What is Mycobacterium leprae?
- Mycobacterium leprae is the bacterium responsible for causing Hansen’s disease, also known as leprosy. This chronic infectious disease primarily affects the peripheral nerves, skin, eyes, and muscles. Discovered by Norwegian physician Gerhard Armauer Hansen in 1873, M. leprae was the first bacterium identified as the cause of a human disease. Though once misunderstood, research over the last century has greatly advanced our understanding of the pathogenesis, causes, and treatment of leprosy.
- M. leprae is an acid-fast, Gram-positive bacterium that is rod-shaped with a distinctive waxy coat due to the presence of mycolic acids. Like other mycobacteria, it cannot be cultured in artificial media because it is an obligate intracellular parasite, depending on host cells for nutrients and metabolic functions. This limitation is likely due to a process of reductive evolution, where the bacterium’s genome has been reduced over time. Its narrow host range is primarily restricted to humans, though other natural hosts include nine-banded armadillos and red squirrels. The bacterium typically infects macrophages and Schwann cells, where it forms palisade structures or clumps known as globi within granulomatous lesions.
- When examining M. leprae under the microscope, the bacteria can be found either singly or in clusters within the infected cells. These clusters are often seen in the nodules formed in lepromatous leprosy, where the bacteria are especially abundant. M. leprae is sensitive to certain stains like carbol fuchsin, and can be identified through special staining methods like Fite-Faraco, which is more suitable than the traditional Ziehl-Neelsen method used for other acid-fast bacteria, such as Mycobacterium tuberculosis.
- The bacterium thrives at temperatures between 27 to 30°C, making the cooler regions of the human body—such as the skin, nasal mucosa, and peripheral nerves—ideal sites for infection. This temperature preference contributes to the specific tissues affected by the disease.
- Historically, the treatment of leprosy was limited. Dapsone was initially effective, but by the 1960s, M. leprae had developed resistance to it. Today, the World Health Organization recommends a multidrug therapy regimen that includes dapsone, rifampicin, and clofazimine. This treatment approach has been successful in managing the disease, although the stigma associated with leprosy remains a significant social issue in some regions.
- M. leprae’s ability to evade full detection and treatment for extended periods of time can lead to severe, irreversible damage to affected tissues. The persistence of the bacterium in the body, coupled with the immune response it triggers, explains the gradual progression of symptoms and the chronic nature of the disease. Understanding the biology of M. leprae has been crucial in improving diagnostic methods and treatment options, which have significantly reduced the global burden of leprosy.
Scientific classification of Mycobacterium leprae
Domain: | Bacteria |
Phylum: | Actinomycetota |
Class: | Actinomycetia |
Order: | Mycobacteriales |
Family: | Mycobacteriaceae |
Genus: | Mycobacterium |
Species: | M. leprae |
Geographical Distribution and Habitat of Leprosy
Leprosy is a disease with a varied global presence. It is most commonly found in specific regions, with a significant concentration in Southeast Asia, Africa, and parts of the Americas. The disease’s prevalence, though low globally, remains high in certain endemic countries.
Geographical Distribution
- Global Prevalence:
- Leprosy affects roughly 1 in 10,000 people worldwide.
- Despite this, most cases are concentrated in specific regions, particularly in Southeast Asia, Africa, and the Americas.
- Endemic Countries:
- Around 15 countries across these regions report high rates of leprosy, with a prevalence of about 1 case per 10,000 population.
- Brazil, Angola, Central African Republic, Congo, Madagascar, Mozambique, Tanzania, Nepal, and India are the primary hotspots, accounting for 74% of global cases.
- Regional Trends:
- Lepromatous leprosy is more commonly seen in Africa, while tuberculoid leprosy is more prevalent in Asia.
- In India, leprosy is widespread across all states and territories. Some regions like Orissa and Bihar report higher rates, with over 5 cases per 1,000 population, while Haryana reports one of the lowest, at 0.1 per 1,000 population.
- Decline in Prevalence:
- Since the introduction of short multidrug therapy in 1982, the global prevalence of leprosy has significantly decreased.
Habitat
- Infected Host:
- Mycobacterium leprae, the bacteria causing leprosy, is primarily found in large numbers in the nasal secretions of individuals with lepromatous leprosy.
- This highlights the significance of nasal secretions in the transmission and spread of the disease.
Morphology of Mycobacterium leprae
Mycobacterium leprae, the bacterium responsible for leprosy, has a distinct morphology that plays a crucial role in its identification and behavior. Understanding its shape, staining properties, and intracellular characteristics is essential for diagnosing and studying this pathogen.
- Shape and Size:
- M. leprae is a rod-shaped bacterium, with straight or slightly curved bodies.
- The bacilli have parallel sides and rounded ends, measuring between 1–8 micrometers in length and 0.2–0.5 micrometers in diameter.
- This bacterium shows considerable morphological variations, including cubical, lateral, and even branching forms.
- Staining and Acid-Fast Properties:
- M. leprae is Gram-positive and more readily stained than Mycobacterium tuberculosis.
- When stained with carbol fuchsin, it is less acid-fast compared to M. tuberculosis, which is why a 5% sulfuric acid solution is used for decolorization, rather than the stronger 20% sulfuric acid used for other mycobacteria.
- Intracellular Characteristics:
- M. leprae is an obligate intracellular bacterium, meaning it can only survive and multiply inside host cells.
- It primarily infects cells at cooler temperatures, which explains its tendency to target tissues like the skin, peripheral nerves, and nasal mucosa.
- The bacilli are typically found singly or in groups inside infected cells and free outside the cell. When inside the host cells, the bacteria often form parallel bundles of 50 or more bacilli.
- These bacteria can also form masses called globi, which are bundles of bacilli held together by a lipid-like substance that gives them a “cigar bundle” appearance.
- Specialized Cell Forms:
- M. leprae is often seen in the Virchow’s lepra cells, also known as foamy cells. These cells, which have a foamy appearance, house the globi and are typically large, undifferentiated histiocytes.
- The parallel arrangement of the bacilli within the globi contributes to the formation of this distinctive structure.
- Non-motile and Non-sporing:
- M. leprae is nonmotile and does not form spores, which distinguishes it from other bacteria that exhibit these behaviors.
Transmission of Mycobacterium leprae
The transmission of Mycobacterium leprae, the causative agent of leprosy, remains an area of ongoing research. While the exact mechanisms are not fully understood, several routes and risk factors have been identified.
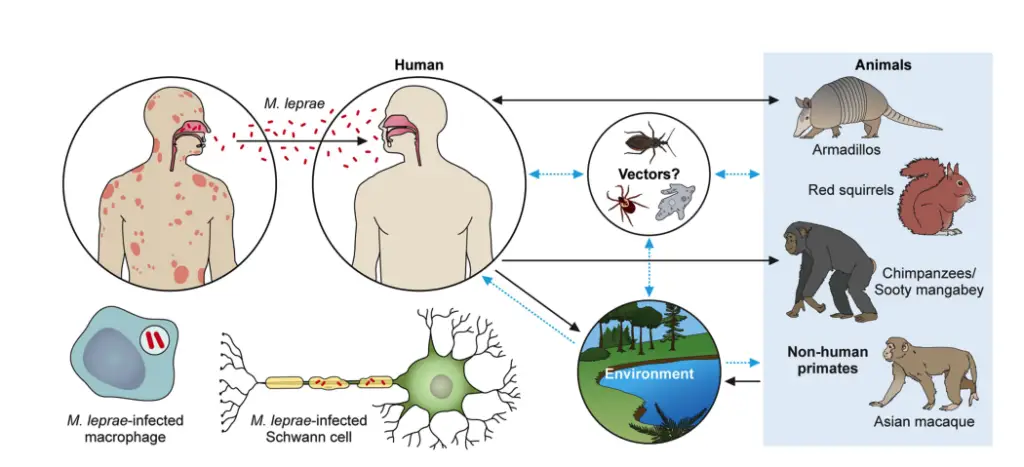
- Prolonged Contact and Overcrowding
- The most commonly suggested risk factors for M. leprae transmission are prolonged contact with infected individuals and environments characterized by overcrowding.
- These conditions likely increase the chance of close, sustained exposure to the bacterium, facilitating its spread.
- Role of the Respiratory Tract
- The respiratory tract is thought to play a potential role in the transmission of M. leprae.
- Studies have shown that a high number of bacterial cells—up to 100 million cells per day—can be found in the nasal mucosa of infected individuals. This suggests that respiratory droplets may be a significant vector for spreading the disease.
- Mother-to-Child Transmission
- Transmission from mother to child during pregnancy has also been documented, making it crucial to carefully monitor pregnant women diagnosed with leprosy to prevent vertical transmission.
- Skin and Fomites as Transmission Routes
- The skin is another potential route for transmission. M. leprae bacilli can survive outside the body for up to 9 days, indicating that direct contact with contaminated skin could contribute to the spread.
- While fomites—inanimate objects contaminated with the bacteria—have been suggested as a possible transmission source, this hypothesis has yet to be confirmed by conclusive evidence.
- Tattooing as a Possible Route
- It has also been reported that leprosy could be transmitted through the practice of tattooing, possibly due to shared equipment or contamination during the process.
Culture of Mycobacterium leprae
Culturing Mycobacterium leprae in vitro remains an unsolved challenge. Unlike many other bacteria, M. leprae cannot be grown in artificial media or tissue cultures, limiting the development of traditional laboratory cultures for study.
- Inability to Culture in Media:
- M. leprae has not been successfully cultured in bacteriological media or tissue cultures.
- Efforts to grow the bacteria in cell-free environments have failed due to its obligate intracellular nature.
- ICRC Bacillus:
- The ICRC bacillus, isolated in 1962 from a leprosy patient, was initially thought to be related to M. leprae.
- It was cultured using human fetal spinal ganglion cell culture and later adapted to grow on Lowenstein–Jensen (LJ) medium.
- However, studies have questioned whether this bacillus is truly M. leprae or a variant of Mycobacterium avium intracellular group.
- Experimental Animal Models: Since culturing M. leprae in vitro is not possible, animal models have been developed for research and experimentation.
- Footpads of Mice:
- The footpad of mice has been used since 1960 as a model for culturing M. leprae.
- When inoculated with lepra bacilli, the footpads develop granulomas within 1-6 months.
- This model is crucial for testing antileprosy drugs, including determining the maximum required concentrations and evaluating bacillary sensitivity to new treatments.
- Thymectomized Mice:
- In thymectomized mice, where cell-mediated immunity (CMI) is suppressed, the introduction of M. leprae leads to a generalized infection.
- This model is used to study lepromatous leprosy and to detect persistent infections even after chemotherapy treatment.
- Nine-Banded Armadillo:
- The nine-banded armadillo is highly susceptible to M. leprae, developing generalized infections and extensive bacterial multiplication.
- M. leprae in armadillos causes lesions typical of lepromatous leprosy and can survive in these animals for up to 400 days.
- This animal is an invaluable source for genetic studies and the development of a leprosy vaccine.
- In Texas and Mexico, wild armadillos have been observed to carry M. leprae-like bacteria, further demonstrating the armadillo’s role in leprosy research.
- Other Animal Models:
- Other animals, such as the slender loris, Indian pangolin, and Korean chipmunks, have been used for experimental infection with M. leprae, although the armadillo remains the primary model.
- Footpads of Mice:
- Slow Growth:
- M. leprae has an exceptionally slow growth rate, with a generation time of 12-13 days in infected mice.
- In comparison, M. tuberculosis doubles in about 14 hours, and coliform bacilli have a generation time of just 20 minutes. This slow growth makes studying the bacterium even more challenging.
Cell Wall Components and Antigenic Structure of Mycobacterium leprae
The cell wall of Mycobacterium leprae plays a crucial role in its structure, immune evasion, and interaction with the host. It consists of distinct layers, each contributing to the bacterium’s function and pathogenesis.
- Cell Wall Structure:
- Peptidoglycan: The innermost layer provides structural rigidity and shape to the bacterium.
- Lipoarabinomannose-B (LAM-B): This layer sits next to the peptidoglycan, contributing to the cell’s stability and interaction with the immune system.
- Mycolic Acid: A dense, long-chain fatty acid layer forms a palisade attached to the LAM-B membrane. This layer is characteristic of mycobacteria and crucial for their pathogenicity.
- Mycosides and Phenolic Glycolipid (PGL)-1: The outermost layer contains mycosides, with PGL-1 being a key antigenic component. PGL-1 is highly immunogenic and shields the bacterium from immune attacks, particularly by cell-mediated immunity (CMI).
- Antigenic Structure:
- The antigenic components of M. leprae play a vital role in both immune response and immune evasion.
- Lipoarabinomannose-B (LAM-B) is a major antigen of M. leprae and is highly immunogenic, stimulating high levels of serum antibodies. It differs from the LAM found in M. tuberculosis, highlighting its unique antigenic properties.
- Phenolic Glycolipid (PGL)-1, located in the outermost layer, serves as the primary antigen and contributes to immune modulation. It suppresses cell-mediated immunity (CMI) and protects M. leprae from host defenses, making it more difficult for the immune system to clear the infection.
- Protein Antigens:Mycobacterium leprae contains a variety of protein antigens that contribute to both humoral and cell-mediated immune responses.
- 65-kDa Heat Shock Protein: This protein induces a humoral antibody response early in infection, and the antibody levels remain elevated throughout the disease stages, including during the reactional stage.
- 28-kDa Protein: This protein is identified as the superoxide dismutase of M. leprae. It triggers a stronger antibody response in individuals with lepromatous leprosy compared to those with tuberculoid leprosy, with the response being especially heightened during type 1 reactions.
- 18-kDa Protein: Considered one of the most crucial antigens, it induces both humoral and cell-mediated responses. This antigen is recognized by individuals with lepromatous leprosy and other forms of the disease (except for tuberculoid leprosy), leading to high antibody levels in their serum.
The Structure of the M. leprae Cell Wall
The cell wall of Mycobacterium leprae is intricate, serving as a protective layer and a key player in host-pathogen interactions. Its multi-layered structure includes components critical for its functionality and pathogenicity.
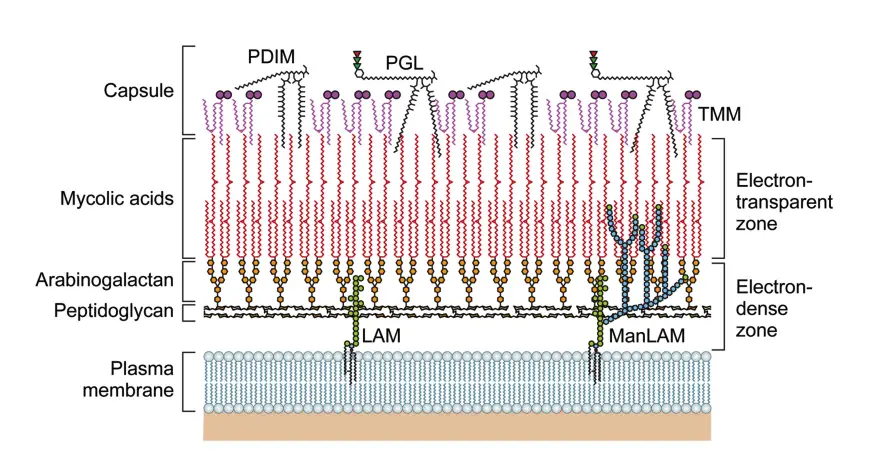
Key Features of the M. leprae Cell Wall:
- Outer Layer:
- Composed of phenolic glycolipids (PGLs), including phthiocerol dimycocerosate (PDIM) and PGL-I.
- Includes lipid-linked polysaccharides such as lipomannan (LM) and lipoarabinomannan (LAM).
- Contains dimycolyl trehalose and small amounts of trehalose mono-mycolate (TMM).
- Inner Layer:
- Electron-dense and rigid, with peptidoglycan (PGN), arabinogalactan (AG), and mycolic acids.
- Features a pseudo-lipid bilayer with an inner leaflet of mycolic acids linked to arabinan termini and an outer leaflet of PGLs and other lipids.
- Mycolic Acid Content:
- Higher in M. leprae compared to M. tuberculosis (ratio of 21:10 versus 16:10 when compared to PGN).
Phenolic Glycolipid-I (PGL-I):
- Structure:
- Contains a triglycosyl unit with 3,6-di-O-methylglucose, 2,3-di-O-methylrhamnose, and 3-O-methylrhamnose linked to phenol-PDIM.
- Functionality:
- Facilitates interaction with the host cell membrane.
- Suppresses inflammatory cytokine secretion.
- Plays a role in Schwann cell adhesion and invasion, promoting neural damage.
- Used in serodiagnosis due to high antibody responses against PGL-I trisaccharide units in lepromatous leprosy (LL).
Lipoarabinomannan (LAM):
- Composition:
- Includes a mannosyl-phosphatidyl-myo-inositol (MPI) anchor, a polysaccharide backbone, and variable capping moieties.
- Types:
- Mannose-capped LAM (ManLAM) is the primary type in M. leprae.
- Immunomodulatory Roles:
- Suppresses T-cell activation.
- Modulates macrophage gene expression induced by interferon-gamma (IFN-γ).
- Inhibits protein kinase C and scavenges oxygen radicals.
- Induces cytokines like tumor necrosis factor-alpha (TNF-α).
Peptidoglycan (PGN):
- Structure:
- Chemotype IV group, with peptide side chains containing diaminopimelic acid (DAP).
- Contains N-glycolylmuramic acid (MurNGlyc) and amidated DAP residues.
- Immune Evasion:
- Recognized by NOD-like receptors (NLRs), initiating immune responses.
- Features like amidated DAP and glycine residues reduce recognition by NOD1 and NOD2, helping the bacteria evade immune responses.
Virulence Factors of Mycobacterium leprae
The virulence of Mycobacterium leprae is primarily linked to its ability to survive and multiply within host cells, particularly macrophages and Schwann cells. The bacterium’s persistence and pathogenicity are shaped by several key factors.
- Intracellular Survival:
- M. leprae is an obligate intracellular pathogen, meaning it can only grow inside host cells.
- Its preference for macrophages and Schwann cells enables it to evade the immune system, particularly by hiding within these cells where it is less accessible to direct immune responses.
- Phenolic Glycolipid-1 (PGL-1):
- PGL-1 is a prominent surface lipid found on the outermost layer of the bacterium’s cell wall.
- This lipid is unique to M. leprae and plays a significant role in the bacterium’s ability to evade the immune system.
- PGL-1 binds to the C3 component of the host’s complement system. This interaction facilitates phagocytosis by receptors like CR1, CR3, and CR4 found on cell surfaces, allowing M. leprae to be engulfed by immune cells.
- Once inside phagocytic cells, PGL-1 helps protect M. leprae from the oxidative killing mechanisms of macrophages by neutralizing harmful molecules such as hydroxyl radicals and superoxide anions.
- This ability to prevent oxidative damage allows M. leprae to survive longer within the host, contributing to chronic infection.
- Immune Modulation:
- The bacterium’s intracellular location is a major factor in its resistance to immune clearance. Once inside the host cells, M. leprae is shielded from the direct effects of immune responses such as phagocytosis and oxidative killing, making it difficult for the body to clear the infection.
- The bacterium’s interaction with the immune system influences the clinical presentation of leprosy. The severity and type of the disease are influenced by the host’s immune response, with variations between lepromatous and tuberculoid forms of the disease.
Pathogenicity of Mycobacterium leprae
The pathogenicity of Mycobacterium leprae is tightly linked to its ability to invade and manipulate host cell systems, particularly within the peripheral nervous system (PNS). This bacterium’s affinity for Schwann cells and its sophisticated methods of cellular interaction allow it to persist and spread, making leprosy a complex and insidious infection.
- Invasion of Schwann Cells
- M. leprae specifically targets Schwann cells, which are responsible for myelinating nerves in the PNS.
- The bacterium binds to the basal lamina of Schwann cells, an essential structure that surrounds axons and facilitates signal transmission.
- The key component for bacterial attachment is laminin-2, particularly the α2 chain. This chain plays a crucial role in the specificity of M. leprae’s binding to Schwann cells.
- M. leprae also interacts with α-dystroglycan, a receptor on Schwann cells, to facilitate its entry. The binding of the bacterium to the G domain of laminin α2 creates a bridge, ensuring the bacterium’s survival and entry into cells.
- Role of PGL-1 in Pathogenicity
- M. leprae’s cell wall contains phenolic glycolipid-1 (PGL-1), a unique glycolipid that is critical to its pathogenicity.
- PGL-1, which contains a trisaccharide specific to M. leprae, is responsible for the bacterium’s affinity for Schwann cells and other neural tissues.
- The trisaccharide component of PGL-1 binds specifically to the G domain of laminin α2, which is abundantly expressed in Schwann cells. This enhances the bacteria’s ability to attach to and infect these cells.
- Disruption of Schwann Cell Function
- Once inside Schwann cells, M. leprae induces changes that disrupt normal cellular function, including the breakdown of myelin.
- M. leprae interacts with ErbB2, a receptor involved in Schwann cell signaling, bypassing other pathways that normally regulate cellular activity. This interaction triggers demyelination, a key feature of nerve damage in leprosy.
- The bacterium further exploits existing Erk1/2 signaling pathways, causing damage to nerve cells and promoting myelin breakdown.
- Schwann Cell Reprogramming
- M. leprae has the ability to reprogram Schwann cells, forcing them into a stem-like state. This allows the bacterium to spread to other tissues, such as muscle fibers, which also serve as a niche for M. leprae.
- Reprogrammed Schwann cells are more effective at propagating the infection compared to non-reprogrammed cells. This increases the bacterium’s ability to persist in the host.
- Macrophage Modulation
- The bacterium also manipulates the immune system, specifically the function of macrophages.
- When infected by M. leprae, macrophages adopt an M2-like phenotype, which supports the survival of the bacterium by suppressing immune responses. This includes decreased production of pro-inflammatory cytokines like IL-6 and TNF-α, while promoting the production of anti-inflammatory IL-10.
- This shift in macrophage behavior helps the bacterium evade immune clearance and persist within the host.
- Impact on T Cell Responses
- Infected macrophages are also able to alter T cell responses, especially by promoting the development of regulatory T cells (Tregs). These Tregs suppress Th1 immune responses, which are normally responsible for fighting infections like leprosy.
- M. leprae’s influence on the immune system helps it avoid detection and destruction by the host’s CD8+ T cells, enhancing its long-term survival.
Classification of Leprosy
Leprosy is classified into different forms based on clinical, histopathological, and immunological findings. The classification system introduced by Ridley and Jopling in 1966 helps differentiate the types of leprosy and assists in predicting the disease’s progression and response to treatment. The primary categories are:
- Tuberculoid Leprosy (TT):
- Found in patients with a strong cell-mediated immune response (CMI).
- Bacilli are scanty or absent in the lesions, which makes this form less infectious.
- Lepromin test results are positive, indicating good immunity against the infection.
- Tuberculin sensitivity is also positive.
- The humoral immune response is weak, so antibodies in the serum are rare.
- Skin lesions are limited and the disease has a generally good prognosis.
- Borderline Tuberculoid Leprosy (BT):
- This type represents transitional forms of leprosy, showing characteristics of both tuberculoid and lepromatous forms.
- The disease may progress towards either tuberculoid or lepromatous lesions, depending on the individual’s immune response.
- The lesions may heal spontaneously in about 75% of affected individuals.
- Lepromin test is variable, reflecting the immune status of the individual.
- Borderline Leprosy (BB):
- A more equivocal form of the disease where lesions can show features of both ends of the spectrum.
- The immune response is neither strong nor weak, and the disease presents an unpredictable course.
- As with other borderline types, the immune system’s strength influences the disease’s progression.
- Borderline Lepromatous Leprosy (BL):
- This form is closer to lepromatous leprosy but may retain some immune response from the tuberculoid side.
- The disease may become more severe over time, progressing towards lepromatous leprosy in some cases.
- Lepromin test may be weak or negative.
- Lepromatous Leprosy (LL):
- Seen in individuals with a weak or absent cell-mediated immune response (CMI).
- The disease is highly infectious, with large numbers of lepra bacilli present in the cells and tissues. This is referred to as multibacillary disease.
- Lepromin test is negative, indicating the absence of an immune response to the infection.
- Tuberculin sensitivity may be suppressed or negative initially, but may become positive once the patient is treated.
- Humoral immunity is strong in lepromatous leprosy, with high levels of antibodies in the serum. Autoantibodies are also commonly found.
- The skin lesions tend to be bilaterally symmetrical and nonanesthetic.
- Purely Neuritic Leprosy:
- This is a recently described form of leprosy characterized by asymptomatic peripheral neuropathies without any visible skin lesions.
- There are no bacilli present in these cases, and skin lesions are absent. This form is negative for the bacteria and often presents a challenge for diagnosis.
The Indian classification tends to highlight the borderline forms (BT, BB, BL), which display features of both tuberculoid and lepromatous leprosy. These forms may shift to either extreme based on how the body’s immune system responds to the bacteria.
Clinical Syndromes of Leprosy
Leprosy is a chronic, granulomatous disease caused by Mycobacterium leprae, with clinical manifestations that vary based on the host’s immune status and the spread of the bacilli. The disease is classified into five forms, each presenting with distinct clinical features and nerve involvement. These forms range from less severe to more debilitating syndromes, with varying degrees of skin lesions and neural damage.
Types of Leprosy
- Tuberculoid Leprosy:
- Skin lesions are few, often circular or serpiginous, and hypopigmented.
- Lesions are usually sharply demarcated with a dry, scaly appearance and a raised, erythematous border.
- Anesthesia of the skin is complete in the affected areas.
- Lesions commonly appear on the face, limbs, and buttocks, but not in the axilla, perineum, or scalp.
- Neural involvement is typical, especially of the ulnar, peroneal, and greater auricular nerves, leading to nerve thickening, wrist drop, or foot drop.
- Borderline Tuberculoid Leprosy:
- This form has smaller and more numerous lesions compared to tuberculoid leprosy.
- Lesions are asymmetric, with almost complete anesthesia.
- Nerve thickening occurs, but the involvement is asymmetric.
- The disease can either regress to tuberculoid leprosy or progress to lepromatous leprosy.
- Mid-Borderline Leprosy:
- Skin lesions in this type are numerous, unequally shaped, and less well-defined than in tuberculoid leprosy.
- Lesions are distributed asymmetrically.
- Anesthesia is moderate, and the disease can either improve, worsen, or remain stable.
- Borderline Lepromatous Leprosy:
- Skin lesions are moderate to numerous and slightly asymmetric.
- Anesthesia is minimal or absent, and nerve enlargement is symmetrical.
- As with other forms of borderline leprosy, this stage can improve, remain stable, or progress to more severe forms.
- Lepromatous Leprosy:
- Skin lesions are extensive, bilaterally symmetrical, and most severe in the cooler parts of the body.
- Lesions include macules, nodules, plaques, or papules.
- Common disfiguring features include a leonine face (thickened, corrugated skin of the face), loss of eyebrows, and pendulous ear lobes.
- Other complications include hoarseness of voice, corneal involvement, perforation of the nasal septum, and nasal collapse.
- The disease can lead to painless adenopathy in the inguinal and axillary regions, scarring of the testes, gynecomastia, and hyperesthesia (increased sensitivity) in the extremities.
Lepra Reactions
Leprosy is often complicated by reactional stages, which are allergic inflammatory responses and can be medical emergencies. These reactions occur in about one-third of patients and include:
- Lepra Type I (Reversal) Reaction:
- A type IV hypersensitivity reaction, often seen in patients with borderline leprosy.
- These reactions typically occur after starting chemotherapy and can last from 2 to 12 months.
- Characterized by erythema, edema, and tenderness of peripheral nerves, suggesting a shift toward a more tuberculoid form of the disease.
- Hormonal changes such as puberty, pregnancy, and childbirth can also trigger this reaction.
- Lepra Type II (Erythema Nodosum Leprosum – ENL):
- A type III hypersensitivity reaction involving immune complex deposition and systemic inflammation.
- Occurs in 20% of lepromatous leprosy patients and 10% of borderline leprosy patients, often after years of therapy.
- Manifested by painful erythematous nodules on the skin and subcutaneous tissue, along with fever, malaise, arthralgia, neuralgia, and proteinuria.
- Lucio Phenomenon:
- A rare and severe form of type II reaction in diffuse lepromatous leprosy.
- Characterized by hemorrhagic infarcts on the skin, particularly in Mexico and Central America.
Reservoir, Source, and Transmission of Leprosy Infection
Leprosy is a human-specific disease primarily caused by Mycobacterium leprae (M. leprae). While humans are the main reservoir for this infection, other animals have also been found to carry the bacteria. Understanding the ways in which leprosy spreads is key to controlling its transmission.
- Human as the Primary Reservoir
- Humans are the main source of M. leprae.
- Infected individuals, especially those with lepromatous leprosy, shed a significant number of bacilli.
- Animal reservoirs include the nine-banded armadillo, chimpanzee, and mangabey monkeys, but humans remain the primary source of infection.
- Transmission Routes
- Leprosy spreads through aerosols and skin contact.
- Nasal secretions from lepromatous leprosy patients are a key transmission route, with high quantities of bacilli being expelled during activities like blowing the nose. Up to 8 x 10^8 bacilli can be released in a single act.
- Inhalation of aerosols containing M. leprae is a primary method of infection.
- Skin contact with respiratory secretions or wound exudates also plays a role in the spread of the bacteria.
- Evidence for Respiratory Transmission
- The presence of intact lepra bacilli in nasal discharges further supports the idea that airborne transmission is a significant route.
- Unlike nasal secretions, lepra bacilli are not found on the surface of the skin, pointing to a limited risk of transmission through casual skin contact.
- Studies have shown that M. leprae can survive outside the human body for several hours or even days, enhancing the potential for transmission through contaminated environments.
- Experimental studies have demonstrated the transmission of leprosy via aerosol exposure or even through topical application in immunosuppressed animals, further solidifying the respiratory route as a major means of spread.
- Infectivity and Disease Variants
- Lepromatous leprosy is more contagious than the tuberculoid form. The bacilli are much more abundant in lepromatous leprosy, making it easier for the infection to spread.
- People with the tuberculoid form of leprosy are generally not infectious, as they have a more robust immune response that keeps bacterial numbers low.
- Vulnerable Populations
- Close contacts of infected individuals are at higher risk of becoming infected.
- Men are more commonly affected by lepromatous leprosy than women, with a male-to-female ratio of about 2:1.
- Children are more likely to develop tuberculoid leprosy, while the disease is rare in infants.
Diagnosis of Mycobacterium leprae
Diagnosing leprosy hinges on both clinical observation and a variety of laboratory tests. The disease presents a set of symptoms that can alert healthcare providers to its possibility, but confirming the diagnosis requires further investigation. Here’s how it’s done:
- Clinical Symptoms as Initial Indicators
- Key signs like sensory impairment, paraesthesia, motor involvement, and nerve tenderness can signal leprosy.
- Enlarged nerves are also a common feature. When these symptoms appear, laboratory testing should follow to confirm the presence of M. leprae.
- Microscopic Detection and Histopathology
- Microscopic examination of tissue smears is a traditional method, but it’s only reliable if there are at least 10,000 bacteria per gram of tissue.
- This method can fail, especially in pure neural leprosy, where no acid-fast bacilli are found in smears.
- Histopathological evaluation can also aid diagnosis, but the presence of bacteria in tissue samples remains a critical factor.
- Fluorescence In-Situ Hybridization (FISH)
- A specialized probe targeting a highly conserved region in M. leprae has been developed for more precise detection.
- This method involves fixing tissue smears with paraformaldehyde, treating with proteinase K and lysozyme, and using a fluorescent probe to bind to M. leprae DNA.
- After incubating overnight, the sample is examined under fluorescent microscopy to detect the bacteria.
- Serological Testing
- A serological test can identify M. leprae up to 6 months before symptoms appear.
- The test detects immunoglobulin G (IgG) reactions against two specific proteins: ML0405 and ML2331.
- A fusion protein combining these two antigens shows promise for detecting presymptomatic leprosy cases, making early intervention possible.
- Polymerase Chain Reaction (PCR)
- PCR is a powerful tool for amplifying and detecting M. leprae DNA, even in small quantities. It can identify the bacteria with as little as 100 attograms (10^-18 g) of DNA, which is about one-tenth of the bacterial genome.
- Freezing-boiling cycles and treatments with sodium hydroxide help remove inhibitors in the sample, ensuring the PCR process is not hindered.
- For multibacillary patients, PCR testing has shown 100% detection. However, paucibacillary patients show a 79% detection rate.
- TaqMan real-time PCR also allows differentiation between multibacillary and paucibacillary forms of leprosy, offering precise categorization.
- M. leprae Flow Test
- The M. leprae flow test is a rapid diagnostic method. It detects antibodies to PGL-1, a unique surface glycoprotein of M. leprae.
- In just 10 minutes, this test has demonstrated 97.4% sensitivity and 90.2% specificity, making it an efficient option for diagnosing leprosy.
Treatment of Mycobacterium leprae Infection
Treating leprosy effectively requires a combination of medications tailored to the specific form and severity of the infection. The World Health Organization (WHO) has outlined a standard regimen that relies on multiple drugs to prevent resistance, reduce infectivity, and manage symptoms.
- WHO-Recommended Treatment Regimens
- Multibacillary leprosy: The standard treatment includes:
- Rifampicin (600 mg/month)
- Dapsone (100 mg/daily)
- Clofazimine (300 mg/month and 50 mg/daily)
- This regimen is administered for one year.
- Paucibacillary leprosy: The treatment consists of:
- Rifampicin (600 mg/month)
- Dapsone (100 mg/daily)
- Single skin lesions: A combination of:
- Rifampicin (600 mg)
- Ofloxacin (400 mg)
- Minocycline (100 mg) as a single-dose treatment.
- Multibacillary leprosy: The standard treatment includes:
- Dapsone:
- Initially introduced in 1941, dapsone was used as a monotherapy. However, its usage was limited due to drug resistance in M. leprae discovered in the 1960s.
- Mechanism: Dapsone is bacteriostatic, inhibiting folic acid synthesis in the bacteria.
- Side effects: Gastritis, urinary tract issues, fatigue, methemoglobinemia, and more. Resistance led to the shift towards multidrug therapy.
- Multidrug Therapy (MDT)
- Following the discovery of resistance to dapsone, multidrug therapy became the go-to strategy. It combines drugs like rifampicin and clofazimine to reduce bacterial resistance and improve treatment efficacy.
- MDT helps reduce infectivity, recurrent episodes, and reactional states.
- Rifampicin:
- Rifampicin is a bactericidal drug, discovered in 1963, which disrupts bacterial RNA synthesis by inhibiting RNA polymerase.
- Effectiveness: Rifampicin is highly effective, almost eliminating M. leprae from skin and mucosal lesions.
- Side effects: Liver toxicity, psychosis, thrombocytopenia, and other rare symptoms like shock, hemolytic anemia, and renal failure.
- Clofazimine:
- Developed in 1954 and used since 1959, clofazimine binds to bacterial DNA, offering both bacteriostatic and slow bactericidal action.
- Side effects: Skin pigmentation, lower limb edema, and gastrointestinal issues.
- Ofloxacin and Minocycline:
- Ofloxacin, a fluoroquinolone, works by inhibiting DNA gyrase in M. leprae, rendering the bacteria nonviable after about 4 weeks of treatment.
- Side effects include gastrointestinal issues, photodermatitis, and neurological symptoms like insomnia and headaches.
- Minocycline, a semisynthetic tetracycline, inhibits protein synthesis in the bacteria, with side effects like skin pigmentation and gastrointestinal disturbances.
- Managing Reactions
- Type 1 reactions: Treatment involves starting prednisolone at a dose of 20-60 mg, then gradually reducing the dose to avoid side effects from long-term steroid use. Clofazimine can also be added in high doses for severe reactions.
- Type 2 reactions: Typically treated with thalidomide (100-400 mg/day) along with systemic corticosteroids like prednisolone.
- Type 3 reactions: Primarily treated with systemic corticosteroids.
- Other Drugs:
- A number of other drugs, like levofloxacin, sparfloxacin, rifamycin, and rifapentine, have been explored but are not considered standard treatments for leprosy.
The Mechanism of Leprosy Reactions
Leprosy reactions are intense immune responses to Mycobacterium leprae and its components, often triggered by antibiotic therapy. These reactions are a leading cause of nerve damage and disabilities in leprosy patients. They are classified into Type 1 reactions (T1R) and Type 2 reactions, also called erythema nodosum leprosum (ENL). Here’s a breakdown of their mechanisms:
Type 1 Reactions (T1R)
- Nature and Occurrence:
- Also referred to as borderline or reversal reactions.
- Common in borderline leprosy cases (BT, BB, BL) and some lepromatous leprosy (LL) cases.
- Typically begins at diagnosis or within the first two years of multidrug therapy (MDT).
- Affects about 10% of patients with paucibacillary leprosy (PB) and 40% with multibacillary leprosy (MB).
- Immune Mechanism:
- Driven by a Type IV hypersensitivity reaction.
- CD4+ T cells infiltrate tissues, causing immune-mediated damage.
- Key cytokines include TNF-α, IL-2, IL-1β, and IFN-γ.
- Macrophages, activated by Th1 cytokines, release pro-inflammatory signals like iNOS and TGF-β.
- Results in inflammation of pre-existing skin lesions and neuroinflammation.
Type 2 Reactions (ENL)
- Nature and Occurrence:
- Found in patients with LL and some BL cases, particularly with bacterial index (BI) ≥ 4.
- Occurs in over 50% of LL patients and 5–10% of BL cases.
- Frequently observed during the first year of MDT.
- Symptoms include painful red subcutaneous lesions, systemic inflammation, high fever, and damage to nerves, eyes, joints, lymph nodes, and testes.
- Immune Mechanism:
- Characterized by a Type III hypersensitivity reaction due to immune complex deposition in tissues and blood vessels.
- Features increased neutrophil infiltration, although their exact role remains unclear.
- Involves elevated levels of TNF-α and complement activation.
- High expression of CD64 on neutrophils correlates with systemic inflammation severity.
- CD8+ T cells release cytotoxic proteins (perforin, granzymes), inducing apoptosis.
- Cytokine and Cellular Activity:
- Involves both B cells and plasma cells producing antibodies against M. leprae.
- CD4+/CD8+ T cell ratios increase, promoting inflammation.
- Pro-inflammatory cytokines like TNF-α play a central role in the lesions and systemic circulation.
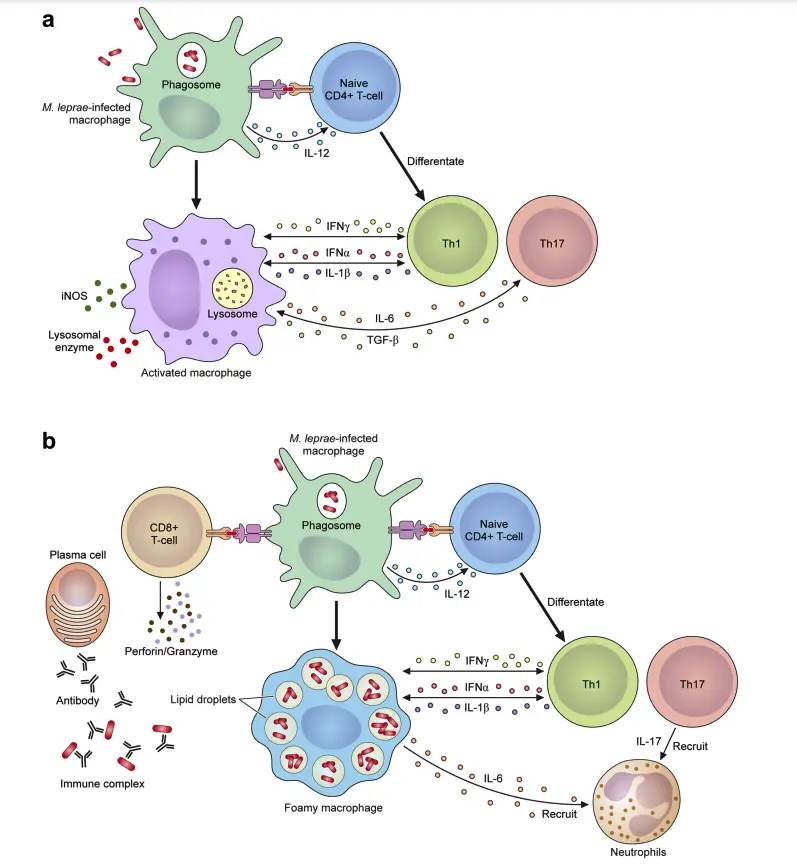
Prevention and Control of Leprosy Infection
Preventing and controlling leprosy relies on early diagnosis, proper treatment, and continuous monitoring. Health education plays a major role in spreading awareness and minimizing transmission. Although research on chemoprophylaxis shows limited effectiveness, detection and management through multi-drug therapy (MDT) remain critical for managing leprosy.
- Early Diagnosis and Treatment
- Leprosy can be tricky because there are no tests to detect carriers before symptoms appear.
- Monitoring the household contacts of leprosy patients is essential. It’s recommended to follow up with these contacts annually for five years after a diagnosis is made.
- Immediate treatment for diagnosed individuals reduces the risk of transmission.
- Health Education
- One of the most powerful tools against leprosy is education.
- Raising awareness about the symptoms, transmission, and the importance of early medical attention can help in controlling the spread of the disease.
- Educating communities also reduces stigma and encourages timely medical intervention.
- Chemoprophylaxis
- Chemoprophylaxis, using drugs like dapsone, acedapsone, and rifampicin, has been tested but doesn’t provide significant protection against leprosy.
- Due to these findings, the current focus is on early detection and MDT (multi-drug therapy) for all patients, which remains the most effective method for preventing the onset and spread of leprosy.
Through consistent monitoring, education, and the appropriate use of MDT, the spread of leprosy can be significantly controlled.
- Sugawara-Mikami M, Tanigawa K, Kawashima A, Kiriya M, Nakamura Y, Fujiwara Y, Suzuki K. Pathogenicity and virulence of Mycobacterium leprae. Virulence. 2022 Dec;13(1):1985-2011. doi: 10.1080/21505594.2022.2141987. PMID: 36326715; PMCID: PMC9635560.
- Mungroo, M. R., Khan, N. A., & Siddiqui, R. (2020). Mycobacterium leprae: Pathogenesis, diagnosis, and treatment options. Microbial Pathogenesis, 104475. doi:10.1016/j.micpath.2020.104475
10.1016/j.micpath.2020.104475 - Pinheiro RO, de Souza Salles J, Sarno EN, Sampaio EP. Mycobacterium leprae-host-cell interactions and genetic determinants in leprosy: an overview. Future Microbiol. 2011 Feb;6(2):217-30. doi: 10.2217/fmb.10.173. PMID: 21366421; PMCID: PMC3123826.
- https://microbiologysociety.org/publication/past-issues/mycobacteria/article/mycobacterium-leprae-the-cause-of-leprosy.html
- https://www.cdc.gov/leprosy/about/index.html
- https://www.osmosis.org/learn/Mycobacterium_leprae
- https://pmc.ncbi.nlm.nih.gov/articles/PMC3123826/
- https://www.sciencedirect.com/science/article/abs/pii/S088240102030841X
- https://www.who.int/news-room/fact-sheets/detail/leprosy
- https://www.sciencedirect.com/topics/medicine-and-dentistry/mycobacterium-leprae
- https://en.wikipedia.org/wiki/Mycobacterium_leprae