What is Malaria?
- Malaria is a mosquito-borne infectious disease caused by single-celled microorganisms of the Plasmodium group. The term “malaria,” derived from the Medieval Italian phrase meaning “bad air,” reflects historical beliefs about its origins related to marshy environments. Although once prevalent in parts of Europe and North America, malaria is now primarily endemic to tropical and subtropical regions, particularly in sub-Saharan Africa, where the majority of cases and deaths occur.
- The disease is transmitted exclusively through the bites of infected female Anopheles mosquitoes. Once a mosquito bites a human, the Plasmodium parasites are injected via the mosquito’s saliva into the bloodstream. The parasites then migrate to the liver, where they mature and reproduce. There are five species of Plasmodium that primarily infect humans, with P. falciparum, P. vivax, and P. knowlesi being associated with more severe cases. P. falciparum is particularly noteworthy as it accounts for the vast majority of malaria-related fatalities.
- Symptoms of malaria typically manifest 10 to 15 days following an infected mosquito bite. Common symptoms include fever, fatigue, vomiting, and headaches, while severe cases can lead to jaundice, seizures, coma, or death. If untreated, the disease can recur months later, although individuals recently recovered may experience milder symptoms upon reinfection. However, this partial immunity fades over time without ongoing exposure to malaria.
- Diagnosis of malaria is commonly conducted through the microscopic examination of blood films or rapid diagnostic tests that detect antigens. Advanced methods such as polymerase chain reaction (PCR) for detecting the parasite’s DNA exist, yet they remain less prevalent in endemic regions due to cost and complexity.
- Prevention strategies focus on minimizing mosquito bites through the use of insecticide-treated bed nets, insect repellents, and other mosquito-control measures such as spraying insecticides and eliminating standing water. Chemoprophylaxis is also recommended for travelers to malaria-endemic areas, with medications such as sulfadoxine/pyrimethamine recommended for certain populations.
- As of 2023, two malaria vaccines have been endorsed by the World Health Organization, marking a significant advancement in malaria prevention. The recommended treatment for malaria involves a combination of antimalarial medications, including artemisinin paired with drugs like mefloquine, lumefantrine, or sulfadoxine/pyrimethamine. In situations where artemisinin is unavailable, alternatives such as quinine in combination with doxycycline can be used.
- Despite advancements in control and prevention, malaria remains a major public health challenge, particularly in low-income regions. In 2022, an estimated 249 million cases of malaria were reported worldwide, leading to approximately 608,000 deaths, with children under five accounting for a significant proportion of these fatalities. The economic impact of malaria is profound, particularly in Africa, where it is estimated to cause losses of around US$12 billion annually due to increased healthcare costs, lost productivity, and adverse effects on tourism. Addressing malaria effectively requires sustained efforts in prevention, treatment, and research to combat the disease and its associated socio-economic burdens.
Classification of Malaria parasite
Malaria, a significant global health challenge, is caused by parasitic organisms that belong to the genus Plasmodium. These parasites are classified under the Phylum Apicomplexa, which encompasses various protozoans known for their complex life cycles. A comprehensive understanding of the classification of malaria parasites is essential for educators and students alike, as it facilitates a deeper appreciation of their biology and impact on human health.
The classification hierarchy for malaria parasites is as follows:
- Phylum: Apicomplexa
This phylum includes a diverse group of protozoans characterized by their apical complex structure, which aids in host cell invasion. These organisms are primarily intracellular parasites, relying on a host for survival and reproduction. - Class: Sporozoa
Within Apicomplexa, the class Sporozoa includes organisms that typically reproduce asexually via a process known as schizogony, and sexually through gametogenesis. This class is recognized for its unique reproductive strategies and lifecycle stages. - Order: Haemosporida
The order Haemosporida encompasses blood parasites, including those that cause malaria. Members of this order are primarily transmitted through the bites of infected female mosquitoes, which inject the parasites into the bloodstream of their hosts. - Genus: Plasmodium
The genus Plasmodium is divided into two main subgenera, each containing distinct species. The primary subgenus is Plasmodium, which includes:- P. vivax: Known for its ability to enter a dormant liver stage, leading to relapses. This species is prevalent in various regions, especially in Asia and Latin America.
- P. malariae: Typically associated with more chronic infections. It is less common than other species but can persist in the human host for extended periods.
- P. ovale: Similar to P. vivax, it can also remain dormant in the liver and cause relapses. This species is primarily found in West Africa.
- P. falciparum: This species is the most notorious for causing severe malaria, responsible for the majority of malaria-related fatalities globally. Its evolutionary lineage suggests a closer relationship to bird malaria parasites than to other human malaria species, which may account for its aggressive nature and adaptation to human hosts.
- Other Notable Species:
- P. knowlesi: This species primarily infects long-tailed macaque monkeys but has been known to infect humans, especially in Southeast Asia. It presents a unique case as it is zoonotic, reflecting the broader complexity of malaria transmission dynamics.
Causative Agents of Human Malaria
- Plasmodium vivax:
- Classification: This species is categorized as causing benign tertian malaria.
- Characteristics: P. vivax is known for its ability to establish a dormant liver stage, known as hypnozoites, which can lead to relapses in malaria symptoms even after initial treatment. This capability distinguishes it from other Plasmodium species, making its management particularly challenging.
- Epidemiology: This parasite is predominantly found in regions such as Asia and Latin America. It is responsible for a significant proportion of malaria cases globally, contributing to the burden of the disease through recurrent infections.
- Plasmodium falciparum:
- Classification: Often referred to as malignant tertian malaria, P. falciparum is the most virulent species among the malaria-causing parasites.
- Characteristics: It is notable for its ability to cause severe illness and complications, including cerebral malaria, which can result in death if left untreated. P. falciparum infects red blood cells rapidly and can lead to high parasitemia levels, increasing the risk of severe outcomes.
- Epidemiology: This species is prevalent in sub-Saharan Africa and is responsible for the vast majority of malaria-related fatalities worldwide. Its aggressive nature and rapid transmission dynamics pose significant challenges for malaria control efforts.
- Plasmodium malariae:
- Classification: This species is categorized as benign quartan malaria.
- Characteristics: P. malariae typically has a more prolonged cycle, leading to symptoms that may appear every three days. It is known for causing chronic infections that can persist for years, even in the absence of symptoms.
- Epidemiology: Though less common than P. vivax and P. falciparum, P. malariae can still contribute to the overall malaria burden, particularly in areas where it coexists with other Plasmodium species.
- Plasmodium ovale:
- Classification: Similar to P. vivax, P. ovale also causes benign tertian malaria.
- Characteristics: This species is characterized by its ability to cause relapses due to dormant liver forms, similar to P. vivax. P. ovale infections can present with symptoms that resemble those of other species but are less common.
- Epidemiology: It is primarily found in West Africa and is less prevalent compared to the other malaria-causing species, contributing to a smaller portion of the overall malaria cases globally.
History and Distribution of Malaria parasite
The scientific understanding of malaria has evolved significantly, with major discoveries paving the way for contemporary knowledge and control measures.
- Historical Discoveries:
- In 1880, Alphonse Laveran, a French army surgeon, identified the malaria parasite in the red blood cells of a patient in Algeria, marking the first time the causative agent was recognized.
- In 1886, Italian scientist Golgi described the asexual reproduction of the parasite within red blood cells, a process later termed the Golgi cycle.
- The staining technique for identifying malaria parasites in blood films was developed by Romanowsky in 1891, enhancing diagnostic capabilities.
- Between 1886 and 1890, the three primary malaria species affecting humans were classified: Plasmodium vivax, Plasmodium malariae, and Plasmodium falciparum. The fourth species, Plasmodium ovale, was identified in 1922.
- Transmission and Epidemiology:
- The mode of transmission was clarified in 1897 when Ronald Ross identified malaria parasites in mosquitoes in Secunderabad, India. This pivotal discovery led to the implementation of mosquito control measures, aiming to mitigate malaria’s spread.
- Both Ross and Laveran received Nobel Prizes for their contributions to malaria research, underscoring the significance of their findings.
- Geographical Distribution:
- The distribution of malaria species varies significantly across regions:
- P. vivax: Most prevalent in Asia, North Africa, and Central and South America.
- P. falciparum: Dominant in sub-Saharan Africa, Papua New Guinea, and Haiti, with a notable spread in Southeast Asia and India.
- P. malariae: Present in many regions, but rare, particularly outside Africa.
- P. ovale: Primarily confined to West Africa, where it ranks second in prevalence after P. falciparum.
- The distribution of malaria species varies significantly across regions:
- Endemic and Epidemic Patterns:
- Malaria can manifest in two main patterns: endemic and epidemic.
- Endemic malaria occurs consistently in an area over several years.
- Epidemic malaria is characterized by sudden increases in incidence, often linked to changes in environmental, economic, or social conditions, such as population migrations or heavy rainfall following droughts.
- Malaria can manifest in two main patterns: endemic and epidemic.
- World Health Organization (WHO) Classifications:
- The WHO classifies malaria endemicity based on spleen or parasite rates within specific population samples:
- Hypoendemic: Low transmission (<10%).
- Mesoendemic: Moderate transmission (11-50%).
- Hyperendemic: Intense but seasonal transmission (51-75%).
- Holoendemic: High-intensity transmission (>75%).
- The WHO classifies malaria endemicity based on spleen or parasite rates within specific population samples:
- Current Situation in India:
- In India, malaria continues to pose a significant public health challenge, affecting approximately 27% of the population in high-transmission areas (>1 case/1,000 population) and 58% in low-transmission areas (0-1 case/1,000 population).
- Although there has been a decline in total malaria cases compared to previous years, the proportion of cases caused by P. falciparum has increased, necessitating ongoing vigilance and control efforts.
Vectors of Malaria parasite
This disease is primarily conveyed through various species of female Anopheles mosquitoes, which serve as vectors. Notably, the female mosquitoes require blood meals to reproduce, while their male counterparts feed exclusively on nectar and other plant juices.
- Role of Female Anopheles Mosquitoes:
- Female Anopheles mosquitoes are the primary vectors responsible for transmitting malaria to humans.
- They require a minimum of two blood meals to develop and lay their first batch of eggs, establishing a direct link between their feeding habits and malaria transmission.
- Species of Anopheles Mosquitoes in India:
- India is home to approximately 45 different species of Anopheles mosquitoes. However, only a select few are recognized as effective malaria vectors. These include:
- Anopheles culicifacies: This species is highly prevalent in rural areas and is a significant vector for malaria transmission.
- Anopheles fluviatilis: Known for its adaptability to various habitats, this mosquito is also a notable vector in specific regions.
- Anopheles stephensi: An urban vector that has adapted to thrive in cities, contributing to the spread of malaria in urban settings.
- Anopheles minimus: Often found in forested regions, this species plays a role in rural malaria transmission.
- Anopheles philippinensis: Predominantly located in certain areas of Southeast Asia, this species also contributes to malaria spread.
- Anopheles sundaicus: Present in coastal regions, it serves as a vector in specific geographic locales.
- India is home to approximately 45 different species of Anopheles mosquitoes. However, only a select few are recognized as effective malaria vectors. These include:
- Feeding Behavior and Implications for Transmission:
- The need for blood meals is essential for the female mosquito’s reproductive cycle. The act of feeding enables the uptake of malaria parasites from infected hosts, which can then be transmitted to new hosts during subsequent feedings.
- The timing of these feedings plays a crucial role in the malaria transmission cycle, as mosquitoes typically feed during twilight or nighttime, coinciding with human activity patterns.
- Environmental Factors Influencing Vector Populations:
- Factors such as climate, vegetation, and proximity to water bodies significantly impact Anopheles mosquito populations and their distribution.
- Seasonal changes can affect breeding sites, with stagnant water being a crucial breeding ground for these mosquitoes.
- Implications for Malaria Control Strategies:
- Understanding the specific species of Anopheles mosquitoes and their ecological requirements is vital for designing effective malaria control strategies.
- Interventions may include targeted insecticide applications, environmental management to reduce breeding sites, and community education to mitigate contact with mosquitoes.
Life Cycle of Malaria parasite
The life cycle of the malaria parasite is a complex process that involves two distinct hosts: the female Anopheles mosquito, which serves as the definitive host, and humans, who act as the intermediate host. This intricate life cycle encompasses both asexual and sexual reproduction phases, critical for the parasite’s survival and propagation.
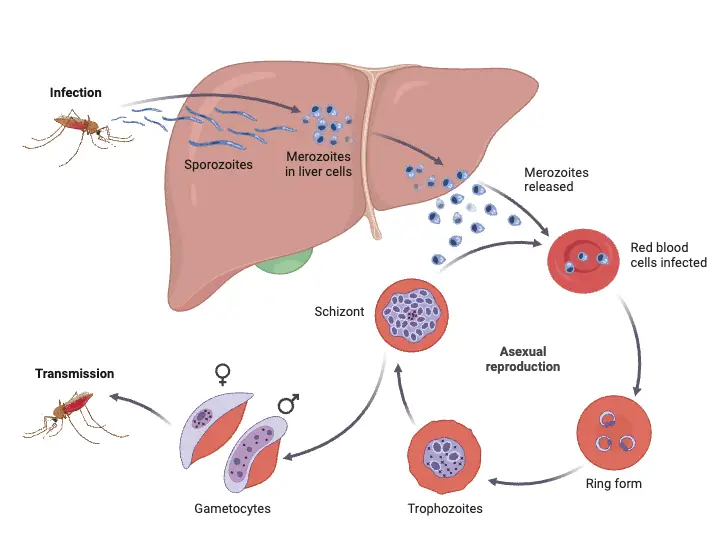
- Hosts Involved:
- Definitive Host: The female Anopheles mosquito, where the sexual phase of the parasite occurs.
- Intermediate Host: Humans, where the asexual phase takes place.
- Life Cycle Phases:
- The life cycle consists of two main stages:
- Asexual Phase: Occurs in humans (intermediate host).
- Sexual Phase: Occurs in the female Anopheles mosquito (definitive host).
- The life cycle consists of two main stages:
- Asexual Phase (Schizogony):
- This phase is characterized by the multiplication of the malaria parasite through a process known as schizogony, which involves division or splitting.
- It is often referred to as the vertebrate, intrinsic, or endogenous phase due to its occurrence within a vertebrate host (humans).
- Locations of Schizogony:
- Erythrocytic Schizogony: This occurs within the red blood cells, where the parasite proliferates and matures.
- Exoerythrocytic Schizogony: This phase takes place in the liver cells. It is crucial for the lifecycle, as it precedes the invasion of red blood cells. This step is also called pre-erythrocytic schizogony.
- Merozoites:
- The products of schizogony, termed merozoites, are released into the bloodstream after the destruction of red blood cells. These merozoites can then invade new red blood cells, perpetuating the cycle of infection.
- Sexual Phase (Sporogony):
- The sexual phase occurs within the female Anopheles mosquito after the uptake of gametocytes from an infected human during a blood meal.
- Within the mosquito, the gametocytes mature and fertilization occurs, leading to the production of sporozoites.
- Process of Sporogony:
- The fertilized gametes undergo a series of developmental stages, resulting in the formation of numerous sporozoites. This process is termed sporogony and is known as the invertebrate, extrinsic, or exogenous phase because it occurs outside the human host.
- Alternation of Generations:
- The life cycle of the malaria parasite demonstrates an alternation of generations, with the asexual generation occurring in humans and the sexual generation in mosquitoes.
- There is a cyclical exchange between hosts, with the completion of the asexual phase in humans leading to the initiation of the sexual phase in mosquitoes.
Human Cycle (Schizogony)
The human cycle of malaria, specifically the process known as schizogony, plays a crucial role in the life cycle of the Plasmodium parasite. This cycle begins with the infection of humans by the Anopheles mosquito, which serves as the primary vector. Understanding this cycle is vital for comprehending malaria transmission and pathology.
- Transmission of Infection:
- Human infection occurs through the bite of the female Anopheles mosquito.
- Infective forms of the parasite, known as sporozoites, are stored in the salivary glands of the mosquito.
- During a blood meal, the mosquito injects approximately 10-15 sporozoites into the bloodstream; however, in some cases, hundreds may be introduced.
- While many sporozoites are eliminated by the body’s phagocytic cells, a portion successfully migrates to the liver.
- Pre-Erythrocytic Stage (Tissue Stage or Exoerythrocytic Stage):
- Within about one hour post-injection, sporozoites infiltrate liver hepatocytes, marking the beginning of pre-erythrocytic schizogony.
- Sporozoites transform from elongated, spindle-shaped bodies into rounded forms within hepatocytes.
- They undergo multiple nuclear divisions, resulting in the formation of daughter nuclei, which are enveloped by cytoplasm. This structure is referred to as a schizont or meront.
- The increasing size of the schizont distorts the hepatocyte, pushing its nucleus to the cell’s periphery.
- Mature liver-stage schizonts can be multinucleate and measure approximately 45-60 µm in diameter, housing between 2000 to 50,000 merozoites.
- Unlike erythrocytic schizogony, liver-stage schizonts do not contain pigment. Typically, these mature forms rupture within 6-15 days, releasing thousands of merozoites into the bloodstream.
- Merozoites then proceed to infect red blood cells (RBCs) via invagination.
- Latent Stage:
- In the case of Plasmodium vivax and Plasmodium ovale, a subset of sporozoites forms hypnozoites, which enter a dormant state within hepatic cells. Occasionally, these hypnozoites may reactivate, leading to the formation of schizonts and subsequent merozoite release, causing clinical relapses.
- Conversely, in Plasmodium falciparum and Plasmodium malariae, the initial tissue phase is absent, and no hypnozoites are present. Here, a small number of erythrocytic parasites persist in the bloodstream, multiplying over time and contributing to short-term relapses or recrudescence.
- Erythrocytic Stage:
- The merozoites released from liver schizonts invade RBCs, with glycophorin serving as the receptor for these parasites. Variations in the glycophorin on RBCs account for the species specificity observed in malaria.
- Merozoites, approximately 1.5 µm long and possessing an apical complex (rhoptry), attach to erythrocytes by their apex. Once inside, they reside within a parasitophorous vacuole formed by the invagination of the red cell membrane.
- Inside the erythrocyte, the merozoite sheds its internal organelles, transforming into a rounded body with a central vacuole, cytoplasm pushed to the periphery, and a nucleus situated at one pole. This developmental stage is referred to as the ring form or young trophozoite.
- The parasite consumes the hemoglobin of the erythrocyte but incompletely metabolizes it, resulting in the production of hemozoin, also known as malaria pigment, which varies in appearance by Plasmodium species:
- P. vivax: Fine golden-brown dust-like particles.
- P. falciparum: Few solid black pigment blocks.
- P. malariae: Coarse dark brown particles.
- P. ovale: Numerous blackish-brown particles.
- Hemozoin released during the rupture of parasitized cells is subsequently taken up by reticuloendothelial cells, serving as histological evidence of previous malaria infections.
- Development of Trophozoites:
- As the ring form matures, it enlarges and acquires an irregular shape with amoeboid motility, referred to as the amoeboid form or late trophozoite form.
- Upon reaching a certain maturity level, the trophozoite undergoes mitosis followed by cytokinesis, resulting in mature schizonts or meronts, which contain 8-32 merozoites along with hemozoin.
- The rupture of mature schizonts liberates merozoites into circulation, where they can invade fresh erythrocytes and perpetuate the cycle of erythrocytic schizogony. This continuous cycle significantly increases parasitemia until the host’s immune response intervenes.
- Clinical Manifestations:
- The rupture of mature schizonts leads to the release of pyrogens, contributing to the febrile paroxysms commonly associated with malaria.
- The incubation period—the duration from sporozoite entry to the onset of clinical symptoms—differs from the prepatent period, which refers to the time from sporozoite entry to the first appearance of the malaria parasite in peripheral blood.
- In Plasmodium falciparum infections, erythrocytic schizogony occurs primarily within the capillaries and vascular beds of internal organs, resulting in schizonts and merozoites being rarely detected in peripheral blood.
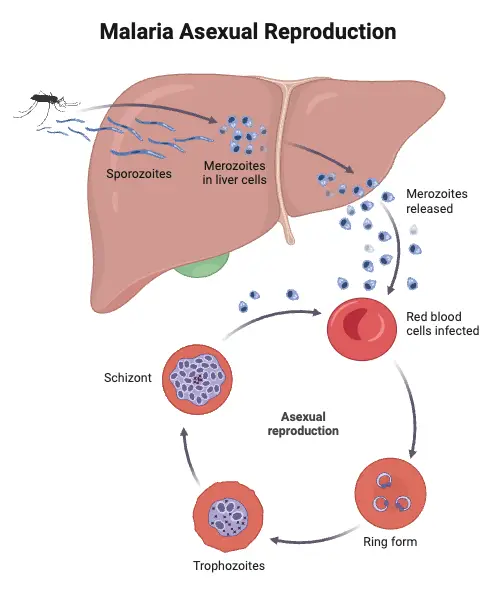
Gametogony
Gametogony is a critical stage in the life cycle of the Plasmodium parasite, facilitating the transition from asexual reproduction in humans to sexual reproduction in the mosquito vector. This process occurs after several cycles of erythrocytic schizogony and is essential for the continuation of the malaria transmission cycle.
- Development of Gametocytes:
- Following multiple erythrocytic cycles, certain merozoites do not mature into trophozoites or schizonts. Instead, they differentiate into gametocytes, the sexual forms of the parasite.
- Gametocytes gradually increase in size and nearly fill the erythrocyte, while the nucleus remains undivided throughout this process.
- Typically, the development of gametocytes takes place within the internal organs of the host, and only the mature forms are released into the bloodstream.
- Morphological Characteristics:
- Mature gametocytes exhibit distinct shapes across different Plasmodium species. They are generally round, except in Plasmodium falciparum, where they appear crescent-shaped.
- The female gametocyte, termed macrogametocyte, is larger than its male counterpart, the microgametocyte.
- Macrogametocytes possess dark blue-staining cytoplasm and a compact nucleus that stains deep red, indicating a higher density of cytoplasmic materials.
- Microgametocytes are smaller, with pale blue or pink cytoplasm and a larger, diffuse, pale-staining nucleus. The presence of pigment granules is notably prominent in both types.
- Quantitative Aspects:
- In the bloodstream, female gametocytes are usually more numerous than male gametocytes.
- The appearance of gametocytes in circulation occurs approximately 4-5 days after the first detection of asexual forms in the case of Plasmodium vivax and around 10-12 days for Plasmodium falciparum.
- Role in Malaria Transmission:
- Individuals carrying gametocytes in their blood serve as reservoirs for malaria transmission. Notably, gametocytes do not cause any clinical illness in the host; however, they are vital for the propagation of the infection.
- For mosquitoes to become infected with Plasmodium, a threshold concentration of 12 or more gametocytes per cubic millimeter of blood is required.
The Mosquito Cycle (Sporogony)
The sporogony phase represents a crucial segment in the life cycle of the Plasmodium parasite, occurring within the female Anopheles mosquito after it has fed on infected blood. This phase is fundamental for the transmission of malaria, as it facilitates the development of infectious sporozoites that will later infect humans.
- Ingestion of Gametocytes:
- When a female Anopheles mosquito takes a blood meal, it ingests parasitized red blood cells (RBCs) containing gametocytes.
- The asexual forms of the malaria parasite are digested, while the gametocytes are released in the midgut of the mosquito, where they undergo further development.
- Male Gametocyte Development:
- Male gametocytes undergo a process of division, wherein the nuclear material and cytoplasm separate to produce eight motile microgametes.
- This process, known as exflagellation, occurs rapidly, completing within approximately 15 minutes for Plasmodium vivax and Plasmodium ovale, and taking between 15 to 30 minutes for Plasmodium falciparum at a temperature of 25°C.
- Female Gametocyte Development:
- Zygote Transformation:
- The zygote initially appears as a motionless, round body. It gradually elongates and transforms into a motile form known as the ookinete, which possesses an apical complex at one end.
- The ookinete’s movement allows it to penetrate the epithelial lining of the mosquito’s stomach wall, positioning itself just beneath the basement membrane.
- Oocyst Development:
- The ookinete develops into an oocyst, a spherical structure approximately 500 µm in diameter.
- Within the oocyst, multiple sporozoites are produced. The mature oocyst eventually ruptures, releasing the sporozoites into the hemocoel (body cavity) of the mosquito.
- Infectivity of the Mosquito:
- Some of the released sporozoites migrate to the mosquito’s salivary glands, making the mosquito infectious.
- Upon feeding on a human host, the sporozoites are injected into the skin capillaries, initiating the cycle of human infection.
- Duration of Sporogony:
- The entire process of sporogony takes approximately 1 to 4 weeks to complete, a period referred to as the extrinsic incubation period. This duration varies based on environmental temperature and the specific species of Plasmodium.
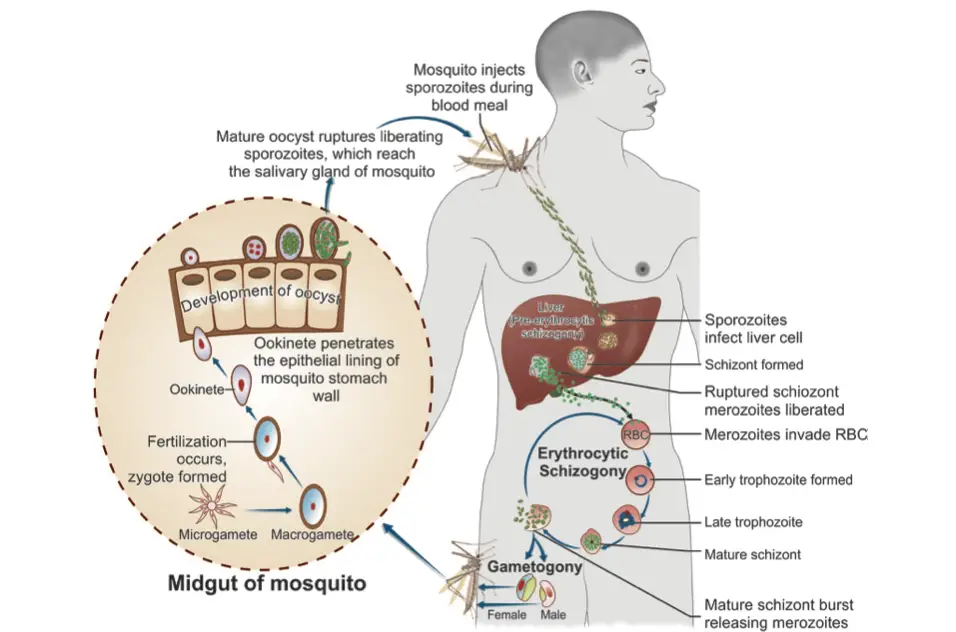
Types of Malarial Parasites
The genus Plasmodium encompasses several species that vary in their biological characteristics, geographical distribution, and clinical manifestations. Here, we discuss the four primary species of Plasmodium responsible for malaria in humans, outlining their distinct features and the implications for health.
- Plasmodium Vivax:
- P. vivax is the most widely distributed malaria parasite, found across tropics, subtropics, and temperate regions, accounting for approximately 80% of malaria infections.
- It predominantly causes benign tertian malaria, characterized by frequent relapses.
- The sporozoites are narrow and slightly curved, initiating two types of infection upon entering liver cells:
- Tachysporozoites develop into exoerythrocytic schizonts.
- Bradysporozoites persist as hypnozoites, entering a dormant state.
- The pre-erythrocytic schizogony lasts about eight days, yielding around 10,000 merozoites per tissue schizont.
- Merozoites preferentially infect reticulocytes, leading to a lower degree of parasitization (2-5% of red blood cells).
- The infected erythrocytes display Schuffner’s dots and become enlarged, with the trophozoite showing active motility.
- Schizont formation occurs after 36-40 hours, with the erythrocytic schizogony taking approximately 48 hours.
- Plasmodium Falciparum:
- Known for its high pathogenicity, P. falciparum causes malignant tertian malaria and is responsible for the majority of malaria-related deaths.
- This species shows a high rate of complications, often leading to fatal outcomes if untreated.
- The sporozoites are sickle-shaped, and the parasite undergoes only one cycle of pre-erythrocytic schizogony, releasing about 30,000 merozoites.
- P. falciparum targets both young and mature erythrocytes, resulting in a high parasitization rate, sometimes exceeding 50% in severe cases.
- The early ring forms are delicate and often appear as “appliqué” forms, sometimes binucleate. Mature schizonts are typically smaller compared to other species and have fewer merozoites (8-24, usually 16).
- Erythrocytic schizogony occurs rapidly, usually in less than 48 hours, with a febrile paroxysm periodicity of 36-48 hours.
- Gametocytes appear around 10 days after the initial ring stage, characterized by crescentic shapes, with females longer and more slender than males.
- Plasmodium Malariae:
- First discovered by Laveran in 1880, P. malariae causes quartan malaria, with fever paroxysms every four days.
- This species is noted for its long persistence in the bloodstream, remaining undetectable for years and potentially causing recrudescence under specific conditions.
- The pre-erythrocytic phase takes about 15 days, with schizonts releasing around 15,000 merozoites. Hypnozoites are absent.
- P. malariae preferentially infects older erythrocytes, exhibiting a low degree of parasitization, typically characterized by large pigment granules.
- The ring forms resemble those of P. vivax, but the old trophozoites appear as broad bands, a distinctive feature of this species.
- The erythrocytic schizogony lasts approximately 72 hours.
- Plasmodium Ovale:
- P. ovale produces a tertian fever similar to that caused by P. vivax, but the symptoms are usually milder, with prolonged latency and fewer relapses.
- This species is the rarest among human-infecting Plasmodium species, primarily found in tropical Africa.
- The pre-erythrocytic stage lasts about nine days, with mature liver schizonts releasing approximately 15,000 merozoites. Hypnozoites are present.
- Trophozoites resemble those of P. vivax, though they are more compact with abundant Schuffner’s dots.
- Infected erythrocytes exhibit an oval shape with fimbriated margins, contributing to the species name “ovale.”
Mixed Infections
Below is an overview of mixed infections, focusing primarily on Plasmodium vivax and Plasmodium falciparum.
- Mixed infections commonly occur when an individual harbours two or more species of Plasmodium parasites simultaneously.
- The most frequent combination encountered is that of P. vivax and P. falciparum. In these cases, there tends to be a predominance of one species over the other.
- Clinical manifestations of mixed infections can be atypical, with patients experiencing daily febrile episodes. This can lead to challenges in diagnosis and treatment, as the symptoms may not align with those typical of single-species malaria infections.
- The presence of mixed infections can result in altered disease severity. For example, the co-infection of P. falciparum—which is known for its severe pathogenicity—can exacerbate the clinical outcomes typically associated with P. vivax infections.
- Diagnosis of mixed infections is primarily conducted through the analysis of thin blood smears, which allow for the identification of the distinct parasitic forms characteristic of each species present in the bloodstream.
- It is crucial to note that the presence of mixed infections can complicate treatment protocols. Standard antimalarial therapies may not be equally effective against all species involved. Therefore, healthcare providers must carefully assess the species composition to tailor effective treatment regimens.
- Laboratory identification also aids in monitoring the progression of the disease and response to treatment. By observing changes in parasitic load, clinicians can adapt treatment plans accordingly.
- The epidemiological implications of mixed infections are significant. They highlight the need for public health interventions that address multiple species of malaria, ensuring comprehensive control measures are in place.
- Awareness of mixed infections is essential in endemic areas, as it necessitates improved diagnostic capabilities and treatment strategies that consider the presence of multiple Plasmodium species to mitigate the overall burden of malaria.
Pathogenesis of Malarial Parasites
- Clinical manifestations of malaria stem from the products of erythrocytic schizogony, wherein the malarial parasite reproduces within red blood cells. This leads to the release of toxic substances that provoke a systemic response from the host’s immune system.
- The disease primarily arises from the host’s local or systemic responses to parasite antigens, coupled with tissue hypoxia resulting from obstructed blood flow. Parasitized erythrocytes can impede circulation, leading to reduced oxygen delivery to various tissues.
- In the liver:
- The organ becomes enlarged and congested due to the accumulation of parasites within Kupffer cells, which are specialized macrophages in the liver.
- Parenchymal cells exhibit signs of fatty degeneration, atrophy, and necrosis, particularly centrilobular necrosis.
- Hemozoin pigments, a byproduct of hemoglobin digestion by the parasite, accumulate in liver cells, contributing to cellular damage.
- The brain is notably affected in Plasmodium falciparum infections:
- Congestion of the brain occurs as capillaries become occluded by parasitized red blood cells.
- During acute infections, the brain’s cut surface displays a slate grey cortex, while chronic cases show hardening of the spleen accompanied by multiple punctiform hemorrhages in subcortical areas.
- The thickened capsule of the spleen may appear slate grey, dark brown, or even black, indicative of pigment accumulation and fibrosis.
- In the spleen:
- The organ is soft, moderately enlarged, and congested during acute infections. In chronic cases, it becomes firmer with significant pigment deposits.
- The spleen actively contributes to anemia through the destruction of numerous unparasitized erythrocytes and by facilitating complement-mediated hemolysis.
- In the kidneys:
- The kidneys often become enlarged and congested, with glomeruli frequently containing malarial pigments.
- Tubular damage may occur, with hemoglobin casts appearing in renal tubules, reflecting the systemic impact of malaria.
- Anemia in malaria is multifactorial, arising from:
- The destruction of a substantial number of red blood cells.
- Decreased erythropoiesis in the bone marrow, exacerbated by the toxic effects of tumor necrosis factor (TNF).
- The host’s impaired ability to recycle iron bound in hemozoin pigments, leading to iron deficiency.
- Cytokines such as TNF, interleukin (IL)-1, and interferon (IFN)-gamma play critical roles in the pathogenesis of malaria-related end-organ disease:
- These cytokines contribute to inflammation and tissue damage, further complicating the host’s response and disease progression.
Clinical Features of Malarial Parasites
Malarial parasites, primarily transmitted through the bites of infected mosquitoes, exhibit a range of clinical features that vary in severity depending on the species involved. This variation encompasses benign forms, like those caused by Plasmodium vivax, to more severe manifestations linked to Plasmodium falciparum. Understanding the clinical features of these parasites is crucial for diagnosis and management.
- Benign Malaria
- Characterized by cyclical episodes of fever, the classic presentation includes:
- Periodic Fever: Typically marked by three distinct stages:
- Cold Stage: Lasts 15–60 minutes; the patient experiences intense cold and shivering.
- Hot Stage: Lasts 2–6 hours; fever spikes, often exceeding 41°C, causing discomfort and a feeling of intense heat.
- Sweating Stage: Following the hot stage, profuse sweating occurs, leading to a rapid decrease in temperature. Patients often fall into a deep sleep and wake feeling rejuvenated.
- Periodic Fever: Typically marked by three distinct stages:
- Fever Cycle: The febrile paroxysms typically begin in the early afternoon and last for 8–12 hours, correlating with the life cycle of the parasites in red blood cells.
- Tertian Malaria: Exhibits a periodicity of approximately 48 hours (seen in P. vivax, P. falciparum, and P. ovale).
- Quartan Malaria: Exhibits a periodicity of 72 hours (P. malariae).
- Quotidian Periodicity: A fever occurring every 24 hours may indicate dual infections or variations in parasite maturation.
- Additional Symptoms:
- Commonly includes severe headache, nausea, vomiting, anemia, splenomegaly, and hepatomegaly.
- Metabolic disturbances such as hypoglycemia or hyperglycemia may occur.
- Patients may experience both hyperkalemia and acidosis due to red blood cell lysis.
- Characterized by cyclical episodes of fever, the classic presentation includes:
- Malignant Tertian Malaria
- Caused by Plasmodium falciparum, this form is the most severe and can lead to life-threatening complications.
- Cerebral Malaria: The leading cause of mortality in this category.
- Symptoms include severe headaches, high fever, confusion, and potential paralysis.
- Typically occurs in non-immune individuals who remain untreated for extended periods.
- The pathogenic mechanism involves infected red blood cells forming adhesive surfaces that cause blockage in cerebral microvasculature, leading to ischemia and hemorrhage.
- Blackwater Fever: Associated with repeated infections or inadequate treatment.
- Characterized by hemoglobinuria (dark urine), severe vomiting, and potential renal failure.
- Pathophysiology involves intravascular hemolysis leading to significant renal complications.
- Algid Malaria: Presents with circulatory failure and symptoms like low blood pressure, cold clammy skin, and severe abdominal pain.
- Septicemic Malaria: Features high, continuous fever and widespread parasite dissemination, often leading to multiorgan failure with a mortality rate as high as 80%.
- Cerebral Malaria: The leading cause of mortality in this category.
- Caused by Plasmodium falciparum, this form is the most severe and can lead to life-threatening complications.
- Merozoite-Induced Malaria
- While typical malaria is initiated by sporozoites, direct injection of merozoites can cause clinical malaria in specific scenarios:
- Transfusion Malaria: Can occur if blood is transfused from an infected donor, with merozoites remaining viable for 1–2 weeks in blood banks.
- Congenital Malaria: Parasites may be transmitted from an infected mother to the fetus during pregnancy.
- Renal Transplantation and Shared Syringes: Both can also lead to transmission if the donor was infected.
- While typical malaria is initiated by sporozoites, direct injection of merozoites can cause clinical malaria in specific scenarios:
- Tropical Splenomegaly Syndrome (TSS)
- A chronic benign condition prevalent in certain endemic regions.
- Characterized by massive splenomegaly and elevated anti-malarial antibody levels despite the absence of detectable parasites in blood smears.
- Additional features include hypergammaglobulinemia, cryoglobulinemia, and normocytic normochromic anemia unresponsive to conventional treatments.
- Histopathological changes include congested spleen and liver with marked lymphocytic infiltration.
- A chronic benign condition prevalent in certain endemic regions.
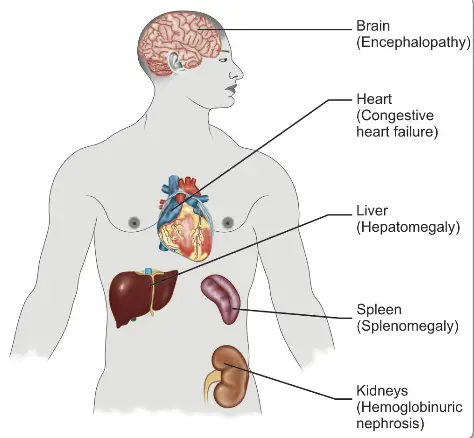
Immunity in malaria
Immunity in malaria encompasses both innate and acquired immune responses, each contributing to the host’s resistance against the malarial parasites. Understanding these mechanisms is vital for comprehending how individuals respond to malaria infections and can aid in the development of effective treatments and preventive strategies.
- Innate Immunity
- Innate immunity refers to the natural, non-specific defence mechanisms that the host possesses against malaria. Key aspects include:
- Duffy Negative Red Blood Cells: Individuals lacking the Duffy blood group antigens (Fya and Fyb) are resistant to infection by Plasmodium vivax. This phenomenon occurs because the merozoites require these specific receptors for successful invasion of red blood cells.
- Hemoglobin Variants: Certain hemoglobin types provide protection against malaria:
- Hemoglobin E: Offers natural defence against P. vivax.
- Sickle Cell Trait (HbS): Sickle cells are less conducive to the multiplication of P. falciparum, thus providing a survival advantage in malaria-endemic regions, particularly in Africa.
- Fetal Hemoglobin (HbF): Infants have increased resistance to all Plasmodium species due to the presence of HbF.
- Glucose-6-Phosphate Dehydrogenase (G6PD) Deficiency: This genetic trait, prevalent in Mediterranean, African, Middle Eastern, and Indian populations, is associated with an innate resistance to malaria.
- HLA-B53: This human leukocyte antigen has been linked to increased protection against malaria infections.
- Pregnancy Factors: P. falciparum infections are more severe during pregnancy, especially in first-time mothers. Iron supplementation may exacerbate this severity, highlighting the complex interplay of nutritional status and immunity.
- Role of the Spleen: The spleen is critical for filtering blood and removing infected cells. Splenectomy significantly enhances susceptibility to malaria.
- Innate immunity refers to the natural, non-specific defence mechanisms that the host possesses against malaria. Key aspects include:
- Acquired Immunity
- Acquired immunity develops as a result of exposure to the malarial parasites, leading to a more specific immune response. This form of immunity is characterized by:
- Induction of Specific Immunity: Infection triggers both humoral and cellular immunity, which can lead to clinical resolution of symptoms but does not eliminate the parasites completely from the body.
- Premunition: This concept describes a state where continued asymptomatic infections confer some level of resistance to superinfection. However, this immunity wanes once the parasite is cleared from the system.
- Humoral Antibodies:
- Antibodies directed against asexual forms of the parasite can inhibit red blood cell invasion and restrict parasite growth within these cells.
- Antibodies against sexual stages of the parasite are believed to diminish transmission rates.
- Maternal Antibody Transfer: In endemic areas, maternal antibodies are transferred to infants during pregnancy, providing passive immunity. This mechanism protects infants up to three months old against malaria.
- Development of Immunity in Children: Young children are particularly vulnerable to malaria. With age and exposure to subclinical or clinical infections, they gradually develop immunity, leading to a lower incidence of malaria in older children and adults.
- Acquired immunity develops as a result of exposure to the malarial parasites, leading to a more specific immune response. This form of immunity is characterized by:
Laboratory Diagnosis of Malarial Parasites
The laboratory diagnosis of malaria is pivotal in identifying the presence of malarial parasites within the host’s blood. Various methodologies have been developed to facilitate accurate detection, each with its specific advantages and limitations. The following points detail the primary diagnostic techniques employed in laboratory settings.
- Microscopy for Parasite Demonstration
- The detection of malarial parasites primarily involves microscopy, where blood samples are examined for the presence of the parasite.
- Thin Smears:
- Prepared from capillary blood, typically obtained from a fingertip. A second slide is employed at an angle of 30° to 45° to create a tail.
- An ideal thin smear consists of a uniform layer of red blood cells that tapers off at the edge of the slide.
- Thin smears are air-dried, fixed in alcohol, and stained using Romanowsky stains such as Giemsa, Leishman, or JSB stains.
- This method is essential for detecting the parasites and determining their species.
- Thick Smears:
- Thick smears can be prepared either on the same slide as the thin smear or separately.
- Typically involves spreading approximately three drops of blood over a small area (around 10 mm), resulting in a thicker layer than the thin smear.
- The thick film is air-dried and allowed to dehemoglobinise in a Koplin jar for 5 to 10 minutes without fixing in methanol.
- After staining, the film is examined under an oil immersion microscope, concentrating 20 to 30 layers of blood cells in a small area.
- Thick smears are more sensitive for detecting malarial parasites, particularly when parasite density is low (as few as 20 parasites per μL).
- Unlike thin smears, thick smears do not contain red blood cells, focusing solely on leukocytes and the parasites. This can hinder species identification due to distortion of the parasites.
- Quantitative Buffy Coat (QBC) Test
- The QBC test, developed by Becton-Dickinson, offers a rapid and sensitive alternative for diagnosing malaria.
- A small blood sample (50 to 110 μL) is centrifuged at high speed (12,000 revolutions per minute for 5 minutes).
- RBCs containing malaria parasites are less dense than normal RBCs and accumulate just beneath the leucocyte buffy coat.
- Tubes pre-coated with acridine orange enhance the visualization of parasites, which fluoresce due to their DNA content.
- Although QBC is faster and more sensitive than thick smears, it is less sensitive than conventional thick films and is relatively expensive. Nevertheless, careful microscopic examination remains the gold standard for malaria diagnosis.
- Microconcentration Technique
- This method involves collecting blood in microhematocrit tubes, which are then centrifuged at high speeds.
- The sediment is mixed with normal serum to prepare a smear, thereby increasing the positivity rate.
- However, this technique can alter the morphology of the parasites, potentially complicating identification.
- Culture of Malaria Parasites
- Initial attempts to culture malaria parasites date back to 1912, but significant advancements were made in 1976 with the development of a continuous culture method by Trager and Jensen for Plasmodium falciparum.
- The original culture method used a candle jar to maintain a specific atmosphere (3% oxygen, 10% carbon dioxide) along with a culture medium supplemented with serum.
- Periodic addition of fresh red blood cells is essential for the ongoing growth and multiplication of the parasites.
- Computer-controlled culture systems now allow for a steady supply of parasites, facilitating extensive research into their biology and pathology.
- Cultured parasites retain their infectivity and can be used for studies on antigenic structure, drug sensitivity testing, and immunoprophylaxis.
- Serodiagnosis
- Although serological tests are not typically helpful for clinical diagnosis, they are useful in epidemiological surveys and identifying infected blood donors.
- Tests employed include indirect hemagglutination (IHA), indirect fluorescent antibody tests (IFA), and enzyme-linked immunosorbent assays (ELISA).
- These tests cannot distinguish between active and past infections, limiting their application in acute diagnostic settings.
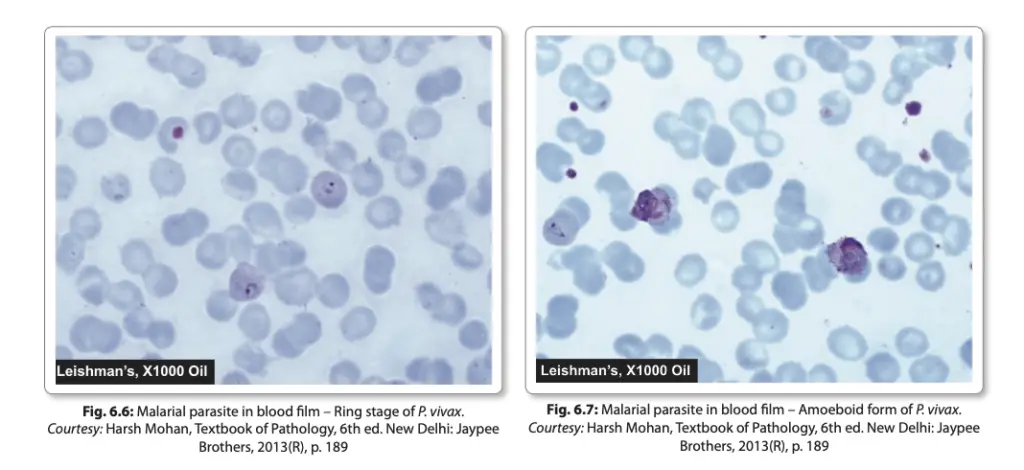
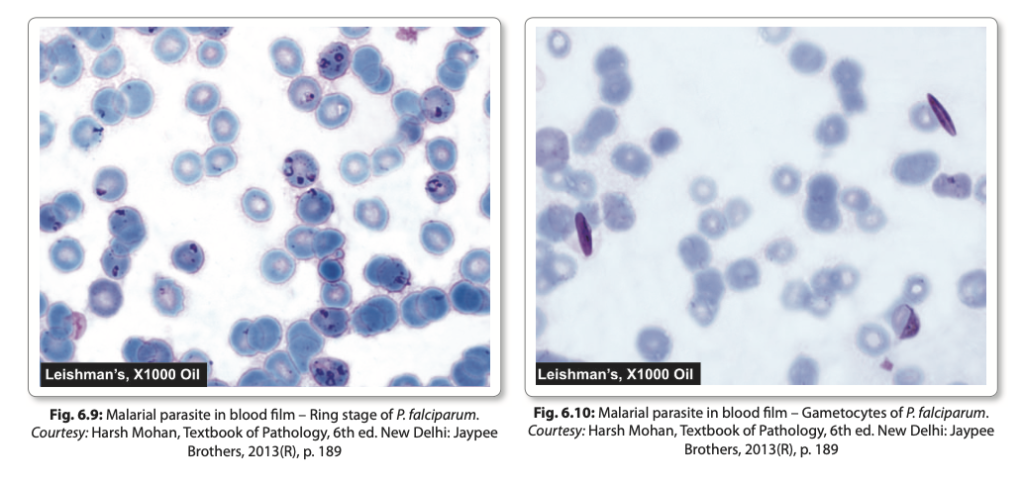
Newer Methods of Diagnosis for Malarial Parasites
The following points elaborate on the newer methods for diagnosing malaria:
- Fluorescence Microscopy
- This technique employs fluorescent dyes, such as acridine orange or benzothiocarboxy purine, which specifically stain malarial parasites within red blood cells (RBCs) while leaving white blood cells (WBCs) unstained.
- Acridine orange stains DNA as fluorescent green and cytoplasmic RNA as red, allowing for differential visualization of the parasites.
- The stained slide is examined using a fluorescence microscope, making this method particularly useful for mass screening in field laboratories due to its speed and efficiency.
- Rapid Antigen Detection Tests
- These tests utilise immunochromatographic methods to detect specific antigens associated with malaria parasites.
- Various formats, including dipsticks, cards, and cassettes, are available commercially, capable of detecting Plasmodium species within 15 minutes.
- Parasite-F Test:
- This test identifies histidine-rich protein-2 (HRP-2) antigen produced by the asexual stages of P. falciparum, which is expressed on the surface of infected RBCs.
- It employs monoclonal antibodies against the HRP-2 antigen (Pf band) and boasts a sensitivity of 98% and specificity, capable of detecting low asexual parasitemia of over 40 parasites/μL.
- Although quick (results within 10 minutes), it has limitations: it cannot detect the other three malaria species and may remain positive for up to two weeks post-treatment. Additionally, the Pf HRP-2 antigen is absent during the gametogony stage, potentially misleading interpretations in carrier states.
- Dual Antigen Test:
- This assay detects both parasite lactate dehydrogenase (pLDH) produced by trophozoites and gametocytes of all Plasmodium species, alongside the Pf HRP-2 antigen.
- It is a rapid two-site sandwich immunoassay that allows for specific detection and differentiation between P. falciparum and P. vivax in regions with high mixed infection rates.
- The test is advantageous for monitoring the success of antimalarial therapy, especially for P. vivax infections, as it only detects live parasites. However, it is relatively expensive and cannot differentiate between P. vivax, P. ovale, and P. malariae.
- Molecular Diagnosis
- DNA Probe:
- This highly sensitive method can detect fewer than 10 parasites/μL of blood, making it an invaluable tool for early diagnosis.
- Polymerase Chain Reaction (PCR):
- PCR has gained prominence for species specification and for detecting drug resistance mechanisms in malaria.
- Mutations in the PfCRT gene are associated with chloroquine resistance in P. falciparum, while point mutations in the PfMDR1 gene have also been implicated in in vitro resistance.
- Resistance to pyrimethamine and sulfadoxine is linked to mutations in dihydrofolate reductase (DHFR) and dihydropteroate synthase (DHPS) genes, respectively, while mutations in the PfATPase gene correlate with reduced susceptibility to artemisinin derivatives.
- DNA Probe:
- Other Diagnostic Tests
- Measurement of hemoglobin and packed cell volume (PCV) is crucial, particularly in cases of heavy parasitemia in children and pregnant women.
- Total white blood cell (WBC) and platelet counts are necessary in severe cases of P. falciparum malaria.
- Monitoring blood glucose levels is essential for detecting hypoglycemia, especially in young children and pregnant women with severe P. falciparum malaria, as well as in patients receiving quinine treatment.
- Coagulation tests, including measurements of anti-thrombin III levels, plasma fibrinogen, fibrin degradation products (FDPs), and prothrombin time (PTT), are important if abnormal bleeding is suspected in P. falciparum infections.
- Urine analysis for free hemoglobin is indicated if blackwater fever is suspected.
- Blood urea and serum creatinine measurements help monitor renal function.
- Screening for glucose-6-phosphate dehydrogenase (G6PD) deficiency is recommended before treatment with oxidative drugs such as primaquine.
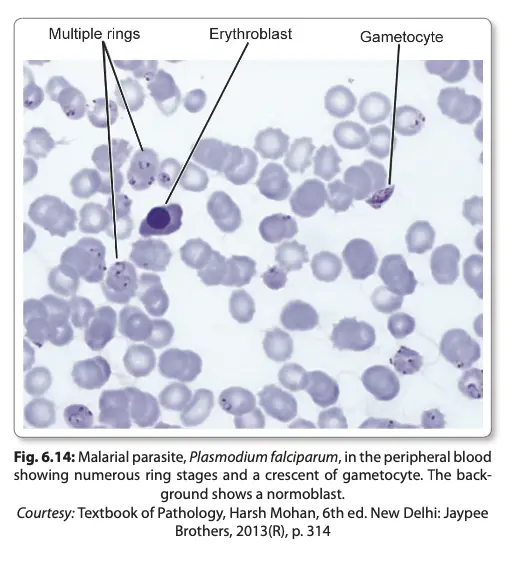
Treatment of Malarial
The treatment of malaria is a complex and evolving field, focusing on the eradication of the parasite, prevention of relapse, and reduction of transmission. Anti-malarial drugs serve various purposes, including clinical cure, prevention of relapse, prevention of transmission, and prophylaxis. The following points provide an overview of the treatment modalities for malaria, emphasizing specific objectives and the types of drugs used.
- Objectives of Anti-Malarial Treatment
- Therapeutic Cure: The primary goal is to eliminate the erythrocytic cycle of the malaria parasites, ensuring clinical recovery for the patient.
- Radical Cure: This aims to eradicate the exoerythrocytic cycle in the liver, effectively preventing future relapses.
- Gametocidal Action: This focuses on destroying gametocytes to halt transmission to mosquitoes, thereby reducing the human reservoir of the parasite.
- Chemoprophylaxis: This strategy is employed to prevent malaria infections in non-immune individuals visiting endemic areas.
- Commonly Used Anti-Malarial Drugs
- Established treatments include chloroquine, amodiaquine, quinine, pyrimethamine, doxycycline, sulfadoxine, proguanil, and primaquine.
- Newer agents, such as artemisinin, lumefantrine, mefloquine, and halofantrine, are increasingly utilized for treating multidrug-resistant P. falciparum infections.
- Treatment of Uncomplicated Malaria
- For confirmed cases of P. vivax, P. ovale, and P. malariae, chloroquine is administered at a dosage of 25 mg/kg, divided over three days.
- Given that P. vivax malaria can relapse due to the presence of hypnozoites in the liver, a relapse rate of approximately 30% is observed in India. To prevent this, primaquine is prescribed at a dosage of 0.25 mg/kg daily for 14 days under medical supervision.
- However, primaquine is contraindicated for individuals with G6PD deficiency, infants, and pregnant women.
- In instances of chloroquine resistance, quinine is administered at a dosage of 600 mg every eight hours for seven days, alongside doxycycline at 100 mg/day.
- Treatment of Complicated (Falciparum) Malaria
- The management of P. falciparum malaria is contingent on whether the strain is chloroquine-sensitive or resistant.
- In chloroquine-sensitive cases, treatment involves chloroquine (as previously mentioned) and primaquine at a dosage of 45 mg (0.75 mg/kg) as a single dose for its gametocidal effect.
- For chloroquine-resistant infections, artemisinin-based combination therapy (ACT) is recommended.
- ACT typically combines an artemisinin derivative with a long-acting anti-malarial agent, such as amodiaquine, lumefantrine, mefloquine, or sulfadoxine-pyrimethamine. In India, the national programme often employs artesunate combined with sulfadoxine-pyrimethamine.
- It is crucial that artemisinin derivatives are not used as monotherapy for uncomplicated malaria to avoid the development of resistance among parasites.
- Drug Resistance in Malarial Parasites
- The emergence of drug-resistant malaria, particularly P. falciparum, presents significant challenges for effective treatment. Resistance to commonly used quinoline and antifolate drugs is spreading throughout endemic regions.
- A drug-resistant parasite is defined as one that survives and proliferates despite receiving doses typically effective in curing the infection. Resistance can be classified as relative (where increased doses may overcome resistance) or complete (where even maximum tolerated doses are ineffective).
- The development of resistance often stems from spontaneous mutations in the parasite’s genome or gene duplications.
- To mitigate the emergence of resistance, it is essential to use drug combinations with distinct mechanisms of action targeting various biological pathways of the parasite.
- Classification of Drug Resistance
- The World Health Organization (WHO) delineates three levels of resistance:
- RI: Parasitemia decreases but recrudescence occurs post-treatment.
- RII: There is a partial reduction in parasitemia, but not a complete clearance.
- RIII: No reduction in parasitemia is observed following treatment.
- This classification relies on daily monitoring of trophozoite counts in blood films for seven days after treatment, along with assessments for any subsequent recrudescence. Patients exhibiting P. falciparum parasitemia of more than one trophozoite per high-power field in suspected drug resistance areas should be closely monitored for parasite clearance following treatment.
- The World Health Organization (WHO) delineates three levels of resistance:
Prophylaxis of Malarial
Various strategies, including vaccination, vector control, and chemoprophylaxis, have been developed to effectively reduce the incidence of malaria.
- Malaria Vaccines
- The pursuit of an effective malaria vaccine has seen substantial advancements over recent decades, though no completely effective vaccine is currently available.
- Several vaccine candidates targeting different stages of the Plasmodium life cycle have been investigated, with mixed results.
- Notably, the RTS,S/AS01 vaccine, commercially known as Mosquirix, targets the pre-erythrocytic stage of the parasite. This vaccine incorporates genes from the outer protein of P. falciparum along with a portion of the hepatitis B virus, augmented by a chemical adjuvant to enhance immune response.
- While previous candidates like SPf66 showed promise during clinical trials in the 1990s, they ultimately demonstrated inadequate efficacy.
- Vector Control Strategies
- Residual Spraying: This involves applying insecticides, such as dichloro-diphenyl-trichloroethane (DDT) or malathion, to the indoor surfaces of houses. This method helps reduce the number of mosquitoes that can transmit malaria.
- Space Application: This technique utilizes fogging or misting to release insecticidal formulations into the atmosphere, effectively killing adult mosquitoes.
- Individual Protection Measures: To minimize human-mosquito contact, various preventive measures are advised. These include using insect repellents, wearing protective clothing, employing insecticide-treated bed nets, using mosquito coils, and installing screens on windows and doors.
- For travelers to endemic regions, chemoprophylaxis is critical. Recommended drugs for prevention include proguanil, chloroquine, or mefloquine on a weekly basis, and doxycycline on a daily basis.
- Anti-Larval Measures
- Traditional anti-larval strategies, such as oiling standing water and using insecticides like Paris green, are being revisited due to increasing insecticide resistance among mosquito populations.
- Source Reduction: This encompasses various methods to decrease mosquito breeding sites, including drainage of stagnant water, filling in breeding areas, flushing out potential habitats, and managing water levels through techniques like intermittent irrigation.
- Integrated Control Strategies
- In order to diminish reliance on residual insecticides, there is a growing emphasis on integrated vector control methodologies. This approach combines environmental management with personal protection measures, creating a more holistic strategy for malaria prevention.
- Malaria Control Programmes
- The National Malaria Control Programme in India, initiated in 1958, aimed for the ultimate eradication of malaria. Initially, this program was successful, reducing the annual incidence from 75 million cases in 1953 to just 50,000 by 1961. However, setbacks occurred in subsequent decades, leading to a resurgence of cases.
- The situation necessitated a modified operational plan in 1977, which successfully lowered the incidence to 2.1 million cases by 1984.
- In response to ongoing challenges, the “Roll Back Malaria Initiative” was launched in 1998, a collaborative effort involving WHO, UNICEF, UNDP, and the World Bank. This initiative, through the National Vector Borne Disease Control Programme (NVBDCP), aims to reduce malaria cases and deaths significantly. The goal established was a 50% reduction in cases and fatalities by 2010, and a 75% reduction by 2015.
- Paniker’s Textbook of Medical Parasitology
- Buck E, Finnigan NA. Malaria. [Updated 2023 Jul 31]. In: StatPearls [Internet]. Treasure Island (FL): StatPearls Publishing; 2024 Jan-. Available from: https://www.ncbi.nlm.nih.gov/books/NBK551711/
- https://www.who.int/news-room/fact-sheets/detail/malaria
- https://www.medicalnewstoday.com/articles/150670
- https://www.cdc.gov/malaria/index.html
- https://emedicine.medscape.com/article/221134-overview?form=fpf
- https://www.mayoclinic.org/diseases-conditions/malaria/symptoms-causes/syc-20351184
- https://en.wikipedia.org/wiki/Malaria
- https://my.clevelandclinic.org/health/diseases/15014-malaria
- https://www.cdc.gov/malaria/about/index.html
- https://www.who.int/health-topics/malaria#tab=tab_1