brown tides.” These blooms are caused by toxin-producing algae, such as Pfiesteria bacteria. These toxins can sicken or kill marine organisms and pose health risks to humans and other animals that consume contaminated seafood.
- Disrupting Aquatic Food Webs: The excessive growth of certain algae and plants in response to high nitrogen levels can disrupt aquatic food webs. Some organisms may thrive, while others, like fish and other aquatic animals, may suffer due to habitat degradation or loss of food sources.
- Soil Degradation: Prolonged use of artificial nitrate fertilizers can lead to soil degradation and reduced fertility over time. The excessive application of nitrates can alter soil pH and nutrient balance, affecting the growth of crops and native plants.
- Finding Sustainable Solutions: Scientists are actively researching and developing sustainable agricultural practices to reduce reliance on artificial nitrate fertilizers. Natural or genetically engineered nitrogen-fixing plants are being explored as potential alternatives to traditional fertilizers. These practices aim to maintain soil fertility and crop yields without causing excessive nitrogen accumulation in the environment.
Examples of the Nitrogen Cycle
Examples of the nitrogen cycle can be found in various real-life scenarios, showing the interconnectedness of nitrogen’s movement in the environment. Here are three examples:
- Thanksgiving Story: The story of the first Thanksgiving highlights the significance of nitrogen in agriculture. When European settlers arrived in the Americas, they faced challenges in growing crops due to the lack of nitrogen in the soil. Without nitrogen-fixing crops or manure, which served as a common fertilizer in the old world, their crops struggled. However, they learned from the Native American Indians to bury dead fish in their crop fields. This practice restored nitrogen from the fish’s proteins and nucleotides back to the soil, enriching it with essential nutrients. As a result, their crops flourished, and they learned valuable agricultural practices from the Native Americans, demonstrating the role of nitrogen in promoting plant growth.
- The Three Sisters: Native American tribes traditionally grow three crops together – corn, beans, and squash – known as “the three sisters.” This combination is ingenious for multiple reasons. Firstly, it provides a balanced diet with proteins containing all essential amino acids when these three plants are consumed together. Secondly, it includes a nitrogen-fixing plant – beans. Beans have Rhizobium nodules in their roots, which contain bacteria capable of converting atmospheric nitrogen into a usable form for soil bacteria and plants. The nitrogen-fixing capability of beans benefits the surrounding soil, ensuring that it remains fertile for the growth of new plants, including corn and squash.
- Artificial Fertilizer: Modern agriculture heavily relies on artificial fertilizers, which are industrially produced nitrates that plants can directly utilize for growth. These fertilizers have revolutionized agriculture by providing an easily accessible source of nitrogen for crops, leading to increased food production. However, the use of artificial fertilizers also poses environmental challenges. Excessive application of fertilizers can lead to nutrient runoff into water bodies, causing algal blooms and eutrophication. The growth of toxic algae can have detrimental effects on aquatic ecosystems and even human health when it contaminates drinking water sources.
Importance of Nitrogen Cycle
The nitrogen cycle plays a vital role in maintaining the balance and sustainability of ecosystems. Its importance can be seen in various aspects of the natural world and human activities:
- Plant Growth and Chlorophyll Synthesis: Nitrogen is a crucial element required for the synthesis of chlorophyll, the green pigment responsible for photosynthesis in plants. Through the nitrogen cycle, nitrogen compounds are transformed into usable forms like nitrate and ammonium, which are essential for plant growth and development.
- Nitrogen Fixation: The nitrogen cycle involves nitrogen fixation, where specialized bacteria convert atmospheric nitrogen (N2) into ammonia (NH3), which can be utilized by plants. This process provides a renewable source of nitrogen for plants and contributes to maintaining soil fertility.
- Decomposition and Environmental Cleanup: Ammonification, a part of the nitrogen cycle, involves the breakdown of organic matter by bacteria. This decomposition helps in nutrient recycling and contributes to cleaning up the environment by breaking down dead plant and animal materials.
- Enriching Soil Nutrients: The conversion of nitrogen to nitrates and nitrites enriches the soil with essential nutrients required for plant growth. Plants take up these nitrogen compounds from the soil, which supports healthy plant growth and sustains diverse ecosystems.
- Formation of Biomolecules: Nitrogen is a crucial component of various biomolecules, including amino acids, proteins, and nucleic acids (DNA and RNA). These biomolecules are essential for the structure and function of living organisms, making nitrogen a fundamental element of life.
- Human Activities: The nitrogen cycle is impacted by human activities, such as the combustion of fossil fuels and the use of nitrogen-based fertilizers in agriculture. These activities increase the levels of nitrogen-containing compounds in the atmosphere, contributing to air pollution and affecting global nitrogen levels.
- Eutrophication: Excessive use of nitrogen-based fertilizers and wastewater runoff can lead to eutrophication in aquatic ecosystems. Elevated levels of nitrates and nitrites in water bodies promote the rapid growth of algae and other aquatic plants, leading to imbalances in the ecosystem and detrimental effects on other organisms.
Quiz
FAQ
Where does denitrification occur?
Denitrification is a microbiological process that removes beneficial nitrogen from soil and releases the greenhouse gas nitrous oxide (N2O) and tropospheric pollutant nitric oxide (NO). The biological cycle of denitrification involves an enzyme cascade that converts nitrate to dinitrogen.
Why does denitrification occur?
When the soil’s oxygen (O2) supply becomes depleted, a variety of microorganisms use oxygen for respiration instead of nitrate. Denitrification happens most frequently in wet, moist, or flooded soil when the oxygen supply for respiration is limited or restricted. Some fungi are capable of denitrification, however they are regarded insignificant.
When does the Denitrification process occur?
Denitrification is more active in locations where the percentage of water-filled soil pores reaches 60 percent. The end-product gas is contingent on the conditions of the soil and the microbial community. As the oxygen deficiency grows, bacteria fulfil their jobs by converting an increasing amount of nitrate to dinitrogen (N2) gas. Denitrification occurs in the loss of valuable nitrogen (N) for the purposes of nutrient management, but the effects on the atmosphere will vary.
What is nitrification?
The process by which bacteria convert atmospheric nitrogen into nitrites and nitrates is known as nitrogen fixation. It is utilised by plants, which then incorporates it into the food chain.
Name some denitrifying microorganisms.
Microorganisms that denitrify include Thiobacillus denitrificans and Micrococcus denitrificans.
What is the effect of denitrification?
Denitrification decreases the quantity of soil nitrogen that is fixed.
Why is nitrogen important for life?
Nitrogen constitutes several cellular components and is vital in many biological functions. For instance, the amino acids include nitrogen and serve as the building blocks for hair, tissues, and muscles, among other components of the human body.
Why do plants need nitrogen?
Nitrogen is required by plants since it is an essential component of chlorophyll. As a result, chlorophyll is essential for photosynthesis, and a lack of nitrogen can result in deficiency illnesses such stunted growth and other abnormalities.
What are the steps of Nitrogen Cycle?
Nitrogen Fixation
Assimilation
Ammonification
Nitrification
Denitrification
What is Ammonification?
During the degradation of organic matter, ammonifying bacteria convert organic nitrogen into inorganic compounds such as ammonia and ammonium ions.
What is Nitrification?
Nitrification is the process by which bacteria convert ammonia to nitrate. The bacteria Nitrosomonas, Nitrococcus, etc., convert ammonia to nitrite (NO2), which is then transformed to nitrate (NO3–) by Nitrobacter.
What is Denitrification?
Denitrification is the process by which bacteria such as Pseudomonas, Thiobacillus, Bacillus subtilis, etc. transform nitrate into molecular nitrogen.
What is the function of nitrifying bacteria?
Nitrifying bacteria are a tiny category of aerobic bacteria primarily responsible for the transformation of ammonia into nitrates.
Which part of the plant is involved in nitrogen fixation?
Nitrogen fixation occurs spontaneously in the root nodules of the plant in the soil.
References
- Burt, T. P. (2013). Nitrogen Cycle. Reference Module in Earth Systems and Environmental Sciences. doi:10.1016/b978-0-12-409548-9.00944-1
- Holland, E. A., & Weitz, A. M. (2003). Nitrogen Cycle, Biological. Encyclopedia of Physical Science and Technology, 441–448. doi:10.1016/b0-12-227410-5/00940-6
- Karl, D. M., & Michaels, A. F. (2001). Nitrogen Cycle. Encyclopedia of Ocean Sciences, 1876–1884. doi:10.1006/rwos.2001.0275
- Widdison, P. E., & Burt, T. P. (2008). Nitrogen Cycle. Encyclopedia of Ecology, 2526–2533. doi:10.1016/b978-008045405-4.00750-3
- Karl, D. M., & Michaels, A. F. (2001). Nitrogen Cycle. Encyclopedia of Ocean Sciences, 32–39. doi:10.1016/b978-012374473-9.00275-7
- Burt, T. P. (2013). Nitrogen Cycle. Reference Module in Earth Systems and Environmental Sciences. doi:10.1016/b978-0-12-409548-9.00944-1
- Gruber, N. (2008). The Marine Nitrogen Cycle. Nitrogen in the Marine Environment, 1–50. doi:10.1016/b978-0-12-372522-6.00001-3
- Global Nitrogen Cycle: Critical Enzymes, Organisms, and Processes for Nitrogen Budgets and Dynamics Xinning Zhang, Bess B. Ward, and Daniel M. Sigman Chemical Reviews 2020 120 (12), 5308-5351 DOI: 10.1021/acs.chemrev.9b00613
- Takai K. The Nitrogen Cycle: A Large, Fast, and Mystifying Cycle. Microbes Environ. 2019;34(3):223-225. doi: 10.1264/jsme2.ME3403rh. PMID: 31554776; PMCID: PMC6759337.
- https://www.toppr.com/guides/biology/microorganisms/nitrogen-cycle/
- https://kids.frontiersin.org/articles/10.3389/frym.2019.00041
- https://www.khanacademy.org/science/biology/ecology/biogeochemical-cycles/a/the-nitrogen-cycle
- https://www.vedantu.com/biology/nitrogen-cycle
- https://www.sciencelearn.org.nz/resources/960-the-nitrogen-cycle
- http://cceonondaga.org/resources/nitrogen-basics-the-nitrogen-cycle
- https://www.clearias.com/nitrogen-cycle/
- https://www.lenntech.com/nitrogen-cycle.htm
- https://flexbooks.ck12.org/cbook/ck-12-biology-flexbook-2.0/section/6.7/primary/lesson/nitrogen-cycle-bio/
- https://www.nature.com/scitable/knowledge/library/the-nitrogen-cycle-processes-players-and-human-15644632/
- https://www.visionlearning.com/en/library/Earth-Science/6/The-Nitrogen-Cycle/98
- https://royalsocietypublishing.org/doi/10.1098/rstb.2013.0164
- https://microbiologysociety.org/why-microbiology-matters/what-is-microbiology/microbes-and-the-outdoors/nitrogen-cycle.html
- https://biologydictionary.net/nitrogen-cycle/
- https://www.britannica.com/science/nitrogen-cycle
- https://en.wikipedia.org/wiki/Nitrogen_cycle
- https://prepp.in/news/e-492-nitrogen-cycle-environment-notes