Key Facts
- Definition: A dihybrid cross is a genetic experiment involving the simultaneous study of two different traits or characteristics in an organism’s inheritance. It helps determine how genes for these traits segregate and assort independently during gamete formation and fertilization.
- Mendel’s Law of Independent Assortment: Dihybrid crosses provide experimental evidence for Gregor Mendel’s Law of Independent Assortment, which states that alleles for different traits segregate independently during gamete formation, assuming the genes are located on different chromosomes.
- Genotype Representation: In dihybrid crosses, alleles for each trait are often designated with specific letters, typically using uppercase letters for dominant alleles and lowercase letters for recessive alleles. For example, in a cross involving seed shape and seed color in pea plants, “R” might represent the dominant allele for round seeds, while “r” represents the recessive allele for wrinkled seeds.
- Gamete Formation: Dihybrid crosses involve determining the possible combinations of alleles in the gametes produced by the parents. These gametes combine randomly during fertilization to produce offspring with different genotypes.
- Punnett Square: A Punnett square is a commonly used tool in dihybrid crosses to predict the genotypic and phenotypic ratios of offspring. It organizes the possible combinations of alleles from both parents and allows for easy visualization of potential outcomes.
- Phenotypic Ratios: The phenotypic ratios in the offspring of a dihybrid cross often follow the classic 9:3:3:1 ratio. This ratio represents the relative frequencies of different phenotypes among the offspring, taking into account the combinations of alleles for both traits.
- Genetic Mapping: Dihybrid crosses can be used to map the relative positions of genes on the same chromosome. When genes are linked (located close together), they tend to be inherited together more frequently, leading to deviations from the expected phenotypic ratios.
- Real-World Application: Dihybrid crosses model genetic scenarios that are more realistic, as most organisms inherit multiple traits simultaneously. Understanding how these traits are inherited together is essential in fields such as agriculture, genetics research, and medical genetics.
- Educational Tool: Dihybrid crosses are a fundamental component of genetics education. They provide students with hands-on experience in applying Mendelian principles, making predictions, and calculating expected ratios, helping build a strong foundation in genetics.
- Basis for More Complex Studies: Dihybrid crosses serve as a starting point for studying more complex genetic scenarios, including trihybrid crosses and cases involving gene interactions and epistasis. They are essential for unraveling the intricacies of genetic inheritance.
What is Dihybrid Cross?
- A dihybrid cross is a fundamental concept in genetics, which involves the mating or crossing of two individuals that have different genetic characteristics for two specific traits. In other words, it’s an experiment designed to produce offspring that are identical hybrids for two distinct qualities. This type of genetic cross is crucial in understanding how genes control multiple traits and how they segregate during inheritance.
- Genes, the segments of DNA responsible for controlling various traits, come in pairs, with one allele inherited from each parent. When we talk about a dihybrid cross, it means that both parents have two different pairs of alleles for two separate traits. One parent carries a homozygous dominant allele for one trait, while the other parent carries a homozygous recessive allele for the same trait. This genetic setup sets the stage for examining the inheritance of multiple traits simultaneously.
- Dihybrid crosses are more complex than monohybrid crosses, which involve only one genetic trait. In monohybrid crosses, we typically focus on the inheritance of a single trait, while in dihybrid crosses, we consider two traits at the same time.
- One of the main purposes of conducting a dihybrid cross is to demonstrate or validate the Law of Independent Assortment proposed by Gregor Mendel, the father of modern genetics. This law states that different pairs of alleles segregate, or assort, independently of one another during gamete formation. However, this principle holds true only for genes located on different chromosomes and are not linked. When genes are linked, they tend to be inherited together and do not assort independently.
- During a dihybrid cross, there is a higher number of possible combinations of alleles in the gametes compared to a monohybrid cross. This is because there are two separate genes under consideration, each with its set of alleles. The increased genetic diversity in the gametes results in a larger number of potential offspring genotypes.
- Additionally, the offspring obtained from a dihybrid cross exhibit a greater range of phenotypic variations than those from monohybrid crosses. This diversity in phenotypes is a direct consequence of the combinations of alleles inherited from the parents.
- In terms of expressing the phenotypic ratios of the offspring in the first generation after a dihybrid cross, it is typically represented as 9:3:3:1. This ratio reflects the different possible combinations of alleles for the two traits. However, the genotypic ratios are even more diverse, with a ratio of 1:2:2:4:1:2:1:2:1, accounting for all the possible genetic combinations.
- In summary, a dihybrid cross is a genetic experiment that involves the mating of two individuals with different genetic characteristics for two distinct traits. It demonstrates the inheritance patterns of multiple traits simultaneously, confirming the Law of Independent Assortment for unlinked genes on different chromosomes. Dihybrid crosses result in a higher number of possible gamete combinations, greater phenotypic variation among offspring, and complex genotypic ratios, making them a valuable tool in understanding genetic inheritance.
Definition of Dihybrid Cross
A dihybrid cross is a genetic experiment involving the mating of individuals with differing genetic characteristics for two distinct traits, demonstrating how genes for multiple traits segregate and assort independently during inheritance.
Steps/Process of Dihybrid Cross
A dihybrid cross is a crucial genetic experiment that helps us understand how genes for two different traits segregate and assort independently during inheritance. To perform a dihybrid cross effectively, one must follow a series of structured steps. Here is an outline of the process:
- Selection of Parents:
- The first step in conducting a dihybrid cross is selecting the two individuals that will be used as parents. These individuals should have known genetic characteristics for the two traits you wish to study.
- It is essential to choose individuals from pure lines. To ensure this, selfing, or self-fertilization, of the selected individuals for at least three generations can be performed. This process helps confirm that the chosen parents carry only homozygous alleles for the traits of interest.
- Designation of Alleles:
- Once the parents are selected, the alleles responsible for the two traits under investigation need to be designated with specific alphabet characters.
- Typically, uppercase letters are used to represent dominant alleles, while lowercase letters represent recessive alleles for each trait. Assigning these symbols helps in keeping track of the alleles during the cross.
- Formation of Gametes:
- In a dihybrid cross, the focus is on the formation of gametes (sperm and egg cells) by the selected parents. Each parent will produce gametes with one allele for each of the two traits.
- As a result of meiotic division, each parent will produce four distinct haploid gametes, each carrying one allele for each trait.
- Construction of a Punnett Square:
- To visualize the possible outcomes of the dihybrid cross, a Punnett square is set up. The Punnett square is a grid that lists the phenotype and genotype of the parents and predicts the genotypes of their potential offspring.
- The Punnett square takes into account all possible combinations of alleles from the parents, and it is important to remember that the process of fertilization is random.
- Determination of Ratios:
- After completing the Punnett square, the next step is to determine the phenotypic and genotypic ratios of the offspring.
- By analyzing the combinations of alleles in the Punnett square, you can calculate the expected ratios of different phenotypes and genotypes among the offspring.
Examples of Dihybrid Cross
Dihybrid crosses are essential experiments in genetics that help us understand how two different traits are inherited together. Two classic examples of dihybrid crosses involve pea plants and Drosophila flies.
- Dihybrid Cross in Pea Plants:
- Parental Traits: In this example, we’ll consider two traits in pea plants: seed shape and seed color. We have a homozygous pea plant with round yellow seeds (RRYY) and another with wrinkled green seeds (rryy).
- Gamete Formation: The alleles for round yellow seeds (RRYY) will produce gametes with alleles RY, while the alleles for wrinkled green seeds (rryy) will produce gametes with alleles ry.
- F1 Generation: When these two homozygous parents are crossed, the F1 generation hybrids (RrYy) are produced. These hybrids exhibit round yellow seeds because the dominant R allele controls seed roundness, and the dominant Y allele determines the yellow color.
- Allelic Combinations: The alleles RY, Ry, rY, and ry can combine in four different ways due to independent assortment.
- F2 Generation: In the F2 generation, the gametes combine randomly during fertilization, resulting in sixteen different possible combinations of alleles.
- Phenotypic Ratios: The offspring in the F2 generation occur in the classic phenotypic ratio of 9 round yellow seeds, 3 round green seeds, 3 wrinkled yellow seeds, and 1 wrinkled green seed. This ratio is a result of the various combinations of the alleles R and r for seed shape and Y and y for seed color.
- Dihybrid Cross in Drosophila:
- Parental Traits: In this example, we examine two traits in Drosophila flies: wing length and body color. We have homozygous long-winged and black-bodied flies and homozygous vestigial-winged and grey-bodied flies.
- F1 Generation: When these two homozygous parental flies are crossed, the F1 generation hybrids are obtained. These hybrids have long wings and grey bodies.
- F2 Generation: The F1 generation hybrids are then crossed with each other to produce the F2 generation of flies.
- Phenotypic Ratios: The F2 generation exhibits the classic phenotypic ratio of 9 long-winged grey-bodied flies, 3 long-winged black-bodied flies, 3 vestigial-winged grey-bodied flies, and 1 vestigial-winged black-bodied fly. This ratio reflects the different combinations of alleles for wing length and body color.
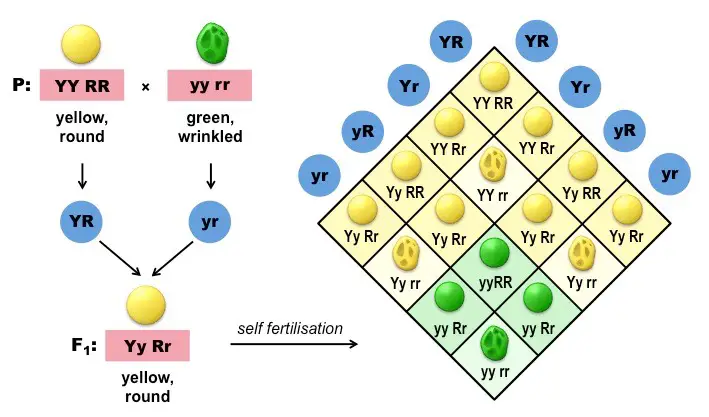
- Dihybrid Cross in Guinea Pigs:
- Parental Traits: Consider a dihybrid cross in guinea pigs involving fur color and fur texture. One parent has a homozygous dominant genotype for both traits (CCFF), resulting in a smooth-coated guinea pig with black fur. The other parent is homozygous recessive for both traits (ccff), leading to a long-haired guinea pig with white fur.
- Gamete Formation: The alleles for smooth coat and black fur (CCFF) will produce gametes with alleles CF, while the alleles for long hair and white fur (ccff) will produce gametes with alleles cf.
- F1 Generation: Crossing these two homozygous parents results in F1 generation hybrids (CcFf) with a smooth coat and black fur, as the dominant alleles for both traits prevail.
- F2 Generation: When the F1 generation guinea pigs are bred with each other, they produce an F2 generation with various combinations of alleles for fur color and texture.
- Phenotypic Ratios: The phenotypic ratio in the F2 generation typically follows a 9:3:3:1 ratio. This means 9 guinea pigs will have a smooth coat and black fur, 3 will have a smooth coat and white fur, 3 will have a long coat and black fur, and 1 will have a long coat and white fur.
- Dihybrid Cross in Corn Plants:
- Parental Traits: In corn plants, a dihybrid cross can be performed involving seed color and seed shape. One parent can be homozygous for round, yellow seeds (RRYY), and the other parent homozygous for wrinkled, green seeds (rryy).
- Gamete Formation: The alleles for round, yellow seeds (RRYY) will produce gametes with alleles RY, while the alleles for wrinkled, green seeds (rryy) will produce gametes with alleles ry.
- F1 Generation: Crossing these two homozygous parents results in F1 generation hybrids (RrYy) with round, yellow seeds due to the dominance of R and Y alleles.
- F2 Generation: Breeding the F1 generation plants with each other leads to the F2 generation, which exhibits various combinations of alleles for seed color and shape.
- Phenotypic Ratios: The phenotypic ratio in the F2 generation generally follows the 9:3:3:1 ratio, with 9 plants having round, yellow seeds, 3 having round, green seeds, 3 having wrinkled, yellow seeds, and 1 having wrinkled, green seeds.
Importance of Dihybrid Cross
- Demonstrating Independent Assortment:
- Dihybrid crosses provide experimental evidence for one of Mendel’s fundamental principles, the Law of Independent Assortment. This law states that alleles for different traits segregate independently of one another during gamete formation, assuming that the genes are located on different chromosomes. Dihybrid crosses confirm this principle by showing that alleles for one trait do not affect the inheritance of alleles for another trait.
- Real-World Application:
- Dihybrid crosses are more reflective of real-world genetic scenarios. In most organisms, multiple traits are controlled by different genes simultaneously. Understanding how genes for two or more traits are inherited together is essential for making predictions about the characteristics of offspring in complex genetic scenarios.
- Gene Mapping:
- Dihybrid crosses can help researchers map the relative positions of genes on the same chromosome. When two genes are located close to each other on a chromosome, they tend to be inherited together, violating the Law of Independent Assortment. By studying the frequency of recombinant offspring in dihybrid crosses, scientists can estimate the genetic distance between these genes.
- Genetic Variation:
- Dihybrid crosses illustrate the diversity of genetic combinations and phenotypic outcomes that can result from the assortment of alleles. This genetic variation is essential for the survival and evolution of populations, as it allows for adaptation to changing environments and the emergence of new traits.
- Predicting Inheritance Patterns:
- Dihybrid crosses enable the prediction of phenotypic ratios in offspring, helping geneticists and breeders make informed decisions about which traits are likely to be passed on to the next generation. This knowledge is valuable in agriculture, animal breeding, and medical genetics.
- Educational Tool:
- Dihybrid crosses are a fundamental part of genetics education. They provide students with hands-on experience in applying Mendelian principles, understanding allele interactions, and calculating expected ratios. This practical knowledge serves as a foundation for more complex genetic studies.
- Advancing Genetics Research:
- Dihybrid crosses are the building blocks for understanding more complex genetic scenarios, including trihybrid crosses and beyond. They form the basis for studying gene interactions, epistasis, and the inheritance of quantitative traits, contributing to advancements in genetics research.