Structure of The Kidney
The kidney serves as a vital organ in the excretory system of many animals, functioning to filter waste from the bloodstream and regulate fluid balance. While mammalian kidneys are more complex than their fish counterparts, understanding their structure and function provides insight into their crucial role in maintaining homeostasis.
- Location and Structure:
- In fish, kidneys are paired, small, and soft structures that typically extend along the vertebral column and are surrounded by coelomic epithelium.
- The kidneys in fish vary in type and location depending on the species, with differences observed between various teleost fish.
- Functional Unit – Nephron:
- The nephron is the fundamental functional unit of the kidney, comprising:
- Glomerulus: A network of capillaries where blood filtration occurs. Here, blood plasma is filtered to form the glomerular filtrate.
- Renal Tubule: This structure processes the filtrate and is segmented into specific regions:
- Bowman’s Capsule: A cup-like sac that encases the glomerulus and collects the filtrate.
- Proximal Tubule I: Responsible for reabsorbing nutrients and a significant portion of water and electrolytes.
- Proximal Tubule II: Continues the reabsorption process, further concentrating the filtrate.
- Distal Tubule: Involved in the selective reabsorption and secretion of ions, playing a crucial role in electrolyte balance.
- Collecting Duct: Finalizes the concentration of urine through further water reabsorption, regulated by hormones.
- The nephron is the fundamental functional unit of the kidney, comprising:
- Types of Kidneys in Fish:
- Fish exhibit two primary types of kidneys:
- Pronephric Kidney: Typically found in larval stages or some primitive species, characterized by a simpler structure.
- Mesonephric Kidney: More advanced and prevalent in adult fish, featuring a greater number of nephrons.
- Fish exhibit two primary types of kidneys:
- Classification by Ogawa (1961):
- Ogawa classified teleostean kidneys into five distinct classes based on their structure and function.
- Teleost fish possess two distinct regions within their kidney:
- Head Kidney: Comprising the pronephric components located in the anterior region.
- Trunk Kidney: Comprising the mesonephric components situated in the posterior region.
- Nephron Bundles:
- Teleost fish kidneys contain bundles of nephrons, each comprising glomeruli, renal tubules, and collecting ducts.
- The structure allows for efficient filtration and processing of urine.
- Archinephric Ducts:
- The kidneys are connected to two regions of archinephric ducts that converge at the posterior end.
- This arrangement facilitates the transport of urine from the kidneys to the bladder or exterior.
Functions of The Kidney
The kidney serves as a crucial organ for maintaining homeostasis in various organisms, particularly in aquatic environments. Its primary functions encompass osmoregulation, waste excretion, and maintaining fluid balance. By examining these functions, one can appreciate the kidney’s role in adapting to different environmental conditions.
- Osmoregulation:
- The kidney is essential for regulating the body’s fluid and electrolyte balance. This function is particularly vital for fish, which inhabit diverse aquatic environments, including freshwater and marine ecosystems.
- In freshwater environments, fish kidneys work to conserve solutes and maintain a higher concentration of salts in their bloodstream compared to their external environment. The process involves:
- Osmosis: Water from the surrounding environment diffuses into the fish’s body, driven by the lower solute concentration outside.
- Excretion of Excess Water: To counteract the influx of water, the kidneys excrete surplus water, thus preventing dilution of body fluids and maintaining optimal salt concentrations.
- Adaptations to Marine Environments:
- In marine environments, where the surrounding water has a higher salt concentration than the fish’s internal body fluids, the kidney adapts to counteract osmotic pressure:
- Water Loss: Due to the osmotic gradient, water naturally moves out of the fish’s body.
- Intake of Water: Fish compensate for this loss by actively drinking seawater.
- Excretion of Excess Salt: The kidneys excrete excess salt, enabling the fish to retain water and maintain homeostasis despite living in a saline environment.
- In marine environments, where the surrounding water has a higher salt concentration than the fish’s internal body fluids, the kidney adapts to counteract osmotic pressure:
- Waste Excretion:
- The kidneys filter and excrete various metabolic waste products, which include:
- Nitrogenous Wastes: Urine contains urea, creatinine, and ammonia, all of which are byproducts of protein metabolism.
- The kidneys serve to eliminate these wastes from the bloodstream, facilitating their removal from the organism.
- The kidneys filter and excrete various metabolic waste products, which include:
- Role of Gills:
- Besides the kidneys, fish gills also play a role in excreting ammonia directly into the surrounding water, enhancing the elimination of nitrogenous wastes.
- Urine Composition:
- Urine is primarily composed of water, along with solutes such as creatine, creatinine, urea, ammonia, and other nitrogenous waste products. This composition varies depending on the fish’s environment and its physiological needs.
- Urinary Bladder:
- Some fish possess a urinary bladder, which is a posterior evagination of the mesonephric ducts. It is mesodermal in origin, distinguishing it from the endodermal urinary bladders found in tetrapods. This organ serves to store urine temporarily before excretion.
Excretion of nitrogenous wastes, water and ion balance
The excretion of nitrogenous wastes, along with the regulation of water and ion balance, is crucial for the survival of fish in their aquatic environments. Fish employ multiple systems for excretion, including the kidneys, gills, digestive tract, and skin. Understanding these mechanisms is essential to appreciate how fish maintain homeostasis in the face of varying environmental conditions.
- Excretory Mechanisms:
- The primary organ responsible for excretion in fish is the kidney, but significant contributions also come from the gills, which facilitate ammonia excretion, and the skin.
- The digestive tract also plays a role in eliminating wastes, highlighting the multifaceted approach fish utilize to maintain internal balance.
- Osmoregulation:
- Fish must regulate their internal environment to maintain a stable concentration of dissolved solutes, known as homeostasis. This regulation is particularly influenced by their habitat—either freshwater or marine environments.
- Freshwater Fish:
- Freshwater fish have larger kidneys and numerous glomeruli (approximately 10,000 per kidney), with sizes ranging from 48 to 104 µm.
- These fish experience water influx due to osmosis, necessitating the excretion of large volumes of dilute urine to prevent water retention or “waterlogging.”
- To compensate for the loss of salts to the surrounding water, freshwater fish actively absorb ions through specialized cells in their gills and skin. This process allows them to transport salts against the concentration gradient.
- Freshwater fish consume little water and derive minimal hydration from their food, relying primarily on dietary sources for salt intake.
- Marine Fish:
- In contrast, marine fish must conserve water due to the high salinity of seawater. Their kidneys are adapted to excrete small amounts of water while retaining as much as possible.
- Marine fish often drink large quantities of seawater to maintain hydration, excreting the excess salts through specialized cells known as chloride cells in their gills.
- Nitrogenous waste, primarily in the form of ammonia, is expelled through the gills, minimizing the burden on the kidneys.
- Some marine species, such as sharks and rays, possess blood osmotic concentrations that match that of seawater, allowing them to balance their internal environment without needing to drink additional water. They maintain osmotic pressure by retaining urea in their blood.
- Ammonotelism and Nitrogenous Waste Management:
- Many fish are classified as ammonotelic, meaning they primarily excrete ammonia directly into the water. However, certain species possess mechanisms to detoxify ammonia into less toxic compounds, such as urea or glutamine.
- Some fish can tolerate high ammonia levels in the brain, utilizing adaptations to enhance ammonia excretion through active transport of ammonium ions (NH₄⁺), manipulation of the ambient pH, and reducing ammonia permeability through their gill and skin membranes.
Urea cycle
The urea cycle, also known as the ornithine-urea cycle (OUC), plays a critical role in nitrogen excretion and osmoregulation in certain groups of fish, particularly marine elasmobranchs and some teleosts. This cycle allows these fish to convert ammonia, a toxic byproduct of protein metabolism, into urea, which is less harmful and can be excreted efficiently.
- Basic Function:
- The primary purpose of the urea cycle in fish is to synthesize urea from ammonia, facilitating its safe excretion and helping to regulate osmotic balance in marine environments.
- Ureogenic Fishes:
- Elasmobranchs, holocephalans, and coelacanths are classified as ureogenic, meaning they possess a functional OUC primarily located in the mitochondria of liver and muscle cells.
- Urea serves as a significant end product of nitrogen metabolism in these species, with more than 50% of nitrogenous wastes excreted as urea, thereby designating them as ureotelic.
- Urea Transport and Retention:
- In marine elasmobranchs, the permeability of urea in the gills is reduced to facilitate retention of urea within the body. This is achieved through specialized Na⁺-coupled urea transporters located in the gills and kidneys.
- Additionally, modifications to the lipid composition of the gills, characterized by a higher cholesterol-to-phospholipid ratio, further assist in retaining urea.
- Enzymatic Components:
- The urea cycle involves several key enzymes:
- Carbamoyl Phosphate Synthetase III (CPS III): This enzyme uses glutamine as a substrate to produce carbamoyl phosphate, initiating the urea synthesis process.
- Ornithine Transcarbamoylase (OTC): Converts carbamoyl phosphate into citrulline.
- Argininosuccinate Synthetase and Argininosuccinate Lyase: These enzymes act in the cytosol to further convert citrulline into arginine.
- Arginase: This enzyme, found in the mitochondrial matrix of fish (except lungfishes), catalyzes the final step of urea synthesis, converting arginine to urea and regenerating ornithine for the cycle to continue.
- The urea cycle involves several key enzymes:
- Ammonia Detoxification:
- The process begins with the detoxification of ammonia, where ammonia is converted to glutamine, which then feeds into the urea cycle. The localization of CPS III and glutamine synthetase (GS) within the liver mitochondria allows for efficient urea synthesis while mitigating ammonia toxicity.
- Energy Consumption:
- The urea cycle is energy-intensive, utilizing a significant amount of ATP. Specifically, approximately five moles of ATP are consumed for each mole of urea synthesized. In teleosts, a similar amount of energy is expended—around 2.5 moles of ATP per mole of nitrogen assimilated.
- Adaptations in Teleosts:
- Certain teleosts, such as the gulf toadfish (Opsanus beta), exhibit ureotelic behavior, excreting over 50% of their nitrogenous waste as urea, particularly during conditions of ammonia loading.
Osmoregulation in fish
Osmoregulation is a vital physiological process that allows fish to maintain fluid and electrolyte balance within their bodies, adapting to diverse environmental conditions, whether they inhabit freshwater, seawater, or estuarine systems. This process is crucial for survival, as it ensures that fish can thrive in habitats that present varying osmotic challenges.
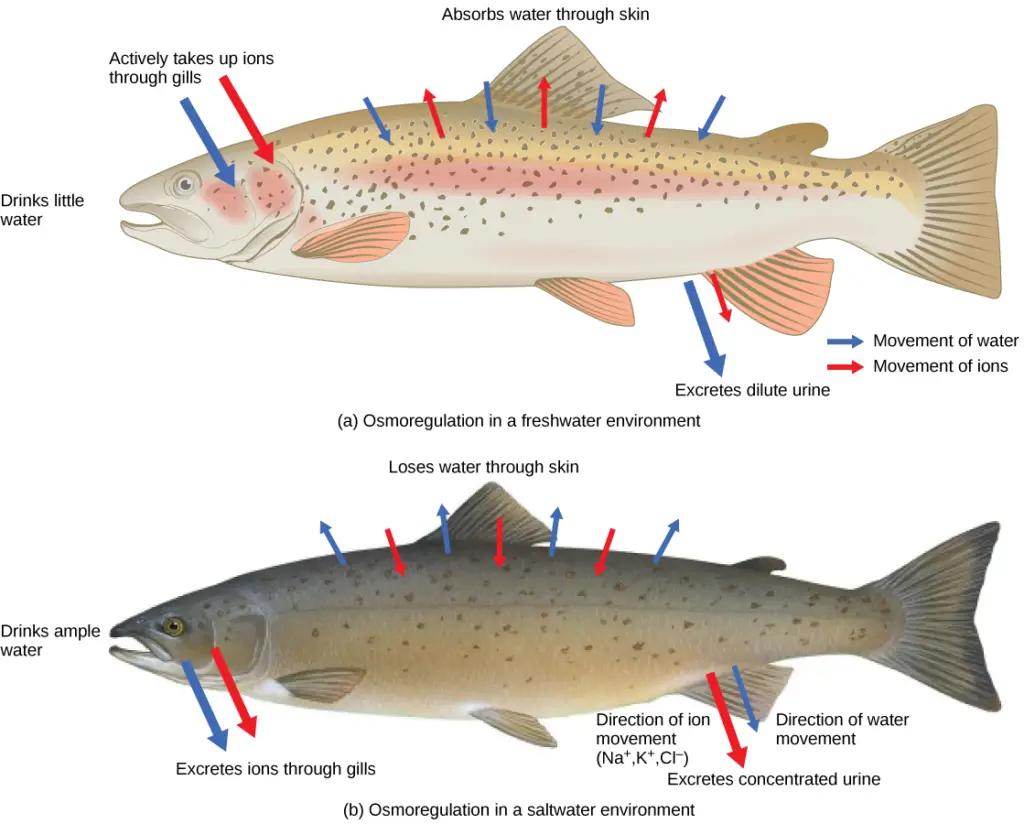
- Freshwater Fish:
- Freshwater environments are characterized as hypoosmotic, meaning they contain fewer solutes than the fish’s bodily fluids. Consequently, freshwater fish encounter two primary challenges:
- Osmotic Water Loading: Water tends to enter the fish’s body through osmosis.
- Salt Depletion: Fish naturally lose salts (primarily NaCl) to the surrounding water.
- To counteract these challenges, freshwater fish utilize the following mechanisms:
- Active Uptake of Sodium Chloride: Specialized cells in the gills actively absorb Na+ and Cl− ions from the water.
- Renal Excretion of Water: The kidneys play a pivotal role in excreting excess water. The nephron structure, comprising glomeruli, proximal and distal tubules, and collecting ducts, facilitates this process.
- Glomeruli filter large volumes of extracellular fluid, allowing for the reabsorption of filtered salts while excreting dilute urine.
- The distal nephron and urinary bladder reabsorb filtered salts without water, helping to conserve overall fluid volume.
- Freshwater environments are characterized as hypoosmotic, meaning they contain fewer solutes than the fish’s bodily fluids. Consequently, freshwater fish encounter two primary challenges:
- Marine Fish:
- Marine environments are hyperosmotic, presenting a different set of osmoregulatory challenges. Fish in these environments lose water through osmosis and gain salts through diffusion.
- Key adaptations in marine fish include:
- Water Replacement through Sea Water Consumption: Marine fish consume large quantities of seawater to replace lost body fluids.
- Gills for Salt Excretion: Gills assist in the excretion of excess NaCl gained from ingested seawater.
- Kidneys for Divalent Ion Excretion: The kidneys excrete divalent ions such as magnesium (Mg), calcium (Ca), and sulfate (SO₄) while managing overall osmotic balance.
- In stenohaline marine fish, the size and function of glomeruli may be reduced or absent, shifting the excretion of divalent ions primarily to renal tubules.
- Cartilaginous Fish:
- Cartilaginous fishes, including sharks, skates, rays, and chimeras, possess unique adaptations for osmoregulation:
- Urea-Based Osmoregulation: These species use urea as an osmolyte, allowing them to regulate osmotic pressure effectively.
- Complex Nephron Structure: Their kidneys feature a four-loop configuration and specific zones that enhance urea reabsorption from primary urine.
- Plasma Ion Regulation: They maintain plasma ion concentrations at approximately half that of surrounding seawater while storing high urea concentrations, rendering their body fluids slightly hyperosmotic to seawater and minimizing dehydration.
- Ornithine Urea Cycle (OUC): This metabolic pathway is crucial for synthesizing and maintaining elevated urea levels, providing an osmotic advantage.
- Cartilaginous fishes, including sharks, skates, rays, and chimeras, possess unique adaptations for osmoregulation:
- Osmotic Capacity Classifications:
- Elasmobranchs can be classified based on their osmotic capacities:
- Stenohaline Marine Species: Limited to stable, saline environments.
- Stenohaline Freshwater Species: Adapted to freshwater habitats with narrow salinity tolerances.
- Euryhaline Species: Capable of thriving in a wide range of salinities.
- Elasmobranchs can be classified based on their osmotic capacities:
- Sturgeon Adaptations:
- Sturgeons demonstrate varied salinity tolerance, categorized into three types:
- Stenohaline Species (e.g., lake sturgeon): Thrive in specific salinities throughout their lifecycle.
- Euryhaline and Anadromous Species (e.g., Adriatic sturgeon): Can migrate between freshwater and brackish environments, spawning in freshwater.
- Amphihaline and Diadromous Species (e.g., green sturgeon): Adapt to both freshwater and seawater, returning to freshwater to spawn after growing in marine conditions.
- Sturgeons demonstrate varied salinity tolerance, categorized into three types:
Stenohaline Teleosts, Euryhaline Teleosts, Migratory Teleosts
The classification of teleosts based on their salinity tolerance and migratory behaviors highlights the remarkable adaptations of these fish to varying aquatic environments. Stenohaline, euryhaline, and migratory teleosts exhibit distinct physiological mechanisms that allow them to thrive in freshwater and seawater, showcasing their evolutionary resilience and ecological versatility.
- Stenohaline Teleosts:
- Stenohaline fish are primarily adapted to live in environments with stable salinity, either freshwater or seawater. These species exhibit the following characteristics:
- Limited Osmotic Tolerance: They cannot tolerate significant fluctuations in salinity and are generally confined to one type of environment throughout their life cycle.
- Impenetrable Skin: The skin of stenohaline teleosts is relatively impermeable to water and ions, minimizing unwanted osmotic exchanges.
- Gills Functionality: Gills have a large surface area with permeable epithelium, allowing for the exchange of ions with the surrounding water. In freshwater species, this results in a passive influx of water and a loss of ions, while the reverse occurs in seawater species.
- Osmoregulation Strategies:
- Ion Retention: Freshwater stenohaline fish actively uptake ions through the gills and dietary sources to counteract ion loss.
- Water Excretion: These fish produce copious amounts of dilute urine to remove excess water gained through osmosis.
- The kidney structure in freshwater stenohaline teleosts aids in excreting water, producing dilute urine. In contrast, seawater stenohaline teleosts have adapted their kidneys to excrete divalent cations like calcium and magnesium while conserving water by minimizing urine production.
- Some marine teleosts have evolved aglomerular kidneys that lack glomeruli, preventing significant urine production while still allowing for solute regulation.
- Stenohaline fish are primarily adapted to live in environments with stable salinity, either freshwater or seawater. These species exhibit the following characteristics:
- Euryhaline Teleosts:
- Euryhaline teleosts possess the unique ability to transition between freshwater and seawater. They exhibit the following adaptations:
- Flexible Osmoregulation: These fish can alter their osmoregulatory processes to accommodate changes in environmental salinity. For example, catadromous species, such as eels, migrate from freshwater to spawn in the ocean, while anadromous species, like salmon, move from the sea to freshwater for spawning.
- Renal Adaptations: When euryhaline teleosts switch between environments, they undergo significant changes in kidney function:
- Glomerular Filtration Rate (GFR): The GFR is typically higher in freshwater conditions, facilitating greater urine production.
- Divalent Ion Regulation: In freshwater, euryhaline teleosts actively pump divalent cations out of the filtrate, whereas in seawater, they switch to pumping these ions into the filtrate.
- Tubule Permeability Adjustments: The distal segments and collecting tubules of their kidneys change permeability, allowing for reabsorption or excretion of water based on the surrounding salinity.
- Euryhaline teleosts possess the unique ability to transition between freshwater and seawater. They exhibit the following adaptations:
- Migratory Teleosts:
- Many teleosts exhibit migratory behaviors that involve traveling between freshwater and seawater environments. Notable examples include salmon, which undertake extensive migrations during their life cycle.
- Osmotic Gradient Reversal: These migratory fish can adjust their physiological processes to accommodate the reversal of osmotic gradients encountered during their migrations.
- Intermediate Habitats: Migratory teleosts often utilize brackish waters, which serve as transitional environments, allowing them to gradually adjust to the salinity changes before entering freshwater or seawater.
- Many teleosts exhibit migratory behaviors that involve traveling between freshwater and seawater environments. Notable examples include salmon, which undertake extensive migrations during their life cycle.