What is Fatty acid?
Fatty acids are essential components in biochemistry, playing significant roles in various biological processes. These carboxylic acids consist of an aliphatic chain, which can be either saturated or unsaturated. In nature, most fatty acids have a straight chain with an even number of carbon atoms, ranging from 4 to 28. They are found abundantly in lipids, constituting up to 70% of the lipid’s weight in certain species, such as microalgae. However, in other organisms, fatty acids exist primarily in the form of esters, namely triglycerides, phospholipids, and cholesteryl esters. These esters serve as important sources of fuel for animals and contribute to the structural integrity of cells.
The concept of fatty acids was first introduced by Michel Eugène Chevreul in 1813. Although he initially used terms like “acid fat” and “oily acid,” Chevreul’s research laid the foundation for our understanding of these compounds. Fatty acids are composed of hydrocarbon chains that terminate with carboxylic acid groups. Together with their derivatives, they form the fundamental components of lipids. The length and degree of saturation of the hydrocarbon chain vary greatly among fatty acids and determine their physical properties, such as melting point and fluidity. Additionally, the hydrophobic properties of lipids, their insolubility in water, can be attributed to the presence of fatty acids.
Fatty acids consist of carbon, hydrogen, and oxygen atoms arranged in a variable-length linear carbon chain skeleton, typically containing an even number of atoms. While fatty acids can range from 2 to 30 carbon atoms or more, those with 12 to 22 carbon atoms are the most common and biologically significant. These fatty acids can be found in various animal and plant fats. Fatty acids are rarely found in free form in nature but instead exist as integral components of different lipid structures, such as:
- Triacylglycerols (or triglycerides): These are molecules formed by combining three fatty acid molecules with a glycerol molecule. Triglycerides serve as a major energy storage form in our bodies and are also present in many dietary fats.
- Diacylglycerols and monoacylglycerols: These lipid compounds are often added to processed foods and can contribute to their texture, taste, and stability.
- Phospholipids: These are essential components of cell membranes. Phospholipids consist of two fatty acid molecules, a glycerol molecule, a phosphate group, and a polar head group. They play a crucial role in maintaining the integrity and functionality of cell membranes.
- Sterol esters: Fatty acids can also combine with sterols, such as cholesterol, to form sterol esters. These compounds are involved in various physiological processes and are found in both food sources and our bodies.
Fatty acids serve as the building blocks of fat in our bodies and the food we consume. During digestion, fats are broken down into fatty acids, which are then absorbed into the bloodstream. The body typically combines fatty acid molecules in groups of three, forming triglycerides for storage and energy utilization. Additionally, triglycerides can be synthesized within our bodies from the carbohydrates we consume.
Fatty acids play numerous vital functions in the body. Apart from energy storage, they are involved in cell signaling, hormone production, and the insulation and protection of vital organs. When glucose, a type of sugar, is unavailable as an energy source, the body turns to fatty acids to fuel the cells. This process is particularly important during prolonged periods of fasting or intense physical activity.
In conclusion, fatty acids are crucial components of lipids and play significant roles in various biological processes. They are carboxylic acids with aliphatic chains, either saturated or unsaturated, and are present in triglycerides, phospholipids, and cholesteryl esters. Fatty acids serve as important dietary fuel sources and contribute to the structural composition of cells. Their functions range from energy storage to hormone production, making them essential for the proper functioning of the body.
Definition of Fatty acid
A fatty acid is a type of carboxylic acid with an aliphatic chain, which can be saturated or unsaturated. It is a fundamental component of lipids and plays important roles in energy storage and as structural components in cells.
Fatty Acid Structure
The structure of fatty acids plays a crucial role in determining their properties and functions. Fatty acids are organic molecules that consist of carbon, hydrogen, and oxygen atoms. They are building blocks of lipids and possess distinct features that influence their behavior.
Fatty acids are often described as long molecules due to their elongated structure. They are composed of a straight chain of carbon and hydrogen atoms, with a carboxyl acid group (―COOH) at one end and a methyl group at the other. The general formula for a fatty acid is RCOOH, where R represents the hydrocarbon chain, including the methyl group. The R-group can be either saturated or unsaturated.
The hydrocarbon chain in fatty acids can vary in length, typically ranging from 12 to 20 carbon atoms. This variation in chain length contributes to the diversity of fatty acids found in biological systems.
Due to the predominantly hydrocarbon nature of the fatty acid structure, they are considered hydrophobic or water-insoluble. This is because the hydrocarbon chain contains a large number of carbon and hydrogen atoms but relatively fewer oxygen atoms from the carboxyl group. As a result, fatty acids repel water and exhibit hydrophobic properties.
Based on the presence or absence of double bonds in the hydrocarbon chain, fatty acids can be classified as saturated or unsaturated. Saturated fatty acids have only single bonds between carbon atoms and are denoted by “S” for saturated. Unsaturated fatty acids, on the other hand, have one or more double bonds (“D” for double bonds). The presence of double bonds introduces kinks or bends in the fatty acid structure, affecting their ability to stack together. Unsaturated fatty acids have a lower melting point compared to saturated fatty acids.
In addition to the number of double bonds, the position of double bonds along the hydrocarbon chain is also important. The position is indicated by a numerical system, such as C 18:2 (9,12), which represents an 18-carbon chain with double bonds at the 9th and 12th carbon positions. Furthermore, the omega (ω) notation is used to denote the position of the farthest double bond from the carboxylic acid end. For example, C 18:2 (9,12) is classified as omega-6 fatty acid because the farthest double bond is 6 carbon atoms away from the carboxylic acid group (18 – 12 = 6).
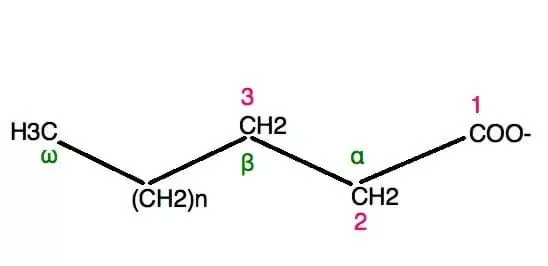
The distinct regions of fatty acid molecules include the hydrophobic hydrocarbon chain, which is non-reactive, and the hydrophilic carboxyl (-COOH) group, which is reactive and water-soluble. The carboxyl group allows fatty acids to form covalent bonds with other molecules in cellular processes.
The structure of fatty acids not only determines their physical properties, such as melting point and solubility, but also influences their stability and reactivity. Saturated fatty acids are highly stable, while unsaturated fatty acids are more prone to oxidation. Understanding the structure-function relationships of fatty acids is essential for comprehending their roles in biological systems and their implications for health and disease.
Properties of Fatty Acids
Fatty acids possess several properties that contribute to their behavior and reactivity in various chemical processes. Understanding these properties is crucial for studying their role in biological systems and their applications in different fields.
- Reactivity: Fatty acids exhibit similar chemical reactions to other carboxylic acids. They can undergo esterification reactions, where the carboxyl group (-COOH) reacts with an alcohol to form an ester. Additionally, fatty acids participate in acid-base reactions, involving the exchange of protons (H+) between the carboxyl group and a base.
- Acidity: The acidity of fatty acids is relatively constant, with minor variations as indicated by their pKa values. The pKa values reflect the tendency of fatty acids to donate a proton (H+) from the carboxyl group. The close proximity of the pKa values suggests that fatty acids have similar acid strengths. For example, nonanoic acid has a pKa of 4.96, making it slightly weaker than acetic acid (pKa 4.76).
- Solubility: The solubility of fatty acids in water decreases as the length of the hydrocarbon chain increases. Longer-chain fatty acids have limited solubility in water, resulting in minimal effects on the pH of an aqueous solution. This hydrophobic nature arises from the large number of carbon and hydrogen atoms in the hydrocarbon chain, which repel water molecules.
- Conjugate Bases: At near-neutral pH, fatty acids predominantly exist as their conjugate bases. For example, oleic acid can exist as oleate in a solution at neutral pH. The conjugate base is formed when the carboxyl group donates a proton (H+) and becomes negatively charged (-COO-). The presence of conjugate bases influences the chemical behavior and interactions of fatty acids in biological systems.
- Auto-oxidation: Unsaturated fatty acids are prone to auto-oxidation, a chemical change that occurs in the presence of oxygen (air). Auto-oxidation can lead to the formation of free radicals and the breakdown of unsaturated fatty acids. Trace metals, such as iron or copper, accelerate this process, making it more rapid and detrimental. Antioxidants are often used to inhibit or delay auto-oxidation in food and cosmetic products.
- Ozonolysis: Ozonolysis is a chemical reaction that involves the degradation of unsaturated fatty acids using ozone (O3). This reaction is commonly employed in the synthesis of compounds such as azelaic acid from oleic acid. Ozonolysis helps break the carbon-carbon double bonds in unsaturated fatty acids, allowing for the production of specific derivatives.
By considering these properties, researchers and scientists can better understand the behavior and reactivity of fatty acids, enabling them to explore their diverse applications in areas such as biochemistry, nutrition, pharmaceuticals, and chemical synthesis.
Chemistry of Fatty acids
The chemistry of fatty acids encompasses various aspects related to their structure, isomerism, and physical properties. Understanding the chemistry of fatty acids is crucial for studying their behavior, reactivity, and impact on biological systems. Here are some key points regarding the chemistry of fatty acids:
- Structure of Saturated Fatty Acids: The carbon chains of saturated fatty acids exhibit a zigzag pattern when extended at low temperatures. However, at higher temperatures, certain bonds within the chain undergo rotation, leading to chain shortening.
- Geometric Isomerism in Unsaturated Fatty Acids: Unsaturated fatty acids contain carbon-carbon double bonds, which introduce a type of geometric isomerism. The orientation of atoms or groups around these double bonds determines the isomeric form. In the cis isomer, the acyl chains are on the same side of the double bond, as observed in oleic acid. In contrast, the trans isomer, such as elaidic acid, has the acyl chains on opposite sides of the double bond.
- Configuration of Naturally Occurring Unsaturated Fatty Acids: Most naturally occurring unsaturated long-chain fatty acids have a cis configuration. This means that the acyl chains on each side of the double bond are on the same side, resulting in a bent or kinked structure. For example, oleic acid forms an L shape due to its cis configuration. In contrast, the trans configuration, as seen in elaidic acid, results in a straighter structure.
- Influence of Double Bond Position and Number: The number and position of double bonds in a fatty acid can significantly impact its spatial arrangement and physical properties. Increasing the number of cis double bonds introduces more bends or kinks in the fatty acid chain. For instance, arachidonic acid, with four cis double bonds, exhibits a U shape due to the multiple kinks. Trans double bonds, however, alter the spatial relationships and can disrupt the natural bent conformation.
- Melting Points of Fatty Acids: The melting points of fatty acids are influenced by both chain length and unsaturation. Generally, as the chain length increases, the melting point of even-numbered-carbon fatty acids also increases. On the other hand, the presence of double bonds, which introduce unsaturation, decreases the melting point. This is due to the disruption of intermolecular forces caused by the kinks and reduced packing efficiency in unsaturated fatty acids.
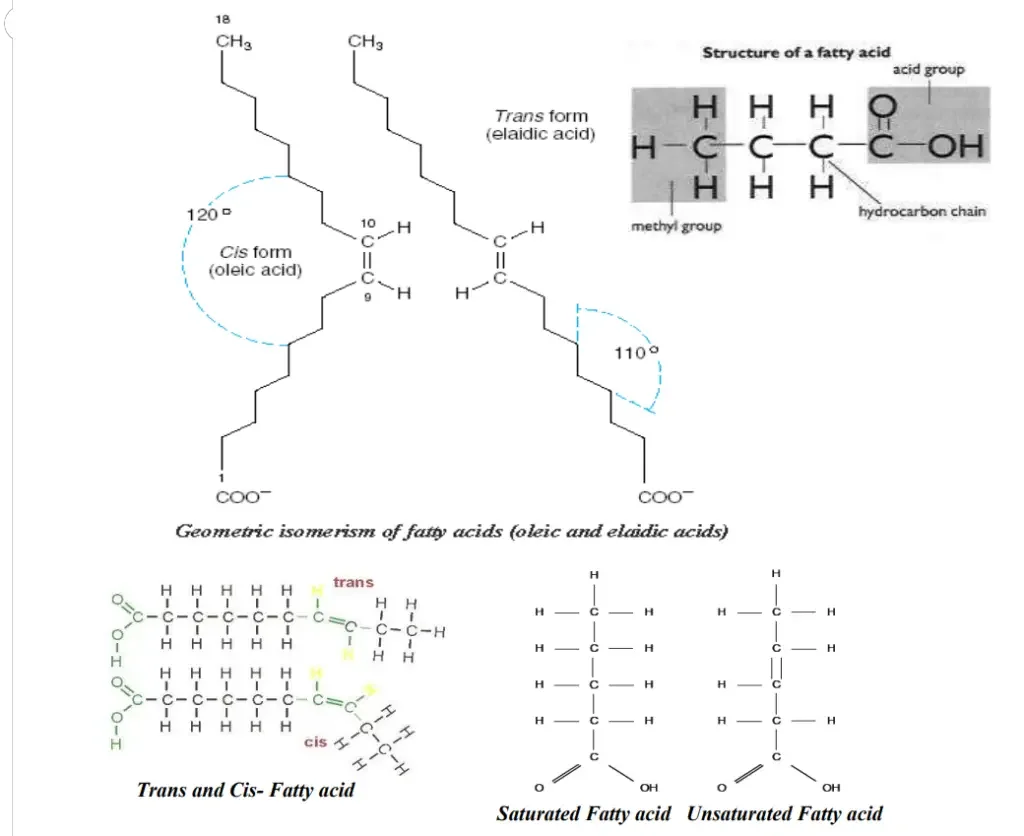
Understanding the chemistry of fatty acids allows scientists to predict their physical properties, reactivity, and behavior in various environments. It is also crucial for exploring the role of fatty acids in biological processes, as well as their applications in fields such as nutrition, biochemistry, and lipid chemistry.
Types of Fatty Acids – Classification of Fatty Acids
Fatty acids are organic compounds that play a crucial role in various physiological processes in the body. They are essential components of lipids, which are the main structural constituents of cell membranes and a major energy source. Fatty acids are classified into different types based on various characteristics, including their degree of saturation/unsaturation, presence or absence of double/triple bonds, ability to be synthesized by animals, and functional properties. Here are the main types of fatty acids:
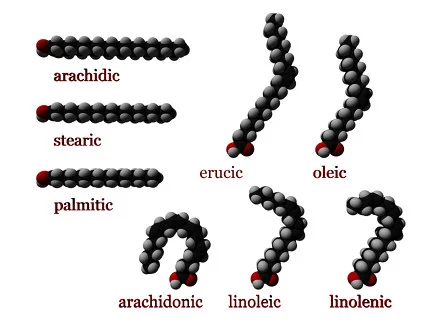
Types of Fatty Acids Based on Degree of Saturation/Unsaturation
Fatty acids are classified into three types based on their degree of saturation/unsaturation in the carbon chain:
- Saturated Fatty Acids: These fatty acids have no double bonds in the carbon chain. They are fully saturated with hydrogen atoms.
- Monounsaturated Fatty Acids: Fatty acids with one double bond in the carbon chain are classified as monounsaturated.
- Polyunsaturated Fatty Acids: Fatty acids with two or more double bonds in the carbon chain are considered polyunsaturated.
Types of Fatty Acids Based on Presence/Absence of Double/Triple Bonds
Another classification of fatty acids is based on the presence or absence of double/triple bonds in the carbon chain:
- Saturated Fatty Acids: These fatty acids lack double bonds in the carbon chain, resulting in a fully saturated structure.
- Unsaturated Fatty Acids: Fatty acids with one or more double bonds in the carbon chain are classified as unsaturated.
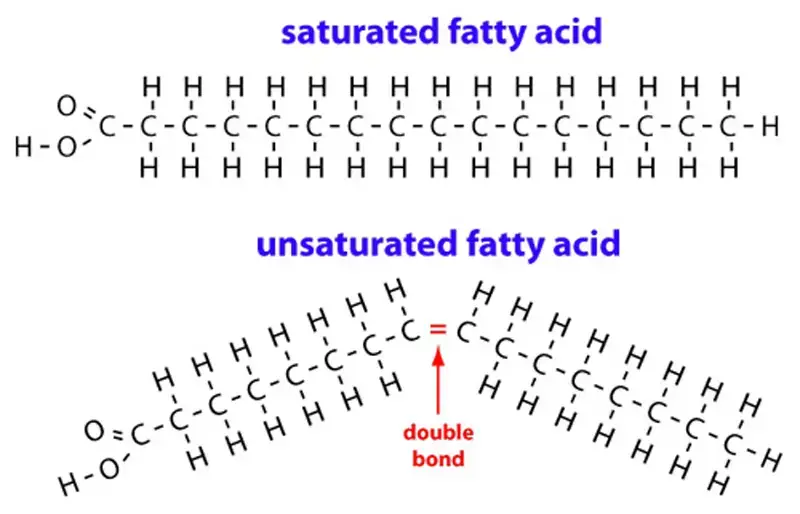
Types of Fatty Acids Based on the Ability to be Synthesized by Animals
Fatty acids can be classified based on their ability to be synthesized by animals and whether their deficiency can be reversed by dietary addition:
- Essential Fatty Acids: These are fatty acids that cannot be synthesized by the body and must be obtained through the diet. They are essential for various physiological functions.
- Non-Essential Fatty Acids: These fatty acids can be synthesized by the body and are not considered essential in the diet.
Types of Fatty Acids Based on Chain Length
Fatty acids can also be classified based on their chain length:
- Short-chain Fatty Acids: These fatty acids contain up to 6 carbon atoms in their chain.
- Medium-chain Fatty Acids: Fatty acids with 8 to 12 carbon atoms are categorized as medium-chain fatty acids.
- Long-chain Fatty Acids: Fatty acids with 14 to 18 carbon atoms in their chain fall into the category of long-chain fatty acids.
- Very Long-chain Fatty Acids: Fatty acids with 20 or more carbon atoms in their chain are referred to as very long-chain fatty acids.
Other Fatty Acids
Apart from the aforementioned classifications, there are additional types of fatty acids based on their functional properties:
- Oxygenated Fatty Acids: These fatty acids contain additional functional groups such as hydroxyl, keto, and epoxy groups. Ricinoleic acid, found in castor oil, is an example of an oxygenated fatty acid.
- Cyclic Fatty Acids: These fatty acids possess a cyclic unit with three, five, or even six carbon atoms in their structure, resembling compounds like prostaglandins.
Understanding the different types of fatty acids is important as they have distinct roles in various biological processes and can impact overall health. The classification of fatty acids helps in studying their functions, dietary requirements, and implications for human physiology.
What is Even- vs odd-chained fatty acids?
- Fatty acids, the building blocks of lipids, can be classified into different categories based on their chain length and the number of carbon atoms they contain. One such classification is based on whether the fatty acid has an even or odd number of carbon atoms in its chain. Let’s explore the difference between even-chained and odd-chained fatty acids and how they are biosynthesized and metabolized.
- Even-chained fatty acids are the most common type of fatty acids found in nature. They are composed of an even number of carbon atoms in their chain. Examples of even-chained fatty acids include stearic acid (C18:0) and oleic acid (C18:1), both of which consist of 18 carbon atoms. These fatty acids are widely distributed in various food sources and play important roles in biological processes.
- On the other hand, odd-chained fatty acids (OCFA) have an odd number of carbon atoms in their chain. The most prevalent odd-chained fatty acids are pentadecanoic acid (C15:0) and heptadecanoic acid (C17:0). These fatty acids, with 15 and 17 carbon atoms respectively, are commonly found in dairy products. While odd-chained fatty acids are not as abundant as their even-chained counterparts, they still have biological significance.
- From a molecular perspective, odd-chained fatty acids are biosynthesized and metabolized slightly differently from even-chained fatty acids. The pathways involved in their synthesis and breakdown exhibit some variations. For instance, odd-chained fatty acids are synthesized by specific enzymes that incorporate propionyl-CoA, a three-carbon molecule, during fatty acid synthesis. This results in the formation of fatty acids with an odd number of carbon atoms.
- Metabolically, odd-chained fatty acids undergo distinct processes compared to even-chained fatty acids. Upon ingestion, odd-chained fatty acids are metabolized through a pathway called beta-oxidation, which breaks down fatty acids into acetyl-CoA units. During beta-oxidation of odd-chained fatty acids, the final product is propionyl-CoA, which can enter the citric acid cycle and be further metabolized.
- Odd-chained fatty acids have drawn scientific interest due to their potential health implications. Some studies suggest that pentadecanoic acid (C15:0) and heptadecanoic acid (C17:0) may have beneficial effects on metabolic health, including insulin sensitivity and cholesterol metabolism. However, more research is needed to fully understand the specific mechanisms and health benefits associated with odd-chained fatty acids.
In summary, fatty acids can be categorized as even-chained or odd-chained based on the number of carbon atoms in their chain. Even-chained fatty acids, with an even number of carbon atoms, are more common, while odd-chained fatty acids contain an odd number of carbon atoms. These two types of fatty acids have some differences in their biosynthesis and metabolism. Odd-chained fatty acids, such as pentadecanoic acid and heptadecanoic acid, are found in dairy products and have unique metabolic pathways. Further research is needed to fully elucidate the physiological and health effects of odd-chained fatty acids.
Unsaturated fatty acids
- Unsaturated fatty acids are a class of fatty acids that contain one or more carbon-carbon double bonds (C=C) in their hydrocarbon chain. The presence of these double bonds gives rise to distinct structural and chemical properties, contributing to their functional roles in biological systems.
- The configuration of the C=C double bonds in unsaturated fatty acids can be either cis or trans isomers. In the cis configuration, the two hydrogen atoms adjacent to the double bond are on the same side of the carbon chain, causing the chain to bend. This cis configuration limits the conformational freedom of the fatty acid and reduces its flexibility. The degree of curvature increases with the number of cis double bonds in the chain. For example, oleic acid, with a single cis double bond, exhibits a slight kink, while linoleic acid, with two cis double bonds, has a more pronounced bend. α-Linolenic acid, with three cis double bonds, adopts a hooked shape. This structural rigidity due to cis double bonds affects the physical properties of unsaturated fatty acids, such as their ability to pack closely together in lipid bilayers or triglycerides. Cis unsaturated fatty acids increase membrane fluidity, which is important for cellular processes, while trans unsaturated fatty acids have properties more similar to straight saturated fatty acids.
- In contrast to cis unsaturated fatty acids, trans unsaturated fatty acids have the hydrogen atoms adjacent to the double bond on opposite sides of the chain. This trans configuration allows the chain to remain relatively straight, resembling saturated fatty acids in shape. Trans fatty acids are not commonly found in nature, except in small amounts in certain dairy products and meat from ruminant animals. The majority of trans fatty acids are artificially produced through a process called hydrogenation, which converts unsaturated fats into more saturated forms.
- Naturally occurring unsaturated fatty acids usually have three, six, or nine carbon atoms following each double bond. These are denoted as n-3, n-6, or n-9 fatty acids, respectively. The nomenclature indicates the number of carbon atoms counted from the double bond to the end of the chain. The majority of naturally occurring unsaturated fatty acids have cis configurations, while trans configurations are predominantly a result of industrial processing.
- Unsaturated fatty acids, including those with cis and trans double bonds, play crucial roles in biological processes and the construction of biological structures, such as cell membranes. They contribute to membrane fluidity, signal transduction, and the synthesis of bioactive molecules. Additionally, specific unsaturated fatty acids, such as omega-3 (n-3) and omega-6 (n-6) fatty acids, are considered essential because they cannot be synthesized by the body and must be obtained from the diet.
- Examples of unsaturated fatty acids include oleic acid (cis-Δ9), linoleic acid (cis,cis-Δ9,Δ12), and alpha-linolenic acid (cis,cis,cis-Δ9,Δ12,Δ15), among others. These fatty acids have diverse physiological functions and can be obtained from various dietary sources.
- Understanding the characteristics and properties of unsaturated fatty acids is crucial for comprehending their impact on human health, metabolism, and the structure-function relationships within biological systems.
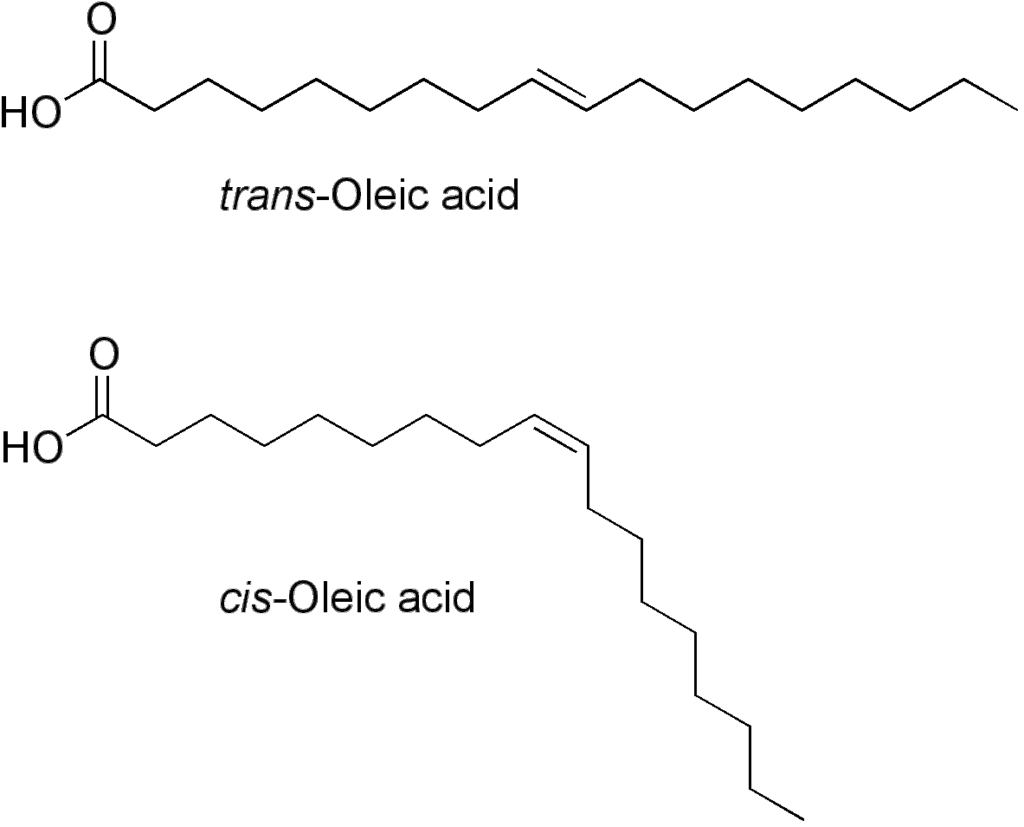
Saturated fatty acids
Saturated fatty acids are a type of fatty acid that do not contain any carbon-carbon double bonds (C=C) in their hydrocarbon chain. Instead, they are fully saturated with hydrogen atoms, which is why they are called “saturated.” Saturated fatty acids have the general formula CH3(CH2)nCOOH, where n represents the number of carbon atoms in the chain.
One of the most well-known saturated fatty acids is stearic acid, which has 18 carbon atoms (n = 16) in its chain. When stearic acid is neutralized with sodium hydroxide, it forms the most common form of soap.
Saturated fatty acids can vary in chain length, and different saturated fatty acids have different names and chemical structures. Here are some examples of saturated fatty acids and their corresponding chain lengths:
- Caprylic acid: CH3(CH2)6COOH (8 carbon atoms) – 8:0
- Capric acid: CH3(CH2)8COOH (10 carbon atoms) – 10:0
- Lauric acid: CH3(CH2)10COOH (12 carbon atoms) – 12:0
- Myristic acid: CH3(CH2)12COOH (14 carbon atoms) – 14:0
- Palmitic acid: CH3(CH2)14COOH (16 carbon atoms) – 16:0
- Stearic acid: CH3(CH2)16COOH (18 carbon atoms) – 18:0
- Arachidic acid: CH3(CH2)18COOH (20 carbon atoms) – 20:0
- Behenic acid: CH3(CH2)20COOH (22 carbon atoms) – 22:0
- Lignoceric acid: CH3(CH2)22COOH (24 carbon atoms) – 24:0
- Cerotic acid: CH3(CH2)24COOH (26 carbon atoms) – 26:0
These saturated fatty acids can be found in various natural sources, including animal fats (such as butter and lard) and plant oils (such as coconut oil and palm oil). They are also components of many foods we consume on a daily basis.
Saturated fatty acids have distinct properties and characteristics compared to unsaturated fatty acids. Due to the absence of double bonds, saturated fatty acids have a straight and rigid structure, allowing them to pack closely together. This dense packing contributes to the solid or semi-solid state of fats at room temperature. Saturated fats are typically solid at room temperature, while unsaturated fats, with their double bonds, tend to be liquid.
Consumption of saturated fatty acids in moderation is generally considered acceptable as part of a balanced diet. However, excessive intake of saturated fats has been associated with an increased risk of cardiovascular diseases. Therefore, it is recommended to consume saturated fats in moderation and focus on a diet that includes a variety of healthy fats, including unsaturated fats.
Understanding the different types of fatty acids, such as saturated fatty acids, helps in making informed dietary choices and maintaining overall health and well-being.
Production of Fatty Acids
The production of fatty acids occurs through various processes, including industrial methods and biological synthesis in animals. Understanding how fatty acids are produced is crucial for understanding their roles and functions in both industrial applications and biological processes.
- In industrial settings, fatty acids are commonly produced through the hydrolysis of triglycerides, which are the main constituents of natural fats and oils. During hydrolysis, triglycerides are broken down into their component fatty acids, with glycerol being separated. This process, often referred to as oleochemical production, is a widely used method for obtaining fatty acids on a large scale. Additionally, phospholipids can serve as another source of fatty acids for industrial production. Some fatty acids are also synthesized synthetically by the hydrocarboxylation of alkenes.
- In animals, fatty acids are primarily synthesized from carbohydrates. The process of fatty acid synthesis occurs predominantly in the liver, adipose tissue (fat cells), and mammary glands during lactation. The conversion of carbohydrates into fatty acids involves several steps. Initially, carbohydrates are converted into pyruvate through glycolysis. Pyruvate is then decarboxylated to form acetyl-CoA, which takes place in the mitochondria. However, acetyl-CoA needs to be transported from the mitochondria to the cytosol, where fatty acid synthesis occurs. This transportation is facilitated by converting acetyl-CoA into citrate, which can cross the inner mitochondrial membrane and be cleaved back into acetyl-CoA and oxaloacetate in the cytosol. The cytosolic acetyl-CoA is then carboxylated by an enzyme called acetyl CoA carboxylase, resulting in the formation of malonyl-CoA. This step is considered the first committed step in fatty acid synthesis.
The process of fatty acid synthesis involves a series of reactions that lengthen the growing fatty acid chain by two carbon units at a time. As a result, most natural fatty acids have an even number of carbon atoms. Once the synthesis is complete, the free fatty acids are often combined with glycerol to form triglycerides, which serve as the primary storage form of fatty acids and a source of energy in animals. Fatty acids also play a crucial role in the formation of phospholipids, which are essential components of cell membranes and organelle membranes within cells.
In animals, the breakdown of stored triglycerides leads to the release of “free fatty acids” into the bloodstream through a process called lipolysis. These free fatty acids, being insoluble in water, are transported in the blood bound to plasma albumin. Cells with mitochondria can take up free fatty acids from the bloodstream, except for cells in the central nervous system due to the blood-brain barrier’s limited permeability to most free fatty acids. Fatty acids can only be broken down within mitochondria through a process called beta-oxidation, followed by further oxidation in the citric acid cycle. This process generates energy in the form of ATP.
The production of fatty acids can vary between different animal species. Studies have shown that mammalian cell membranes contain a higher proportion of polyunsaturated fatty acids compared to reptiles. Bird fatty acid composition is similar to mammals but with lower levels of omega-3 fatty acids compared to omega-6. The composition of fatty acids in cell membranes affects their fluidity and permeability, leading to variations in metabolic rates and thermoregulation in different species. Environmental factors, such as temperature, can also influence the fatty acid composition of cell membranes in organisms.
Overall, the production of fatty acids involves intricate biochemical processes, both in industrial settings and within living organisms. Understanding these processes is essential for various applications, including the production of oleochemicals, the study of metabolic pathways, and the maintenance of cellular structure and function.
Nomenclature Process of Amino Acids
The nomenclature process of amino acids involves naming and categorizing these organic compounds based on their structural characteristics. Amino acids are the building blocks of proteins and play essential roles in various biological processes.
The standard nomenclature system for amino acids involves using a three-letter abbreviation and a one-letter code. The three-letter abbreviation represents the amino acid’s name, while the one-letter code is a single letter that represents the amino acid. For example, the three-letter abbreviation for alanine is “Ala,” and its one-letter code is “A.” This system allows for a concise representation of amino acids in scientific literature and databases.
In addition to the abbreviations, amino acids are classified based on various properties such as polarity, charge, and structure. Here are some common classifications:
- Nonpolar (hydrophobic) amino acids: These amino acids have side chains that are primarily composed of hydrocarbon groups. Examples include alanine (Ala/A), valine (Val/V), and leucine (Leu/L). Nonpolar amino acids tend to be insoluble in water and are often found in the interior of proteins.
- Polar (hydrophilic) amino acids: Polar amino acids have side chains that contain functional groups capable of forming hydrogen bonds with water molecules. This group includes amino acids such as serine (Ser/S), threonine (Thr/T), and asparagine (Asn/N). Polar amino acids can interact with water and are often found on the surfaces of proteins.
- Positively charged (basic) amino acids: These amino acids have side chains that carry a positive charge at physiological pH. Examples include lysine (Lys/K), arginine (Arg/R), and histidine (His/H). Positively charged amino acids can participate in electrostatic interactions and are often involved in protein binding and enzymatic activities.
- Negatively charged (acidic) amino acids: Negatively charged amino acids have side chains that carry a negative charge at physiological pH. Aspartic acid (Asp/D) and glutamic acid (Glu/E) are examples of negatively charged amino acids. They also participate in electrostatic interactions and are involved in protein-protein interactions and enzymatic reactions.
The nomenclature of amino acids also considers the position of functional groups within their structures. For example, the amino group (-NH2) and carboxyl group (-COOH) are present in all amino acids. The central carbon, known as the alpha carbon, is the site where the amino group, carboxyl group, hydrogen atom, and side chain (R-group) are attached.
The R-group varies for each amino acid, giving them their unique properties. For instance, glycine (Gly/G) has a hydrogen atom as its R-group, while phenylalanine (Phe/F) has a phenyl ring as its R-group. The specific arrangement of atoms in the R-group contributes to the amino acid’s chemical and physical properties.
In summary, the nomenclature of amino acids involves using three-letter abbreviations and one-letter codes to represent their names. Amino acids are further categorized based on their properties, such as polarity and charge. Understanding the nomenclature and classification of amino acids is fundamental in studying protein structure, function, and the various biological processes in which they participate.
Carbon atom numbering
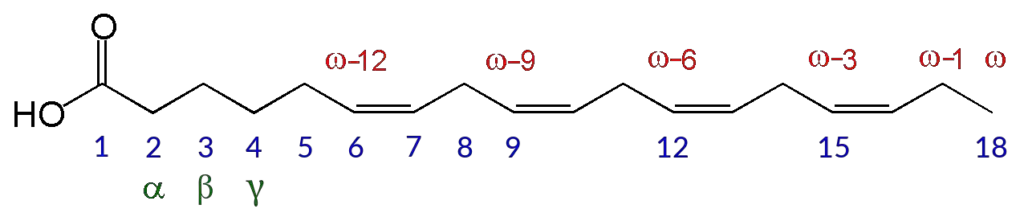
- Carbon atom numbering is an important aspect of understanding the structure and properties of fatty acids. Fatty acids are organic compounds that consist of a chain of carbon atoms, with a carboxyl group (–COOH) at one end and a methyl group (–CH3) at the other end.
- The numbering of carbon atoms in a fatty acid chain is typically done in two main conventions. The first convention, recommended by the International Union of Pure and Applied Chemistry (IUPAC), involves counting the carbon atoms from 1 at the carboxyl (-COOH) end of the molecule. Each carbon atom is often abbreviated as C-x (or Cx), where x represents the position of the carbon atom along the chain. For example, C-1 refers to the carbon atom closest to the carboxyl end, C-2 refers to the second carbon atom, and so on.
- The second convention uses Greek letters to label the carbon atoms sequentially, starting with the first carbon after the carboxyl group. In this convention, the carbon atom immediately following the carboxyl group is labeled as carbon α (alpha), the next one as carbon β (beta), and so forth. This labeling system continues until the last carbon atom in the chain, which is always designated as carbon ω (omega), the last letter of the Greek alphabet.
- Alternatively, a third numbering convention involves counting the carbon atoms from the ω end of the chain. In this convention, the labels “ω,” “ω−1,” “ω−2,” and so on are used to indicate the position of the carbon atoms. Another representation for this convention is “n−x,” where “n” represents the total number of carbon atoms in the chain. Both the “ω−x” and “n−x” notations are used to indicate the position of carbon atoms when discussing fatty acids.
- When it comes to fatty acids with double bonds, the position of the double bond is specified by giving the label of the carbon atom closest to the carboxyl end. For example, if a fatty acid has 18 carbon atoms and a double bond between carbon atoms 12 and 13, it is said to have a double bond “at” position C-12 or ω−6. The IUPAC naming of fatty acids, such as “octadec-12-enoic acid” or “12-octadecanoic acid,” is always based on the carbon atom numbering.
- Traditionally, the notation Δx,y,… is used to indicate the presence of double bonds at specific positions in a fatty acid chain. The capital Greek letter “Δ” (delta) corresponds to the Roman letter “D,” representing “double bond.” For example, arachidonic acid, which has 20 carbon atoms and double bonds between carbons 5 and 6, 8 and 9, 11 and 12, and 14 and 15, is represented as Δ5,8,11,14.
- In the context of human diet and fat metabolism, unsaturated fatty acids are often classified based on the position of the double bond closest to the ω carbon. For instance, linoleic acid, γ-linolenic acid, and arachidonic acid are all classified as “ω−6” fatty acids because their double bond closest to the ω carbon is located at position 6. This classification is used to describe their formula, where the chain ends with –CH=CH–CH2–CH2–CH2–CH3.
- Finally, fatty acids with an odd number of carbon atoms are referred to as odd-chain fatty acids, while those with an even number of carbon atoms are called even-chain fatty acids. This distinction is relevant to processes like gluconeogenesis, which involves the synthesis of glucose from non-carbohydrate sources.
- Understanding carbon atom numbering in fatty acids is crucial for identifying and discussing their structures, properties, and biological functions.
Naming of fatty acids
The naming of fatty acids encompasses various systems to describe and classify these organic compounds. The most common systems of naming fatty acids are as follows:
- Trivial Names: Trivial names, also known as common names, are historical names that are frequently used in literature. These names are often concise and easily recognizable but do not follow a systematic pattern. For example, palmitoleic acid is a trivial name.
- Systematic Names: Systematic names, also known as IUPAC names, follow the standard rules of the International Union of Pure and Applied Chemistry (IUPAC) for organic chemistry nomenclature. The systematic names for fatty acids are derived from the IUPAC Rules for the Nomenclature of Organic Chemistry. In this naming system, carbon atom numbering begins from the carboxylic end of the fatty acid molecule. Double bonds are labeled using cis-/trans- notation or E-/Z- notation when appropriate. Systematic names are more technically clear and descriptive but tend to be more verbose. For example, cis-9-octadec-9-enoic acid or (9Z)-octadec-9-enoic acid is a systematic name.
- Δx Nomenclature: In Δx (delta-x) nomenclature, each double bond in the fatty acid chain is indicated by Δx, where the double bond starts at the xth carbon–carbon bond, counting from the carboxylic end of the molecule backbone. The notation is accompanied by cis- or trans- prefixes to indicate the configuration of the molecule around the bond. This naming system provides a concise representation of fatty acids but is not more technically clear or descriptive. For instance, linoleic acid is designated as cis-Δ9, cis-Δ12 octadecadienoic acid using this nomenclature.
- n−x (or ω−x) Nomenclature: The n−x (or ω−x) nomenclature is used to name individual fatty acids and classify them based on their likely biosynthetic properties in animals. In this system, a double bond is located on the xth carbon–carbon bond, counting from the methyl end of the molecule backbone. For example, α-linolenic acid is classified as an n−3 or omega-3 fatty acid, indicating its likely biosynthetic pathway and relationship to other compounds of this type. While the “omega” notation is commonly used in popular nutritional literature, IUPAC recommends the n−x notation in technical documents. This system helps in understanding the biosynthesis of fatty acids.
- Lipid Numbers: Lipid numbers are represented as C:D, where C indicates the number of carbon atoms in the fatty acid chain, and D represents the number of double bonds. If there are multiple double bonds, they are assumed to be interrupted by CH2 units, occurring at intervals of 3 carbon atoms along the chain. For example, α-linolenic acid is an 18:3 fatty acid, indicating it has 18 carbon atoms and three double bonds located at positions Δ9, Δ12, and Δ15. However, lipid numbers can be ambiguous, so they are often paired with Δx or n−x terms for clarity. To address this ambiguity, IUPAC recommends including a list of double bond positions in parentheses, appended to the C:D notation. For instance, α-linolenic acid is denoted as 18:3(9,12,15) using this notation.
These different systems of naming fatty acids provide various levels of information about their structure, properties, and biosynthetic relationships. Scientists and researchers use these nomenclatures depending on the context and purpose of their studies.
Fatty acids Reactions – Fischer esterification
Fischer esterification is a chemical reaction that involves the esterification of a carboxylic acid by heating it with an alcohol in the presence of a strong acid catalyst. This reaction is classified as a nucleophilic acyl substitution reaction. Let’s explore the steps involved in the Fischer esterification process:
- Step 1: Acid/Base Reaction – The first step involves the protonation of the carbonyl group of the carboxylic acid. This protonation increases the electrophilicity of the carbonyl carbon, making it more susceptible to nucleophilic attack.
- Step 2: Nucleophilic Attack – In this step, the oxygen atom of the alcohol acts as a nucleophile and attacks the electrophilic carbon in the carbonyl group. This leads to the formation of a tetrahedral intermediate, with the electrons shifting towards the oxonium ion.
- Step 3: Acid/Base Reaction – The next step is an acid/base reaction where the alcoholic oxygen is deprotonated, resulting in the formation of an alkoxide ion.
- Step 4: Acid/Base Reaction – To facilitate the departure of a leaving group, an -OH group is converted into a good leaving group by protonation. This step involves an acid/base reaction.
- Step 5: Leaving Group Departure – The electrons from an adjacent oxygen atom help to “push out” the leaving group, which is a neutral water molecule. This results in the formation of the desired ester product.
- Step 6: Acid/Base Reaction – The final step involves deprotonation of the oxonium ion, leading to the formation of the ester product and revealing the carbonyl group in the ester.
Fischer esterification is an important reaction in organic chemistry and is widely used for the synthesis of esters. The presence of a strong acid catalyst promotes the reaction by facilitating the protonation and deprotonation steps. This process is commonly employed in the production of various esters for applications in industries such as fragrance, flavoring, and pharmaceuticals.
Hydrolysis of fatty acid
The reaction can be catalyzed by acid, base, or lipase, but it also occurs as an uncatalyzed reaction between fats and water dissolved in the fat phase at suitable temperatures and pressures.
Nonenzymatic ester hydrolysis and the soap-making process
Hydrolysis of fatty acids refers to the chemical reaction in which esters, such as triglycerides, undergo cleavage in the presence of water. This process results in the formation of the corresponding carboxylic acids and alcohols. Let’s explore the mechanisms involved in the base-catalyzed and acid-catalyzed hydrolysis of esters, including fatty acids:
Base-Catalyzed Hydrolysis:
Step 1: Nucleophilic Attack – In this step, hydroxide ions (OH-) act as nucleophiles and attack the electrophilic carbon of the ester carbonyl group (C=O). This leads to the formation of a tetrahedral intermediate.
Step 2: Intermediate Collapse – The tetrahedral intermediate collapses, reforming the carbonyl group (C=O) and resulting in the loss of the leaving group, which is an alkoxide ion (RO-). This leads to the formation of the corresponding carboxylic acid.
Step 3: Acid/Base Reaction – The alkoxide ion (RO-) functions as a base and deprotonates the carboxylic acid (RCO2H), resulting in a rapid equilibrium. An acidic work-up can be employed to obtain the carboxylic acid as the final product of the reaction.
Acid-Catalyzed Hydrolysis:
Step 1: Acid/Base Reaction – In acid-catalyzed hydrolysis, the weak nucleophile and poor electrophile of the ester need to be activated. Protonation of the ester carbonyl group makes it more electrophilic.
Step 2: Nucleophilic Attack – Water molecules (H2O) act as nucleophiles and attack the electrophilic carbon of the ester carbonyl group (C=O). This results in the formation of a tetrahedral intermediate.
Step 3: Acid/Base Reaction – The oxygen atom originating from the water molecule is deprotonated, neutralizing the charge in the system.
Step 4: Acid/Base Reaction – The leaving group, typically a methoxy group (OCH3), needs to be converted into a good leaving group through protonation.
Step 5: Leaving Group Departure – The electrons from an adjacent oxygen atom help in pushing out the leaving group, which is a neutral methanol molecule.
Step 6: Acid/Base Reaction – Deprotonation of the oxonium ion reveals the carbonyl group (C=O) in the carboxylic acid product and regenerates the acid catalyst.
Hydrolysis of fatty acids is an important process in the digestion and metabolism of lipids. It plays a crucial role in breaking down complex ester bonds present in dietary fats, allowing the body to absorb and utilize the resulting carboxylic acids and alcohols for energy and other biological processes.
Function of Fatty Acids
Fatty acids serve various essential functions in the body, contributing to signal transduction pathways, cellular fuel sources, the composition of hormones and lipids, protein modification, and energy storage within adipose tissue.
- Biological Signalling: Fatty acids play a role in numerous biological signaling pathways. They can act as precursors for signaling mediators, such as eicosanoids, which are involved in immune responses. Fatty acids also influence the peroxidation of LDL (low-density lipoprotein) and can impact metabolic and neurological pathways.
- Metabolism as Fuel Source: Fatty acids are metabolized as a source of cellular energy. They are taken up by cells through fatty acid-binding proteins and undergo activation via acyl-CoA. Fatty acids can be used in the mitochondria or peroxisomes to produce ATP and heat, facilitate gene expression, or be esterified in the endoplasmic reticulum for energy storage as different lipid classes.
- Energy Storage: Fatty acids are stored as triacylglycerols within specialized fat cells called adipocytes. This form of storage provides thermal and electrical insulation and protection against mechanical compression. Fatty acids, as stored triacylglycerols, are a preferred energy source over glucose due to their higher energy yield.
- Cell Membrane Formation: Fatty acids are vital for the formation of cell membranes. They contribute to the phospholipid bilayer structure, with hydrophobic fatty acid tails and hydrophilic head groups. The composition of fatty acids in cell membranes influences membrane fluidity, which affects membrane function and characteristics. Incorporation of specific fatty acids, such as omega-3 fatty acids, can impact the function of retinal cells and red blood cells.
- Protein Modification: Fatty acids interact with proteins, contributing to their acylation, folding, and anchoring. Polyunsaturated fatty acids play a critical role in protein acylation, which affects protein function. Fatty acids can also bind to nuclear receptor proteins and act as transcription factors, regulating the expression of genes related to metabolism, cellular proliferation, and apoptosis.
Overall, fatty acids have diverse and essential functions in the body, ranging from cellular signaling and energy metabolism to membrane formation, protein modification, and gene regulation.
FAQ
What are fatty acids?
Fatty acids are organic molecules that consist of a long hydrocarbon chain and a carboxyl group at one end. They are building blocks of lipids and play essential roles in various biological processes.
What is the function of fatty acids in the body?
Fatty acids serve as a source of energy for the body, contribute to the structure of cell membranes, support the absorption of fat-soluble vitamins, and are involved in the synthesis of hormones and signaling molecules.
Are all fatty acids the same?
No, fatty acids can vary in terms of chain length, degree of saturation, and configuration of double bonds. These differences impact their physical properties and biological functions.
What is the difference between saturated and unsaturated fatty acids?
Saturated fatty acids have no double bonds in their hydrocarbon chains, while unsaturated fatty acids have one or more double bonds. Saturated fats are typically solid at room temperature, while unsaturated fats are usually liquid.
Are all unsaturated fatty acids healthy?
Unsaturated fatty acids are generally considered healthier than saturated fats. However, the health effects depend on the specific types of unsaturated fats. Monounsaturated and certain polyunsaturated fats, like omega-3 fatty acids, are associated with health benefits.
What are essential fatty acids?
Essential fatty acids are types of polyunsaturated fats that the body cannot produce on its own and must be obtained from the diet. Examples include omega-3 and omega-6 fatty acids, which are important for proper growth, development, and functioning of the body.
Can fatty acids be synthesized in the body?
While the body can synthesize some fatty acids, it cannot produce essential fatty acids. Therefore, it is necessary to obtain them from dietary sources.
How are fatty acids metabolized in the body?
Fatty acids are broken down through a process called beta-oxidation, which occurs in the mitochondria of cells. This process generates energy by converting fatty acids into acetyl-CoA, which enters the citric acid cycle.
What is the role of fatty acids in cardiovascular health?
The types and amounts of fatty acids consumed can affect cardiovascular health. Consuming excessive saturated fats and trans fats is associated with an increased risk of heart disease, while consuming unsaturated fats, particularly omega-3 fatty acids, can have a protective effect.
Can fatty acids be obtained from plant-based sources?
Yes, plant-based sources like nuts, seeds, avocados, and vegetable oils are rich in various fatty acids. While animal products are also sources of fatty acids, it is possible to follow a balanced diet and obtain sufficient fatty acids solely from plant-based sources.