What is Glycogenesis?
- Glycogenesis is the biological process by which glycogen, a branched polymer of glucose, is synthesized from glucose molecules. This process is vital for maintaining glucose homeostasis and providing a reliable energy reserve. Glycogen synthesis primarily occurs in the liver and skeletal muscles, where glucose is stored for later use when the body’s immediate glucose supply is depleted.
- The process begins with the activation of glucose-6-phosphate, which is isomerized into glucose-1-phosphate. This intermediate is then converted into UDP-glucose, a high-energy precursor, by the enzyme UDP-glucose pyrophosphorylase. Glycogen synthase, the key enzyme in glycogenesis, catalyzes the addition of glucose units from UDP-glucose to a pre-existing glycogen primer. The branching enzyme, amylo-(1,4 to 1,6)-transglucosidase, introduces branches into the glycogen molecule, enhancing its solubility and accessibility.
- Glycogenesis is tightly regulated by hormonal signals. Insulin, released in response to elevated blood glucose levels, activates glycogen synthase and promotes glycogen storage. In contrast, epinephrine and glucagon inhibit glycogenesis, favoring glycogenolysis to release glucose during periods of low blood sugar or high energy demand. Calcium ions also play a role in regulating glycogen metabolism, particularly in muscle tissues during physical activity.
- This process is essential for energy balance. After glucose intake, glycogenesis helps store excess glucose as glycogen. During fasting or between meals, the body can access these reserves through glycogenolysis, the breakdown of glycogen back into glucose. Unlike fats, which serve as long-term energy stores, glycogen provides a rapid source of glucose to meet immediate energy needs.
Definition of Glycogenesis
Glycogenesis is the biological process of synthesizing glycogen from glucose molecules, primarily in the liver and muscles, for energy storage and glucose homeostasis.
Key Enzymes in Glycogenesis
Glycogenesis is the biochemical process that converts glucose into glycogen for storage in the liver and muscle tissues. This process relies on a series of enzymes that work together to facilitate the conversion. Here’s an overview of the key enzymes involved in glycogenesis, their specific functions, and the steps they contribute to.
- Hexokinase/Glucokinase
- Function: The process begins with the phosphorylation of glucose to form glucose-6-phosphate (G6P).
- Hexokinase is present in most tissues, while glucokinase is specific to the liver and pancreatic beta cells.
- Reaction:
- Glucose + ATP → Glucose 6-phosphate + ADP
- Phosphoglucomutase
- Function: This enzyme converts glucose-6-phosphate into glucose-1-phosphate (G1P), which is the next step in glycogen synthesis.
- Reaction:
- Glucose 6-phosphate ⇌ Glucose 1-phosphate
- UDP-Glucose Pyrophosphorylase
- Function: This enzyme converts glucose-1-phosphate into UDP-glucose, the activated form of glucose that will be used in glycogen synthesis.
- Reaction:
- Glucose 1-phosphate + UTP → UDP-glucose + PPi
- Glycogenin
- Function: Glycogenin starts the glycogen synthesis process by autoglycosylation. It adds the first few glucose units (around seven) from UDP-glucose to itself, creating a short primer for glycogen synthase to extend.
- Mechanism: Glycogenin catalyzes the transfer of glucose from UDP-glucose to a tyrosine residue on its own structure.
- Glycogen Synthase
- Function: This enzyme plays the central role in glycogenesis. It elongates the glycogen chain by adding UDP-glucose to the non-reducing end of the growing glycogen molecule, forming α(1→4) glycosidic bonds.
- Regulation: The activity of glycogen synthase is regulated by insulin and is allosterically activated by glucose-6-phosphate.
- Glycogen Branching Enzyme (GBE)
- Function: GBE introduces α(1→6) branch points into the glycogen molecule, making it more soluble and allowing for efficient mobilization during glycogenolysis.
- Mechanism: GBE transfers a segment of 7–11 glucose residues from a growing chain to a new branch point, forming the characteristic branched structure of glycogen.
Steps of Glycogenesis
Glycogenesis, the process of glycogen synthesis, involves several steps that allow the body to store glucose in the form of glycogen for future energy needs. These steps take place primarily in the liver and muscle cells, following a precise sequence of enzymatic reactions.
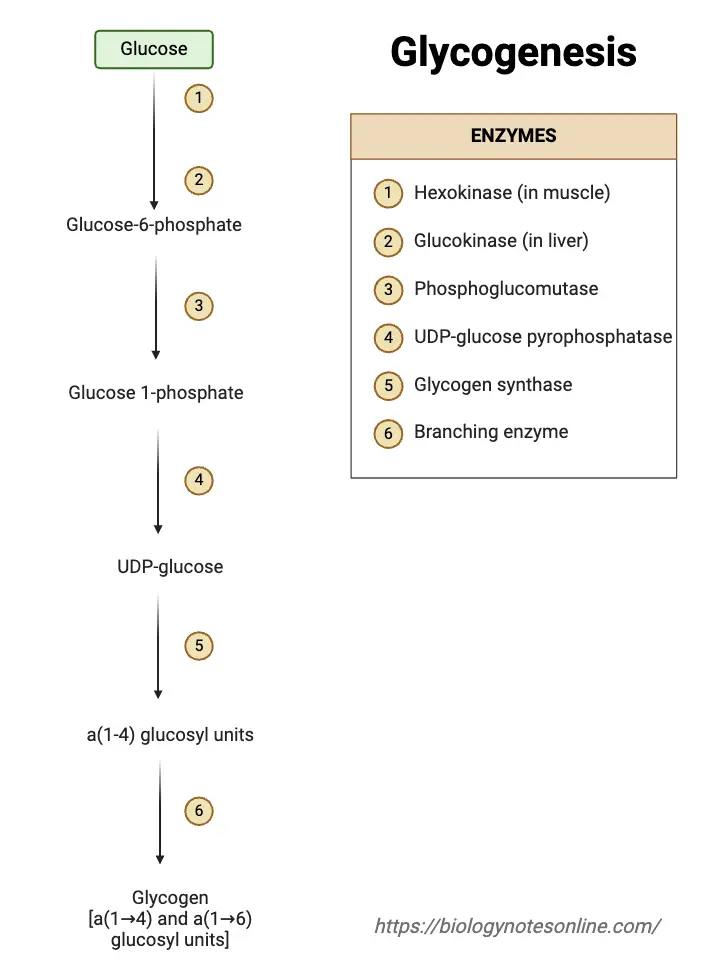
- Glucose Uptake
- Glucose is transported into liver and muscle cells via glucose transporters like GLUT2 and GLUT4.
- This allows cells to obtain glucose for glycogen synthesis, ensuring that the process starts with an adequate supply.
- Phosphorylation to Glucose-6-Phosphate
- Inside the cell, glucose is converted to glucose-6-phosphate (G6P) by the enzyme hexokinase (or glucokinase in the liver).
- This phosphorylation traps glucose inside the cell and prepares it for further processing.
- Isomerization to Glucose-1-Phosphate
- Glucose-6-phosphate is converted into glucose-1-phosphate (G1P) by the enzyme phosphoglucomutase.
- This step is essential as G1P is the active form of glucose needed for glycogen synthesis.
- Activation of Glucose
- G1P is then activated by UDP-glucose pyrophosphorylase, transferring a UTP molecule to G1P to form UDP-glucose.
- This activation step ensures that glucose is in the correct form for incorporation into the growing glycogen chain.
- Glycogen Chain Elongation
- Glycogen synthase adds UDP-glucose units to the growing glycogen chain.
- It forms α-1,4-glycosidic bonds, extending the glycogen chain by one glucose unit at a time.
- Formation of Glycogen Primer
- The enzyme glycogenin initiates the glycogen synthesis by creating short glycogen chains.
- Glycogenin adds about seven glucose molecules to a tyrosine residue on the glycogenin homodimer, forming α-1,4-glycosidic bonds.
- Branching of Glycogen
- Once the glycogen chain reaches a certain length, the branching enzyme (α-1,6-glucosyltransferase) introduces branches by transferring a segment of the chain and linking it with an α-1,6-glycosidic bond.
- This creates branches that allow for faster breakdown and synthesis of glycogen when needed.
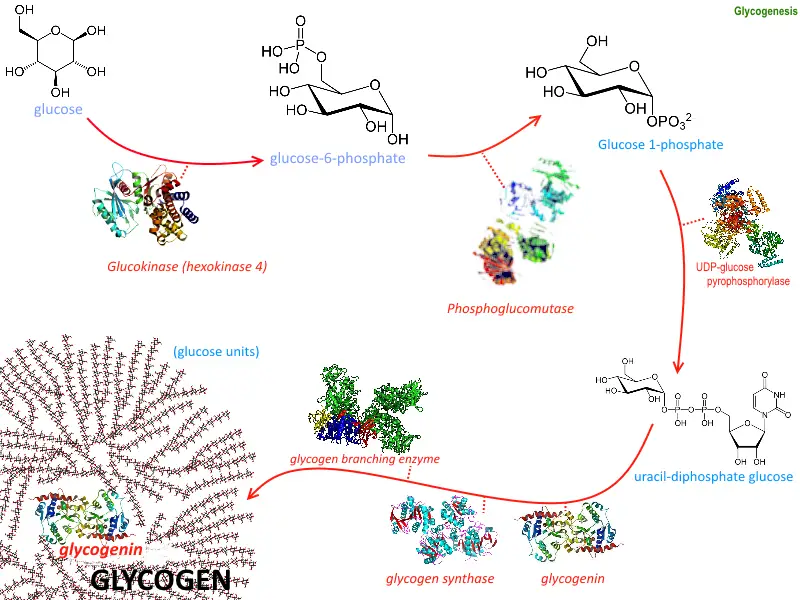
Reactions of Glycogenesis Steps
Step | Reaction | Enzyme |
---|---|---|
Glucose Uptake | – | GLUT2 (liver) / GLUT4 (muscle) |
Phosphorylation to G6P | Glucose + ATP → Glucose-6-Phosphate + ADP | Hexokinase / Glucokinase |
Isomerization to G1P | Glucose-6-Phosphate ⇌ Glucose-1-Phosphate | Phosphoglucomutase |
Activation of Glucose | Glucose-1-Phosphate + UTP ⇌ UDP-Glucose + PPi | UDP-glucose pyrophosphorylase |
Elongation of Glycogen | UDP-Glucose + Glycogen (n residues) → Glycogen (n+1 residues) + UDP | Glycogen synthase |
Formation of Glycogen Primer | UDP-Glucose → Glycogen primer (7 glucose units linked via α-1,4 bonds) | Glycogenin |
Branching of Glycogen | Glycogen (linear chain) → Branched glycogen (via α-1,6 glycosidic bonds) | Branching enzyme (α-1,6-glucosyltransferase) |
Regulation of Glycogenesis
Glycogenesis, the process of glycogen synthesis, is tightly regulated to ensure that glycogen is produced when energy needs and glucose availability are optimal. Several key factors influence this regulation, including hormones, enzyme activity, and intracellular signaling pathways.
- Insulin Regulation
- Insulin plays a primary role in activating glycogenesis.
- When blood glucose levels are elevated, insulin is secreted by the pancreas to promote glucose uptake by cells, especially in the liver and muscle tissues.
- Once inside the cell, glucose is converted to glucose-6-phosphate (G6P) and subsequently to glucose-1-phosphate (G1P).
- Insulin activates glycogen synthase, the enzyme responsible for elongating the glycogen chain, thus driving glycogen formation.
- Glucagon Regulation
- In contrast to insulin, glucagon is secreted when blood glucose levels are low, such as during fasting or between meals.
- Glucagon opposes the action of insulin by promoting glycogen breakdown (glycogenolysis) and inhibiting glycogenesis.
- It activates glycogen phosphorylase, the enzyme that breaks down glycogen, by a signaling cascade.
- Glucagon inhibits glycogen synthase through phosphorylation, reducing the production of glycogen.
- Covalent Modification: Toggle Control
- Glycogen metabolism is further regulated through reversible phosphorylation of key enzymes.
- Protein kinase A (PKA), activated by hormones like glucagon and epinephrine, phosphorylates glycogen synthase, deactivating it and inhibiting glycogenesis.
- Protein phosphatase 1 (PP1), when active, dephosphorylates glycogen synthase, activating it to promote glycogen synthesis.
- The activity of glycogen phosphorylase is also regulated by phosphorylation. When phosphorylated, it is active and promotes glycogen breakdown. When dephosphorylated, it is inactive.
- Epinephrine (Adrenaline) Regulation
- During stress or physical exertion, the adrenal glands release epinephrine (adrenaline).
- Epinephrine triggers glycogen breakdown by activating glycogen phosphorylase through a PKA-mediated phosphorylation event.
- At the same time, epinephrine inhibits glycogen synthesis by deactivating glycogen synthase through PKA.
- Calcium Ion Regulation
- Calcium ions play a key role in muscle cells during physical activity.
- Increased intracellular calcium concentrations, typically due to muscle contraction, activate calmodulin.
- Calmodulin activates CaMK (calcium/calmodulin-dependent kinase), which then activates phosphorylase kinase.
- Phosphorylase kinase phosphorylates and activates glycogen phosphorylase, thus promoting glycogen breakdown to provide glucose for energy.
Factors Affecting Glycogenesis
Glycogenesis is regulated by a combination of hormonal, nutritional, physical, and metabolic factors. These factors ensure that glycogen stores are adequately replenished or mobilized based on the body’s energy needs.
- Hormonal Regulation
- Insulin: This hormone is the main driver of glycogenesis. It promotes glucose uptake into cells and activates glycogen synthase, the enzyme responsible for converting glucose into glycogen. Insulin is particularly effective in stimulating glycogenesis after meals rich in carbohydrates.
- Glucagon: Released when blood glucose levels drop, glucagon counteracts insulin by promoting glycogenolysis—the breakdown of glycogen into glucose—rather than glycogenesis.
- Epinephrine: Released during stress or physical activity, epinephrine stimulates glycogen breakdown to supply immediate energy, reducing glycogen stores.
- Growth Factors: Insulin-like growth factors (IGF-1 and IGF-2) influence glycogen synthesis, with IGF-1 being more effective than IGF-2 in activating glycogen synthase.
- Nutritional Factors
- Carbohydrate Intake: Carbohydrates are crucial for replenishing glycogen stores. A diet rich in carbohydrates ensures an adequate supply of glucose for glycogenesis, especially following exercise, when muscles are primed for glycogen storage.
- Protein Supplementation: When carbohydrates are low, protein can help support glycogen synthesis. Consuming protein with carbohydrates after exercise can enhance muscle recovery and boost glycogen replenishment.
- Dietary Composition: High-fat diets can impair carbohydrate oxidation during intense exercise but may enhance fat oxidation during lower-intensity activities, potentially conserving muscle glycogen.
- Physical Activity
- Exercise Intensity and Duration: Intense or prolonged exercise depletes glycogen stores in muscles and the liver. The greater the intensity and duration of the exercise, the more glycogen is used up. Proper recovery, including carbohydrate intake, is essential to replenish these stores.
- Fitness Level: Well-trained athletes typically have more efficient glycogen storage. Regular exercise enhances the body’s ability to store and utilize glycogen, meaning athletes have higher muscle glycogen levels compared to sedentary individuals.
- Cellular Metabolism
- Glucose-6-Phosphate Levels: This metabolite plays a key role in glycogenesis. It activates glycogen synthase while inhibiting glycogen phosphorylase, promoting the conversion of glucose into glycogen when glucose levels are high.
- Energy State of the Cell: Cellular energy levels also influence glycogenesis. When ATP levels are high, the body prefers to store glucose as glycogen. In contrast, low energy states trigger processes like glycogenolysis to provide glucose for energy.
Diseases Related to Glycogenesis
Glycogenesis is crucial for maintaining energy reserves in the body, but when the enzymes involved in this process are deficient, it leads to a group of inherited metabolic disorders known as glycogen storage diseases (GSDs). These conditions cause improper glycogen metabolism, resulting in abnormal glycogen storage or utilization. Below are various GSDs, highlighting their enzyme deficiencies, symptoms, and affected tissues.
- Type I (Von Gierke Disease)
- Enzyme Deficiency: Glucose-6-phosphatase.
- Symptoms: Enlarged liver and kidneys, hypoglycemia (low blood sugar), high lactate and uric acid levels, growth delays, and increased susceptibility to infections.
- Affected Tissues: Primarily the liver and kidneys.
- Type II (Pompe Disease)
- Enzyme Deficiency: Acid alpha-glucosidase.
- Symptoms: Muscle weakness, enlarged heart (cardiomyopathy), respiratory issues, and in severe cases, heart failure in infants.
- Affected Tissues: Heart and skeletal muscles, with widespread effects on multiple organs.
- Type III (Cori Disease)
- Enzyme Deficiency: Debranching enzyme (AGL).
- Symptoms: Enlarged liver, hypoglycemia, muscle weakness, and growth delays.
- Affected Tissues: Liver and skeletal muscles.
- Type IV (Andersen Disease)
- Enzyme Deficiency: Branching enzyme.
- Symptoms: Liver cirrhosis, muscle weakness, and failure to thrive in infants.
- Affected Tissues: Liver, with abnormal glycogen structure.
- Type V (McArdle Disease)
- Enzyme Deficiency: Muscle phosphorylase.
- Symptoms: Exercise intolerance, muscle cramps during exertion, and myoglobinuria (presence of myoglobin in urine).
- Affected Tissues: Skeletal muscles.
- Type VI (Hers Disease)
- Enzyme Deficiency: Hepatic phosphorylase.
- Symptoms: Mild hypoglycemia, enlarged liver, and growth delays.
- Affected Tissues: Liver.
- Type VII (Tarui Disease)
- Enzyme Deficiency: Phosphofructokinase.
- Symptoms: Exercise intolerance, muscle cramps, and hemolytic anemia.
- Affected Tissues: Muscles and red blood cells.
Importance of Glycogenesis
Glycogenesis plays a crucial role in energy storage and regulation, ensuring that the body can maintain glucose homeostasis and meet energy demands under varying conditions. It allows for the efficient storage of glucose, preventing the negative consequences of excessive glucose accumulation while ensuring a steady supply during periods of low blood sugar.
- Energy Storage and Regulation
- Energy Reserve: Glycogen, stored primarily in the liver and muscles, serves as a short-term energy reserve. During physical activity or between meals, glycogen can quickly be converted back into glucose to fuel the body’s needs.
- Glucose Homeostasis: When glucose levels rise after meals, glycogenesis is triggered, converting excess glucose into glycogen for future use. In contrast, during fasting or energy-deprived states, glycogen is broken down into glucose via glycogenolysis to maintain stable blood sugar levels.
- Hormonal Regulation: The process of glycogenesis is controlled by hormones like insulin and glucagon. Insulin facilitates glycogenesis when blood glucose is high by promoting glucose uptake and its conversion into glycogen. Conversely, glucagon stimulates glycogen breakdown when glucose levels are low, ensuring the body has adequate fuel.
- Metabolic Significance
- Osmolarity Control: Glycogen synthesis helps manage osmotic pressure within cells. Storing glucose as glycogen prevents the harmful osmotic load that free glucose would impose, avoiding cellular damage.
- Rapid Energy Availability: The branched structure of glycogen enables rapid release of glucose units when immediate energy is needed. This ensures that the body can quickly mobilize glucose molecules during intense physical activity or periods of stress.
- Prevention of Hypoglycemia: Adequate glycogen storage is essential in preventing hypoglycemia. Low glycogen levels can lead to dangerously low blood sugar, resulting in fatigue, confusion, and other health complications. Conditions that affect glycogen storage can disrupt energy reserves, leading to metabolic imbalances.
- Pathophysiological Implications
- Disruptions in glycogenesis can cause glycogen storage disorders (GSDs). These disorders can result from the accumulation of excess glycogen or insufficient glycogen storage, leading to various symptoms like muscle weakness, hypoglycemia, and organ dysfunction. Studying glycogenesis is critical for developing therapies for these metabolic disorders, helping to correct or manage these disruptions.
- Adeva-Andany MM, González-Lucán M, Donapetry-García C, Fernández-Fernández C, Ameneiros-Rodríguez E. Glycogen metabolism in humans. BBA Clin. 2016 Feb 27;5:85-100. doi: 10.1016/j.bbacli.2016.02.001. PMID: 27051594; PMCID: PMC4802397.
- Daghlas SA, Mohiuddin SS. Biochemistry, Glycogen. [Updated 2023 May 1]. In: StatPearls [Internet]. Treasure Island (FL): StatPearls Publishing; 2024 Jan-. Available from: https://www.ncbi.nlm.nih.gov/books/NBK539802/
- Gümüş E, Özen H. Glycogen storage diseases: An update. World J Gastroenterol. 2023 Jul 7;29(25):3932-3963. doi: 10.3748/wjg.v29.i25.3932. PMID: 37476587; PMCID: PMC10354582.
- https://emedicine.medscape.com/article/1116574-overview
- https://www.chp.edu/our-services/transplant/liver/education/liver-disease-states/glycogen-storage-diseases
- https://my.clevelandclinic.org/health/diseases/15553-glycogen-storage-disease-gsd
- https://www.britannica.com/science/glycogenolysis
- https://www.chop.edu/conditions-diseases/glycogen-storage-disease-gsd
- https://bio.libretexts.org/Bookshelves/Biochemistry/Fundamentals_of_Biochemistry_(Jakubowski_and_Flatt)/02:_Unit_II-_Bioenergetics_and_Metabolism/15:_Glucose_Glycogen_and_Their_Metabolic_Regulation/15.02:_Glycogenesis
- https://en.wikipedia.org/wiki/Glycogen_debranching_enzyme
- https://byjus.com/chemistry/glycogenesis/
- https://en.wikipedia.org/wiki/Glycogenesis