What is Mycorrhiza?
- Mycorrhiza refers to the mutualistic symbiotic relationship between specific fungi and the roots of vascular plants. This intricate association, derived from the Greek words ‘mukes’ (fungus) and ‘rhiza’ (roots), is crucial for the survival and growth of many plant species. Approximately 90% of plant roots form associations with diverse fungal taxa, indicating the widespread nature of this relationship.
- The fungi involved in mycorrhiza envelop the developing roots of plants with a network of fine, fibrous structures. These fungal networks extend into the root cells by penetrating the epidermal layer. This interaction allows for a significant exchange of nutrients between the two organisms. The plant provides the fungi with carbohydrates derived from photosynthesis, while the fungi assist the plant in absorbing essential nutrients like phosphorus from the soil. This exchange defines the mutualistic aspect of the relationship, where both parties benefit.
- However, the relationship can vary based on the specific fungal and plant species involved. While mycorrhizae are typically mutualistic, there are instances where the fungi may exhibit parasitic behavior, extracting nutrients from the plant without offering significant benefits in return. In some cases, the plant’s defense mechanisms might be triggered, potentially leading to harm if the fungal invasion becomes too aggressive. Nonetheless, the association is predominantly beneficial for both the plant and the fungus.
- It’s important to note that the structures formed by mycorrhizal associations are microscopic, invisible to the naked eye. These fine networks within the plant roots play a pivotal role in nutrient exchange, enhancing the plant’s ability to thrive in various environments.
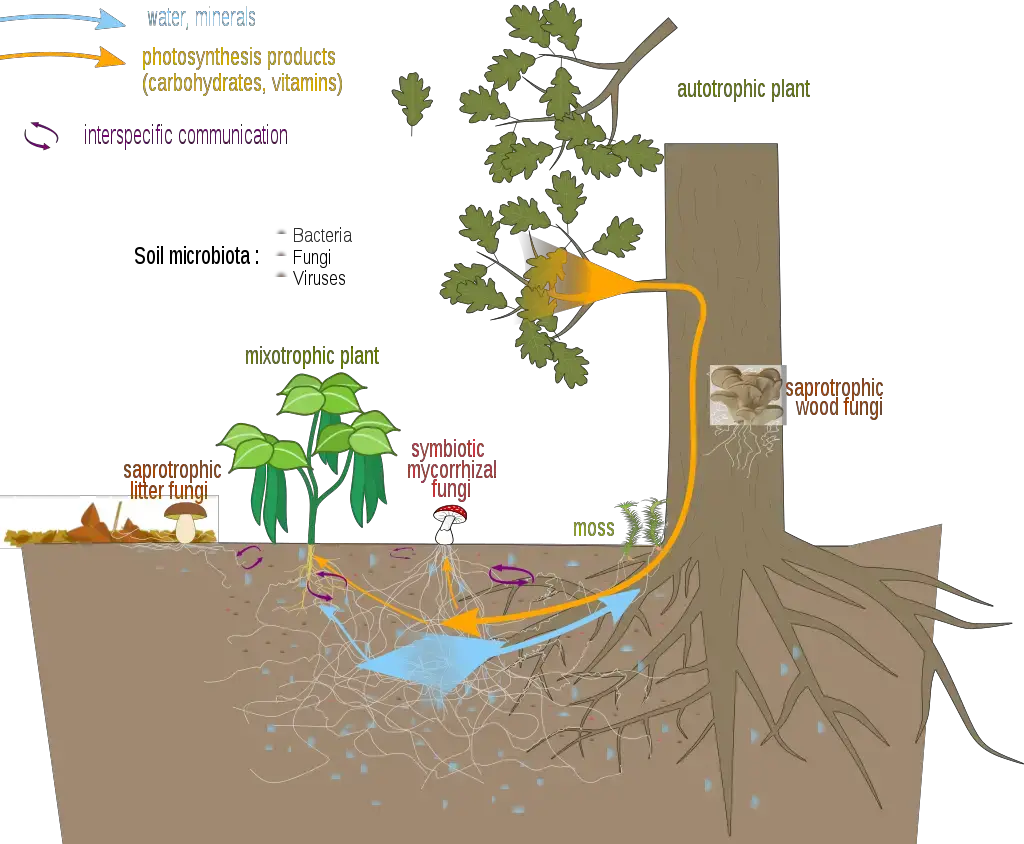
Definition of Mycorrhiza
Mycorrhiza refers to the symbiotic association between specific fungi and plant roots, where the fungus aids in nutrient and water uptake for the plant, and in return, the plant provides the fungus with essential nutrients produced through photosynthesis.
Types of Mycorrhizae
Mycorrhizae represent diverse symbiotic associations between fungi and plant roots, classified based on the location and interaction of the fungal hyphae within or around the plant root tissues. This classification, originally simplified into two main types—ectotrophic and endotrophic—has evolved to recognize a more complex array of mycorrhizal types. Here is an overview of the key mycorrhizal types:
- Ectomycorrhiza
- Characteristics: Ectomycorrhizae involve fungi that form a sheath around the outside of plant roots. This fungal sheath encloses the root, and hyphae penetrate between the root’s cortical cells but do not invade the plant cell walls. This structure, known as the Hartig Net, facilitates nutrient and carbon exchange between the plant and the fungus.
- Function: Ectomycorrhizal fungi alter root development by suppressing root hair formation and changing root branching patterns. This adaptation increases the root surface area for enhanced nutrient and water absorption. These fungi include species like Scleroderma cepa, Laccaria bicolor, and Rhizopogon luteolus.
- Endomycorrhiza
- Characteristics: In endomycorrhizae, the fungal partner lives entirely within the plant root tissues. The fungal hyphae penetrate the cortical cell walls of the roots, increasing the surface area for nutrient exchange.
- Types:
- Arbuscular Mycorrhiza (AM):
- Fungal Partners: Members of the Glomeromycota, such as Glomus spp.
- Characteristics: Fungi form arbuscular structures inside the root cells, increasing contact surface area for nutrient exchange. Vesicles may also be produced, though not all fungi form these structures.
- Function: AM fungi enhance nutrient uptake, especially phosphorus, and are associated with about 80% of plant species, including many agricultural crops.
- Orchidaceous Mycorrhiza:
- Fungal Partners: Basidiomycetes, such as Rhizoctonia, and related genera like Tulasnella, Sebacina, and Thanatephorus.
- Characteristics: Orchids, particularly in their seedling stages, rely on these fungi for nutrients and carbohydrates due to their initial non-photosynthetic state.
- Function: The fungi provide essential nutrients to the orchid seedlings, which are dependent on the fungal partner for early development.
- Arbutoid Mycorrhiza:
- Fungal Partners: Basidiomycetes associated with Ericaceae family plants like Arbutus and Arctostaphylos.
- Characteristics: A thin fungal sheath surrounds the plant roots, and hyphae penetrate both the epidermal and outer cortical layers, forming a paraepidermal Hartig Net.
- Function: This type allows nutrient exchange between arbutoid plants and adjacent tree species.
- Ericoid Mycorrhiza:
- Fungal Partners: Ascomycota and Deuteromycota fungi, such as Hymenoscyphus ericae, associated with Ericaceae, Epacridaceae, and Empetraceae plants.
- Characteristics: Similar to arbutoid mycorrhizae, ericoid fungi invade cortical cells and form hyphal coils within epidermal cells.
- Function: These fungi are crucial for nitrogen acquisition, aiding plants in nutrient-poor soils.
- Monotropoid Mycorrhiza:
- Fungal Partners: Basidiomycetes, especially boletes, associated with non-chlorophyllous Monotropa plants.
- Characteristics: These fungi form ectomycorrhizal associations with other plants and transfer nutrients to Monotropa, which cannot photosynthesize.
- Function: The fungi support Monotropa by providing essential nutrients through their interactions with other plant species.
- Arbuscular Mycorrhiza (AM):
- Ectendomycorrhiza
- Characteristics: Ectendomycorrhizae display features of both ectomycorrhizae and arbuscular mycorrhizae. Fungi involved show characteristics similar to ectomycorrhizae but also penetrate intracellularly into the host root cells.
- Fungal Partners: These mycorrhizae include ‘E-strain’ fungi from the genus Wileoxins within the Ascomycota phylum, order Pezizales.
- Function: They combine the nutrient uptake capabilities of ectomycorrhizae with the intracellular penetration characteristic of arbuscular mycorrhizae, offering a hybrid mode of nutrient acquisition.
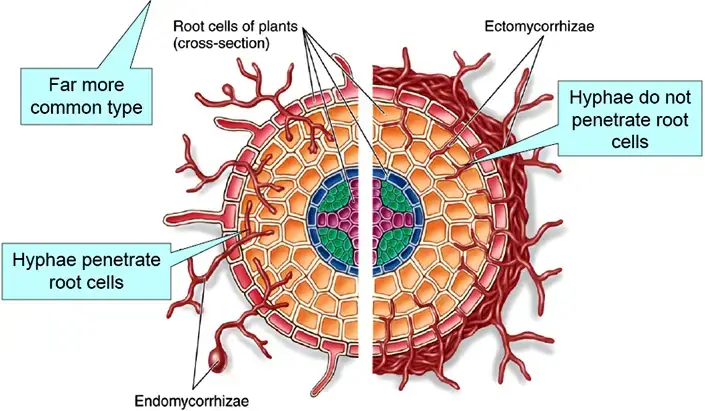
A. Ectomycorrhiza
- Definition and Overview:
- Ectomycorrhiza (EcM) is a type of symbiotic association primarily involving certain fungi and the roots of woody plants, such as trees from the birch, beech, willow, oak, pine, spruce, fir, and eucalyptus genera.
- Structural Characteristics:
- Hartig Net: This is a key feature of ectomycorrhiza, an intercellular network of fungal hyphae that interacts with the epidermal and cortical cells of the plant root. It is the primary site for nutrient and carbon exchange.
- Mantle: A dense sheath of fungal hyphae encases the root surface, distinguishing ectomycorrhizae from other types of mycorrhizal associations. This structure remains external to the root cells, with the fungal biomass primarily located outside the root.
- Extraradical Hyphae: These hyphae extend from the mantle into the surrounding soil, further contributing to the fungal network.
- Root Modifications:
- Suppressed Root Hair Development: Colonization by ectomycorrhizal fungi leads to the suppression of root hair growth.
- Altered Root Branching Patterns: The presence of ectomycorrhizal fungi induces changes in the branching patterns of the host plant’s roots. This can result in extensive branching and multiple root tips enveloped by a continuous hyphal sheath, often referred to as coralloid or tuberculate.
- Cytokinin Influence: The altered root branching is due to fungi-induced changes in cytokinin levels, which stimulate increased root branching, maximizing the root system’s surface area for nutrient and water absorption.
- Fungal Associations:
- Ectomycorrhizal fungi are commonly associated with forest and woodland mushrooms such as Russula, Hebeloma, and Amanita, as well as truffles like Tuber. These fungi belong to various fungal groups, including Basidiomycota, Ascomycota, and Zygomycota.
- While many ectomycorrhizal fungi are generalists, capable of forming symbiotic relationships with multiple tree species, some exhibit specificity, associating only with particular tree genera.
- Unique Variants:
- Ectendomycorrhiza: This is a unique variant that exhibits characteristics of both ectomycorrhizae and arbuscular mycorrhizae. It is particularly observed in pines and larches and involves specific fungi, such as those from the Pezizales order.
- Ecological Significance:
- Ectomycorrhizae play a crucial role in terrestrial ecosystems, facilitating nutrient exchange between fungi and host plants. This symbiotic relationship enhances the survival and growth of woody plants, contributing to the health and stability of forest ecosystems.
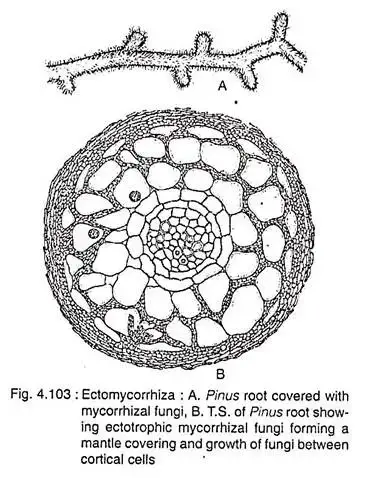
Morphology/Structure of Ectomycorrhiza
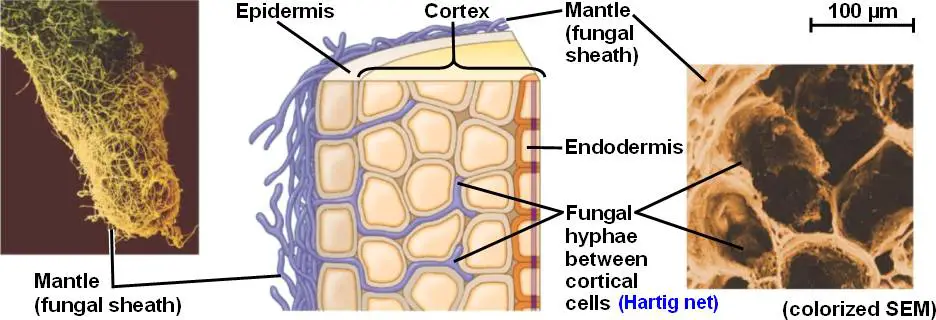
Ectomycorrhiza, a symbiotic relationship between certain fungi and plant roots, is characterized by distinct structural components that facilitate nutrient exchange and support plant growth. The primary structures involved in ectomycorrhiza include the Hartig net, the mantle, and the extraradical hyphae. Each of these structures plays a specific role in the symbiosis, contributing to the overall function of the ectomycorrhizal association.
- Hartig Net
- Primary Interface for Exchange: The Hartig net serves as the crucial interface where nutrient and carbon exchange occurs between the fungal partner and the plant. This network is formed by specialized fungal hyphae that penetrate the plant root.
- Formation and Origin: The Hartig net is initiated by hyphae originating from the inner regions of the mantle. These hyphae extend into the root of the host plant, establishing an intricate network.
- Structural Orientation: Within the root, the hyphae arrange themselves orthogonally to the root axis, creating an efficient interface for exchange with the root’s outer cells.
- Functional Role: The Hartig net is where the actual nutrient and carbon exchange takes place. The points where fungal hyphae and root cells contact are critical for this exchange, highlighting the importance of the Hartig net in the symbiotic relationship.
- Depth of Penetration: The extent to which the Hartig net penetrates the root varies among plant species. In some, like Eucalyptus, it remains within the epidermal layer, while in many gymnosperms, it extends deeper, reaching cortical cells and occasionally the endodermis.
- Root Adaptations: Root cells often elongate to increase the surface area for interaction, especially in epidermal Hartig nets. This adaptation enhances the efficiency of nutrient exchange.
- Mantle
- Encasing Sheath: The mantle is a sheath of fungal hyphae that envelops the root tip, forming a significant part of the fungal biomass in ectomycorrhiza.
- Structural Variation: The structure of the mantle can range from a loosely organized mesh of hyphae to a complex, multilayered system resembling plant parenchyma tissue, termed “pseudoparenchymatous.”
- Impact on Root Development: The mantle often inhibits root hair development, an adaptation that may enhance the efficiency of nutrient absorption through the fungal network.
- Cytokinin Influence: The mantle can stimulate the production of cytokinins, leading to increased root branching. In some cases, extensive branching results in multiple root tips being enveloped by a single, unified mantle, forming structures known as tuberculate or coralloid ectomycorrhizas.
- Identification Markers: The mantle’s color, branching pattern, and structural complexity serve as key identifiers for different ectomycorrhizal associations. These characteristics, along with molecular analyses, aid in determining the specific fungal partner involved.
- Extraradical Hyphae
- Soil Interaction: Extraradical hyphae extend from the mantle into the surrounding soil, compensating for the loss of root hairs and enhancing nutrient acquisition.
- Rhizomorph Formation: These hyphae can aggregate to form rhizomorphs, which are specialized structures that facilitate efficient nutrient transport. Rhizomorphs vary in complexity, with some resembling plant meristematic tissue in their growth patterns.
- Nutrient Transport: The extraradical hyphae play a critical role in transporting nutrients from the soil to the plant, often extending over significant distances to ensure adequate nutrient uptake.
- Common Mycorrhizal Networks (CMNs): These hyphae can connect multiple plants, forming CMNs that allow for the exchange of carbon and nutrients between interconnected hosts. CMNs also play a role in ecological processes such as seedling establishment and forest succession.
- Fruiting Bodies
- Reproductive Structures: Fruiting bodies, or sporocarps, are reproductive structures formed by ectomycorrhizal fungi. These bodies are essential for the sexual reproduction and dispersal of fungal spores.
- Biochemical Composition: Fruiting bodies are rich in nitrogen and complex carbohydrates, making them ecologically significant and potentially valuable as a nutritional resource for certain fauna.
- Diversity and Function: Fruiting bodies can be epigeous (above ground) or hypogeous (below ground), with both types serving the function of spore production and dispersal.
- Ecological Role: Hypogeous fruiting bodies, in particular, may serve as a food source for animals, contributing to the spread of fungal spores through mycophagy.
Mechanism of Ectomycorrhiza
Ectomycorrhiza (EcM) represents a sophisticated form of symbiosis between fungi and plant roots, characterized by a series of intricate mechanisms that facilitate nutrient exchange, offer protection, and promote mutual growth. To comprehend the full scope of this relationship, it is essential to understand the detailed processes involved in ectomycorrhizal interactions.
1. Presymbiosis
The presymbiotic phase marks the beginning of the ectomycorrhizal relationship and involves several critical steps:
- Fungal Invasion: Fungal hyphae emerge from the soil and navigate towards the plant roots. This migration is crucial for establishing a successful symbiotic interaction.
- Root Cap Infection: Upon reaching the plant roots, the fungi encase and infect the root cap cells. This step is vital for forming the initial contact necessary for the establishment of the symbiosis.
- Gene Expression: Both the plant and the fungus activate specific genes in response to the impending interaction. These genes are involved in secretory processes, apical growth, and the mechanisms of infection.
- Chemical Signaling: The plant releases chemical compounds into the rhizosphere that promote fungal growth and initiate the early stages of ectomycorrhizal formation.
2. Symbiosis
Once initial contact is established, the symbiotic relationship progresses through the formation of specialized structures and the exchange of nutrients:
- Mantle Formation: Fungal hyphae grow inward from the root cap cells to the epidermal cells, forming a mantle. This layer of fungal tissue encases the root and plays a crucial role in regulating root growth and branching.
- Polypeptide and mRNA Synthesis: During this phase, significant changes occur in the synthesis of polypeptides and mRNA. Ectomycorrhizins, specific polypeptides produced only during this phase, are critical for maintaining the symbiosis.
- Mantle’s Role: The mantle not only encases the root but also influences root hair production and branching. Research into fungal exudates provides insights into the mantle’s functional significance.
- Hartig Net Formation: The Hartig net, a network of fungal hyphae, forms within the inner mantle layer and extends into the root apoplast. Despite some plant cells producing defensive proteins that may inhibit the Hartig net’s development, this resistance generally wanes over time.
- Nutrient Uptake and Exchange: Nutrients are exchanged through three primary interfaces: soil-fungus, fungus-apoplast, and apoplast-root cell. The fungal hyphae induce root cells to bulge outward, facilitating effective nutrient transfer between the plant and the fungus.
3. Non-nutritional Benefits
Beyond nutrient exchange, ectomycorrhiza provides several non-nutritional benefits to the host plant:
- Water Transport: Extraradical hyphae, including rhizomorphs, extend the plant’s water absorption zone, enhancing its access to moisture.
- Protection: The hyphal sheath surrounding the root tips acts as a physical barrier, shielding the plant from pathogens and herbivores.
- Biochemical Defense: The fungi produce secondary metabolites that serve as biochemical defenses against pathogenic microorganisms attempting to invade the mycorrhizal roots.
- Tolerance to Stress: Ectomycorrhizal fungi improve the plant’s tolerance to heavy metals, salts, radionuclides, and organic contaminants, making them valuable in stressful soil conditions.
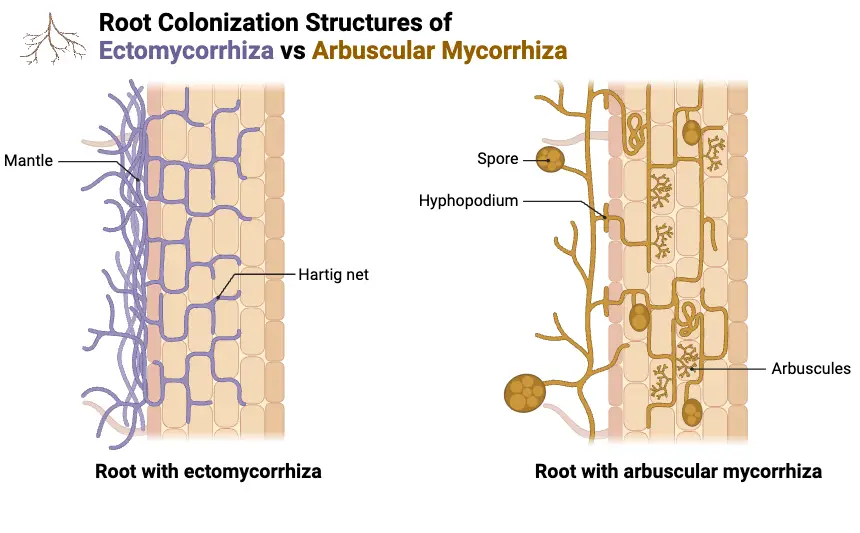
4. Ectendomycorrhiza
Some ectomycorrhizal fungi exhibit versatility by also forming arbuscular mycorrhizas, penetrating the cortical cells of plant roots. These dual interactions, termed ectendomycorrhizas, illustrate the adaptability of EcM fungi in creating diverse symbiotic relationships.
Importance of Ectomycorrhiza
Ectomycorrhiza (EcM) plays a pivotal role in various ecological, environmental, and agricultural contexts. Their importance spans across multiple domains, from agriculture and forestry to pollution mitigation and climate change adaptation.
- Agricultural Implications: Modern agricultural practices, such as tilling, excessive fertilization, and fungicide application, inadvertently jeopardize the health of mycorrhizae and the broader ecosystem. Such practices can inadvertently alter local ectomycorrhizal communities, with repercussions like diminished sporocarp production due to heightened fertilization.
- Forestry and Tree Plantations: In commercial forestry, the transplantation of trees to novel regions often necessitates the concurrent introduction of an ectomycorrhizal counterpart. This is particularly pertinent for trees with specialized mycobiont requirements or those introduced to regions with unfamiliar fungal species. Obligate ectomycorrhizal trees, exemplified by species like Eucalyptus and Pinus, often necessitate the application of a local EcM fungal inoculum for optimal growth in plantations. While such plantations are occasionally proposed as climate change mitigation strategies, the potential soil carbon reduction by their associated ectomycorrhizal fungi remains a topic of debate.
- Ecosystem Restoration: Given the integral role of ectomycorrhizas in supporting host plants, there’s a burgeoning interest in leveraging EcM fungi for ecosystem restoration endeavors. The reintroduction of mycorrhizal fungi, following their loss from a habitat, emerges as a vital step in re-establishing vegetation and rejuvenating ecosystems.
- Heavy Metal Detoxification: Ectomycorrhizal fungi exhibit resilience to heavy metals, which are detrimental to most life forms. These fungi can colonize metal-contaminated soils and bind significant amounts of heavy metals. Intracellularly, these metals undergo various detoxification processes, from sequestration to complex formation. Moreover, ectomycorrhizal fungal fruiting bodies can act as concentrators of heavy metals.
- Pollution Mitigation and Phytoremediation: EcM fungi demonstrate beneficial effects in diverse contamination scenarios:
- Salinity: Certain EcM fungi enhance host plant survival in high soil salinity conditions.
- Radionuclides: Some ectomycorrhizal species, especially within the Cortinariaceae family, exhibit hyperaccumulation of radionuclides.
- Organic Pollutants: Specific EcM species can degrade persistent organic pollutants, detoxifying compounds like 2,4-dichlorophenol and tetrachloroethylene.
- Climate Change Adaptations: Ectomycorrhizal communities exhibit varied responses to the multifaceted challenges of climate change. Elevated CO2 levels can amplify fungal mycelium growth and root colonization in certain EcM associations. Temperature fluctuations elicit diverse responses, ranging from detrimental to beneficial. Notably, many EcM species bolster host plant resilience during droughts, enhancing root water uptake capabilities.
Examples of Ectomycorrhiza
Below are notable examples of ectomycorrhizal fungi, each demonstrating unique characteristics and functions within their symbiotic relationships.
- Rhizopogon villosulus
- Description: Rhizopogon villosulus is a species of ectomycorrhizal fungus commonly associated with various coniferous trees.
- Function:
- Nutrient Uptake: This fungus helps in the absorption of essential nutrients, particularly phosphorus, which is often limiting in forest soils.
- Root Protection: It provides a protective mantle around the plant roots, safeguarding them from pathogens and environmental stress.
- Rhizopogon luteolus
- Description: Rhizopogon luteolus is another ectomycorrhizal species, known for its distinctive yellow to orange spore color.
- Function:
- Nutrient Exchange: It facilitates the transfer of nutrients between the soil and plant roots, enhancing plant growth.
- Soil Interaction: This species contributes to soil health by interacting with various soil components and improving soil structure.
- Rhizopogon amylopogon
- Description: Rhizopogon amylopogon features a distinctive amylaceous spore surface and forms a symbiotic association with several types of plants.
- Function:
- Phosphorus Acquisition: This fungus plays a significant role in phosphorus acquisition for the host plants.
- Symbiotic Benefits: It enhances plant resistance to environmental stresses and improves overall plant health.
- Rhizopogon fulvigleba
- Description: Rhizopogon fulvigleba is characterized by its pale yellow to brownish spore color and its role in ectomycorrhizal relationships.
- Function:
- Nutrient Transfer: It assists in the uptake of vital nutrients, contributing to plant vigor.
- Ecosystem Role: This fungus supports forest ecosystem health by promoting nutrient cycling.
- Pisolithus tinctorius
- Description: Pisolithus tinctorius is a widely studied ectomycorrhizal fungus known for its robust and durable mycelium.
- Function:
- Heavy Metal Tolerance: This species can help plants tolerate heavy metals and other contaminants in the soil.
- Nutrient Uptake: It aids in the acquisition of essential nutrients, including nitrogen and phosphorus.
- Suillus granulatus
- Description: Suillus granulatus is an ectomycorrhizal fungus commonly found in association with pine trees.
- Function:
- Mycelial Network: This fungus forms extensive mycelial networks that enhance nutrient uptake.
- Root Interaction: It contributes to the health of the host plant roots through nutrient exchange and protective mechanisms.
- Laccaria bicolor
- Description: Laccaria bicolor is a well-studied ectomycorrhizal species known for its distinctive, bright-colored fruiting bodies.
- Function:
- Nutrient Cycling: It plays a crucial role in nutrient cycling, particularly in the uptake of phosphorus and nitrogen.
- Ecosystem Health: This fungus supports forest ecosystem health by interacting with various soil organisms and plant species.
- Laccaria laccata
- Description: Laccaria laccata is recognized for its widespread distribution and association with a variety of tree species.
- Function:
- Nutrient Acquisition: It assists in the uptake of nutrients from the soil, benefiting the host plants.
- Symbiotic Interaction: This fungus contributes to the overall health of the plant by enhancing root growth and resilience.
- Scleroderma cepa
- Description: Scleroderma cepa is an ectomycorrhizal fungus with a distinctive, globular fruiting body and spore structure.
- Function:
- Nutrient Exchange: It facilitates efficient nutrient exchange between the soil and plant roots.
- Protection: This species offers protection to plant roots by forming a dense mantle.
- Scleroderma citrinum
- Description: Scleroderma citrinum is characterized by its bright yellow spore color and its role in ectomycorrhizal associations.
- Function:
- Nutrient Transfer: It aids in the transfer of nutrients such as phosphorus and potassium.
- Root Shielding: This fungus provides a protective layer around the roots, enhancing plant resistance to pathogens.
- Symbiotic Relationship: Ectomycorrhiza involves a mutualistic relationship between fungi and plant roots, where both partners benefit. The fungus aids in nutrient uptake for the plant, while the plant provides carbohydrates and other organic compounds to the fungus.
- Fungal Mantle: The fungi form a protective sheath, or mantle, around the roots. This mantle consists of fungal hyphae that envelop the root tips and play a role in nutrient absorption and root protection.
- Hartig Net Formation: Within the root, the fungi develop a network called the Hartig net, which penetrates the root’s intercellular spaces (apoplast). This structure facilitates nutrient exchange between the fungus and the plant.
- Nutrient Uptake: Ectomycorrhizal fungi are particularly effective at acquiring nutrients such as phosphorus and nitrogen from the soil. They extend their hyphal networks into the soil, increasing the surface area for nutrient absorption.
- Enhanced Plant Tolerance: Plants associated with ectomycorrhizal fungi often show improved tolerance to environmental stressors, including heavy metals, drought, and soil contaminants.
- Forest Ecosystems: Ectomycorrhizal fungi are critical components of many forest ecosystems. They help in nutrient cycling, enhance soil structure, and contribute to forest health and stability.
- Diverse Host Range: Ectomycorrhizal fungi can form associations with a wide range of plant species, including conifers, hardwoods, and some shrubs. This diversity allows them to inhabit various ecological niches.
- Reproductive Structures: Ectomycorrhizal fungi produce distinctive reproductive structures, such as mushrooms or toadstools, which release spores into the environment for dispersal and colonization.
- Role in Soil Health: These fungi improve soil health by enhancing organic matter decomposition, which contributes to soil fertility and structure.
- Complex Interactions: Ectomycorrhizal fungi often engage in complex interactions with other soil microorganisms, including bacteria and other fungi. These interactions can influence nutrient availability and plant health.
B. Endomycorrhiza
Endomycorrhiza refers to a category of mycorrhizal symbioses where the fungal component penetrates the host plant’s root cells, establishing a close and internal association. This form of mycorrhiza is characterized by the presence of fungal hyphae within the cortical cells of the plant roots, creating a profound and intricate relationship between the two organisms.
Key Characteristics of Endomycorrhiza
- Fungal Penetration:
- Internal Localization: The fungi involved in endomycorrhizae penetrate the cortical cell walls of plant roots. This internal penetration allows for a close association between the fungal hyphae and the host plant’s cells.
- Arbuscules Formation: A defining feature of many endomycorrhizae, particularly arbuscular mycorrhizae, is the formation of arbuscules. These are highly branched, tree-like structures within the host plant’s cells that facilitate nutrient exchange.
- Types of Endomycorrhiza:
- Arbuscular Mycorrhizae (AM):
- Structure: AM fungi form arbuscules and vesicles within the cortical cells of the plant roots.
- Function: They enhance nutrient uptake, particularly phosphorus, and improve plant growth and resilience.
- Ericoid Mycorrhizae (ERM):
- Association: Primarily associated with plants in the Ericaceae family, such as heathers.
- Function: They help in nutrient acquisition from acidic and nutrient-poor soils.
- Arbutoid Mycorrhizae:
- Host Plants: Found in plants of the Arbutus genus and related species.
- Role: They contribute to the plant’s nutrient absorption and soil interaction.
- Orchidaceous Mycorrhizae:
- Specificity: These mycorrhizae are specific to orchids.
- Function: They assist in nutrient uptake, which is crucial for the typically nutrient-poor environments where orchids grow.
- Arbuscular Mycorrhizae (AM):
- Prevalence:
- Wide Distribution: Endomycorrhizae are associated with over 80% of contemporary plant species, including a broad range of agricultural crops, greenhouse plants, vegetables, grasses, flowers, and fruit-bearing trees.
- Internal Exchange: The fungi’s hyphal networks extend into the root cells, creating a more intimate relationship compared to ectomycorrhizae.
- Benefits to Plants:
- Nutrient Acquisition: The internal structures formed by the fungi, such as arbuscules, facilitate efficient nutrient uptake, especially phosphorus and other essential minerals.
- Improved Growth: Endomycorrhizae enhance plant growth, health, and stress tolerance by improving nutrient availability and uptake.
- Fungal Hyphal Extension:
- Outside the Root: Besides penetrating root cells, fungal hyphae extend outside the root system, increasing the area for nutrient absorption and soil exploration.
- Ecological Role:
- Soil Health: Endomycorrhizal fungi contribute to soil health by promoting nutrient cycling and enhancing soil structure.
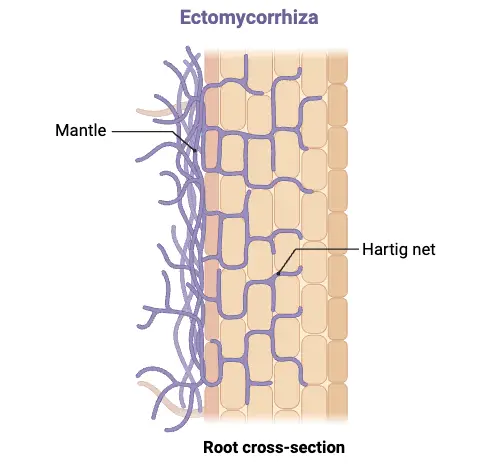
Types/Classification of Endomycorrhiza
Endomycorrhizas are varied and have been further characterised as arbuscular, ericoid, arbutoid, monotropoid, and orchid mycorrhizas.
1. Arbuscular mycorrhiza
Arbuscular mycorrhiza (AM) is a specialized type of mycorrhizal symbiosis involving fungi from the phylum Glomeromycota and a wide range of vascular plants. This symbiotic relationship is significant for various plant species, including grasses, herbs, and tropical trees, due to its crucial role in nutrient uptake and soil health.
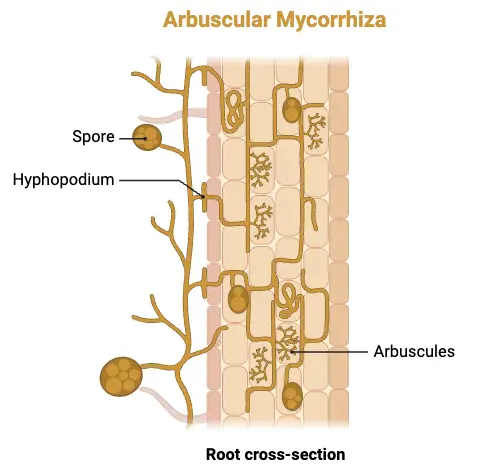
Key Characteristics of Arbuscular Mycorrhiza
- Symbiotic Association:
- Fungal Species: Arbuscular mycorrhiza involves fungi primarily from the phylum Glomeromycota.
- Plant Hosts: This association encompasses a broad array of vascular plants, including agricultural crops, grasses, and tropical trees.
- Fungal Penetration and Appressorium Formation:
- Initial Penetration: The fungal hyphae penetrate the plant’s epidermal cells through a combination of enzymatic action and hydrostatic pressure.
- Appressorium: This process begins with the formation of an appressorium, a swollen structure on the root’s exterior that generates the pressure needed to facilitate hyphal entry.
- Hyphal Navigation and Arbuscule Formation:
- Hyphal Pathway: After penetrating the epidermal cells, hyphae traverse the cortical cell walls, pushing aside the plasma membranes to establish a deeper connection within the plant tissues.
- Arbuscules: Inside the plant cells, the fungi develop arbuscules—highly branched, tree-like structures that increase the surface area for nutrient and carbon exchange between the fungus and the host plant.
- Types of Arbuscular Structures:
- Arum Type:
- Structure: Features multibranched, tree-like hyphal formations.
- Function: Facilitates extensive nutrient exchange through its complex branching.
- Paris Type:
- Structure: Characterized by hyphal coils within the host cells.
- Function: Provides a different structural adaptation for nutrient exchange.
- Arum Type:
- Impact on Root Hair Development:
- Suppression of Root Hairs: Fungal colonization often leads to the suppression of root hair development.
- Extraradical Hyphae: Instead, extraradical hyphae, which extend into the surrounding soil, enhance the root’s absorptive surface area and improve nutrient uptake.
- Vesicle Formation:
- Presence of Vesicles: Certain fungi, though not all, form vesicles within the root cells.
- Function of Vesicles: These vesicles, which stain lipid-rich, serve as storage units for nutrients and other materials.
- Spores Production:
- Asexual Spores: Fungi produce asexual spores both externally and within the root tissues.
- Identification: These spores vary in size and characteristics and are essential for identifying fungal species.
- Dispersal Mechanisms:
- Spores Dispersal: Spores are dispersed through multiple mechanisms, including air currents, water, and soil-dwelling organisms.
- Ecological Role: Dispersal ensures the colonization of new areas and the continuation of the symbiotic relationship.
- Nutrient Exchange:
- Enhanced Uptake: The primary function of arbuscular mycorrhiza is to enhance the uptake of nutrients, particularly phosphorus, by increasing the surface area for absorption.
- Mutual Benefits: In return, the plant supplies the fungi with carbohydrates and other organic compounds.
- Ecological Importance:
- Soil Health: AM fungi contribute to soil health by improving soil structure and fertility.
- Plant Growth: This mycorrhizal association supports plant growth and resilience, making it critical in various ecosystems, including agricultural and natural environments.
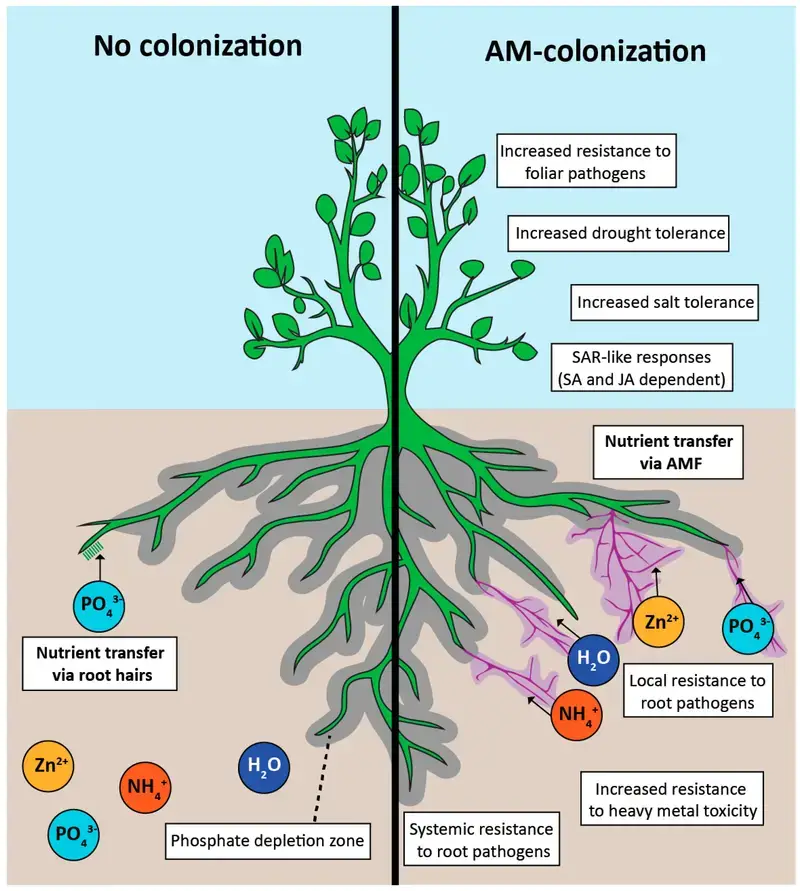
Vesicular Arbuscular Mycorrhizae (VAM)
Vesicular Arbuscular Mycorrhizae (VAM) represents a specialized symbiotic relationship predominantly observed in herbaceous angiosperms. This association, characterized by its distinct internal colonization patterns, differs significantly from ectomycorrhizae and plays a crucial role in plant nutrient acquisition and soil interaction.
Key Characteristics of Vesicular Arbuscular Mycorrhizae
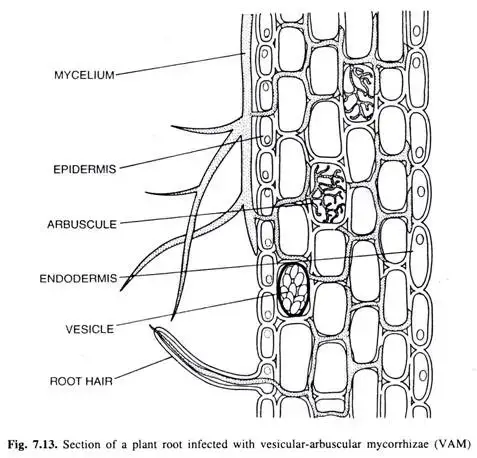
- Colonization Process:
- Entry into Roots: VAM begins when fungal mycelium infiltrates the host plant’s roots through root hairs and epidermal cells.
- Hyphal Growth: The hyphae navigate through the cortical cells of the root, forming two primary structures: vesicles and arbuscules.
- Structural Features:
- Arbuscules:
- Morphology: Arbuscules are highly branched, tree-like structures that are enveloped by the host plant’s plasma membrane. They penetrate only the cell walls, leaving the protoplast intact.
- Function: They increase the surface area for nutrient exchange between the fungal hyphae and the host’s cortical cells, facilitating efficient nutrient transfer.
- Vesicles:
- Shape and Function: Vesicles are ellipsoid-shaped structures that serve as storage units for polyphosphate and other minerals. They are involved in storing nutrients that can be mobilized during the symbiotic interaction.
- Nutrient Storage: These vesicles play a crucial role in storing and subsequently releasing nutrients, particularly polyphosphate, into the host cells.
- Arbuscules:
- Nutrient Exchange:
- Arbuscular Function: Arbuscules enhance the contact surface between fungal hyphae and the host’s cortical cells, allowing for direct nutrient transfer or the release of nutrients through arbuscular disintegration.
- Cytoplasmic Increase: VAM colonization can increase the cytoplasmic volume of host cortical cells by approximately 20-25%, indicating a significant impact on nutrient uptake and cellular function.
- External Structures:
- Absence of Mantle: Unlike ectomycorrhizae, VAM does not form a dense mantle around the roots.
- Hyphal Network: Externally, VAM consists of a sparse network of septate hyphae that extend into the soil. These hyphae include various spore types such as chlamydospores, sporocarps, and zygospores.
- Spore Formation and Dispersal:
- Types of Spores: The fungal hyphae contain spores that are critical for the reproductive cycle and dispersal of VAM fungi.
- Dispersal Mechanisms: Spores are dispersed through soil, water, and other environmental factors, aiding in the colonization of new plant hosts.
- Evolutionary Significance:
- Historical Evidence: VAM-like associations have been traced back to ancient terrestrial plants such as Rhynia and Asteroxylon, indicating the deep evolutionary roots of this symbiotic relationship.
- Role in Plant Evolution: This ancient association highlights the importance of mycorrhization in the evolution and sustenance of terrestrial plants.
- Impact on Plant Growth:
- Nutrient Acquisition: VAM enhances the plant’s ability to acquire essential nutrients, particularly phosphorus, from the soil.
- Soil Interaction: The symbiotic relationship contributes to improved soil health and structure, benefiting plant growth and ecosystem stability.
- Physiological Impact:
- Arbuscule Lysis: After approximately four days, arbuscules undergo lysis, releasing stored nutrients such as polyphosphate into the host cells, which supports plant nutrition and growth.
- Ecological Role:
- Soil Health: VAM contributes to soil nutrient cycling and enhances soil fertility, playing a significant role in various ecosystems, including agricultural and natural environments.
- Comparative Characteristics:
- Comparison with Ectomycorrhizae: Unlike ectomycorrhizae, VAM lacks a fungal mantle and has a more internal colonization pattern. Its biomass is relatively lower, constituting about 10% of the root weight
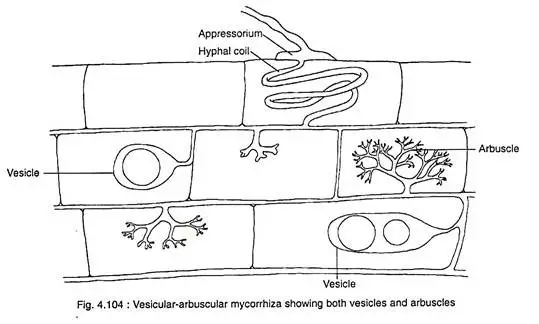
Mechanism of Arbuscular mycorrhiza
Arbuscular mycorrhiza (AM) represents a complex and highly specialized mutualistic symbiosis between certain fungi and the roots of most terrestrial plants. This relationship involves several phases: presymbiosis, symbiosis, and nutrient uptake and exchange. Each phase plays a critical role in facilitating efficient nutrient transfer and optimizing plant growth.
1. Presymbiosis
The presymbiotic phase establishes the groundwork for the mycorrhizal association:
- Spore Germination:
- Independence and Stimuli: AM fungal spores, characterized by their multinucleated structures and robust walls, can germinate independently of a host. However, root exudates from potential host plants can accelerate germination, especially in nutrient-rich conditions.
- Hyphal Growth:
- Influence of Exudates: The development and branching of AM hyphae are influenced by root exudates, particularly strigolactones, and soil phosphorus levels. Low phosphorus concentrations stimulate hyphal growth and branching, while high phosphorus levels may inhibit these processes.
- Host Recognition:
- Role of Exudates: Root exudates from plants, especially those grown in phosphorus-deficient soils, guide the growth and branching of AM fungal hyphae. This interaction enhances the fungi’s efficiency in locating a suitable plant host.
- Appressorium Formation:
- Infection Structure: Upon contact with the host root, AM fungal hyphae form an appressorium, a specialized infection structure on the root’s epidermis. This allows the hyphae to penetrate the root’s parenchyma cortex, marking the initiation of the symbiotic relationship.
2. Symbiosis
The symbiotic phase involves the development of arbuscules and other hyphal forms:
- Arbuscule Formation:
- Distinctive Structures: Arbuscules are highly branched, tree-like structures within the plant root. They come in two main forms: Paris and Arum types, depending on the host plant’s family. These structures facilitate the exchange of phosphorus, carbon, water, and other nutrients between the plant and the fungus.
- Host Regulation:
- Cellular Changes: The host plant regulates the growth of intercellular hyphae and the development of arbuscules. Cells containing arbuscules undergo significant changes, including organelle multiplication and cytoskeletal rearrangement, to accommodate the fungal structures.
- Other Hyphal Forms:
- Runner Hyphae: AM fungi produce short-lived runner hyphae that extend into the soil, enhancing phosphate and micronutrient absorption. These hyphae possess a higher surface-to-volume ratio compared to plant roots, improving their nutrient absorption efficiency.
- Unique Morphologies:
- Diverse Functions: AM fungi exhibit four distinct forms of hyphae, each with unique morphologies that support various functions within the symbiotic relationship.
3. Nutrient Uptake and Exchange
Nutrient exchange and uptake are central to the AM symbiosis, with specific mechanisms involved:
- Carbon Exchange:
- Dependence on Host: AM fungi rely on the host plant for carbon, absorbing hexoses produced through photosynthesis. This carbon transfer occurs via arbuscules or intraradical hyphae.
- Metabolic Pathways:
- Carbon Storage: The intraradical mycelium of AM fungi converts hexoses into storage forms like trehalose and glycogen. These compounds buffer intracellular sugar concentrations.
- Lipid Production:
- Role of Lipids: Lipids are produced within the intraradical mycelium and may be stored or transported to extraradical hyphae. Gluconeogenesis, the conversion of lipids into hexoses, occurs in extraradical mycelium.
- Phosphorus Absorption:
- Efficiency: AMF hyphae can absorb phosphorus more efficiently than plant roots due to their larger surface area and enhanced transport capacity. Phosphorus uptake can be up to six times faster than through root hairs.
- Nitrogen Flow:
- Impact on Soil Chemistry: AM symbiosis affects nitrogen flow by altering soil pH, enhancing the solubility of phosphorus precipitates, and influencing ammonium ion absorption and release.
- Carbon Investment:
- Plant Contribution: Plants invest a significant amount of carbon into the mycorrhizal network, which enhances phosphorus absorption and transfer from fungi to the plant.
- Phosphorus Absorption Variability:
- Species Differences: Various AMF species exhibit differing capacities to provide phosphorus to plants. Some species may act as poor symbionts, consuming more carbon than they contribute in phosphorus.
Importance of Arbuscular mycorrhiza
Arbuscular mycorrhizal fungi (AMF) play a critical role in plant nutrition and ecosystem functioning. Their importance is evident in several key areas, which are outlined below:
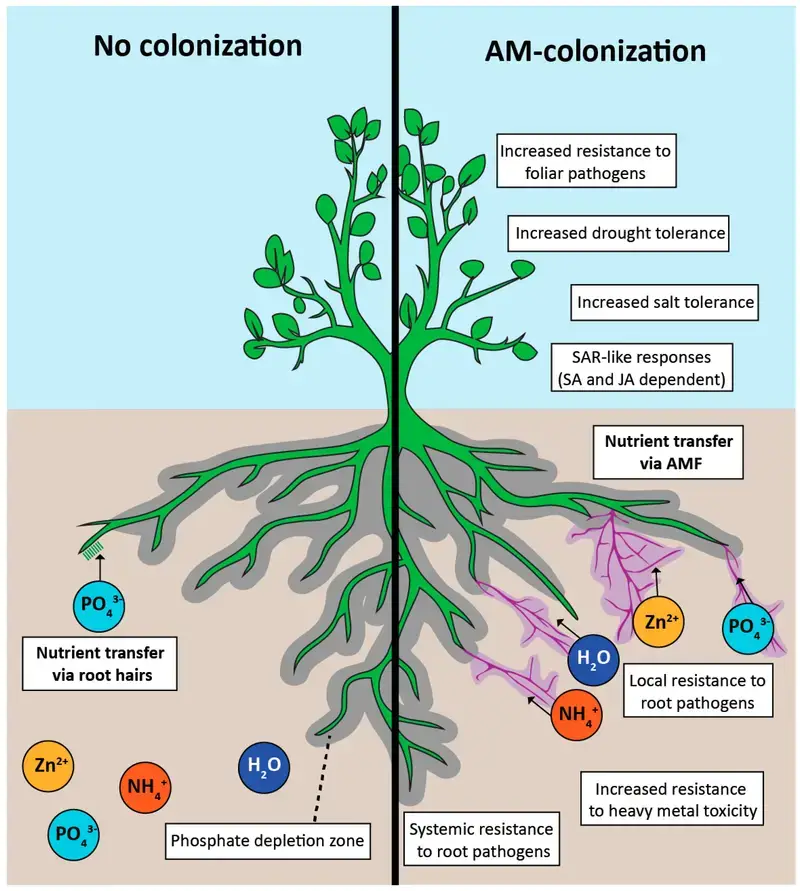
- Role in Phosphorus Uptake
- AMF are essential for the effective uptake of phosphorus, particularly in low-input agricultural systems where phosphorus availability is limited.
- The presence of phosphate fertilizers can inhibit AMF colonization and growth. As soil phosphorus levels increase, the benefits of AMF diminish since the carbon cost of maintaining the symbiotic relationship outweighs its benefits.
- Additionally, reduced AMF activity due to high soil phosphorus can lead to copper deficiency in plants. This is because copper uptake, which is facilitated by AMF, may decrease when mycorrhizal colonization declines.
- Enhancement in Perennial Cropping Systems
- The use of cover crops during fallow periods can significantly benefit AMF. These crops extend the growing season, providing continuous host plants for AMF.
- The presence of cover crops enhances the inoculum potential and hyphal networks of AMF, promoting more extensive and effective colonization in subsequent cropping seasons.
- This extended colonization period results in better phosphorus nutrition early in the growth stages of subsequent crops, which can contribute to increased crop yields.
- Augmentation of Soil Quality
- AMF contribute to soil quality by increasing aggregate stability through their extraradical hyphae and the production of glomalin, a soil protein.
- Glomalin-related soil proteins (GRSP) are critical for soil health, as they help in soil aggregation and nutrient retention.
- The application of native AMF in ecological restoration projects can accelerate soil recovery and improve overall soil quality.
- Phytoremediation Potential
- Inoculating soils with AMF before planting in ecological restoration projects is a promising method for land rehabilitation.
- AMF enhance plant growth and nutrient uptake, particularly in degraded soils, thereby improving soil quality.
- Soils inoculated with a consortium of indigenous AMF species show significant long-term improvements in soil parameters compared to non-inoculated soils.
- Native AMF strains are effective in extracting heavy metals from contaminated soils, making them valuable for agricultural use and environmental cleanup.
- Influence on Rhizosphere Ecology
- The rhizosphere, or the soil surrounding plant roots, is heavily influenced by AMF symbiosis.
- AMF affect the diversity and community structure of soil organisms through both direct interactions and alterations in plant root exudates.
- These interactions can modify the microbial and fungal communities in the rhizosphere, influencing overall soil health and plant growth.
- Implications in Global Climate Change
- AMF communities and their interactions with plant hosts are influenced by global climate change.
- Studies indicate that AMF can enhance plant biomass under drought conditions by improving water and nutrient uptake.
- However, AMF may reduce plant biomass under simulated nitrogen deposition scenarios.
- Elevated atmospheric CO2 levels have been shown to increase plant biomass when AMF are present, suggesting that AMF can play a role in mitigating some effects of climate change.
Plants lacking arbuscular mycorrhizae
Mustard plants (Brassicaceae) including cabbage, cauliflower, canola, and crambe are unable to colonise their root systems with arbuscular mycorrhizal fungus.
3. Ericoid mycorrhizae
Ericoid mycorrhizae represent a specialized form of symbiosis predominantly observed in members of the Ericaceae plant family. This mutualistic relationship is essential for the survival and adaptation of these plants in nutrient-poor and acidic environments. The key aspects of ericoid mycorrhizae are detailed below:
- Habitat and Adaptation
- Plants forming ericoid mycorrhizae are primarily found in acidic and nutrient-deficient soils. These habitats include bogs, heathlands, and boreal forests.
- This mycorrhizal association is crucial for these plants as it enhances their ability to acquire nutrients in challenging environments.
- Molecular clock analyses suggest that ericoid mycorrhizae originated approximately 140 million years ago, highlighting its long evolutionary history.
- Plant Families Involved
- The primary participants in ericoid mycorrhizal relationships are plants within the Ericaceae family. This family includes species such as heathers and blueberries.
- Additionally, other plant families like Epacridaceae and Empetraceae also form ericoid mycorrhizal associations. This indicates a broader evolutionary adaptation among these families to similar environmental stresses.
- Fungal Partners
- The fungal species Hymenoscyphus (Pezizella) ericae was initially identified as the principal endosymbiont in ericoid mycorrhizae.
- Recent studies have identified additional fungal genera involved in these associations, including Oidiodendron, Myxotrichium, and Gymnascella. These fungi contribute to the formation and maintenance of the symbiotic relationship with ericoid plants.
- Morphological Characteristics
- Ericoid mycorrhizae share some structural similarities with arbuscular mycorrhizae. The fungal hyphae penetrate the cortical cells of the host plant’s fine roots.
- Within these cells, the hyphae form complex coils, which are somewhat analogous to the arbuscules seen in arbuscular mycorrhizae.
- The fine roots of ericoid plants are distinct in having a central vascular bundle encased by a single layer of cortical or epidermal cells. This structural arrangement is integral to the functioning of the mycorrhizal association.
Structure and function of Ericoid mycorrhizae
Ericoid mycorrhizae represent a specialized form of symbiotic association between fungi and plants of the Ericaceae family. This relationship is characterized by unique structural features and functional roles that facilitate nutrient exchange and adaptation in nutrient-poor environments. Below is a detailed overview of the structure and function of ericoid mycorrhizae:
- Structural Characteristics
- Fungal Coils: Ericoid mycorrhizae are distinguished by the formation of fungal coils within the epidermal cells of the fine hair roots of ericaceous plants. These coils are created when fungal hyphae penetrate the cell walls of the plant’s cortical cells.
- Intracellular Penetration: Unlike some other mycorrhizal types, the hyphae of ericoid mycorrhizae do not invade the plasma membranes of plant cells but remain within the intercellular spaces. This structural arrangement allows the hyphae to form an extensive network around the exterior of the root hairs.
- Transient Nature: The coils are transient structures, typically persisting for a few weeks before the plant cell and the surrounding fungal hyphae disintegrate.
- Functional Role of Coils
- Nutrient Exchange: The coils function as the primary sites for nutrient exchange between the plant and the fungus. The plant supplies carbohydrates, derived from photosynthesis, to the fungus. In return, the fungus provides the plant with nutrients absorbed from the soil.
- Mutualistic Benefits: This mutualistic exchange is crucial for the survival and growth of both symbiotic partners. The plant benefits from enhanced nutrient uptake, while the fungus gains access to organic carbon sources.
- Enzymatic Capabilities
- Breakdown of Organic Compounds: Ericoid mycorrhizal fungi possess enzymatic capabilities that enable them to break down complex organic compounds. This function is significant for mineral acquisition.
- Nutrient Access: These enzymes help the fungi access organic forms of essential minerals, such as nitrogen, which are less available in the habitats of ericaceous plants. While there is some evidence of saprophytic tendencies, the primary role of these enzymes is to facilitate mineral acquisition from organic matter.
- Adaptation to Nutrient-Poor Environments
- Survival Strategy: The ability of ericoid mycorrhizal fungi to utilize organic nutrient sources is a key adaptation to the nutrient-poor environments in which ericaceous plants typically grow. These environments include acidic, boggy, and nutrient-deficient soils.
- Complementary Roles: The symbiotic relationship between the fungi and the plants ensures a steady supply of essential nutrients. The fungi’s enzymatic functions complement the plants’ photosynthetic processes, making the relationship indispensable for survival in challenging habitats.
Fungal symbionts of Ericoid mycorrhizae
Ericoid mycorrhizae, a specialized form of symbiotic association, is characterized by its interaction with a diverse range of fungal symbionts. These fungi play pivotal roles in the establishment and functioning of this symbiosis, especially in nutrient-poor environments where ericaceous plants predominantly thrive.
- Dominant Fungal Symbionts: Historically, much of the research on the physiology and function of ericoid mycorrhizal fungi has centered around isolates that were morphologically identified as Rhizoscyphus ericae. This fungus, belonging to the Ascomycota order Helotiales, has since been reclassified as a species of Pezoloma.
- Other Culturable Ascomycota: Apart from Rhizoscyphus ericae, several other culturable fungi from the Ascomycota phylum have been recognized as contributors to ericoid mycorrhizal formation. Notable among these are Meliniomyces, which shares a close phylogenetic relationship with Rhizoscyphus ericae, as well as Cairneyella variabilis, Gamarada debralockiae, and Oidiodendron maius.
- Diversity Revealed by DNA Sequencing: Modern molecular techniques, particularly DNA sequencing, have unveiled a broader spectrum of fungal communities associated with ericoid roots. However, it’s imperative to note that while these fungi are present, not all of them have been confirmed to form the characteristic ericoid mycorrhizal coils. Some might function as non-mycorrhizal endophytes, saprophytes, or even potential parasites, emphasizing the complexity of interactions within the ericoid mycorrhizal system.
- Basidiomycota Involvement: Beyond the realm of Ascomycota, fungi from the phylum Basidiomycota have also been identified as partners in ericoid mycorrhizal associations. Sebacina species, although not readily cultivable, are frequent inhabitants of ericoid roots and have demonstrated the capability to form ericoid mycorrhizas. Furthermore, certain basidiomycetes from the order Hymenochaetales have been implicated in the formation of this unique mycorrhizal type.
Economic significance of Ericoid mycorrhizae
- Several commercial and ornamental plant species, including blueberries, cranberries, and Rhododendron, have symbiotic relationships with ericoid mycorrhizal fungi.
- Inoculation with ericoid mycorrhizal fungus can affect plant development and nutrient absorption.
- However, ericoid mycorrhizal fungi have been the subject of significantly less agricultural and horticultural research than arbuscular and ectomycorrhizal fungi.
4. Orchid mycorrhiza
Orchid mycorrhizae represent a pinnacle of symbiotic relationships between plants and fungi, showcasing an intricate interdependence unparalleled in the realm of mycorrhizal interactions. With approximately 17,000 orchid species in existence, these plants heavily rely on their basidiomycete fungal counterparts for essential nutrient acquisition.
- Orchid-Fungal Interdependency: The degree of reliance between orchids and their fungal partners is profound. In certain orchid species, the symbiotic relationship is so deeply ingrained that the orchid seeds carry fungal propagules. This ensures that the fungus is immediately available during seed germination, facilitating nutrient uptake during the critical early stages of plant growth.
- Significance in Seed Germination: Given the diminutive size of orchid seeds, they possess limited nutrient reserves due to the absence of an endosperm. Consequently, the initial development of the protocorm post-germination is contingent upon the symbiotic fungus, not just for nutrient acquisition but also for carbohydrate provision. This symbiotic reliance persists until the orchid develops its photosynthetic capabilities—a process that can span up to a year in certain species. Remarkably, some achlorophyllous orchid species never achieve this photosynthetic capacity, rendering their dependence on the fungal partner perpetual.
- Fungal Structures: Arbuscules (Peletons): Within the cortical cells of the host orchid, the fungi form intricate, coiled structures known as arbuscules or peletons. These structures are transient in nature, and upon reaching the end of their lifespan, they leave behind deposits of cellulose and pectin within the host cell. Intriguingly, new fungal hyphae can subsequently invade these cells, tapping into the stored carbohydrates and nutrients.
5. Monotropoid mycorrhiza
Monotropoid mycorrhizae are specialized mycorrhizal associations predominantly found in the Monotropoideae subfamily within the Ericaceae family. These plants exhibit a unique adaptation, having largely lost their photosynthetic capabilities and existing as achlorophyllous plants on forest floors.
- Initial Misconceptions: Initially, plants associated with monotropoid mycorrhizae were believed to be parasitic on forest tree species. However, contemporary understanding reveals a more intricate relationship. These plants, rather than being direct parasites, leverage shared mycorrhizal symbionts common to neighboring trees.
- Carbohydrate Acquisition via Mycorrhizal Bridges: Monotropoid plants obtain essential carbohydrates from adjacent trees. This transfer is facilitated through mycorrhizal bridges, which connect the root systems of the monotropoid plants and the neighboring trees. This intricate network ensures a continuous supply of photosynthates to the achlorophyllous plants.
- Fungal Partnerships: Several fungal species, including Tricholoma, Russula, and Rhizopogon, have been identified as partners in these mycorrhizal associations. On their monotropoid hosts, these fungi exhibit characteristics similar to ectomycorrhizal associations on trees. They form fungal sheaths and Hartig nets, which are typical of ectomycorrhizal relationships. Additionally, these fungi produce unique structures called hyphal pegs, which originate from the inner sheath layer of hyphae and penetrate the tangential wall of the host’s cortical cells. Each cortical cell typically houses a single peg. The formation of these pegs is closely tied to the host plant’s growth and development, peaking during the flowering stage and subsequently diminishing.
- Nutrient Transfer and Acquisition: The pioneering work of Bjo¨rkman in the 1960s, utilizing radiotracers, provided evidence for the transfer of photosynthates from trees to Monotropa plants. Further research corroborated that this transfer is mediated through mycorrhizal bridges formed by shared fungal hyphae. While the exact mechanisms of nutrient acquisition by monotropoid mycorrhizae remain to be fully elucidated, it is postulated that the process mirrors that of ectomycorrhizal associations.
Other Mycorrhizae
1. Arbutoid (Ectendomycorrhizae)
Arbutoid mycorrhizae, also referred to as ectendomycorrhizae, represent a distinct type of mycorrhizal association predominantly observed between specific members of the Ericaceae family, namely Arbutus and Arctostaphylos, and several genera within the Pyrolaceae family.
- Fungal Partnerships: A variety of ectomycorrhizal fungal species have been identified to establish these arbutoid associations. These fungi play a pivotal role in facilitating nutrient exchange and enhancing the overall health and growth of their host plants.
- Structural Characteristics: The hallmark of arbutoid mycorrhizae is the formation of a delicate fungal sheath that envelops the exterior of the root. This is accompanied by the development of a paraepidermal Hartig net, which is characterized by fungal penetration between the plant’s epidermis. Furthermore, the outermost layer of cortical cells witnesses the invasion of fungal hyphae, leading to the formation of intricate hyphal coils, also known as intracellular hyphal complexes.
- Nutrient and Photosynthate Exchange: One of the primary functions of arbutoid mycorrhizae is to facilitate the exchange of essential nutrients and photosynthates. It is postulated that arbutoid plants may engage in nutrient and photosynthate exchange with neighboring tree species, further emphasizing the interconnectedness and mutualistic nature of these symbiotic relationships.
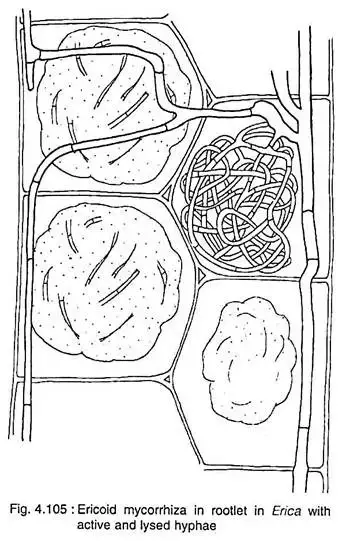
2. Dark septate endophytes
Dark septate endophytes (DSE) represent a group of root endophytes that have been detected in a myriad of plant species spanning almost all plant families. Their prevalence is particularly notable in environments characterized by cool temperatures and limited nutrient availability.
- Taxonomic Diversity: While a significant proportion of these fungi remain unidentified, certain genera such as Chloridium, Leptodontidium, Phialocephala, and Phialophora have been recognized in plant roots. This highlights the vast taxonomic diversity and potential ecological roles of DSE.
- Structural Adaptations: DSE fungi typically gain entry into the plant root system either through root hairs or cortical cells. Once inside, they form runner hyphae that navigate between the cortical cells. A distinctive feature of these fungi is the formation of microsclerotia, which are densely branched structures that establish themselves within the host cell. The initial hyaline hyphae of these fungi often undergo melanin deposition in their cell walls, resulting in a dark hue, which is the origin of their nomenclature.
- Functional Significance: The exact functional role of DSE remains an area of ongoing research. However, their pronounced presence in oligotrophic (nutrient-poor) and climatically challenging environments suggests a potential role in nutrient acquisition. It is postulated that DSE may possess specialized enzymatic capabilities that enable them to extract nutrients from inorganic sources, thereby benefiting their host plants in nutrient-scarce settings.
Functions of Mycorrhiza
Mycorrhizae, the symbiotic associations between fungi and plant roots, are known for their significant role in enhancing nutrient uptake. However, their functions extend beyond mere nutrient acquisition, impacting plant growth, water uptake, and defense mechanisms. This overview details the multifaceted roles of mycorrhizal associations, supported by scientific findings.
- Nutrient Acquisition
- Enhanced Uptake:
- Mechanism: Mycorrhizal fungi extend their hyphae into the soil, creating a larger surface area for nutrient absorption compared to the plant’s root hairs. This extended reach facilitates more efficient acquisition of essential nutrients like phosphorus.
- Evidence: Studies using radioactively labeled phosphate have demonstrated that mycorrhizal roots absorb more phosphorus than non-mycorrhizal roots. The presence of extensive fungal hyphae increases the nutrient depletion zone around the roots, enhancing phosphorus uptake.
- Energy Efficiency:
- Cost-Benefit: The production of extensive hyphae is thought to be energetically advantageous compared to developing root hairs, as it provides a greater absorptive surface at a lower energy cost. However, this hypothesis has been subject to scrutiny in recent studies.
- Carbohydrate Exchange:
- Function: Mycorrhizal fungi, particularly ectomycorrhizae, receive carbohydrates from the host plant. This exchange supports various fungal functions, including defense against pathogens. The plant increases its expression of hexose transporter genes to facilitate this exchange.
- Phosphate and Nitrogen Transport: Arbuscular mycorrhizae use proteins such as PHT phosphate transporters for phosphate uptake. Similarly, ectomycorrhizal fungi utilize ammonium and nitrate transporters for nitrogen acquisition. Both types of mycorrhizae may also store nutrients in polyphosphate granules.
- Agricultural and Horticultural Benefits:
- Growth Improvement: Mycorrhizal fungi enhance plant growth parameters such as height, stem diameter, and foliar nutrient content. They are used commercially to improve crop yields and plant health in agriculture and nurseries.
- Enhanced Uptake:
- Water Acquisition
- Water Transport:
- Hyphal Role: Mycorrhizal hyphae and rhizomorphs (in ectomycorrhizae) can transport water from the soil to the plant. This function is especially crucial in dry environments, where water availability is limited.
- Species-Specific Effects: The impact of mycorrhizae on water acquisition varies among plant species. For instance, arbuscular mycorrhizae have shown inconsistent results in drought tolerance across different species, such as Acacia and Leucaena.
- Deep Root Systems:
- Water Access: Mycorrhizal associations have been observed to reach deep soil layers, which can be beneficial for accessing water in arid regions, as seen in studies of Acacia in Senegal.
- Water Transport:
- Plant Defense
- Physical and Biochemical Protection:
- Barrier Formation: Ectomycorrhizal fungi create a physical barrier around the root system, which can protect against grazing soil fauna and pathogens.
- Chemical Defense: Mycorrhizal fungi produce secondary metabolites that enhance plant resistance to pathogens and pests. For instance, Glomus mosseae has been shown to significantly improve plant growth and protect against pathogens like Fusarium solani and Rhizoctonia solani.
- Pathogen Resistance:
- Examples: Studies have shown that mycorrhizal fungi such as Laccaria laccata and Paxillus involutus reduce the impact of root pathogens on plant growth. This protective function is valuable in agriculture and forestry, where high plant densities and intensive management increase pathogen risks.
- Quantitative Data:
- Meta-Analysis: Research by Borowicz (2001) has demonstrated that mycorrhizal fungi can inhibit the effects of pathogens and nematodes. However, the extent of this defense can vary, with evidence suggesting that mycorrhizal protection against certain nematodes may be less pronounced.
- Physical and Biochemical Protection:
Significance of Mycorrhiza
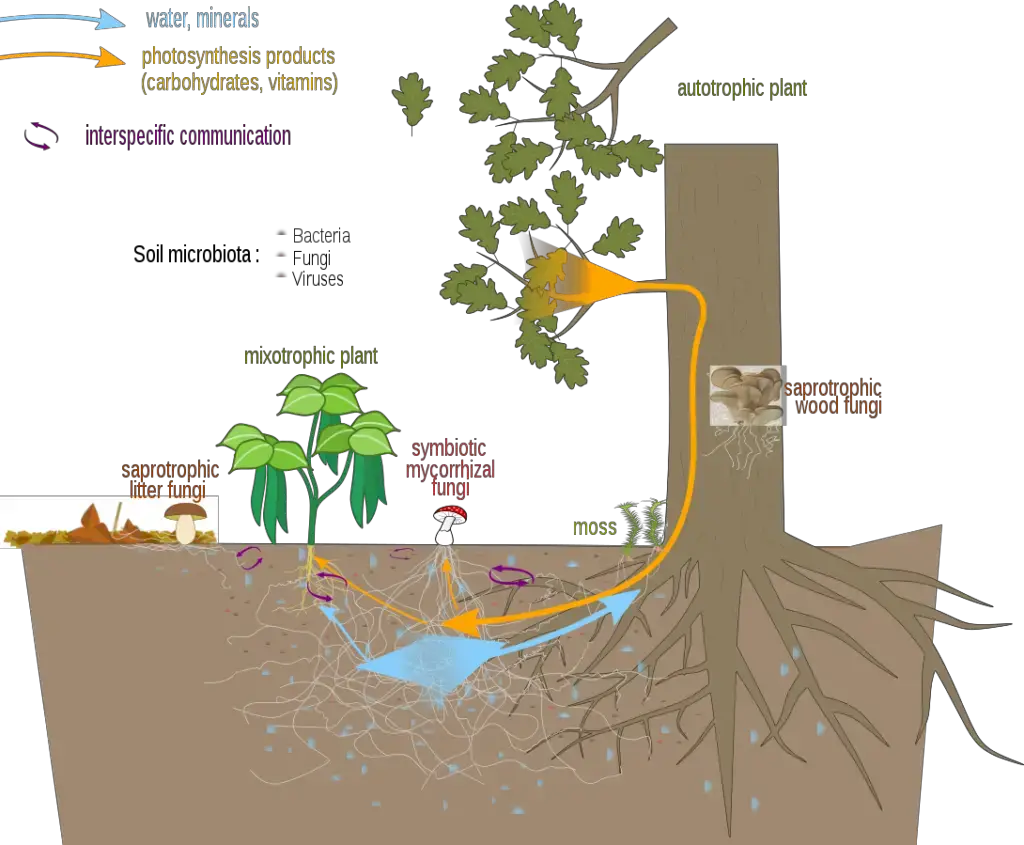
Mycorrhizal associations, the symbiotic relationships between fungi and plant roots, play a crucial role in enhancing plant health, soil quality, and ecosystem stability. The following points outline the significant contributions of mycorrhizae:
- Enhanced Plant Survival Under Stress
- Salinity and Drought Stress: Mycorrhizae improve plant resilience to environmental stresses such as salinity and drought. The fungal networks assist plants in accessing water and nutrients more efficiently, thereby enhancing their ability to withstand adverse conditions.
- Disease Control
- Resistance to Pathogens: Mycorrhizal associations contribute to plant defense mechanisms against soil and root-borne pathogens. The fungi can act as a biological control agent, reducing the incidence of diseases and promoting plant health.
- Soil Conservation and Structure
- Stabilization: Mycorrhizae play a vital role in soil conservation by stabilizing soil structure. The fungal hyphae bind soil particles together, preventing erosion and enhancing soil aggregation.
- Increased Water-Holding Capacity
- Soil Moisture: The presence of mycorrhizae increases the water-holding capacity of the soil. The fungal networks improve soil structure and enhance its ability to retain moisture, which is beneficial for plant growth, especially in arid regions.
- Toxic Compound Avoidance
- Arsenate Uptake: Mycorrhizae help plants avoid the uptake of toxic compounds such as arsenate. This function is crucial in contaminated soils, as it prevents the accumulation of harmful substances in plant tissues.
- Nutrient Uptake Enhancement
- Phosphorus and Other Nutrients: Mycorrhizae are essential for the uptake of phosphorus (P) and other immobile nutrients including zinc (Zn), cobalt (Co), magnesium (Mg), iron (Fe), and copper (Cu). These nutrients are vital for plant growth and development.
- Mineral Weathering: The fungi enhance mineral weathering in the soil, making these nutrients more available to plants.
- Plant Hormone Production
- Hormonal Regulation: Mycorrhizae can stimulate the production of plant hormones such as auxins and cytokinins. These hormones are crucial for the initiation and development of flowers and fruits, thus supporting overall plant growth and reproductive success.
- Optimized Fertilizer Use
- Fertilizer Efficiency: Mycorrhizae improve the efficiency of fertilizer use, particularly phosphorus. By enhancing the plant’s ability to access and utilize phosphorus, mycorrhizae contribute to increased productivity and sustainability in agricultural and horticultural systems.
- Biodiversity Enhancement
- Ecosystem Diversity: The presence of mycorrhizae enhances biodiversity by supporting a variety of plant species in different ecosystems. This diversity contributes to the stability and resilience of ecological communities.
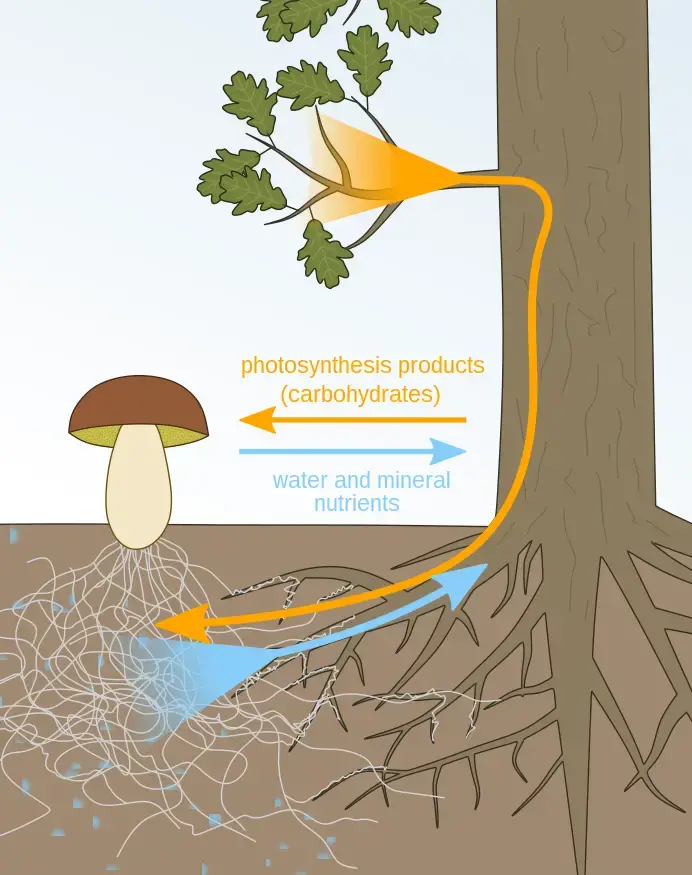
Ecology of Mycorrhizae
Mycorrhizae, symbiotic associations between fungi and plant roots, play a pivotal role in the ecological dynamics of various ecosystems. Their influence spans from nutrient cycling to plant community structuring and even extends to the food web.
- Global Distribution and Soil Nutrient Dynamics: The distribution of mycorrhizal types is intrinsically linked to the global distribution of their respective host plants. However, as postulated by Read, the relationship between mycorrhizal types and soil factors is more profound. In regions characterized by colder climates or higher altitudes, organic matter accumulation is prevalent. Here, nutrient reserves might be abundant, but their bioavailability is limited. Mycorrhizal types, like ericoid mycorrhizae, in these regions possess enzymes that allow them to access organic nitrogen forms directly. In contrast, in temperate regions, ectomycorrhizal communities can access both organic nitrogen and phosphorus. In tropical regions, where nutrient mineralization is rapid, the mycorrhizal community predominantly consists of arbuscular mycorrhizae with limited enzymatic capabilities.
- Influence on Plant Communities: Mycorrhizae play a crucial role in shaping plant communities. The ability of a plant to form mycorrhizal associations and its dependency on these associations can influence its competitive advantage within a community. Bever and Schultz (2005) highlighted that the interplay between plant-mycorrhizal strategies can lead to niche partitioning, thereby maintaining diversity within plant communities. The diversity of the mycorrhizal community often mirrors that of plant species diversity. Moreover, mycorrhizal bridges between plants can facilitate resource sharing, leading to both competitive and synergistic interactions that challenge traditional plant community theories.
- Mycorrhizae as a Food Source: Mycorrhizal fungi, particularly their fruiting bodies, serve as a rich nutrient source for various fauna. Ectomycorrhizal fungi, such as mushrooms, are consumed by invertebrates and small mammals. In Australia, for instance, fungi constitute a significant portion of the diet for several mammalian species. These fungi, especially the hypogeous ones, are nutrient-dense, making them a valuable food resource. Furthermore, the role of small mammals in dispersing fungal spores has been observed to aid in vegetation recovery in areas affected by natural disasters, such as volcanic eruptions. Below the ground, mycorrhizal mycelia serve as food for soil fauna, including nematodes and springtails. However, the impact of soil fauna grazing on mycorrhizal efficiency remains a topic of debate.
Mycorrhizae and Plant Production
Mycorrhizal associations, the symbiotic relationships between fungi and plant roots, have garnered considerable attention for their potential in various fields, including agriculture, commercial forestry, and ecosystem restoration. These associations offer several advantages, from improved plant nutrition to protection against pathogens and stressors. Here, we delve into their applications in these sectors:
- Agriculture: In agricultural settings, the growth-enhancing properties of mycorrhizae have been extensively explored. However, their impact can vary due to the widespread use of fertilizers and the presence of native fungal propagules in the soil. Mycorrhizal associations can enhance plant nutrition by increasing nutrient uptake. Additionally, they provide protective benefits, safeguarding crops against root-feeding nematodes and various bacterial and fungal pathogens. Research has also focused on optimizing methods for mycorrhizal delivery, including incorporating spores into pellets or producing them in matrix materials like rockwool and clay-brick granules. Several companies have developed commercial mycorrhizal inoculum products for use by gardeners, horticulturalists, and forest nurseries.
- Commercial Forestry: The use of mycorrhizal inoculation in commercial forestry has gained attention due to its potential to enhance tree seedling growth. Ectomycorrhizal and arbuscular mycorrhizal inocula are commercially available and are often delivered in perlite-peat mixtures or alginate-entrapped mycelial cultures. These inocula have demonstrated growth benefits in nursery settings and have contributed to the survival and growth of seedlings in challenging environments. For example, ectomycorrhizal fungi like Pisolithus tinctorius have facilitated tree seedling growth on heavy metal-contaminated mine spoils. However, the extent of growth enhancement varies depending on tree species and location, with outplanted seedlings sometimes facing competition from native mycorrhizal flora.
- Restoration: Mycorrhizal associations play a pivotal role in ecosystem restoration projects. They aid in establishing vegetation on nutrient-poor soils typically found in restoration sites. This facilitates the return of organic matter to the soil, improving its health. Moreover, mycorrhizal fungi contribute to soil stability by physically binding soil mineral particles or producing substances like glomalin, which prevent soil erosion. These actions increase soil aggregation and carbon storage within soil aggregates, potentially serving as a means of long-term carbon sequestration. In regions with saline soils resulting from frequent crop irrigation, arbuscular mycorrhizae have been instrumental in enabling crop growth under stressful conditions, allowing continued agricultural production in otherwise challenging areas.
Mycorrhizae and Pollution
Mycorrhizal associations, crucial for plant nutrient uptake and ecosystem health, can be profoundly influenced by various types of pollution. Here, we delve into how mycorrhizae respond to different pollutants, including acidifying substances, heavy metals, radionuclides, organic pollutants, and the impacts of climate change.
- Acidifying Pollutants: Research on acid rain in the late 1970s revealed its detrimental effects on mycorrhizae. Acid rain, primarily composed of sulfuric acid from dissolved SO2 in rain, can affect root growth and alter the ectomycorrhizal status of trees. Acid rain’s impact on mycorrhizae is twofold: (1) It reduces photosynthesis in the tree canopy, diminishing energy supply to roots, and (2) it increases the availability of toxic metal ions (e.g., aluminum, manganese, magnesium) in soil, leading to root damage and changes in the composition of ectomycorrhizal fungal symbionts. These effects disrupt mycorrhizal formation and the associated benefits, such as enhanced nutrient uptake.
- Heavy Metal Pollutants: Heavy metals are toxic to many organisms, including fungi. However, mycorrhizal fungi, especially ectomycorrhizae, exhibit tolerance to heavy metals. Studies have shown that mycorrhizal inoculation can enhance tree survival and growth in polluted environments, with certain fungi like Pisolithus tinctorius reducing the uptake of heavy metals by host trees. The mechanisms behind this protection involve adsorption of heavy metals onto fungal hyphae, preventing their translocation into the host plant’s vascular tissue.
- Radionuclide Pollutants: Mycorrhizal fungi, particularly ectomycorrhizae, have demonstrated an ability to accumulate radionuclides. Research following the Chernobyl nuclear disaster revealed that certain fungi had ten times the concentration of radiocesium compared to surrounding soils. This accumulation is attributed to internal translocation within the mycelium, followed by directional transport to fruiting bodies. Mycorrhizae can enhance radionuclide uptake by host plants while preventing their translocation to shoots, serving as a protective mechanism.
- Organic Pollutants: Mycorrhizal fungi have shown potential in decomposing organic pollutants, such as polychlorinated biphenyls (PCBs). Several mycorrhizal species have been found to degrade PCBs to varying degrees. While the interactions between mycorrhizal fungi, rhizospheric bacteria, and organic pollutant decomposition are still emerging fields of study, there is evidence of fungal involvement in mitigating organic pollution.
- Climate Change: Climate change factors, including increased atmospheric CO2 levels, rising temperatures, and altered rainfall patterns, can influence mycorrhizal communities. Elevated CO2 levels impact the C:nutrient ratio of plant material entering decomposition pathways, altering nutrient availability and affecting mycorrhizal community composition. Research on the responses of mycorrhizae to climate change-related factors, such as ozone, UV light, and altered rainfall, is ongoing.
- Fungal Conservation: Long-term fungal records have revealed shifts in fungal populations, with ectomycorrhizal species declining in dominance. This change has been attributed to acidifying pollutants, particularly nitrogen deposition. The decline in fungal species has raised concerns about fungal conservation, prompting efforts to maintain habitats for declining fungal species and assess the impact of mushroom harvesting on fungal decline. Forest management practices may also influence the composition of ectomycorrhizal fungal flora.
Benefits of Mycorrhiza
Mycorrhiza, the symbiotic association between fungi and plant roots, offers a wide range of benefits to both plants and ecosystems. These benefits are crucial for nutrient uptake, plant health, and overall ecosystem functioning. Here are some of the key advantages of mycorrhizal associations:
- Improved Nutrient Uptake:
- Mycorrhizal fungi extend their hyphae into the soil, increasing the root’s capacity to absorb essential nutrients such as phosphorus, nitrogen, and micronutrients.
- They can access nutrient sources that are otherwise unavailable to plants, enhancing nutrient acquisition efficiency.
- Enhanced Water Uptake:
- Mycorrhizal networks can extend deep into the soil, accessing water in deeper layers during drought conditions, which helps plants maintain adequate hydration.
- Disease Resistance:
- Mycorrhizal associations can enhance a plant’s resistance to soil-borne pathogens by forming physical barriers between pathogens and roots.
- Some mycorrhizal fungi also produce antimicrobial compounds that inhibit the growth of harmful microorganisms.
- Improved Plant Growth:
- Mycorrhizal fungi stimulate plant growth by facilitating nutrient uptake and increasing the availability of water.
- Enhanced growth can lead to improved crop yields in agriculture and healthier, more robust plants in natural ecosystems.
- Stress Tolerance:
- Mycorrhizal plants are often more resilient to environmental stressors, such as drought, salinity, and heavy metal contamination, due to their improved nutrient and water uptake capabilities.
- Ecosystem Restoration:
- Mycorrhizal fungi play a critical role in the restoration of degraded ecosystems. They help establish vegetation on poor soils, stabilize soil structure, and sequester carbon.
- Increased Biodiversity:
- Mycorrhizal associations are associated with greater plant diversity, as they enable a wider range of plant species to thrive by accessing limited soil resources.
- Carbon Sequestration:
- Mycorrhizal fungi store carbon in soil organic matter, contributing to carbon sequestration, which is essential for mitigating climate change.
- Support for Non-Photosynthetic Plants:
- Mycorrhizal networks can connect different plant species, allowing non-photosynthetic plants (mycoheterotrophs) to obtain carbon and nutrients from nearby photosynthetic plants.
- Improved Soil Structure:
- Mycorrhizal fungi produce extracellular substances like glomalin, which help bind soil particles together, enhancing soil structure, and preventing erosion.
- Increased Ecosystem Productivity:
- Mycorrhizal associations contribute to overall ecosystem productivity by promoting the growth of plants, which, in turn, support higher trophic levels.
- Reduced Fertilizer Dependency:
- In agriculture, mycorrhizal associations can reduce the need for synthetic fertilizers by enhancing nutrient uptake efficiency, potentially reducing production costs and environmental impacts.
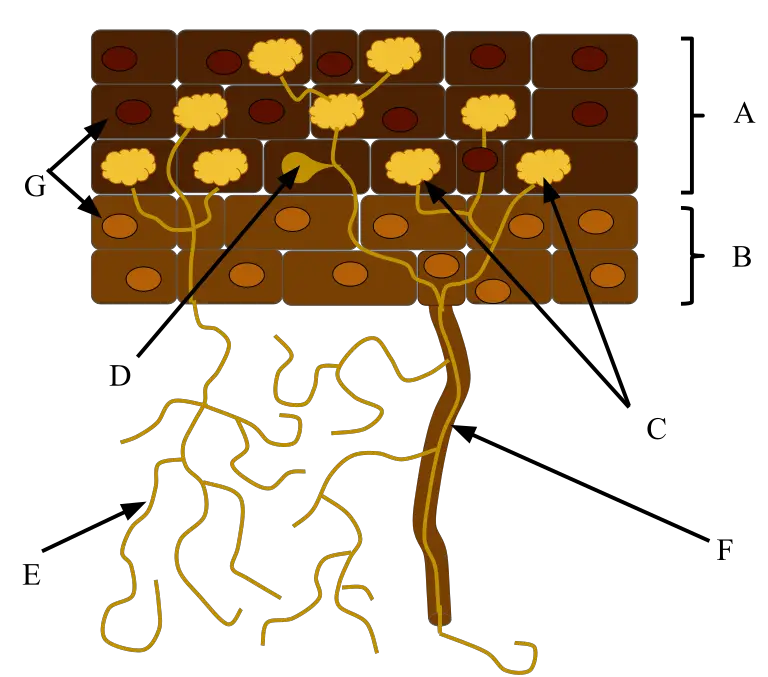
Examples of Mycorrhiza
Mycorrhiza, the symbiotic associations between fungi and plant roots, exhibit various types and are found in a range of plant species across diverse ecosystems. These associations play critical roles in nutrient uptake, plant health, and ecosystem functioning. Here are some examples of mycorrhiza:
- Orchid Mycorrhiza:
- Orchids, particularly those that are non-photosynthetic or have limited photosynthesis during their early growth stages, form mycorrhizal associations with specific fungi.
- Orchid seeds depend on fungal invasion for germination, as they cannot acquire sufficient nutrients independently.
- Orchidaceous mycorrhiza involves the formation of hyphal coils (pelotons) within the root cells, facilitating nutrient exchange between the fungus and the orchid.
- Arbuscular Mycorrhiza:
- Arbuscular mycorrhizae are among the most widespread mycorrhizal associations, commonly found in many plant species.
- Fungi involved in arbuscular mycorrhiza are typically members of the Zygomycota family.
- These fungi form arbuscules, which are intricate structures within root cells that serve as sites for nutrient exchange, particularly for phosphorus, carbon, and water.
- Arbuscular mycorrhizae are considered obligate symbionts, as the fungi cannot thrive without their plant hosts.
- Ericaceous Mycorrhiza:
- Ericaceous mycorrhizae are found in plants belonging to the Ericales order, often in acidic environments.
- These mycorrhizae help regulate the plant’s acquisition of minerals such as iron, manganese, and aluminum.
- While they do penetrate root cells, they do not form arbuscules. Instead, they create hyphal coils outside of root cells, significantly increasing root volume.
- Arbutoid Mycorrhiza:
- Arbutoid mycorrhiza is a type of endomycorrhizal association that resembles ectomycorrhizal fungi in appearance.
- Fungi involved in this association form a fungal sheath around plant roots and penetrate cortical cells.
- This differs from ectomycorrhizal fungi, which do not penetrate root cells.
- Ectotrophic Mycorrhiza:
- Ectotrophic mycorrhizae are formed between fungi belonging to the Ascomycota and Basidiomycota families and many trees in cooler environments.
- Unlike wood-rotting fungi from the same families, ectotrophic mycorrhizal fungi do not degrade plant materials.
- Instead, they derive nutrients and sugars from the living roots of their host plants.
Mycorrhiza Lecture Video
Quiz
What is mycorrhiza?
A. A type of plant disease
B. A symbiotic association between fungi and plant roots
C. A mineral-rich soil
D. A type of plant hormone
Which part of the plant is primarily involved in mycorrhizal associations?
A. Leaves
B. Stems
C. Roots
D. Flowers
What is the main benefit of mycorrhizal associations for plants?
A. Increased water absorption
B. Enhanced photosynthesis
C. Improved disease resistance
D. Enhanced nutrient uptake
Which type of mycorrhiza is known for its high affinity for phosphorus?
A. Arbuscular mycorrhiza
B. Ectotrophic mycorrhiza
C. Ericaceous mycorrhiza
D. Orchid mycorrhiza
What do arbuscules represent in arbuscular mycorrhizal associations?
A. Fungal spores
B. Root hairs
C. Sites of nutrient exchange
D. Protective structures
Which of the following mycorrhizal types is commonly found in acidic environments?
A. Ectotrophic mycorrhiza
B. Arbutoid mycorrhiza
C. Orchid mycorrhiza
D. Ericaceous mycorrhiza
What is the primary role of ericaceous mycorrhiza in plant nutrition?
A. Enhancing photosynthesis
B. Regulating water uptake
C. Facilitating mineral absorption
D. Promoting root growth
In which type of mycorrhizal association do fungi form a sheath around plant roots?
A. Ectotrophic mycorrhiza
B. Arbuscular mycorrhiza
C. Orchid mycorrhiza
D. Ericaceous mycorrhiza
Which fungal families are typically involved in ectotrophic mycorrhizal associations?
A. Zygomycota
B. Ascomycota and Basidiomycota
C. Glomeromycota
D. Chytridiomycota
What is the ecological significance of mycorrhizal associations?
A. They promote fungal diseases in plants
B. They reduce plant growth and productivity
C. They contribute to nutrient cycling and ecosystem health
D. They have no impact on ecosystems
FAQ
What is mycorrhiza?
Answer: Mycorrhiza is a symbiotic association between fungi and the roots of plants. It involves a mutually beneficial exchange of nutrients between the two organisms.
What is the role of mycorrhizal fungi in plants?
Answer: Mycorrhizal fungi help plants by enhancing their nutrient and water uptake capabilities. They also improve the plant’s resistance to diseases and environmental stress.
How does the symbiosis between mycorrhizal fungi and plants work?
Answer: Mycorrhizal fungi form a network of fine threads (hyphae) that extend into the soil, increasing the root’s surface area. This allows the plant to access more nutrients and water, while the fungi receive sugars produced by the plant through photosynthesis.
Are mycorrhizal associations beneficial for all plants?
Answer: Mycorrhizal associations are beneficial for most plants, especially in nutrient-poor soils. However, some plants have evolved to grow without mycorrhizal partners.
What are the different types of mycorrhizae?
Answer: There are several types of mycorrhizae, including arbuscular mycorrhizae, ectotrophic mycorrhizae, ericaceous mycorrhizae, and orchid mycorrhizae, each forming unique associations with plants.
Can mycorrhizal fungi protect plants from diseases?
Answer: Yes, mycorrhizal fungi can enhance a plant’s resistance to diseases by competing with harmful pathogens for resources and producing antimicrobial compounds.
How do I know if my soil has mycorrhizal fungi?
Answer: Mycorrhizal fungi are present in most soils. You can encourage their growth by maintaining healthy, diverse plant communities and avoiding excessive use of synthetic fertilizers.
Can mycorrhizal fungi be used in agriculture?
Answer: Yes, mycorrhizal inoculants are used in agriculture to improve crop yields and reduce the need for synthetic fertilizers. They can be applied to the roots of plants during transplantation.
Do mycorrhizal associations have an impact on ecosystems?
Answer: Yes, mycorrhizal associations play a crucial role in nutrient cycling, soil health, and plant diversity within ecosystems. They contribute to the overall ecological balance.
Can mycorrhizae help mitigate climate change?
Answer: Mycorrhizal fungi can assist in carbon sequestration by improving soil structure and storing carbon in soil aggregates. This has the potential to contribute to climate change mitigation efforts.
- Egerton-Warburton, L. M., Querejeta, J. I., Allen, M. F., & Finkelman, S. L. (2013). Mycorrhizal Fungi. Reference Module in Earth Systems and Environmental Sciences. doi:10.1016/b978-0-12-409548-9.05226-x
- Dighton, J. (2009). Mycorrhizae. Encyclopedia of Microbiology, 153–162. doi:10.1016/b978-012373944-5.00327-8
- https://www.slideshare.net/slideshow/mycorrhiza-235816364/235816364
- https://www.slideshare.net/slideshow/mycorrhizae-234539847/234539847
- Egerton-Warburton, L. M., Querejeta, J. I., Allen, M. F., & Finkelman, S. L. (2005). MYCORRHIZAL FUNGI. Encyclopedia of Soils in the Environment, 533–542. doi:10.1016/b0-12-348530-4/00455-0
- Egerton-Warburton, L. M., Querejeta, J. I., Allen, M. F., & Finkelman, S. L. (2013). Mycorrhizal Fungi. Reference Module in Earth Systems and Environmental Sciences. doi:10.1016/b978-0-12-409548-9.05226-x
- Bonfante, Paola & Anca, Iulia-Andra. (2009). Plants, Mycorrhizal Fungi, and Bacteria: A Network of Interactions. Annual review of microbiology. 63. 363-83. 10.1146/annurev.micro.091208.073504.
- Devi, S. H. , Bhupenchandra, I., Sinyorita, S., Chongtham, S., & Devi, E. L. (2021). Mycorrhizal Fungi and Sustainable Agriculture. In T. Ohyama, & K. Inubushi (Eds.), Nitrogen in Agriculture – Physiological, Agricultural and Ecological Aspects. IntechOpen. https://doi.org/10.5772/intechopen.99262
- https://people.unil.ch/iansanders/mycorrhizas/
- https://collegedunia.com/exams/mycorrhiza-biology-articleid-1551
- https://soils.ifas.ufl.edu/soil-microbial-ecology/mycorrhizal-services/
- https://www.gardenorganic.org.uk/mycorrhizal-fungi
- https://biologydictionary.net/mycorrhizae/
- https://mycorrhizae.com/how-it-works/
- https://www.groundworkbioag.com/mycorrhiza/
- https://www2.nau.edu/~gaud/bio300/mycorrhizae.htm
- https://untamedscience.com/biology/ecology/mycorrhizae/
- https://arboretum.harvard.edu/stories/food-poison-and-espionage-mycorrhizal-networks-in-action/
- https://www.spun.earth/networks/mycorrhizal-fungi
- https://en.wikipedia.org/wiki/Mycorrhiza
What is the difference in structure between Ericoid and Orchid mycorrhizal fungi? How are the mycelium coils different?
Ericoid and orchid mycorrhizal fungi differ in how they interact with plant roots. Ericoid fungi make tight hyphal coils inside fine hair root cells, mostly in acidic, low-nutrient soils. These coils help break down organic matter for nutrient exchange. Orchid fungi, on the other hand, form dense, coiled pelotons in root cortical cells. These structures are key for orchid seed germination and early growth. The two types have distinct intracellular structures suited to their plant hosts and environments.