What is Enzyme?
- Enzymes are remarkable proteins that play a pivotal role in catalyzing non-spontaneous chemical reactions within biological systems. As vital components of an organism’s metabolic pathways, enzymes ensure the smooth execution of cellular tasks necessary for growth, repair, and energy acquisition.
- The metabolic network, a complex web of interconnected chemical reactions, adapts its activities in response to internal and external stimuli. For an organism to function and survive effectively, these metabolic responses must be specific in timing and circumstances. Regulatory enzymes are key players in this process, as they control the overall rate of metabolic pathways, determining the pace at which cellular tasks are accomplished.
- Enzymatic reactions are remarkably precise, occurring only in suitable cellular environments and proceeding at a rate proportional to the availability of required substrates or cofactors. The surrounding conditions heavily influence enzyme activity, with factors such as temperature, pH, substrate concentration, and the presence of inhibitors or activators affecting the rate of these chemical reactions.
- Biochemical reactions are the foundation of an organism’s growth, tissue repair, and energy generation. These essential reactions, collectively known as metabolism, continuously occur within all living organisms. If metabolism ceases to function, it inevitably leads to the organism’s demise.
- Metabolic reactions demand high activation energy to occur, which can be energetically costly for cells. To minimize energy consumption, nature has devised a solution – the enzyme. Enzymes serve as biological catalysts, consisting of large protein molecules that accelerate chemical reactions inside cells. Composed of chains of polypeptides made up of amino acids, enzymes remain unchanged during the reaction, enabling them to speed up reactions without being consumed.
- Enzymes exhibit a remarkable specificity compared to other catalysts, with each enzyme specialized for a particular substrate or a select few substrates, thereby ensuring the precise execution of specific reactions. By lowering the activation energy required to initiate a reaction, enzymes facilitate the rapid progress of essential cellular processes.
- Several factors influence the speed of an enzyme’s action. The concentration of the enzyme and substrate, as well as environmental factors like temperature and pH, play significant roles in determining the enzyme’s efficiency. Additionally, the presence of inhibitors can hinder the enzyme’s activity, while activators can enhance it.
- In summary, enzymes are essential and fascinating biological catalysts that orchestrate vital chemical reactions within living organisms. Through their specific and efficient actions, enzymes contribute to the overall functioning and survival of all life forms. Understanding the intricacies of enzymes not only advances our knowledge of biological systems but also opens the door to numerous applications in various fields, including medicine, biotechnology, and environmental science.
Factors That Affects Enzyme Activity
Enzyme activity, a crucial aspect of biological processes, is influenced by several key factors that dictate the rate and efficiency of enzymatic reactions. Understanding these factors is vital for comprehending the intricacies of enzyme functioning. Let us delve into the six main factors that affect enzyme activity:
- Concentration of Enzyme: The availability of enzymes significantly impacts the rate of enzymatic reactions. Higher enzyme concentrations generally lead to more rapid reactions, as there are more enzymes available to interact with substrates. Conversely, low enzyme concentrations can limit the reaction rate, even if an ample amount of substrate is present.
- Concentration of Substrate: The concentration of the substrate, the molecule upon which the enzyme acts, is another crucial determinant of enzyme activity. When substrate concentration increases, the probability of substrate-enzyme collisions rises, leading to more frequent enzyme-substrate interactions and, consequently, increased reaction rates. However, once the enzyme reaches its saturation point, further increases in substrate concentration will not enhance the reaction rate.
- Effect of Temperature: Temperature profoundly influences enzyme activity. As the temperature rises, so does the kinetic energy of molecules, including enzymes and substrates. This heightened energy results in more frequent collisions between the enzyme and substrate, thereby accelerating the reaction rate. However, excessively high temperatures can denature the enzyme, rendering it non-functional.
- Effect of pH: The pH level of the surrounding environment plays a critical role in enzyme activity. Enzymes exhibit optimal activity at specific pH values, which vary depending on the type of enzyme. Deviations from the optimal pH can denature the enzyme or alter its active site, leading to reduced catalytic efficiency.
- Effect of Product Concentration: The accumulation of reaction products can influence enzyme activity. In some cases, high product concentrations can inhibit enzyme activity through negative feedback mechanisms, ensuring that excessive product formation is controlled. This phenomenon helps maintain cellular homeostasis.
- Effect of Activators: Activators are molecules that enhance enzyme activity. These substances can bind to enzymes and induce conformational changes that increase the enzyme’s affinity for its substrate or stabilize the enzyme-substrate complex. As a result, the rate of the enzymatic reaction is boosted.
Factor 1: Enzyme Concentration
The relationship between enzyme concentration and enzyme activity is crucial for understanding biochemical reactions. When studying this effect, it is essential to maintain excess substrate levels, which allows for a clear observation of enzyme dynamics without substrate limitation. Below are key points detailing how enzyme concentration influences enzyme activity.
- Substrate Availability: The impact of enzyme concentration on enzyme activity is most evident when the substrate is present in excess. This scenario ensures that the reaction rate becomes independent of substrate concentration, allowing for an accurate assessment of enzyme effects.
- Zero-Order Reactions: To effectively study the relationship between enzyme concentration and enzyme activity, zero-order kinetics should be examined. In zero-order reactions, the rate of product formation is constant and directly related to enzyme concentration, assuming all active sites on the enzymes are occupied by substrate.
- Measuring Enzyme Activity: The determination of enzyme concentration in a system is achieved by measuring the amount of substrate converted into product over time. This quantification provides insight into the enzyme’s catalytic efficiency and overall activity.
- Influencing Factors: While evaluating enzyme concentration effects, it is vital to consider other factors that can influence enzyme activity, such as temperature and pH. These variables can significantly alter enzyme structure and function, thereby impacting the observed rates of reaction.
- Linear Relationship: Under conditions where the substrate is in excess, an increase in enzyme concentration leads to a linear increase in enzyme activity. For instance, if the reaction time is doubled, the quantity of products formed also doubles, demonstrating a direct relationship between enzyme concentration and reaction rate.
- Active Site Saturation: Enzyme activity continues to rise with increasing enzyme concentration until a point of saturation is reached. At this saturation point, all available active sites on the enzyme molecules are filled with substrate, and the enzyme activity reaches a maximum level. Beyond this point, further increases in enzyme concentration do not lead to an increase in the rate of reaction.
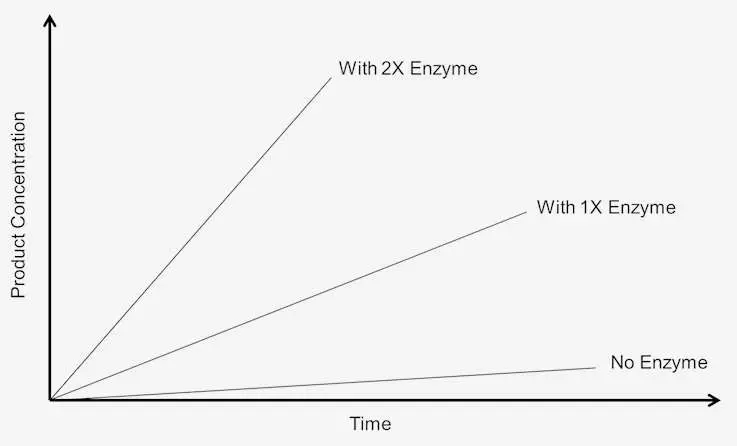
Factor 2: Substrate Concentration
Substrate concentration is a pivotal factor influencing the rate and efficiency of enzymatic reactions. The activity of enzymes is directly related to the availability of substrate, which must effectively interact with the enzyme’s active site for catalysis to occur. Below are key points that elucidate the effect of substrate concentration on enzyme activity.
- Constant Enzyme Concentration: The effect of substrate concentration on enzymatic action should be evaluated while keeping the enzyme concentration constant. Under these conditions, the reaction rate tends to increase with rising substrate concentrations up to a certain point.
- Rate Increase with Substrate: As substrate concentration increases, the number of substrate molecules available to bind to the active sites of the enzyme also rises. This enhanced interaction leads to an increase in the formation of enzyme-substrate complexes, thereby accelerating the reaction rate.
- Maximum Rate (Vmax): Eventually, the reaction reaches a maximum rate known as the maximum velocity (Vmax). At this stage, all the active sites on the enzyme molecules are occupied, resulting in saturation. Consequently, additional increases in substrate concentration do not alter the rate of reaction.
- Saturation of Active Sites: Once the enzyme is saturated with substrate, any further increase in substrate concentration fails to impact enzyme activity. This is because excess substrate molecules cannot participate in the reaction until the bound substrate has been converted into product and released from the enzyme.
- Kinetic Models: The relationship between substrate concentration and enzyme activity can be described using various kinetic models. Most enzymes adhere to Michaelis-Menten kinetics, which initially exhibit a linear increase in reaction rate as substrate concentration rises. This relationship, however, eventually plateaus as the enzyme becomes saturated.
- Allosteric Enzymes: In contrast, allosteric enzymes display a sigmoidal pattern of activity concerning substrate concentration. Initially, the reaction rate increases exponentially as substrate binding occurs. Once saturation is reached, the rate becomes linear, similar to Michaelis-Menten kinetics, ultimately plateauing.
- Biological Regulation: The regulation of substrate concentration is critical for optimal enzymatic function. Cellular mechanisms precisely control substrate availability in response to metabolic demands and regulatory molecules, ensuring efficient catalytic activity and resource utilization.
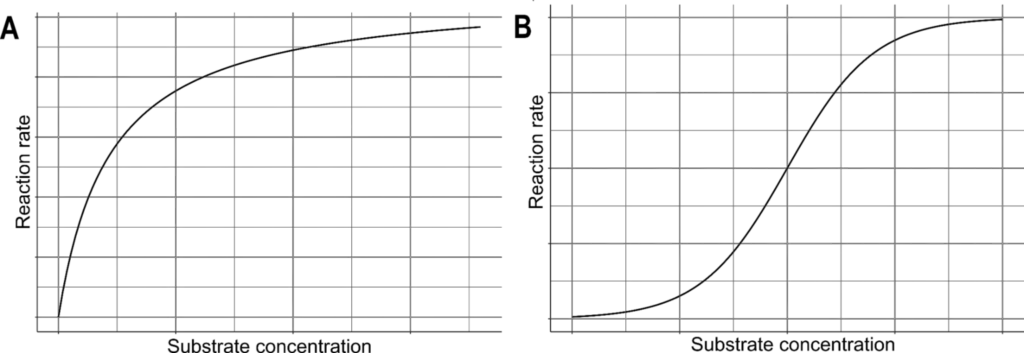
Factor 3: Effect of Temperature
Temperature plays a critical role in influencing enzyme activity, as enzymes are protein molecules sensitive to thermal changes. The relationship between temperature and enzymatic reactions can be described through various key points.
- Thermal Sensitivity: Enzymes are considered thermolabile, meaning their functionality can be significantly altered by temperature changes. As proteins, enzymes have specific structures that are sensitive to heat, which directly impacts their catalytic activity.
- Reaction Rate Increase: Typically, the rate of enzyme action increases with rising temperature. It is observed that for every 10°C increase, the reaction rate may double or even triple, enhancing the efficiency of enzymatic processes.
- Optimal Temperature: Each enzyme has an optimal temperature range where its activity is maximized. This optimal temperature is not easily defined due to variability in experimental conditions but often correlates with the physiological temperature of the organism from which the enzyme originates. For instance, mammalian enzymes typically function best within the range of 35°C to 45°C, whereas thermophilic bacteria possess enzymes with optimal temperatures around 80°C.
- Denaturation at High Temperatures: As temperature rises beyond the optimal range, enzymatic activity declines sharply. High temperatures, particularly above 60°C, can lead to irreversible changes in enzyme structure, causing denaturation and coagulation. This structural alteration prevents the enzyme from functioning effectively, thereby diminishing product formation.
- Low-Temperature Effects: At low temperatures, the catalytic activity of enzymes decreases significantly. As temperatures approach 0°C, enzymes may become inactive. However, this inactivation is typically reversible; if the temperature is subsequently raised to the optimal level, the enzyme can regain its activity.
- Initial Reaction Velocity: When assessing temperature effects on enzyme activity, it is noted that the initial velocity of reactions increases steadily with temperature. However, upon surpassing the optimal temperature, the velocity of the reaction diminishes, leading to a reduced yield of products.
- Storage Conditions: Temperature also impacts enzyme preservation. Enzyme preparations are best preserved through refrigeration or quick freezing, which helps maintain their activity over time. Such methods prevent thermal denaturation that can occur at higher temperatures.
Name | Description/habitat | Optimal pH | Optimal temperature |
---|---|---|---|
1. Thermococcus hydrothermalis | Prokaryotic archaea found in the East Pacific hydrothermal vent | 5.5 | 85°C |
2. Sulfolobus solfataricus | Prokaryotic archaea found in sulfur-rich volcanic fields | 3.0 | 80°C |
3. Halomonas meridiana | Gram-negative bacteria found in Antarctica salt lake | 7.0 | 37°C |
4. Pseudoalteromonas haloplanktis | Fast-growing bacteria found in Antarctic seawater | 7.6 | 4°C |
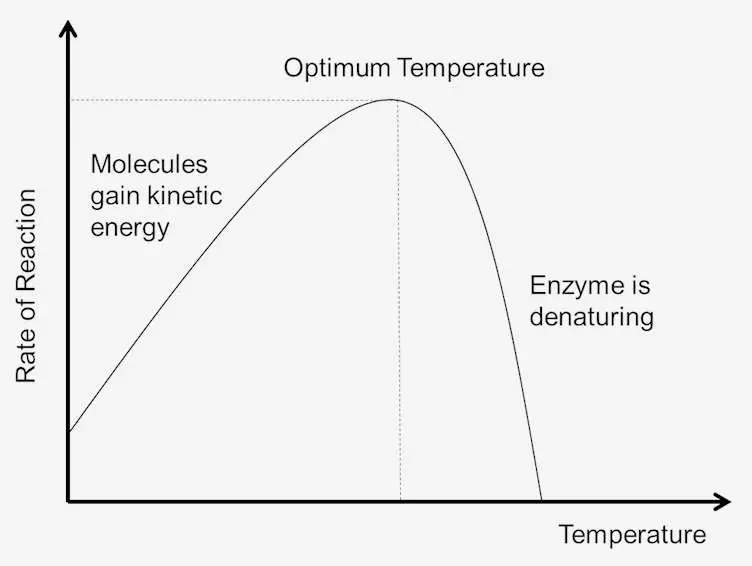
Factor 4: Effect of pH
The pH of a solution is a fundamental factor affecting enzyme activity, as it can significantly alter the shape and structure of enzymes. Enzymes, being protein molecules composed of amino acid chains, have specific pH ranges in which they function optimally.
- Enzyme Structure and Functionality: Each enzyme possesses a unique three-dimensional structure determined by the sequence and arrangement of its amino acids. The presence of charged groups, such as acidic carboxylic (-COOH) and basic amino (-NH2) groups, gives enzymes their characteristic electrical properties. Changes in pH can lead to the ionization or deionization of these groups, thereby affecting the enzyme’s conformation and its ability to bind substrates.
- Optimal pH: Every enzyme has an optimal pH at which its activity is maximized. This specific pH value varies from enzyme to enzyme, influenced by factors such as the enzyme’s composition, structure, and the nature of the substrate. For instance, pepsin operates best in highly acidic conditions, whereas trypsin functions optimally in more alkaline environments. Many enzymes in the human body exhibit optimal activity around a neutral pH of 7.4.
- Impact of pH Changes: Deviations from an enzyme’s optimal pH can lead to a decrease in activity. As the pH shifts away from this ideal range, the enzyme’s structure may change, impacting the formation of the enzyme-substrate complex. This alteration can hinder the catalytic capability of the enzyme, reducing the overall rate of reaction.
- Denaturation Risks: Extreme changes in pH can result in denaturation, a process that permanently disrupts the enzyme’s three-dimensional structure. Denatured enzymes lose their functional configuration and become inactive. Such changes are often irreversible, resulting in a permanent loss of enzymatic activity.
- Substrate Interaction: pH changes can also affect the substrate itself, altering its structure and preventing it from effectively binding to the enzyme’s active site. This disruption in substrate-enzyme interaction can further contribute to reduced enzyme activity.
- Buffer Systems: The use of buffer solutions is crucial in maintaining the optimal pH for enzymatic reactions. Buffer systems help stabilize the pH by minimizing fluctuations that could disrupt enzyme function. The choice of buffer and its pH level can significantly influence enzyme activity.
Enzyme | Function | pH range | Optimal pH |
---|---|---|---|
1. ɑ-Amylase | In saliva, amylase breaks down most polysaccharides in human diets. | 6.4 – 7.0 | 6.6 |
2. Pepsin | Pepsin is one of the many proteases found in the stomach’s gastric juice. It hydrolyzes peptide bonds in the protein’s amino acid chains. | 1.5 – 4.5 | 2 |
3. Trypsin | Found in the small intestine, trypsin is another protease that digests proteins. | 7.5 – 8.5 | 7.8 |
4. Alkaline Phosphatase (ALP) | ALP catalyzes the removal of phosphate groups from its substrate. It is found in all human tissue and is most abundant in the intestine and placenta. | 8 – 10 | 10 |
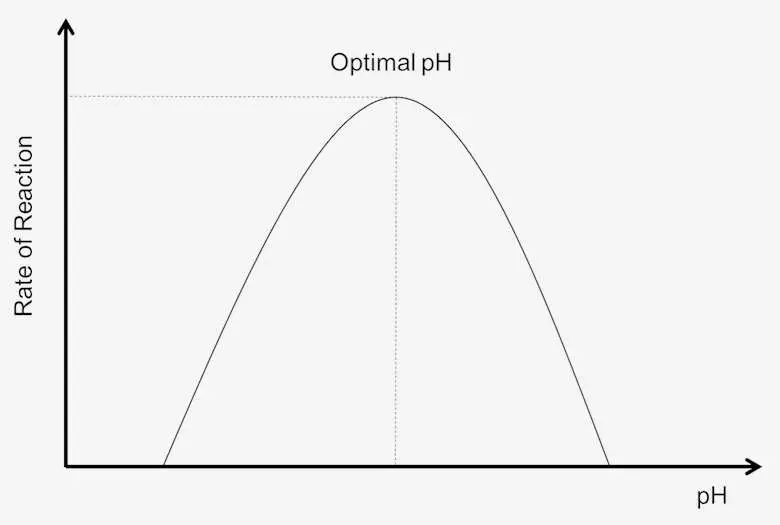
Factor 5: Effect of Effector or Inhibitor
Effectors and inhibitors play crucial roles in regulating enzyme activity, influencing the rate of enzymatic reactions and overall metabolic processes within organisms. By interacting with enzymes, these molecules can enhance or diminish enzymatic function, demonstrating the intricate control mechanisms within biological systems.
- Inhibitors: These compounds can convert enzymes into inactive forms, thereby adversely affecting the rate of enzymatically-catalyzed reactions. Inhibitors can bind either to the active site or other regions of the enzyme, obstructing substrate binding. As the concentration of inhibitors increases, the formation of the enzyme-substrate complex diminishes, resulting in a reduced concentration of products formed. The effects of inhibitors can be classified into different types:
- Competitive Inhibition: In this scenario, inhibitors compete with substrates for binding at the enzyme’s active site. The inhibitory effect can be alleviated by increasing substrate concentration, which enhances the likelihood of substrate binding over that of the inhibitor.
- Non-competitive Inhibition: In contrast, non-competitive inhibitors bind to an allosteric site rather than the active site, causing conformational changes that diminish enzyme activity regardless of substrate concentration. This type of inhibition cannot be overcome by merely adding more substrate.
- Uncompetitive Inhibition: This form of reversible inhibition occurs when the inhibitor binds exclusively to the enzyme-substrate complex, preventing product release and thus reducing the overall reaction rate.
- Effectors: These molecules are crucial for the activation or inhibition of enzyme activity and can include metal ions or other non-substrate molecules. Allosteric enzymes are particularly sensitive to effectors, which bind to regulatory sites distinct from the active site. When effectors bind to these sites, they induce conformational changes in the enzyme that can either enhance or inhibit catalytic activity. This mechanism allows cells to finely tune enzyme functions in response to varying metabolic demands.
- Reversible vs. Irreversible Inhibition: The impact of inhibitors can be reversible or irreversible. Reversible inhibitors bind temporarily to enzymes and can be displaced by other molecules. Conversely, irreversible inhibitors form permanent bonds with enzyme functional groups, leading to long-lasting inactivation.
- Metal Ions and Cofactors: Enzymes often require metal ions or cofactors for optimal activity. Common metallic cations, such as Mg²⁺, Mn²⁺, Zn²⁺, Ca²⁺, Co²⁺, Cu²⁺, Na⁺, and K⁺, play essential roles in various enzymatic processes. The presence of these ions can significantly enhance enzyme function, as they may be integral to the enzyme’s active site or assist in stabilizing substrate interactions.
Factor 6: Effect of Product Concentration
The concentration of products formed during enzymatic reactions is a significant factor influencing the velocity and efficiency of enzyme activity. As enzymes catalyze reactions, they convert substrates into products, and the accumulation of these products can impact enzyme performance in various ways.
- Product Inhibition: As reaction products accumulate, they may bind to the active site of the enzyme, forming a product-enzyme complex. This binding can inhibit enzyme activity, reducing catalytic efficiency. This phenomenon is termed product inhibition and serves as a crucial regulatory mechanism within biological systems.
- Regulatory Mechanisms Against Product Inhibition: To counteract the negative effects of product inhibition, living organisms have developed several strategies:
- Rapid Removal or Utilization of Products: Organisms employ mechanisms to quickly remove or convert products into other substances, preventing their accumulation at the enzyme’s active site. This approach allows enzymes to continue functioning effectively.
- Secondary Enzymes: Many systems utilize specific enzymes dedicated to degrading or utilizing reaction products. These secondary enzymes help transform products into forms that can be further utilized or excreted, thereby maintaining optimal enzyme activity.
- Feedback Inhibition: Feedback inhibition is another regulatory strategy where the final product of a metabolic pathway inhibits one of the earlier enzymes in that pathway. This creates a negative feedback loop that helps regulate the entire pathway’s rate, preventing excessive accumulation of the final product.
- Impact on Metabolic Pathways: The effect of product concentration on enzyme activity is critical in maintaining cellular processes. Product inhibition can slow down enzymatic reactions, potentially disrupting metabolic pathways and affecting overall cellular function. Therefore, the ability of cells to mitigate the impact of product accumulation is vital for ensuring that enzymatic reactions proceed efficiently and accurately.
Examples
- Factor 1: Enzyme Concentration Example: Imagine a scenario where you have an enzyme that breaks down a complex sugar molecule into simpler sugars. If you increase the concentration of this enzyme in a test tube containing the substrate (complex sugar), the reaction rate will increase as more enzyme molecules are available to interact with the substrate. Conversely, if you decrease the enzyme concentration, the reaction rate will slow down due to a lower number of available enzymes to catalyze the reaction.
- Factor 2: Substrate Concentration Example: Let’s consider an enzyme that converts hydrogen peroxide (H2O2) into water (H2O) and oxygen gas (O2). If you increase the concentration of hydrogen peroxide in the reaction mixture, the enzyme’s activity will increase, as there will be more substrate molecules available for the enzyme to act upon. However, once all the enzyme’s active sites are fully occupied with substrate molecules, further increases in hydrogen peroxide concentration will not affect the reaction rate.
- Factor 3: Effect of Temperature Example: Take the example of an enzyme that functions optimally at body temperature (around 37°C). At this temperature, the enzyme’s activity is at its highest because the kinetic energy of molecules is sufficient for efficient enzyme-substrate collisions. However, if you increase the temperature beyond a certain point, such as 50°C, the enzyme’s three-dimensional structure may start to denature, leading to a significant reduction in enzyme activity.
- Factor 4: Effect of pH Example: Consider an enzyme responsible for breaking down proteins in the stomach. This enzyme, known as pepsin, works optimally at a low pH (acidic environment) of around 2.0. If you change the pH to a more basic environment (higher pH), pepsin’s activity will decrease significantly because the enzyme’s active site is adapted to function best in an acidic environment.
- Factor 5: Effect of Activators Example: An example of an enzyme that requires an activator is the enzyme hexokinase, which catalyzes the first step in glucose metabolism. Hexokinase requires the presence of magnesium ions (Mg2+) as a cofactor to function properly. The magnesium ions serve as activators, enhancing the enzyme’s catalytic activity and facilitating the conversion of glucose to glucose-6-phosphate.
- Factor 6: Effect of Product Concentration Example: Imagine an enzyme that converts a precursor molecule (A) into a product (B). As the enzyme catalyzes the reaction, the concentration of product B starts to increase. If the product concentration becomes too high, B molecules may start to bind to the enzyme’s active site, leading to product inhibition. This inhibitory effect slows down the enzyme’s activity, preventing the excessive accumulation of product B. The cell may have additional enzymes or regulatory mechanisms to utilize or remove product B from the reaction to maintain enzyme activity.
What is the affinity of different enzymes for their substrate?
The affinity of enzymes for their substrates is a crucial determinant of enzymatic activity and catalysis. This affinity influences how effectively an enzyme can bind to its substrate and catalyze a reaction, ultimately affecting the rate of the enzymatic process.
- Saturation and Maximum Reaction Rate: As substrate concentration increases, while keeping other conditions constant, the rate of catalysis by an enzyme reaches a maximum value. This phenomenon occurs because all available enzyme molecules bind to the substrate or its products, leading to saturation of the enzyme’s active sites. Therefore, beyond a certain concentration, increasing substrate levels will not further enhance the reaction rate.
- Michaelis Constant (Km): The affinity of an enzyme for its substrate is quantitatively described by the Michaelis constant (Km). Km represents the substrate concentration at which the rate of reaction or enzyme activity is half of its maximum velocity (Vmax). A lower Km indicates a higher affinity of the enzyme for its substrate, as it requires a smaller concentration of substrate to achieve half-maximal enzymatic activity. Conversely, a higher Km suggests a lower affinity.
- Natural Substrates: The substrate that corresponds to the lowest Km value for a given enzyme is often considered the enzyme’s natural substrate, although this is not universally applicable to all enzymes. This means that enzymes are typically more efficient at catalyzing reactions involving substrates that they have a higher affinity for.
- Influence of Enzyme and Substrate Nature: The affinity between an enzyme and its substrate is influenced by several factors, including the specific characteristics of both the enzyme and the substrate, as well as the nature of their interaction. For instance:
- Hydrolytic enzymes that act on crystalloidal substrates tend to exhibit a low affinity.
- Oxidizing-reducing enzymes, excluding catalase, are generally associated with a higher affinity for their substrates.
- Proteolytic enzymes that hydrolyze colloidal substances fall into a category with medium affinity for substrates like oxygen, hydrogen peroxide, and xanthine.
How does enzyme immobilization in alginate compare to the use of free enzymes in solution?
The immobilization of enzymes refers to the process of confining enzyme molecules to a distinct phase, separate from the substrates and products involved in a reaction. This technique can significantly alter the behavior and characteristics of enzymes, particularly when immobilized in materials like calcium alginate.
- Immobilization Process: The creation of calcium alginate involves reacting a mixture of sodium alginate solution and enzyme solution with calcium chloride. This reaction results in a gel-like structure that encapsulates the enzymes, allowing for controlled enzymatic activity.
- Stability and Resistance: Enzymes immobilized on carrier matrices such as alginate exhibit greater stability than free enzymes in solution. Specifically, immobilized enzymes demonstrate increased resistance to fluctuations in pH and temperature, making them more robust under varying reaction conditions. This stability can be critical in industrial applications where conditions may not be perfectly controlled.
- Advantages of Immobilized Enzymes:
- Reusability: One of the key benefits of using immobilized enzymes is the ability to recover them from the reaction mixture. This reusability not only reduces costs but also minimizes waste, making the process more efficient.
- Sustained Activity: Immobilized enzymes maintain their activity for extended periods. While the activity of free enzymes typically increases with enzyme concentration and incubation time up to a certain threshold, immobilized enzymes can continue to show increased activity even after prolonged incubation periods. This property allows for greater flexibility and efficiency in various applications.
- Behavior of Free Enzymes: Free enzymes in solution tend to exhibit a different behavior compared to their immobilized counterparts. The activity of free enzymes generally rises with an increase in enzyme concentration, but only up to a certain point. After this peak, additional increases in concentration do not lead to further enhancements in activity, indicating potential saturation or inhibition effects.
What are the advantages of using immobilized enzymes?
Immobilized enzymes offer a range of benefits that enhance their utility in various biochemical applications. The process of immobilizing enzymes—confining them to a solid support—facilitates their repeated use and enhances their operational characteristics.
- Reusability and Ease of Separation: One of the primary advantages of immobilized enzymes is their ability to be easily separated from the reaction mixture after use. This contrasts with free enzymes, which can be more challenging to recover. The ease of separation not only simplifies the purification process but also enables the enzymes to be reused multiple times, significantly reducing overall enzyme costs.
- Application in Diverse Systems: Immobilized enzymes can be utilized in non-aqueous environments, expanding their applicability in situations where aqueous systems may not be suitable. This flexibility allows for innovative approaches in industries that require specific solvent conditions.
- Continuous Production Systems: The use of immobilized enzymes facilitates continuous production systems, which can streamline processes that are not feasible with free enzymes. This ability to maintain ongoing enzymatic reactions enhances productivity and efficiency in industrial settings.
- Increased Thermostability: Immobilized enzymes demonstrate higher thermostability compared to their free counterparts. This stability enables them to function effectively under a wider range of temperatures, thereby improving their performance in various operational conditions.
- Enhanced Properties and Concentration: The properties of immobilized enzymes are often enhanced, allowing for improved catalytic activity. Furthermore, immobilized enzymes can be used at significantly higher concentrations than free enzymes, which can lead to increased reaction rates and overall efficiency in biochemical processes.
- Multi-Enzyme Reactions: Immobilization allows for the possibility of attaching multiple enzymes to a single support, facilitating multi-enzyme reactions. This capability can be particularly advantageous in complex biochemical pathways where cooperation between different enzymes is necessary.
- Reduction of Effluent Problems: Systems utilizing immobilized enzymes tend to generate less effluent compared to those using free enzymes. This reduction in waste is beneficial for environmental considerations and contributes to more sustainable industrial practices.
FAQ
What factors affect enzyme activity?
Enzyme activity can be influenced by several factors, including temperature, pH, enzyme concentration, substrate concentration, presence of activators, and the concentration of reaction products.
How does temperature affect enzyme activity?
Temperature can significantly impact enzyme activity. Enzymes generally have an optimal temperature at which they work most efficiently. Deviations from this optimal temperature can either increase or decrease enzyme activity, with extreme temperatures leading to denaturation and loss of function.
How does pH affect enzyme activity?
The pH level of the environment in which enzymes operate can affect their activity. Each enzyme has an optimal pH at which it functions best. Deviations from this pH can alter the enzyme’s conformation and reduce its catalytic efficiency.
What is the relationship between enzyme concentration and activity?
Higher enzyme concentrations usually result in faster enzymatic reactions, as more enzyme molecules are available to interact with substrates. However, beyond a certain point, increasing enzyme concentration may not lead to a proportional increase in reaction rate.
How does substrate concentration influence enzyme activity?
Increasing substrate concentration generally increases enzyme activity until a saturation point is reached. At this point, all enzyme active sites are occupied by substrates, and further increases in substrate concentration do not significantly affect the reaction rate.
What are enzyme activators?
Enzyme activators are molecules that enhance the catalytic activity of enzymes. They may bind to the enzyme and induce conformational changes that increase the enzyme’s affinity for its substrate, leading to more efficient reactions.
How do inhibitors affect enzyme activity?
Inhibitors are molecules that reduce or completely halt enzyme activity. They can either bind to the active site of the enzyme (competitive inhibition) or to an allosteric site, leading to conformational changes that hinder catalytic activity (non-competitive inhibition).
What is product inhibition?
Product inhibition occurs when the accumulation of reaction products inhibits the enzyme’s activity. The products can bind to the enzyme’s active site or allosteric sites, reducing its ability to catalyze further reactions.
How do living systems prevent product inhibition?
Living systems have evolved mechanisms to prevent or mitigate product inhibition. These mechanisms include rapid removal of products, utilization of secondary enzymes to degrade or utilize products, and feedback inhibition, where the final product of a metabolic pathway regulates an earlier enzyme in the pathway.
Why is enzyme activity regulation important?
Regulation of enzyme activity is crucial for maintaining proper metabolic balance and cellular function. Precise control of enzymatic reactions ensures that biochemical processes occur at the right time, in the right place, and with the appropriate rate to meet the demands of the organism.
- Factors Affecting Enzyme Activity. (2022, July 26). Community College of Baltimore Country (Cantonsville). https://bio.libretexts.org/@go/page/93116
- Factors Affecting Enzyme Activity. (2023, August 5). https://chem.libretexts.org/@go/page/86311
- Shrief, Eman. (2020). Factors Affecting Enzyme Activity.
- Voet D, Voet JG and Pratt CW, Fundamentals of Biochemistry, 2nd edition. New Jersey: John Wiley & Sons; 2006.
- Boyer R, Concepts in Biochemistry, 3rd edition. New Jersey: John Wiley & Sons; 2006.
- Punekar, N S. 2018. “Hallmarks of an Enzyme Catalyst.” In ENZYMES: Catalysis, Kinetics and Mechanisms, 43–51. Singapore: Springer Singapore. doi:10.1007/978-981-13-0785-0_5.
- Marini I. Discovering an accessible enzyme: Salivary α-amylase : Prima digestio fit in ore: A didactic approach for high school students. Biochem Mol Biol Educ. 2006;33(2):112-116. doi:10.1002/bmb.2005.494033022439
- Piper DW, Fenton BH. pH stability and activity curves of pepsin with special reference to their clinical importance. Gut. 1965;6(5):506-508. doi:10.1136/gut.6.5.506
- Lam MPY, Lau E, Liu X, Li J, Chu IK. Sample Preparation for Glycoproteins. In: Comprehensive Sampling and Sample Preparation. Elsevier; 2012:307-322. doi:10.1016/B978-0-12-381373-2.00085-5
- https://www.worthington-biochem.com/tools-resources/intro-to-enzymes/factors-affecting-enzyme-activity
- https://www.mmcmodinagar.ac.in/bridge-library/pdf/chemistry/M.Sc-III-sem-H-3011-Dr-Mamta-Gupta-28-29,July-2020-FACTORS-AFFECTING-ENZYME-ACTIVITY.pdf
- https://conductscience.com/factors-that-affect-enzyme-activity/?srsltid=AfmBOooaU8Kn_DQfAOjk3Ss8uSDyk_dW_HsrK-kY8V0OAGMAGpjSgndv
- https://www.bbc.co.uk/bitesize/guides/z9jrng8/revision/3
- https://www.khanacademy.org/science/ap-biology/cellular-energetics/environmental-impacts-on-enzyme-function/a/hs-enzymes-review
- https://unacademy.com/content/cbse-class-11/study-material/biology/factors-affecting-enzyme-action/
- https://www.vedantu.com/biology/factors-affecting-enzyme-activity
- https://www.toppr.com/ask/question/explain-the-factors-affecting-enzyme-activity/
- https://byjus.com/question-answer/what-affects-enzyme-action/
- https://www.britannica.com/question/What-factors-affect-enzyme-activity
- https://www.savemyexams.com/gcse/biology/edexcel/18/revision-notes/1-key-concepts-in-biology/1-2-enzymes/1-2-2-factors-affecting-enzymes/
- https://www.toppr.com/ask/question/what-are-the-factors-which-influence-enzyme-activity-discuss-them-in-brief/
- https://alevelbiology.co.uk/notes/factors-affecting-enzyme-activity/