What is Mineral Nutrition?
- Mineral nutrition is a critical aspect of plant biology, where plants absorb essential minerals from the soil through their root systems. These minerals are vital for various physiological processes such as growth, reproduction, and metabolism. Although the soil contains a wide range of mineral elements, plants selectively absorb only a fraction of these, typically around 16 to 20 essential elements, which are necessary for their development.
- Mineral elements are classified into two main categories: macronutrients and micronutrients. Macronutrients are required in large quantities and include elements such as nitrogen (N), phosphorus (P), potassium (K), calcium (Ca), magnesium (Mg), and sulfur (S). Micronutrients, needed in smaller amounts, include iron (Fe), manganese (Mn), zinc (Zn), copper (Cu), boron (B), molybdenum (Mo), and chlorine (Cl). These elements play pivotal roles in processes like photosynthesis, respiration, and overall metabolic activity.
- Plants primarily obtain these essential minerals from the soil. The roots absorb the mineral nutrients, which are then distributed throughout the plant in ionic forms, either as anions or cations. These absorbed minerals contribute to various biological functions necessary for the plant’s survival and growth. The process of mineral absorption and utilization is referred to as mineral nutrition.
- In addition to absorbing minerals directly from the soil, some plants engage in symbiotic relationships with nitrogen-fixing bacteria or mycorrhizal fungi, which assist in the acquisition of certain nutrients. In other cases, plants like epiphytes can absorb mineral ions from dust particles in moisture.
- Mineral nutrition not only supports the plant’s internal functions but also plays a role in the broader ecosystem by transferring essential nutrients up the food chain as plants are consumed by herbivores and, subsequently, by higher trophic levels. Thus, mineral nutrition is a foundational process in both plant biology and ecological nutrient cycling.
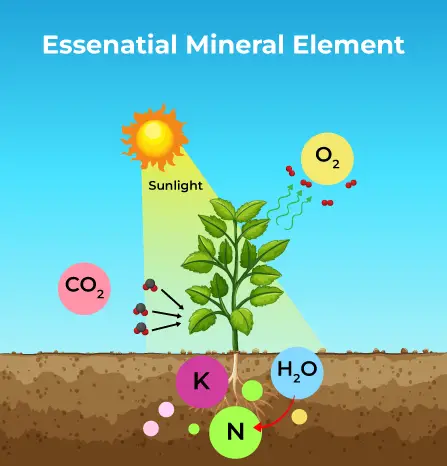
Criteria for Essential Minerals in Plants
The following points outline the criteria used to determine the essentiality of an element:
Criteria for Determining Essential Elements
- Requirement for Normal Growth and Development
- Description: An element is considered essential if it is required for the plant’s normal growth, reproduction, and overall health. It must be a component of vital metabolites or participate in crucial metabolic processes.
- Example: Magnesium is essential for chlorophyll synthesis, which is necessary for photosynthesis and plant health.
- Specificity and Irreplaceability
- Description: The element must have a specific function that cannot be fulfilled by any other element. No other element can substitute for its role in plant physiology.
- Example: Iron is crucial for chlorophyll synthesis and is involved in electron transport during photosynthesis. Its role cannot be substituted by other elements.
- Direct Involvement in Plant Metabolism
- Description: The element must be directly or indirectly involved in metabolic processes or have a structural role in plant tissues. Its function should be integral to the plant’s biochemical pathways.
- Example: Calcium is vital for cell wall stability and signaling, directly influencing plant growth and development.
- Deficiency Symptoms and Recovery
- Description: The absence of an essential element should lead to specific deficiency symptoms. The plant should show improvement in growth and development upon the re-supply of the deficient element.
- Example: Nitrogen deficiency results in chlorosis (yellowing of leaves), and supplying nitrogen can reverse these symptoms, confirming its essential role.
- Beneficial Effect
- Description: The element must contribute to plant health and growth. Deficiency should cause specific disorders or inhibit growth, highlighting its importance.
- Example: Potassium is essential for enzyme activation and osmoregulation. Its deficiency can lead to poor growth and weak plants.
- Universal Occurrence
- Description: The element should be found in all plants or at least in a broad range of plant species. Its essential nature should be evident across different plant types.
- Example: Phosphorus is universally required for ATP formation and nucleic acid structure in all plants.
Types of Essential Nutrients
Below are the primary types of essential nutrients:
- Macronutrients
- Definition: Macronutrients are essential elements required by plants in relatively large quantities, typically exceeding 10 millimoles per kilogram of dry matter.
- Key Elements: These include carbon (C), hydrogen (H), oxygen (O), nitrogen (N), phosphorus (P), sulfur (S), potassium (K), calcium (Ca), and magnesium (Mg).
- Functions: Macronutrients are vital for the formation of cellular structures, energy transfer, and the overall functioning of plants. For example, nitrogen is a critical component of proteins and nucleic acids, while magnesium is central to the chlorophyll molecule, essential for photosynthesis.
- Source: Carbon, hydrogen, and oxygen are primarily obtained from carbon dioxide (CO₂) and water (H₂O), while the remaining macronutrients are absorbed from the soil.
- Micronutrients
- Definition: Micronutrients, also known as trace elements, are required by plants in much smaller quantities, usually less than 10 millimoles per kilogram of dry matter.
- Key Elements: These include iron (Fe), manganese (Mn), copper (Cu), molybdenum (Mo), zinc (Zn), boron (B), chlorine (Cl), and nickel (Ni).
- Functions: Despite their minimal quantities, micronutrients are crucial for various biochemical processes. They often act as cofactors or activators for enzymes. For instance, zinc is an essential cofactor for alcohol dehydrogenase, an enzyme involved in metabolic pathways.
- Source: Micronutrients are primarily absorbed from the soil, and their availability is influenced by factors like soil pH and organic matter content.
- Beneficial Elements
- Definition: Besides the essential macronutrients and micronutrients, some elements, although not universally essential, are beneficial for certain plant species.
- Key Elements: Sodium (Na), silicon (Si), cobalt (Co), and selenium (Se) fall into this category.
- Functions: These elements can enhance growth, improve stress tolerance, or support specific physiological functions. For instance, silicon strengthens cell walls, thereby enhancing resistance to pests and diseases.
- Categorization Based on Functions
- Structural Elements: Some essential elements, such as carbon, hydrogen, oxygen, and nitrogen, are integral components of biomolecules, making up the structural framework of plant cells.
- Energy-Related Components: Elements like magnesium (in chlorophyll) and phosphorus (in ATP) are involved in energy-related processes, vital for photosynthesis and cellular energy transfer.
- Enzyme Activators/Inhibitors: Certain elements serve as activators or inhibitors of enzymes. For example, magnesium is essential for enzymes involved in photosynthesis, while molybdenum is crucial for nitrogen metabolism.
- Osmotic Regulation: Elements like potassium play a significant role in regulating osmotic potential, crucial for processes such as the opening and closing of stomata.
Essential Mineral Elements in Plants
Below is a detailed explanation of these categories and the specific roles each mineral plays in plant life.
1. Macronutrients
Macronutrients are minerals needed by plants in large amounts. They are critical for forming plant tissues, synthesizing chlorophyll, and regulating various metabolic processes. A deficiency in any macronutrient can lead to severe growth issues and reduced yields. The key macronutrients include:
- Nitrogen (N): Vital for the synthesis of amino acids, proteins, nucleic acids, and chlorophyll, nitrogen is essential for plant growth, photosynthesis, and overall metabolism.
- Phosphorus (P): Phosphorus plays a crucial role in energy transfer and storage, primarily in the form of ATP. It is also involved in DNA and RNA synthesis, cell division, root development, and flowering.
- Potassium (K): Potassium regulates water balance, activates enzymes, and contributes to photosynthesis, protein synthesis, and overall plant growth.
- Calcium (Ca): Calcium is essential for maintaining cell wall structure and stability. It also regulates membrane permeability, activates enzymes, and participates in signal transduction.
- Magnesium (Mg): As a central component of chlorophyll molecules, magnesium is crucial for capturing light energy during photosynthesis. It also activates enzymes and aids in the synthesis of nucleic acids.
- Sulfur (S): Sulfur is a component of amino acids, proteins, and vitamins. It is indispensable for protein synthesis and the synthesis of certain compounds involved in plant defense mechanisms.
2. Micronutrients
Micronutrients, also known as trace elements, are required in much smaller quantities but are equally crucial for plant health. They are involved in enzyme activation, hormone synthesis, and chlorophyll production. Despite their minimal amounts, deficiencies in micronutrients can significantly impact plant growth and development. The primary micronutrients include:
- Iron (Fe): Iron is essential for chlorophyll synthesis and plays a vital role in electron transfer reactions and enzyme activation during photosynthesis.
- Zinc (Zn): Zinc serves as a cofactor for numerous enzymes involved in carbohydrate metabolism, protein synthesis, and hormone regulation, making it crucial for overall plant growth.
- Manganese (Mn): Manganese is involved in photosynthesis as an enzyme activator and also plays roles in nitrogen metabolism, lipid synthesis, and antioxidant defense.
- Copper (Cu): Copper acts as a cofactor for enzymes engaged in metabolic processes, including photosynthesis, respiration, lignin synthesis, and antioxidant defense.
- Boron (B): Boron is necessary for cell wall synthesis, carbohydrate metabolism, and pollen germination. It also facilitates sugar translocation within the plant.
- Molybdenum (Mo): Molybdenum is essential for nitrogen fixation in leguminous plants and is involved in enzyme activation and nitrate assimilation.
- Chlorine (Cl): Chlorine plays a role in photosynthesis by being an essential component of the water-splitting enzyme in light-dependent reactions. It also helps in osmoregulation and stomatal opening.
Mechanism of absorption of elements
The absorption of mineral elements by plants is a complex process facilitated through both passive and active mechanisms. Each method plays a critical role in ensuring that essential nutrients are efficiently absorbed from the soil into plant tissues.
A. Passive Absorption of Minerals
Passive absorption does not require the direct expenditure of ATP. The mechanisms involved include:
- Simple Diffusion
- Process: Mineral ions move from the soil solution into root cells through simple diffusion. This process is driven by the concentration gradient of the ions, requiring no additional energy.
- Function: Simple diffusion allows for the gradual movement of minerals into cells, driven solely by the difference in ion concentration between the soil and the root cell.
- Mass Flow
- Process: Mineral ions are absorbed along with the flow of water due to transpiration. As water evaporates from the plant’s surface, it creates a negative pressure that draws water and dissolved ions into the plant.
- Function: Mass flow facilitates the movement of minerals in bulk, ensuring that nutrients are transported efficiently from the soil to the root cells.
- Ion Exchange
- Process: This involves the exchange of mineral ions with ions of the same charge within the soil or root environment.
- Function: Ion exchange helps maintain ion balance and availability in the soil, supporting the uptake of essential nutrients.
- Contact Exchange
- Process: Mineral ions are exchanged with hydrogen (H+) and hydroxide (OH–) ions at the root surface.
- Function: This exchange mechanism aids in the absorption of minerals by altering the ionic environment around the root cells.
- Carbonic Acid Exchange
- Process: Mineral ions exchange with ions from carbonic acid (H2CO3) in the soil solution.
- Function: This method facilitates the uptake of minerals by modifying the ionic composition of the soil solution.
- Donnan Equilibrium
- Process: This theory describes the passive accumulation of ions against their concentration gradient or electrochemical potential. Non-diffusible anions are fixed on the inner side of the cell membrane, while diffusible cations can move freely.
- Function: Donnan equilibrium explains how certain ions can accumulate inside the cell without direct energy input, driven by electrochemical gradients.
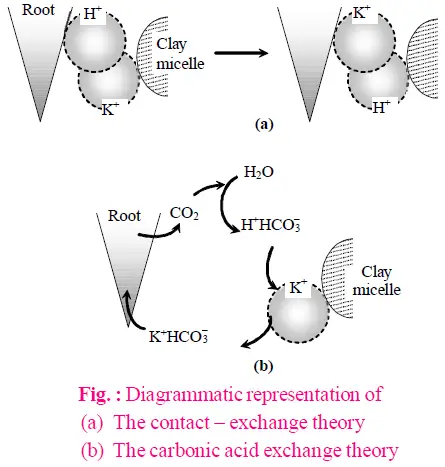
B. Active Ion Absorption
Active absorption involves the expenditure of ATP to transport ions against their concentration gradients. Key mechanisms include:
- Increased Respiration
- Evidence: The rate of respiration in plants increases when exposed to mineral solutions, indicating that active absorption is energy-intensive.
- Function: Enhanced respiration provides the necessary ATP for the active transport of ions.
- Impact of Deficiencies
- Evidence: Factors such as oxygen, carbon dioxide (CO2), and cyanide (CN) deficiencies inhibit respiration and, consequently, mineral ion absorption.
- Function: This relationship highlights the dependence of active absorption on cellular respiration and energy availability.
- K+ Ion Absorption in Nitella Algae
- Evidence: The absorption of potassium ions (K+) in Nitella algae occurs against the concentration gradient, demonstrating active transport.
- Function: This mechanism ensures that K+ ions are absorbed even when their external concentration is lower than inside the cell.
- Cytochrome Pump Theory
- Theory: Proposed by Lundegardh and Burstorm (1933), this theory suggests that anions are actively transported through cytochrome pumps, while the absorption of cations is passive.
- Function: The cytochrome pump theory explains how specific ions are actively transported using energy, while others move passively.
- Carrier Concept
- Theory: According to Vanden Honert, specific protein carrier molecules in the root cell membrane facilitate the absorption of ions. These carriers form ion-carrier complexes that break down inside the cell, using energy.
- Function: The carrier concept illustrates how protein carriers are crucial for the active uptake of ions, enabling the formation and transport of ion complexes.
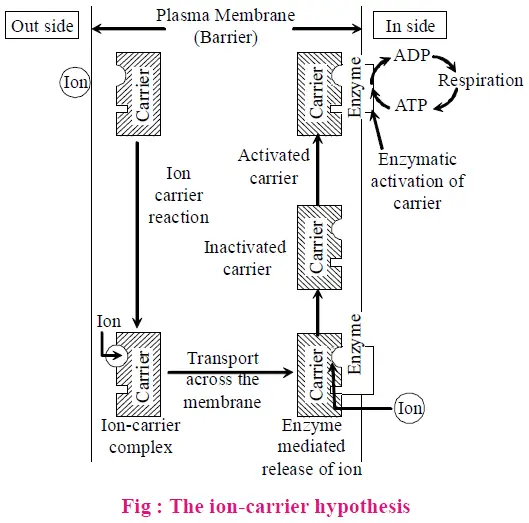
Translocation of solutes (mineral salts)
The movement of mineral salts within plants is a vital process for nutrient distribution, occurring primarily through the xylem vessels. This process involves the transport of inorganic substances from the roots, where they are absorbed, to various parts of the plant. The mechanisms and pathways of translocation are as follows:
Pathways of Mineral Salt Translocation
- Xylem Transport
- Role of Xylem: The xylem serves as the primary conduit for transporting water and dissolved inorganic substances, including mineral salts, from the roots to the rest of the plant. This process is essential for maintaining nutrient distribution and plant hydration.
- Transpiration Pull: Mineral salts are moved through the xylem along with water driven by transpiration pull. Transpiration creates a negative pressure in the leaf stomata, which pulls water and dissolved solutes upward through the plant’s vascular system.
- Rate of Translocation
- Correlation with Water Movement: The rate at which inorganic solutes are translocated through the xylem is directly related to the rate of water translocation. Therefore, any factors influencing water movement, such as transpiration rates or soil moisture, will also impact the movement of mineral salts.
- Measurement with Radio-Isotopes: Studies utilizing radio-isotopes have confirmed that inorganic substances move upward through the xylem, demonstrating the close relationship between water and solute transport.
Pathways for Ion Movement to the Xylem
After mineral ions are absorbed by the roots, they must reach the xylem for translocation. This movement can occur through two distinct pathways:
- Apoplast Pathway
- Description: The apoplast pathway involves the movement of ions through the extracellular spaces and cell walls. This pathway allows ions to travel freely without crossing the cell membranes.
- Function: This pathway facilitates the rapid movement of ions towards the xylem, utilizing the continuous network of cell walls and intercellular spaces.
- Symplast Pathway
- Description: The symplast pathway involves the movement of ions through the cytoplasm of cells connected by plasmodesmata. Ions travel from cell to cell via these cytoplasmic channels, bypassing the cell walls.
- Function: This pathway ensures a more regulated and selective transport of ions, as it involves passage through the cytoplasm and often requires interaction with cellular membranes and transport proteins.
The Pressure-Flow Mechanism
The pressure-flow mechanism describes how solutes, primarily sugars, are transported through the phloem of vascular plants. This mechanism is essential for the translocation of nutrients from sources to sinks within the plant. The following points outline the process and its underlying principles:
Principles of the Pressure-Flow Mechanism
- Source of Pressure in Phloem
- Description: The driving force for phloem transport is pressure generated in the sieve elements and companion cells located in the source tissues, such as leaves.
- Mechanism: In the source tissues, primarily the mesophyll cells in the leaves, sugars are synthesized through photosynthesis and actively transported into the phloem. This active transport requires metabolic energy, which not only facilitates sugar movement but also concentrates the solute in the phloem.
- Osmotic Pressure and Water Influx
- Description: The high concentration of solutes in the phloem leads to osmotic water uptake. Water enters the phloem from surrounding tissues due to osmotic pressure, causing the phloem cells to become turgid.
- Effect: This influx of water generates significant internal pressure, often exceeding ten times the pressure found in a typical automobile tire. The resulting turgor pressure forces the sap to move through the sieve tubes.
- Movement to Sinks
- Description: At the sink tissues, where the phloem sap is directed, the solutes (mainly sugars) are removed for various uses. These include energy production, storage as starch, or assimilation into new cell structures if the sink is actively growing.
- Effect: As sugars are removed, water exits the phloem via osmosis. This reduction in water decreases the internal pressure in the phloem at the sink end.
- Pressure Gradient and Flow
- Description: The differential in pressure between the source (high pressure) and sink (low pressure) regions creates a pressure gradient. This gradient drives the flow of the phloem sap from areas of higher pressure at the source to areas of lower pressure at the sink.
- Analogy: This process is akin to water flowing through a garden hose from a high-pressure end to a low-pressure end, facilitated by the pressure gradient.
Factors affecting mineral absorption
The primary factors include temperature, light, oxygen, pH, interactions with other minerals, and growth. Each factor affects mineral absorption through distinct mechanisms:
- Temperature
- Effect on Absorption Rate: The rate at which minerals and salts are absorbed is directly proportional to temperature. Higher temperatures generally enhance the absorption of mineral ions.
- Temperature Limits: Excessive temperatures can inhibit mineral absorption. This inhibition is likely due to the denaturation of enzymes responsible for the transport of minerals, leading to a reduced efficiency in nutrient uptake.
- Light
- Photosynthesis and Mineral Uptake: Adequate light levels enhance the rate of photosynthesis, which, in turn, increases the availability of food energy. This energy boost leads to an increased uptake of minerals, as the plant can sustain higher levels of metabolic activities.
- Light Intensity: Greater light intensity correlates with more efficient mineral absorption due to enhanced photosynthetic activity and energy availability.
- Oxygen
- Impact of Oxygen Deficiency: A shortage of oxygen (O2) impairs the rate of mineral absorption. This is largely due to decreased ATP production, which is essential for active transport mechanisms involved in mineral uptake.
- Increased Oxygen Tension: Elevated oxygen levels improve the absorption rate of minerals by ensuring adequate ATP supply, which supports the energy-dependent transport processes.
- pH
- pH and Ion Availability: The pH of the surrounding medium influences the solubility and availability of mineral ions. At physiological pH, monovalent ions are absorbed more efficiently. Conversely, an alkaline pH favors the absorption of bivalent and trivalent ions.
- Optimal pH Levels: Maintaining an optimal pH is crucial for maximizing the availability and uptake of specific mineral ions, thus affecting overall nutrient absorption.
- Interaction with Other Minerals
- Competitive Interactions: The presence of certain minerals can affect the absorption of others due to competition for binding sites on transport carriers. For instance, the absorption of potassium (K+) is influenced by the presence of calcium (Ca++) and magnesium (Mg++).
- Mutual Competition: The uptake of potassium (K+), rubidium (Rb), and cesium (Cs) ions involves mutual competition, where the presence of one ion can affect the absorption efficiency of others.
- Growth
- Surface Area and Binding Sites: Growth increases the surface area of the absorption organs, the number of cells, and the number of binding sites for mineral ions. This enhanced surface area and binding capacity facilitate greater mineral absorption.
- Enhanced Absorption: As growth progresses, the increased availability of binding sites and absorption structures supports improved uptake of essential minerals.
Mechanism of Mineral Elements
The mechanism of mineral nutrition in plants can be understood in two main phases: the apoplastic phase and the symplastic phase.
1. Apoplastic Phase
- Passive Transport:
- In the apoplastic phase, mineral ions from the soil enter the plant’s root system, specifically into the cell wall space known as the apoplast.
- This movement occurs passively, meaning it does not require the expenditure of energy by the plant.
- The driving force behind this movement is the concentration gradient, where ions move from an area of higher concentration in the soil to an area of lower concentration within the plant’s apoplast.
- Ion Channels and Transmembrane Proteins:
- The transport is facilitated by ion channels and transmembrane proteins that help in the efficient movement of ions across cell membranes.
- This phase is characterized by rapid ion movement due to the absence of energy barriers.
2. Symplastic Phase
- Active Transport:
- After the ions reach the apoplast, they move into the symplast, which is the continuous network of cytoplasm of plant cells interconnected by plasmodesmata.
- Unlike the apoplastic phase, the symplastic phase requires energy, as ions are transported against their concentration gradient—from areas of low concentration to high concentration.
- Energy Expenditure:
- The energy required for this active transport comes from ATP, which is utilized to pump ions across the plasma membrane into the symplast.
- The movement of ions in this phase is slower compared to the apoplastic phase, due to the energy requirement and the complexity of the symplastic pathway.
Conduction to the Shoot System
- Integration of Apoplast and Symplast:
- The ions, after moving through the apoplast and symplast, enter the xylem vessels, which are the conduits for water and dissolved minerals.
- The integration of these two phases ensures that minerals are effectively transported from the roots to the shoot system.
- Upward Movement:
- Within the xylem, the minerals, along with water, are transported upwards to the leaves and other parts of the plant where they are utilized for various physiological functions.
Key Functional Emphasis
- Role of Stele:
- The stele, a central part of the root, plays a crucial role in regulating the movement of minerals from the soil into the conducting tissues.
- Water Potential:
- Water potential is another critical factor that influences the direction and efficiency of nutrient absorption, as it dictates the osmotic movement of water and dissolved minerals.
Role of macro and micro nutrients
Nutrient elements are crucial for the growth, development, and metabolic processes of plants. These elements are categorized into macro-nutrients and micro-nutrients, each playing specific and essential roles. Understanding these roles can help in managing plant health and optimizing agricultural practices.
Macro-Nutrients
- Nitrogen (N)
- Absorption: Primarily taken up as nitrate (NO3⁻), with some absorption as nitrite (NO2⁻) and ammonium (NH4⁺).
- Function: Essential for protein synthesis, nucleic acids, vitamins, and hormones. Nitrogen is vital for all parts of the plant, especially meristematic tissues.
- Deficiency Symptoms: Includes inhibited growth, chlorosis (yellowing) of leaves, reduced leaf size, delayed flowering, and poor flower development.
- Phosphorus (P)
- Absorption: Absorbed as phosphate ions (H2PO4⁻ or HPO4²⁻).
- Function: Integral component of cell membranes, nucleic acids, nucleotides, and involved in phosphorylation reactions.
- Deficiency Symptoms: Causes premature leaf fall, development of anthocyanin pigments, necrotic areas, and stunted growth.
- Potassium (K)
- Absorption: Taken up as potassium ions (K⁺).
- Function: Maintains cation-anion balance, aids in protein synthesis, stomatal regulation, enzyme activation, and cell turgidity.
- Deficiency Symptoms: Leads to dwarfing, poor cell repair, yellow spots on leaves, and lodging of cereal crops.
- Calcium (Ca)
- Absorption: Absorbed as calcium ions (Ca²⁺).
- Function: Critical for cell wall synthesis, spindle fiber formation during cell division, and cell membrane stability. It also regulates plant metabolism and enzyme activation.
- Deficiency Symptoms: Results in improper chlorophyll function, malformed flowers, early leaf drop, and chlorosis.
- Magnesium (Mg)
- Absorption: Taken up as magnesium ions (Mg²⁺).
- Function: Involved in enzyme activation for respiration and photosynthesis, chlorophyll structure, DNA and RNA synthesis, and ribosomal organization.
- Deficiency Symptoms: Causes chlorosis, particularly in young leaves, increased anthocyanin content, and necrotic spots.
- Sulphur (S)
- Absorption: Absorbed as sulfate ions (SO4²⁻).
- Function: Present in amino acids (methionine and cysteine) and vitamins (thiamine, biotin). Plays a role in protein synthesis and enzyme functions.
- Deficiency Symptoms: Leads to chlorosis, anthocyanin accumulation, growth inhibition, and stunted plants.
Micro-Nutrients
- Iron (Fe)
- Absorption: Taken up as ferric ions (Fe³⁺).
- Function: Component of ferrodoxin and cytochrome involved in electron transport, catalase activation, and chlorophyll biosynthesis.
- Deficiency Symptoms: Results in chlorosis, particularly in young leaves, dark veins, and reduced chlorophyll synthesis.
- Manganese (Mn)
- Absorption: Absorbed as manganese ions (Mn²⁺).
- Function: Activates enzymes in photosynthesis, respiration, and nitrogen metabolism. Essential for water photolysis and oxygen release in photosynthesis.
- Deficiency Symptoms: Appears as interveinal chlorosis and necrotic areas in older leaves.
- Zinc (Zn)
- Absorption: Taken up as zinc ions (Zn²⁺).
- Function: Activates enzymes, particularly carboxylase, and is required for auxin synthesis.
- Deficiency Symptoms: Causes leaf deformation, chlorosis at leaf tips, and poor flowering.
- Copper (Cu)
- Absorption: Absorbed as cupric ions (Cu²⁺).
- Function: Involved in enzyme systems related to redox reactions. Shifts between cuprous and cupric forms.
- Deficiency Symptoms: Leads to diseases such as dieback in citrus and reclamation disease in paddy, along with necrotic areas in young leaves.
- Boron (B)
- Absorption: Taken up as borate ions (BO3³⁻ and B4O7²⁻).
- Function: Important for calcium uptake, membrane function, pollen germination, cell elongation, and carbohydrate translocation.
- Deficiency Symptoms: Results in shoot apex death, inhibited flowering, blackened leaf tips, and stunted growth.
- Molybdenum (Mo)
- Absorption: Absorbed as molybdate ions (MoO4²⁻).
- Function: Component of enzymes such as nitrogenase and nitrate reductase, involved in nitrogen metabolism.
- Deficiency Symptoms: Causes amino acid accumulation, flowering inhibition, and whip tail disease in cauliflower.
- Chlorine (Cl)
- Absorption: Taken up as chloride ions (Cl⁻).
- Function: Works with potassium and sodium to balance solute concentration, and is required for water hydrolysis in photosynthesis.
- Deficiency Symptoms: Results in leaf wilting, chlorosis, necrotic areas, and reduced root length.
- Nickel (Ni)
- Absorption: Absorbed as nickel ions (Ni²⁺).
- Function: Essential for the enzyme urease and seed germination.
- Deficiency Symptoms: Causes chlorosis and necrotic spots on leaves.
Sources of Essential Elements for Plants
Plants obtain essential elements primarily from the soil and, to a lesser extent, from the atmosphere. Below is a detailed explanation of the sources for these key elements:
- Carbon (C)
- Source: Carbon dioxide (CO₂) from the atmosphere.
- Details:
- Plants absorb CO₂ through stomata in their leaves.
- Carbon is vital for photosynthesis, where it is fixed into organic compounds.
- Therefore, CO₂ is a primary source of carbon, which forms the backbone of carbohydrates, proteins, and lipids.
- Oxygen (O)
- Source: Molecular oxygen from the air or water.
- Details:
- Oxygen is absorbed directly from the atmosphere through stomata.
- It is also released during photosynthesis within the plant.
- Oxygen plays a critical role in cellular respiration, where it acts as the final electron acceptor in the electron transport chain.
- Hydrogen (H)
- Source: Water (H₂O).
- Details:
- Hydrogen is released from water during the process of photosynthesis.
- It combines with carbon to form carbohydrates and other organic molecules.
- Consequently, hydrogen is integral to the synthesis of organic compounds and energy production.
- Nitrogen (N)
- Source: Soil, primarily as nitrate ions (NO₃⁻) or ammonium ions (NH₄⁺).
- Details:
- Plants absorb these ions through their root systems.
- Certain soil microorganisms, including bacteria and cyanobacteria, can fix atmospheric nitrogen (N₂) into forms usable by plants.
- Nitrogen is essential for the synthesis of amino acids, nucleic acids, and chlorophyll.
- Potassium (K), Calcium (Ca), Iron (Fe), Phosphorus (P), Sulphur (S), Magnesium (Mg)
- Source: Soil, originating from the weathering of rocks.
- Details:
- These elements are absorbed by plants in their ionic forms, such as K⁺, Ca²⁺, Fe³⁺, H₂PO₄⁻/HPO₄²⁻.
- Potassium: Regulates osmotic pressure and enzyme activation.
- Calcium: Essential for cell wall structure and signal transduction.
- Iron: Vital for chlorophyll synthesis and electron transport.
- Phosphorus: Important for energy transfer and nucleic acid formation.
- Sulphur: Component of amino acids and vitamins.
- Magnesium: Central atom in chlorophyll and necessary for photosynthesis.
Mineral Deficiency in Plants
Mineral deficiency in plants occurs when essential elements are not available in sufficient quantities, leading to various physiological disorders and growth abnormalities. These deficiencies manifest through specific symptoms, each linked to the absence or insufficient availability of particular nutrients. Understanding these symptoms is crucial for diagnosing and correcting mineral deficiencies in plants.
1. Common Deficiency Symptoms
- Chlorosis:
- Definition: Chlorosis refers to the yellowing of leaves due to a loss of chlorophyll.
- Causes: This condition is commonly caused by deficiencies in key nutrients including potassium (K), magnesium (Mg), nitrogen (N), sulfur (S), iron (Fe), manganese (Mn), zinc (Zn), and molybdenum (Mo).
- Effects: Chlorosis impairs the plant’s ability to perform photosynthesis effectively, which can stunt growth and reduce yield.
- Necrosis:
- Definition: Necrosis involves the death of plant tissues, particularly the leaf tissues.
- Causes: This condition can be attributed to deficiencies in potassium (K), calcium (Ca), and magnesium (Mg).
- Effects: Necrotic tissues may lead to reduced photosynthetic capacity and weakened plant structures.
- Inhibition of Cell Division:
- Definition: This symptom is characterized by the suppression of cell division, affecting plant growth and development.
- Causes: It is often caused by deficiencies in nitrogen (N), potassium (K), boron (B), sulfur (S), and molybdenum (Mo).
- Effects: Inhibited cell division can result in stunted plant growth and impaired development of plant organs.
- Stunted Growth:
- Definition: This refers to slower-than-normal growth rates in plants.
- Causes: Deficiencies in nitrogen (N), phosphorus (P), potassium (K), zinc (Zn), and calcium (Ca) are commonly associated with this symptom.
- Effects: Stunted growth can impact the overall health and productivity of the plant.
- Premature Leaf and Bud Drop:
- Definition: Premature leaf and bud drop occurs when leaves and buds fall off before their normal time.
- Causes: This symptom is typically due to deficiencies in potassium (K) and phosphorus (P).
- Effects: Premature drop can lead to reduced photosynthetic area and diminished reproductive success.
- Delayed Flowering:
- Definition: This symptom involves a delay in the onset of flowering.
- Causes: Deficiencies in nitrogen (N), sulfur (S), and molybdenum (Mo) are often responsible for delayed flowering.
- Effects: Delayed flowering can result in reduced fruit or seed production and overall decreased plant productivity.
2. Diagnosis and Indicators
- Hydroponic Studies:
- Hydroponics, a method of growing plants in nutrient solutions without soil, can be used to study and identify specific mineral deficiencies.
- By controlling the nutrient composition in the solution, researchers can induce and observe deficiency symptoms, helping to diagnose similar issues in field conditions.
- Soil Indicators:
- In natural conditions, the presence of deficiency symptoms in plants serves as an indicator of the mineral composition of the soil.
- Observing these symptoms can help in determining which nutrients are lacking in the soil and need to be supplemented.
3. Mineral Toxicity vs. Mineral Deficiency
- Definition: Mineral toxicity occurs when excessive amounts of certain minerals disrupt normal plant functions.
- Symptoms:
- Brown Spots: Manganese (Mn) toxicity can cause brown spots on leaves, often accompanied by chlorotic veins.
- Disruption of Nutrient Uptake: Excess manganese inhibits calcium (Ca) translocation and competes with iron (Fe) and magnesium (Mg) for enzyme binding. This competition can lead to deficiencies in Fe, Ca, and Mg.
- Effects: Toxicity symptoms can impair plant health by disrupting normal nutrient balance and physiological processes.
4. Key Functional Emphasis
- Chlorophyll and Photosynthesis:
- The presence of essential minerals is vital for the synthesis of chlorophyll, which is crucial for photosynthesis.
- Deficiencies that affect chlorophyll production, such as those causing chlorosis, directly impact the plant’s ability to produce energy.
- Cell Structure and Function:
- Minerals like calcium (Ca) and magnesium (Mg) are fundamental to maintaining cell structure and function.
- Deficiencies in these elements can lead to weakened cell walls and disrupted cellular processes, resulting in symptoms like necrosis and inhibited cell division.
Deficiency Symptoms | Mineral Elements |
---|---|
Chlorosis (loss of chlorophyll causing yellowing of leaves) | N, K, Mg, S, Fe, Mn, Zn, Mo |
Stunted plant growth (retardation resulting in rosette appearance) | N, K, Ca, S, Zn, B, Mo, Cl |
Purple coloration of shoot axis/leaves | N, P, Mg, S, Mo |
Necrosis of leaves (localized death of leaf tissue) | Ca, Mg, Cu, K |
Premature fall of leaves/buds | P, Mg, Cu |
Inhibition of cell division | N, K, S, Mo |
Wrinkling of cereal grains, dormancy of lateral buds, late flowering | N, S, Mo |
Die back of stem/leaves | K, Cu |
Wilted leaves | Cl |
Death of root and shoot tips | B |
Bushy habit of shoot, scorched leaf tips | K |
Interveinal chlorosis | Fe |
Whiptail disease of leaves | Mo |
Mode of nutrition in plants
Plant nutrition is categorized into two primary modes: autotrophic and heterotrophic. Each mode reflects different strategies plants use to obtain their nutrients, essential for growth and survival.
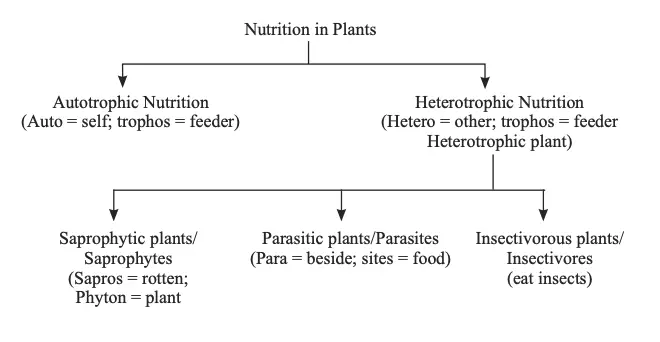
1. Autotrophic Nutrition
Autotrophic nutrition involves organisms producing their own organic food from simple inorganic substances. This mode is characteristic of green plants, which are known as autotrophs. Autotrophic plants utilize external energy sources to synthesize organic compounds.
- Photosynthesis:
- Definition: The process through which green plants convert light energy from the sun into chemical energy stored in organic compounds.
- Mechanism: Plants use chlorophyll to capture sunlight and convert carbon dioxide and water into glucose and oxygen. This process occurs in the chloroplasts of plant cells.
- Types:
- Photoautotrophs: Green plants are photoautotrophs as they rely on sunlight for energy.
- Insectivorous Plants: Although primarily autotrophic, these plants have evolved to trap insects to supplement nitrogen in nitrogen-deficient soils. Examples include:
- Pitcher Plant (Nepenthes): Has modified leaves forming a pitcher that traps and digests insects.
- Sundew (Drosera): Features glandular hairs that secrete sticky substances to capture insects.
- Venus Flytrap (Dionaea): Possesses specialized leaves that snap shut to trap prey.
- Bladderwort (Utricularia): Utilizes bladder-like structures to trap and digest small aquatic organisms.
2. Heterotrophic Nutrition
Heterotrophic nutrition involves organisms that cannot synthesize their own organic nutrients from inorganic substances and must rely on other sources for nourishment. This mode is exhibited by non-green plants, such as fungi and some bacteria, as well as specific plant species.
- Types of Heterotrophic Plants:
- Saprophytes:
- Definition: These plants grow on dead organic matter, including decomposing plant and animal remains.
- Mechanism: Saprophytes secrete extracellular enzymes that break down complex organic compounds into simpler forms, which are then absorbed.
- Examples:
- Fungi: Include molds and mushrooms.
- Monotropa (Indian Pipe Plant): An example of a higher plant that lives as a saprophyte, found in specific regions such as the Khasi Hills.
- Parasitic Plants:
- Definition: These plants lack chlorophyll and derive their nutrients by attaching to and extracting resources from other living plants.
- Mechanism: Parasitic plants use structures called haustoria to penetrate the host plant’s vascular tissues, drawing nutrients directly from the host.
- Examples:
- Dodder (Cuscuta): A yellow, chlorophyll-less climber that attaches to host plants and derives nourishment from their phloem. It produces bell-shaped flowers and lacks roots in its mature state.
- Saprophytes:
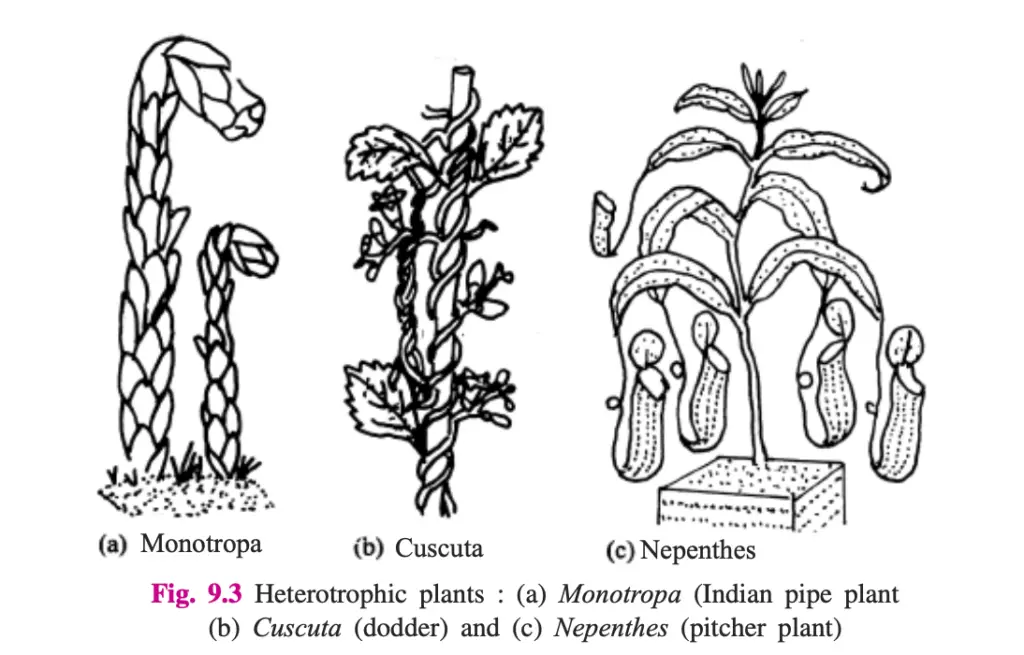
Functions of Mineral Elements
Below is a detailed examination of these essential functions:
- Formation of Plant Tissues
- Roots, Stems, and Leaves: Mineral elements contribute significantly to the structural formation of plant tissues. For instance, calcium is integral in forming cell walls, thus contributing to the strength and stability of roots and stems. Phosphorus, on the other hand, is crucial for the development of leaves and overall plant vigor. The minerals facilitate cellular division and differentiation, leading to the development of robust plant structures.
- Regulation of Soil Acidity
- pH Balance: Mineral elements are vital in regulating the pH of the soil, acting as natural buffers. Elements such as calcium and magnesium help to neutralize excess acidity or alkalinity. By maintaining a balanced pH, these minerals ensure optimal conditions for nutrient availability and microbial activity, which are essential for plant health.
- Toxic Effects of Excess
- Excessive Accumulation: Although mineral elements are crucial, their excessive presence can be detrimental. For example, an overabundance of nitrogen can lead to phenomena such as leaf burn and reduced growth. Excessive levels of other elements like potassium can also disrupt nutrient uptake, leading to imbalances and potential toxicity.
- Enzyme Activation
- Metabolic Processes: Mineral elements are essential for the activation of various enzymes that drive metabolic processes within the plant. Magnesium, for instance, is a central component of chlorophyll and is involved in several enzymatic reactions that facilitate photosynthesis and nutrient metabolism. Without proper mineral nutrition, enzymatic activities would be impaired, affecting overall plant function.
- Chlorophyll Production
- Photosynthesis: Mineral elements, particularly macronutrients like magnesium, are crucial for chlorophyll synthesis. Chlorophyll is necessary for the process of photosynthesis, which allows plants to convert light energy into chemical energy. Magnesium, in particular, is a central component of the chlorophyll molecule, making it essential for efficient photosynthesis and plant energy production.
Metabolism of nitrogen
Nitrogen is a crucial element in biological systems, essential for the synthesis of proteins, nucleic acids, and various other organic molecules. The metabolism of nitrogen involves its transformation and utilization in different chemical forms. This process is facilitated through a series of interrelated steps collectively known as the nitrogen cycle. The following sections provide a detailed explanation of the nitrogen cycle and its key processes.
Nitrogen Cycle
The nitrogen cycle is a continuous process that circulates nitrogen among the atmosphere, soil, and living organisms. It involves several critical stages:
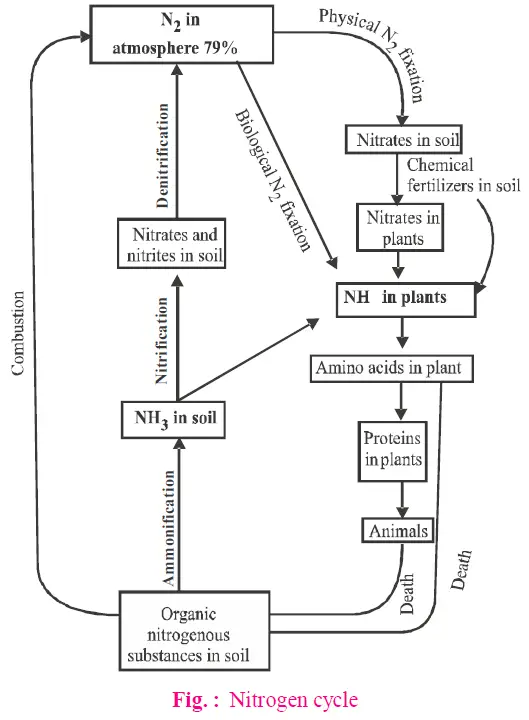
- Nitrogen Fixation
- Abiological Nitrogen Fixation
- Description: Nitrogen fixation refers to the conversion of atmospheric nitrogen (N2) into usable nitrogen compounds. Abiological fixation occurs through natural and industrial processes:
- Natural Fixation: Lightning and ultraviolet radiation convert N2 into nitrogen oxides (NO, NO2, N2O). These oxides dissolve in rainwater, forming nitrous and nitric acids, which then react with soil alkalis to produce nitrates (NO3–), which plants can absorb.
- Industrial Fixation: The Haber-Bosch process converts atmospheric nitrogen into ammonia (NH3) at high temperatures and pressures for use in fertilizers.
- Description: Nitrogen fixation refers to the conversion of atmospheric nitrogen (N2) into usable nitrogen compounds. Abiological fixation occurs through natural and industrial processes:
- Biological Nitrogen Fixation
- Description: Biological nitrogen fixation involves the conversion of N2 into ammonia (NH3) by certain bacteria and cyanobacteria. These organisms possess the enzyme nitrogenase, which facilitates this conversion.
- Free-Living Bacteria: Examples include Azotobacter and Clostridium, which fix nitrogen in the soil.
- Symbiotic Bacteria: Rhizobium forms symbiotic relationships with leguminous plants, while Frankia associates with non-leguminous plants. These bacteria live in nodules on plant roots and fix nitrogen directly for plant use.
- Description: Biological nitrogen fixation involves the conversion of N2 into ammonia (NH3) by certain bacteria and cyanobacteria. These organisms possess the enzyme nitrogenase, which facilitates this conversion.
- Abiological Nitrogen Fixation
- Ammonification
- Description: Ammonification is the process where nitrogenous organic compounds from dead plants and animals are converted into ammonia (NH3) or ammonium ions (NH4+) by ammonifying bacteria.
- Function: This process transforms organic nitrogen into a form that can be absorbed by plants. Ammonium ions are less toxic to plants than ammonia. Ammonifying bacteria include species such as Bacillus mycoides and Bacillus ramosus.
- Process: Proteins from dead organisms are broken down into amino acids, which are then converted into ammonia and organic acids.
- Nitrification
- Description: Nitrification is the conversion of ammonia to nitrate (NO3–) and nitrite (NO2–) by nitrifying bacteria. This process occurs in two stages:
- Ammonia Oxidation: Ammonia is first converted into nitrites (NO2–) by bacteria such as Nitrosomonas.
- Nitrite Oxidation: Nitrites are then converted into nitrates (NO3–) by bacteria such as Nitrobacter.
- Function: Nitrification makes nitrogen available in the form of nitrates, which plants can readily absorb. Nitrifying bacteria are chemoautotrophs that utilize the energy released during oxidation for growth.
- Description: Nitrification is the conversion of ammonia to nitrate (NO3–) and nitrite (NO2–) by nitrifying bacteria. This process occurs in two stages:
- Denitrification
- Description: Denitrification is the process where nitrates and nitrites are converted back into atmospheric nitrogen (N2) by denitrifying bacteria. This process completes the nitrogen cycle.
- Bacteria Involved: Denitrifying bacteria include Micrococcus denitrificans and Pseudomonas.
- Process: Denitrification occurs in waterlogged, anaerobic soils where nitrates are reduced to nitrites, then to nitrogen oxides, and finally to nitrogen gas.
- Function: This process returns nitrogen to the atmosphere, maintaining the balance of nitrogen in the environment.
Difference between Micronutrients and Macronutrients
Here is a detailed comparison:
- Requirements
- Micronutrients
- Quantity: Required in minute quantities, typically less than 1 mg/gm of body weight.
- Details: Essential for various biochemical processes despite their low concentration.
- Macronutrients
- Quantity: Required in large quantities.
- Details: Needed for energy provision and structural functions in the body.
- Micronutrients
- Functions
- Micronutrients
- Role: Crucial in the prevention and management of diseases.
- Details: Micronutrients, such as vitamins and minerals, are integral to enzymatic reactions, immune function, and cellular health.
- Macronutrients
- Role: Play a significant role in providing energy.
- Details: Macronutrients, including carbohydrates, proteins, and fats, are primary sources of energy and are essential for growth and repair.
- Micronutrients
- Consequences of Deficiency
- Micronutrients
- Examples of Deficiencies: Anemia, scurvy, goiter.
- Details: Deficiencies in micronutrients can lead to specific health disorders, affecting various bodily functions.
- Macronutrients
- Examples of Deficiencies: Malnutrition, Kwashiorkor, marasmus.
- Details: Lack of sufficient macronutrients can result in broader health issues, including impaired growth and energy deficits.
- Micronutrients
- Consequences of Overconsumption
- Micronutrients
- Effects: Can lead to hazardous effects such as liver damage and nerve issues, especially with excessive intake of vitamins.
- Details: While essential in small amounts, overconsumption can disrupt metabolic balance.
- Macronutrients
- Effects: Overconsumption can contribute to cardiovascular diseases, diabetes, obesity.
- Details: Excess intake of macronutrients can result in chronic health conditions due to imbalanced energy and nutrient levels.
- Micronutrients
- Concentration
- Micronutrients
- Availability: Found in high concentrations in specific foods but required in very small amounts in the body.
- Details: Often referred to as trace elements due to their minimal quantity.
- Macronutrients
- Availability: Present in large quantities within foods and the body.
- Details: Known as major elements due to their substantial role and quantity.
- Micronutrients
- Composition
- Micronutrients
- Types: Includes vitamins, minerals, and trace elements.
- Examples: Antioxidants, specific minerals (like zinc and iron), and vitamins (like vitamin C and D).
- Macronutrients
- Types: Includes carbohydrates, proteins, and fats.
- Examples: Proteins, fiber, and various types of fats.
- Micronutrients
- Sources
- Micronutrients
- Sources: Found in vegetables, fruits, green leafy vegetables, and eggs.
- Details: These sources are rich in essential trace elements and vitamins.
- Macronutrients
- Sources: Abundant in cereals, legumes, meat, fish, yams, potatoes, nuts, and oilseeds.
- Details: These sources provide the bulk of daily energy and structural components needed by the body.
- Micronutrients
- Advantages
- Micronutrients
- Contribution: Contribute to body growth, disease prevention, and overall health.
- Macronutrients
- Contribution: Provide the energy required for the metabolic system and support various bodily functions.
- Micronutrients
Aspect | Micronutrients | Macronutrients |
---|---|---|
Requirements | Required in minute quantities (less than 1 mg/gm) | Required in large quantities |
Functions | Crucial in the prevention and management of diseases | Significant in providing energy |
Consequences of Deficiency | Anemia, scurvy, goiter | Malnutrition, Kwashiorkor, marasmus |
Consequences of Overconsumption | Hazardous effects such as liver damage and nerve issues | Contributes to cardiovascular diseases, diabetes, obesity |
Concentration | Found in high concentrations in specific foods but required in very small amounts in the body | Present in large quantities within foods and the body |
Composition | Includes vitamins, minerals, and trace elements | Includes carbohydrates, proteins, and fats |
Types | Antioxidants, specific minerals (e.g., zinc, iron), vitamins (e.g., C, D) | Proteins, fiber, various types of fats |
Sources | Vegetables, fruits, green leafy vegetables, eggs | Cereals, legumes, meat, fish, yams, potatoes, nuts, oilseeds |
Advantages | Contribute to body growth, disease prevention, and overall health | Provide energy required for metabolic processes and support bodily functions |
- https://www.geeksforgeeks.org/essential-mineral-elements-mineral-nutrition/
- https://biologyreader.com/mineral-nutrition-in-plants.html
- https://nios.ac.in/media/documents/SrSec314NewE/Lesson-09.pdf
- https://ncert.nic.in/ncerts/l/kebo112.pdf
- https://www.vedantu.com/biology/mineral-nutrition
- https://www.hcpgcollege.edu.in/sites/default/files/MINERAL%20NUTRITION-2.pdf
- https://www.slideshare.net/slideshow/mineral-nutrition-68260703/68260703
- https://www.biologydiscussion.com/plants/minerals/mineral-nutrition-and-elements-in-plants-botany/14934
- https://www.cleariitmedical.com/2019/06/biology-notes-mineral-nutrition.html
- https://www.brainkart.com/article/Mineral-Nutrition-for-Plants_33081/
- https://www.practically.com/studymaterial/blog/docs/class-11th/biology/mineral-nutrition/
- https://jvc.ac.in/assets/pdf/Mineral-Nutrition.pdf