What is Karyotyping?
- Karyotyping refers to the systematic procedure employed to visualize and analyze the entire chromosomal complement of an organism, encompassing their count, size, shape, and potential abnormalities. This process provides a comprehensive view of the chromosomes present in an individual’s cells, offering insights into their structural and numerical characteristics.
- A karyogram, often synonymous with an idiogram, is a graphical representation of a karyotype. In this depiction, chromosomes are typically arranged in pairs, sorted based on their size and the location of the centromere. When chromosomes of identical size are considered, the centromere’s position becomes the distinguishing factor. The process of karyotyping predominantly integrates light microscopy with photography during the metaphase stage of the cell cycle, culminating in a micrographic karyogram. However, a schematic karyogram diverges slightly, presenting a more stylized representation of the karyotype. In these schematics, for the sake of simplicity, usually only one of the sister chromatids of each chromosome is depicted. In actual photomicrographs, unless the resolution is exceptionally high, these chromatids often appear so proximate that they seem singular.
- The discipline dedicated to the comprehensive study of entire chromosome sets is termed karyology. A karyotype provides a detailed description of an organism’s chromosome count and their appearance under a light microscope. Emphasis is placed on various attributes such as their length, centromere position, banding patterns, disparities between sex chromosomes, and other notable physical traits. This meticulous examination and characterization of karyotypes fall under the broader scientific domain of cytogenetics.
- In terms of chromosomal count, the basic number in the somatic cells of an organism or individual is denoted as 2n, representing the diploid state. Conversely, in germ-line cells or sex cells, the chromosome count is halved, represented as n. For instance, in humans, the germ-line chromosome number is 23, leading to a diploid count of 2n = 46 in somatic cells. In standard diploid organisms, autosomal chromosomes exist in duplicate. Depending on the species, sex chromosomes may or may not be present. Furthermore, polyploid cells possess multiple chromosome copies, while haploid cells contain a singular copy.
- The utility of karyotyping extends beyond mere visualization. It serves as a pivotal tool in various domains such as detecting chromosomal aberrations, understanding cellular functions, deciphering taxonomic affiliations, and drawing inferences about historical evolutionary events, a field known as karyosystematics. Moreover, in the realm of medical genetics, karyotyping stands as a quintessential diagnostic instrument, facilitating the identification of chromosomal abnormalities, thereby aiding in medical prognosis and interventions.
- In summation, karyotyping offers a profound understanding of the chromosomal landscape of an organism, bridging the gap between cellular structures and their functional implications, especially in the context of genetic disorders and evolutionary biology.
Definition of Karyotyping
Karyotyping is a laboratory technique used to visualize and analyze the number, size, and shape of chromosomes in an individual’s cells, aiding in the detection of genetic abnormalities and chromosomal disorders.
What is Idiogram?
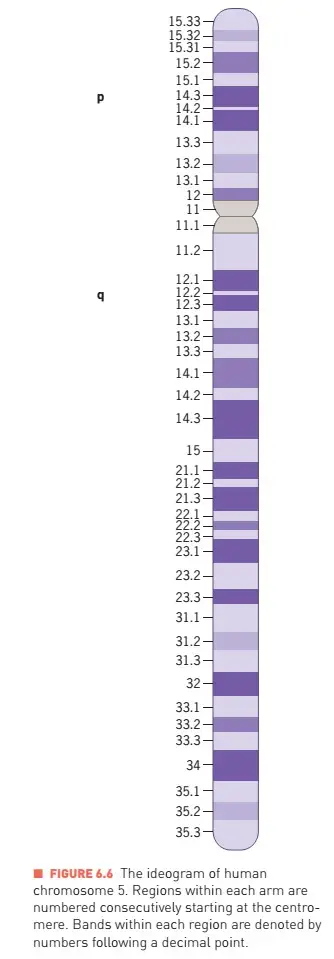
An idiogram is a diagrammatic representation of a karyotype, which showcases all the morphological features of chromosomes. These chromosomes are grouped and ordered based on the position of the centromere and their decreasing size. This representation provides a visual guide to the structure and organization of chromosomes within a cell.
- Techniques for Identification:
- Chromosome banding and painting techniques play a pivotal role in the identification of each chromosome. These methods are not only instrumental in distinguishing each arm of a chromosome but are also crucial for investigating specific regions within them.
- Centromere and Chromosome Arms:
- Some chromosomes possess a centromere that divides the chromosome into two distinct parts: the long arm and the short arm. The short arm is represented by the letter “p,” while the long arm is denoted by the letter “q.”
- Denotation of Chromosome Regions:
- For ease of reference, specific regions of a chromosome arm are denoted using a systematic approach. For instance, the short arm of chromosome 5 is represented as “5p.” Within this arm, regions are further classified. The region closest to the centromere is labeled as “5p11,” followed by “5p12,” “5p13,” “5p14,” and “5p15,” with the latter being the farthest from the centromere.
- Detailed Banding:
- Each region on a chromosome arm contains individual bands. These bands are further identified by numbers following a decimal point. For example, within the region “5p13,” the bands are labeled as “13.1,” “13.2,” and “13.3.” These bands provide a detailed map of the chromosome’s structure.
- Significance of Idiograms:
- The pattern of bands within a chromosome, known as an idiogram, is of paramount importance. It offers a visual representation of the chromosome’s structure, aiding in the study of genetics and the identification of any chromosomal abnormalities.
History of karyotype studies
The study of karyotypes, which involves the characterization and analysis of chromosomes, has a rich history that spans over a century. The journey of understanding karyotypes has been marked by significant discoveries and advancements.
- Initial Observations: The journey began in 1842 when Carl Wilhelm von Nägeli first observed chromosomes in plant cells. Later, in 1882, Walther Flemming described the behavior of chromosomes in animal cells, specifically in salamanders. He is credited with the discovery of mitosis. The term “chromosome” itself was coined in 1888 by Heinrich von Waldeyer, deriving from the Ancient Greek words “karyon” (meaning “kernel” or “nucleus”) and “typos” (meaning “general form”).
- Linking Chromosomes to Genetics: The early 20th century saw the rise of genetics as a scientific discipline. It was during this period that scientists began to understand that chromosomes, which can be visualized through karyotyping, were the carriers of genes. The concept of the karyotype, defined by the appearance of somatic chromosomes, was introduced by Grigory Levitsky in collaboration with other notable scientists of the time.
- Determining the Human Chromosome Count: One of the significant challenges in karyotype studies was determining the exact number of chromosomes in a normal diploid human cell. Early reports by Hans von Winiwarter in 1912 suggested 47 chromosomes in spermatogonia and 48 in oogonia. However, it was Joe Hin Tjio, working in Albert Levan’s lab, who conclusively determined the chromosome count to be 46. He employed advanced techniques, such as:
- Using tissue culture cells.
- Pretreating cells in a hypotonic solution to spread the chromosomes.
- Arresting mitosis in metaphase using colchicine.
- Squashing the preparation to force chromosomes into a single plane.
- Creating a karyogram by cutting up a photomicrograph.
- Comparison with Great Apes: While humans possess 46 chromosomes, other great apes have 48. A significant discovery was the realization that human chromosome 2 resulted from the end-to-end fusion of two ancestral ape chromosomes. This fusion left behind distinctive remnants of telomeres and a vestigial centromere.
Types of karyotype on the basis of symmetry
Karyotyping, a technique developed by G. Levitzky in 1931, offers a comprehensive view of the chromosomal structure within an organism’s cells. On the basis of symmetry, karyotypes can be broadly categorized into two primary types: symmetrical and asymmetrical karyotypes.
- Symmetrical Karyotype: In a symmetrical karyotype, all chromosomes within a set exhibit uniformity in size. Furthermore, these chromosomes possess either a median or sub-median centromere. This type of karyotype is often referred to as a homogenous karyotype. Therefore, due to its uniform nature, it is considered to be a primitive form of karyotype.
- Asymmetrical Karyotype: Contrastingly, an asymmetrical karyotype presents a notable degree of variation in the size of the chromosomes. Besides the size variation, there is also a distinct difference in the position of the centromere. In these karyotypes, the centromere’s position can range from median or sub-median to more extreme positions like sub-terminal or terminal. Then, due to its varied nature and the advanced positioning of the centromere, this type of karyotype is often regarded as a specialized or advanced karyotype.
Parameters used in karyotype preparation
Karyotype preparation is a meticulous process that requires a detailed examination of chromosomes to provide insights into their structure and organization. Several specific parameters are employed to ensure the accurate representation and analysis of the chromosomal complement. The following elucidates these parameters in a sequential and detailed manner:
- Number of Chromosomes in the Set: The first and foremost parameter is determining the total count of chromosomes present in a particular set. This count provides foundational information about the genetic makeup of an organism.
- Relative Length of Chromosome: Besides the count, the relative length of each chromosome is also assessed. This parameter offers insights into the size variations among different chromosomes within the set.
- Arm Ratio: The arm ratio is a critical parameter that denotes the ratio of the length of the long arm to the short arm of a chromosome, measured from the position of the centromere. This ratio aids in distinguishing between chromosomes based on the proportionate lengths of their arms.
- Position of Secondary Constriction: Another vital parameter is the identification of the position of the secondary constriction on the chromosome. This constriction, distinct from the primary centromere, plays a role in the formation of the nucleolus.
- Position and Size of Nucleolus and Satellite: The nucleolus, an essential cellular component involved in ribosomal RNA synthesis, and the satellite, a protrusion connected to the chromosome by the secondary constriction, are both examined in terms of their position and size. These features can vary among chromosomes and are crucial for accurate karyotype analysis.
- Staining Reaction and Differentiation of Bands: Lastly, the chromosomes undergo staining to visualize the bands of euchromatin and heterochromatin. Euchromatin and heterochromatin are regions with varying degrees of chromatin condensation. The staining reactions help differentiate these regions, providing insights into the functional areas of the chromosome.
Human karyotype
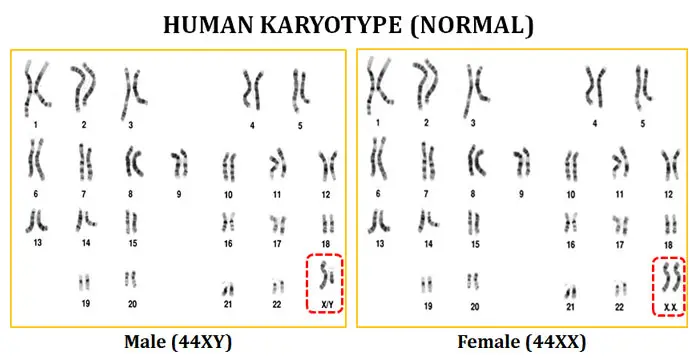
The human karyotype is a detailed representation of the chromosomal composition within human cells. It provides a comprehensive insight into the number, structure, and organization of chromosomes, which are essential carriers of genetic information. The following is a sequential and detailed explanation of the human karyotype:
- Chromosomal Count and Composition: Diploid human cells, which contain two sets of chromosomes, have a total of 46 chromosomes. Out of these, 44 are autosomes, which are non-sex chromosomes, and the remaining two are sex chromosomes. The combination of these sex chromosomes determines the gender of an individual. Specifically, females possess two X chromosomes (XX), while males have one X and one Y chromosome (XY).
- Chromosomal Structure at Mitotic Metaphase: During the mitotic metaphase, a stage in cell division, each of the 46 chromosomes is composed of two identical sister chromatids. When subjected to specific staining techniques, these chromatids exhibit unique banding patterns. Therefore, based on their size, shape, and these banding patterns, each chromosome can be distinctly identified.
- Size and Identification of Autosomes: Among the autosomes, chromosome 1 stands out as the largest, serving as a reference point for size comparison. On the other end of the spectrum, chromosome 21 is the smallest, designated by its number. These size variations among autosomes are crucial for their identification and analysis.
- Sex Chromosomes and Their Characteristics: Besides autosomes, the human karyotype also comprises sex chromosomes, which play a pivotal role in determining an individual’s gender. The X chromosome is intermediate in size, falling between the largest and smallest autosomes. In contrast, the Y chromosome, which is exclusive to males, is roughly equivalent in size to chromosome 22.
Observations on karyotypes
Karyotypes provide a systematic representation of the chromosomal composition within an organism’s cells. The study and observation of karyotypes are facilitated by specific techniques and characteristics, which offer insights into the structure, organization, and variations of chromosomes.
1. Staining Techniques: The primary method that enables the study of karyotypes is staining. Typically, a dye, such as Giemsa, is applied to cells that have been halted during cell division, usually in the metaphase or prometaphase stages when chromosomes are most condensed. To ensure the effective adherence of the Giemsa stain, all chromosomal proteins must undergo digestion and removal. In humans, white blood cells are the preferred choice for this process due to their ease of division and growth in tissue culture. Occasionally, observations might be conducted on non-dividing cells, such as interphase cells, which can be instrumental in predicting the sex of an unborn fetus through the observation of Barr bodies.
2. Key Observational Characteristics: When analyzing karyotypes, six distinct characteristics are typically observed and compared:
- Absolute Sizes of Chromosomes: Chromosomes can exhibit significant size variations, even within genera of the same family. Such differences often reflect varying degrees of DNA duplication.
- Position of Centromeres: Variations in the location of centromeres can arise from translocations.
- Relative Size of Chromosomes: Differences in the relative size of chromosomes can emerge from segmental interchanges of unequal lengths.
- Basic Number of Chromosomes: Variations in the basic chromosome number can result from unequal translocations or fusion events. For instance, humans possess one chromosome pair fewer than the great apes due to a fusion event.
- Satellites: The number and position of satellites, which are small bodies connected to a chromosome by a thread, can vary.
- GC Content: The degree and distribution of GC content (Guanine-Cytosine pairs) can differ. DNA with a high GC content is usually less condensed and contains more coding DNA, making it more transcriptionally active. This DNA appears lighter when stained with Giemsa, producing the characteristic “G-Bands”.
3. Variations in Karyotypes: Karyotypes can exhibit variations in several contexts:
- Between the Sexes: Differences can be observed between male and female karyotypes.
- Germ-line vs. Soma: Variations can exist between gametes and the rest of the body.
- Population Differences: Chromosome polymorphism can be observed within a population.
- Geographic Specialization: Certain regions may exhibit specialized karyotypic features.
- Mosaics or Abnormal Individuals: Individuals with chromosomal abnormalities or mosaics can display unique karyotypic characteristics.
In conclusion, the observation of karyotypes involves a detailed examination of various chromosomal characteristics and variations. Understanding these features and differences is crucial for genetic research, diagnosis of genetic disorders, and evolutionary studies.
Karyotyping Procedural Steps
Karyotyping is a technical procedure used to visualize and analyze the chromosomal composition of an individual. The following are the sequential steps involved in the karyotyping process:
- Sample Collection: The initial step in karyotyping involves obtaining a cell sample. This sample can be sourced from various locations, including tissue, amniotic fluid, or blood. Therefore, the choice of sample largely depends on the purpose of the karyotyping and the source that is most accessible.
- Induction of Cell Division: Once the sample is collected, it is subjected to laboratory conditions that promote cell division. During this phase, the chromosomes within the cells become more condensed and visible, especially during the metaphase stage of cell division. Besides, metaphase is the optimal stage for visualizing chromosomes due to their highly condensed state.
- Staining of Chromosomes: After ensuring that the cells have reached the metaphase stage, the next step involves staining the chromosomes. Giemsa or other specialized dyes are employed for this purpose. These dyes produce a unique pattern of alternating dark and light bands on the chromosomes. Then, these banding patterns facilitate the identification and organization of the chromosomes based on their size and shape.
- Analysis and Representation: The final step in the karyotyping procedure is the creation of a karyogram. This is a graphical representation of an individual’s chromosomal complement. Each chromosome’s number and appearance in the karyogram provide a detailed insight into the person’s genetic makeup. Therefore, any anomalies or variations in the chromosomes can be easily detected and studied.
Detection of Chromosome abnormalities
Chromosome abnormalities are deviations from the normal chromosomal number or structure in an organism. These abnormalities can be broadly categorized into numerical and structural abnormalities.
- Numerical Abnormalities: These involve the presence of extra or missing chromosomes. Such abnormalities are termed aneuploidy. A common cause of numerical abnormalities is nondisjunction during meiosis, which occurs during the formation of gametes. Examples of numerical abnormalities include:
- Trisomies: Where three copies of a chromosome are present instead of the usual two. Notable trisomies include:
- Down Syndrome: Caused by trisomy of chromosome 21.
- Edwards Syndrome: Resulting from trisomy of chromosome 18.
- Patau Syndrome: Due to trisomy of chromosome 13.
- Trisomy 9: This is believed to be the fourth most common trisomy. Affected individuals often function well but may have speech difficulties.
- Other documented trisomies include trisomy 8 and trisomy 16, although they generally do not survive to birth.
- Trisomies: Where three copies of a chromosome are present instead of the usual two. Notable trisomies include:
- Structural Abnormalities: These arise from errors in homologous recombination and involve changes in the structure of chromosomes. Examples include:
- Translocations: Such as the Philadelphia chromosome, commonly associated with chronic myelogenous leukemia.
- Inversions: Where a segment of a chromosome is reversed end to end.
- Deletions: Such as in Cri du chat syndrome, caused by a truncated short arm on chromosome 5, and 1p36 Deletion syndrome, resulting from the loss of part of the short arm of chromosome 1.
- Duplications: Where a segment of the chromosome is duplicated and appears more than once.
- Specific Chromosomal Disorders in Humans: Several chromosomal abnormalities lead to specific disorders in humans. For instance:
- Turner Syndrome: Resulting from a single X chromosome.
- Klinefelter Syndrome: Caused by an extra X chromosome.
- Angelman Syndrome: 50% of cases have a segment of the long arm of chromosome 15 missing due to a deletion of the maternal genes.
- Prader-Willi Syndrome: 50% of cases have a segment of the long arm of chromosome 15 missing due to a deletion of the paternal genes.
Depiction of karyotypes
Karyotyping is a technique used to visualize and analyze the number, size, and shape of chromosomes in an organism. Over the years, various methods have been developed to enhance the depiction of karyotypes, each with its unique features and applications.
- Types of Banding:
- G-banding: This method uses Giemsa stain after treating chromosomes with trypsin. It results in a series of light and dark bands. The dark regions are heterochromatic, late-replicating, and AT-rich, while the light regions are euchromatic, early-replicating, and GC-rich.
- R-banding: This is the reverse of G-banding, where dark regions are euchromatic and light regions are heterochromatic.
- C-banding: This method stains centromeres by binding to constitutive heterochromatin.
- Q-banding: Uses quinacrine for staining, producing a fluorescent pattern similar to G-banding.
- T-banding: This method visualizes telomeres.
- Silver staining: This technique stains the nucleolar organization region-associated protein, highlighting the activity of rRNA genes.
- Classic Karyotype Cytogenetics:
- In this traditional method, dyes like Giemsa or quinacrine are used to stain bands on chromosomes. Each chromosome exhibits a unique banding pattern, aiding in their identification.
- Karyotypes are typically arranged with the short arm (p) on top and the long arm (q) at the bottom. Different stained regions are numerically designated based on their position on the chromosome arms.
- Multicolor FISH (mFISH) and Spectral Karyotype (SKY Technique):
- These molecular cytogenetic techniques allow for the visualization of all chromosome pairs in different colors simultaneously.
- Fluorescently labeled probes specific to each chromosome are used. A combination of fluorophores generates various colors, which are captured and analyzed using specialized microscopes and software.
- These techniques are particularly useful for analyzing chromosomal rearrangements.
- Digital Karyotyping:
- This technique quantifies DNA copy number across the genome. It involves isolating and enumerating short DNA sequences from specific genomic loci.
- Digital karyotyping can detect small genomic alterations that might be missed by traditional methods. It’s especially valuable for identifying loci deletions associated with conditions like cancer.
Diversity and evolution of karyotypes
Karyotypes, the number and appearance of chromosomes in the nucleus of a eukaryotic cell, exhibit significant diversity across species. This variability in karyotypes has provided a rich foundation for studies in evolutionary cytology.
- Variability in Karyotypes:
- Eukaryotic DNA replication and transcription processes are standardized. However, karyotypes are not. They differ in chromosome number and organization, even though they are constructed from identical macromolecules. This variability is a cornerstone for evolutionary cytology studies.
- Within some species, there’s notable karyotypic variation. Despite numerous studies, the significance of karyotype evolution remains elusive.
- Changes during Development:
- Some organisms adjust their karyotype by eliminating heterochromatin or making other visible adjustments. Examples include chromosome elimination in sciarid flies and chromatin diminution in organisms like the roundworm Ascaris suum.
- X-inactivation in mammals, where one X chromosome is inactivated, results in females being mosaics concerning their X chromosomes.
- Number of Chromosomes:
- The number of chromosomes varies widely between unrelated species. For instance, the nematode Parascaris univalens has a haploid number of 1, while the fern Ophioglossum has an average of 1262 chromosomes.
- The fundamental number (FN) of a karyotype represents the number of visible major chromosomal arms per set. Humans, for instance, have an FN of 82.
- Ploidy and Polyploidy:
- Ploidy refers to the number of complete sets of chromosomes in a cell. Polyploidy, with more than two sets of chromosomes, is common in plants and has played a significant role in plant evolution.
- Haplo-diploidy, where one sex is diploid and the other haploid, is common in the Hymenoptera group.
- Endopolyploidy occurs when adult differentiated tissues have cells with more than the typical somatic number of chromosomes.
- Aneuploidy:
- Aneuploidy is when the chromosome number in cells isn’t typical for the species. This can result in developmental defects, such as Down syndrome.
- Aneuploidy can also occur within a group of closely related species, leading to variations in chromosome numbers.
- Chromosomal Polymorphism:
- Some species display polymorphism for different chromosome structural forms. This can be associated with different numbers of chromosomes in different individuals.
- The study of chromosome banding, especially in insects with polytene chromosomes, can reveal relationships between closely related species. An example is the study of Hawaiian drosophilids by Hampton L. Carson.
- Chromosome Banding:
- Chromosomes exhibit banded patterns when stained. These bands are alternating light and dark stripes along the chromosomes. Unique banding patterns help identify chromosomes and diagnose chromosomal aberrations.
- Different chromosome treatments produce various banding patterns, including G-bands, R-bands, C-bands, and more.
Applications of Karyotyping
- Detection of Chromosomal Abnormalities: One of the primary applications of karyotyping is in the field of medical genetics, where it plays a crucial role in identifying chromosomal anomalies that lead to genetic disorders. For instance:
- Numerical Abnormalities: Karyotyping can detect numerical anomalies such as trisomy 21, responsible for Down syndrome, and monosomy X, which leads to Turner syndrome. Therefore, it provides valuable insights into the genetic basis of these conditions.
- Structural Abnormalities: Besides numerical anomalies, karyotyping can also identify structural abnormalities like translocations, inversions, or deletions. These abnormalities can result in diseases such as leukaemia, lymphoma, or muscular dystrophy.
- Prenatal Diagnostics: In the realm of prenatal care, karyotyping is employed to examine samples of amniotic fluid or chorionic villi. Then, this analysis helps in detecting chromosomal abnormalities in the developing fetus, ensuring timely interventions if required.
- Gender Determination: Another significant application of karyotyping is in determining an individual’s gender. Humans possess sex chromosomes, with males having one X and one Y chromosome, while females possess two X chromosomes. By analyzing the presence or absence of the Y chromosome through karyotyping, one can conclusively establish a person’s gender.
- Evolutionary Biology Studies: Karyotyping also finds its application in the field of evolutionary biology. Scientists utilize karyotyping to:
- Investigate Evolutionary Relationships: By comparing the karyotypes of different species, researchers can deduce the evolutionary history and points of divergence between species.
- Chromosomal Variations: For example, while chimpanzees have 24 pairs of chromosomes, humans possess only 23 pairs. The difference in chromosomal number is attributed to the fusion of two ancestral chromosomes in the human lineage, which occurred post the species’ divergence from the chimpanzee lineage.
Limitations of karyotyping
- Limited Genome Analysis: One of the primary limitations of karyotyping is its restricted scope in genome analysis. This test can only detect a small portion of the genome, specifically the number or shape of chromosomes visible during metaphase. Therefore, it provides only a superficial view of the genetic makeup.
- Inability to Detect Certain Anomalies: Karyotyping cannot identify anomalies such as epigenetic changes or mutations that influence gene expression. These changes, which can have significant biological implications, remain undetected in a standard karyotyping analysis.
- Requirement of Sufficient Cells: For a successful karyotyping analysis, a substantial number of cells are needed. This becomes a challenge in certain situations, such as when analyzing early embryos or fetal tissues. In such cases, obtaining an adequate number of cells for analysis might not be feasible.
- Constraints in Gender Determination: While karyotyping can indicate the presence or absence of a Y chromosome, this information is not always conclusive for determining an individual’s gender. There are rare instances where individuals with ambiguous genitalia or differences in sex chromosome composition cannot be accurately identified using karyotyping alone.
- Limitations in Evolutionary Analysis: When examining interspecies evolutionary relationships, karyotyping has several drawbacks:
- Resolution Constraints: The resolution of chromosomal bands in karyotyping might not be sufficient to discern minute variations in chromosome structure or gene content. Therefore, subtle genetic differences between species might go unnoticed.
- Inability to Explain Certain Evolutionary Phenomena: Karyotyping cannot elucidate convergent or parallel evolution. In these phenomena, related features evolve independently across multiple lineages. Thus, karyotyping falls short in providing a comprehensive evolutionary narrative.