What is Chromosome?
- A chromosome is a thread-like structure composed of DNA and proteins, housed within the nucleus of living cells. It plays a critical role in the storage and transmission of genetic information. Genes, which are specific sequences of DNA that encode for proteins, are located along the length of these chromosomes. The arrangement and organization of chromosomes ensure that genetic material is accurately replicated and passed on during cell division.
- The discovery of chromosomes dates back to the 19th century. Initially observed by Hofmeister in 1848, chromosomes were seen as filaments within the nucleus of plant cells. The term “chromosome,” derived from the Greek words chroma (color) and soma (body), was coined by Waldeyer in 1888, as chromosomes became easily visible when stained with certain dyes.
- In terms of structure, chromosomes can appear in various forms—oval, rod-shaped, or thread-like—depending on the stage of the cell cycle. They are composed primarily of DNA, tightly wound around proteins called histones, forming a complex known as chromatin. This arrangement allows the long strands of DNA to fit within the confines of the cell nucleus while also protecting it from damage.
- Functionally, chromosomes act as vehicles for heredity, carrying the genetic instructions needed for an organism’s development, growth, and reproduction. During cell division, they replicate to ensure that each new cell receives an exact copy of the genetic material. Furthermore, they play a key role in transcription, where segments of DNA are used to produce RNA, which in turn synthesizes proteins essential for cellular functions.
- Thus, chromosomes are not only fundamental to the structure of living organisms but are also integral to the proper functioning and inheritance of genetic traits.
What is Chromosomal Theory of Inheritance?
- The Chromosomal Theory of Inheritance is a foundational concept in genetics, proposing that chromosomes are the carriers of genes, and that their behavior during cell division explains the patterns of inheritance observed by Gregor Mendel. This theory was primarily developed by Theodor Boveri and Walter Sutton in the early 20th century, based on observations of chromosome behavior during meiosis.
- In 1902, Boveri demonstrated that normal embryonic development in sea urchins required the presence of a full set of chromosomes. That same year, Sutton observed that chromosomes segregate into daughter cells during meiosis, a process similar to the segregation of Mendelian traits. These findings led to the hypothesis that chromosomes, not just abstract factors, are responsible for the inheritance of traits.
- The Chromosomal Theory of Inheritance aligns with Mendel’s laws of segregation and independent assortment. During meiosis, homologous chromosomes pair up and migrate as distinct units, which are sorted randomly into gametes. This randomness mirrors Mendel’s principle of independent assortment, where different traits are inherited independently of one another. Furthermore, each parent produces gametes with half the chromosome number, ensuring that offspring inherit a full chromosome set upon fertilization, with equal genetic contributions from both parents.
- Though the theory closely matched Mendel’s laws, it initially faced criticism due to the fact that organisms seemed to have more traits than they had chromosomes. The theory gained further support when Thomas Hunt Morgan, through his experiments with fruit flies, provided direct evidence showing that specific traits were linked to specific chromosomes, thus confirming the relationship between genes and chromosomes.
Observations of Chromosomal Theory of Inheritance
The Chromosomal Theory of Inheritance provided a clear link between the behavior of chromosomes and Mendel’s laws of inheritance. It was supported by a series of critical observations that connected chromosomal behavior during meiosis with the inheritance patterns Mendel described. These key observations are:
- Migration of Homologous Chromosome Pairs
During meiosis, homologous chromosome pairs move as distinct, independent units. This migration ensures that each gamete receives one chromosome from each pair, supporting Mendel’s principle of segregation. - Random Sorting of Chromosomes
The distribution of chromosomes into pre-gametes is random. This randomness mirrors Mendel’s law of independent assortment, explaining why different traits can be inherited independently of one another. - Gamete Formation with Half the Chromosome Set
Both parents produce gametes containing only half the usual number of chromosomes (haploid). This reduction in chromosome number is essential for maintaining the correct chromosome count in the offspring after fertilization. - Equal Chromosome Contribution from Male and Female Gametes
Despite the structural differences between male (sperm) and female (egg) gametes, both types carry the same number of chromosomes. This observation supports the idea that each parent contributes equally to the genetic makeup of their offspring. - Restoration of Chromosome Number During Fertilization
Upon fertilization, the combination of male and female gametic chromosomes restores the diploid chromosome number in the offspring, ensuring continuity of genetic information across generations.
Historical Development of Chromosome Theory
The development of chromosome theory represents a critical moment in the understanding of genetic inheritance. Grounded in both cytological and experimental findings, this theory forms the basis for modern genetics. The progression of these discoveries unfolded through a series of important contributions made by scientists, each advancing our knowledge of how chromosomes carry and transfer hereditary information.
- Wilhelm Roux (1883)
- Roux was among the first to propose that chromosomes play a crucial role in heredity. He suggested that chromosomes within a cell are responsible for transferring hereditary information from one generation to the next.
- His work laid the foundation for understanding that heredity is rooted in cellular structures, particularly chromosomes.
- Montgomery (1901)
- Montgomery’s research focused on the structure of chromosomes. He was the first to recognize the existence of distinct pairs of chromosomes, an observation critical for the understanding of chromosomal behavior during reproduction.
- He further concluded that during meiosis, maternal and paternal chromosomes pair together. This discovery added clarity to the mechanisms behind genetic recombination and variability.
- Theodor Boveri (1902)
- Boveri made a significant contribution by supporting the notion that chromosomes contain the genetic determinants responsible for heredity. He played a leading role in shaping the chromosomal theory of inheritance.
- His research demonstrated that proper embryonic development depends on the correct number and type of chromosomes, emphasizing the functional role of chromosomes in inheritance.
- W.S. Sutton (1902)
- Sutton, an American student, independently recognized that chromosomes exhibit behavior similar to Mendelian factors during gamete formation. He observed how chromosomes segregate during meiosis, which mirrored the patterns described by Gregor Mendel in his experiments with pea plants.
- Alongside Boveri, Sutton proposed the chromosomal theory of inheritance in 1903. Sutton’s work was instrumental in uniting the knowledge of chromosomal segregation with Mendelian principles, creating a cohesive theory that explained the transmission of hereditary traits through chromosomes.
- Sutton and Boveri (1903)
- Together, Sutton and Boveri independently but concurrently proposed what is now known as the chromosome theory of inheritance. They posited that chromosomes are the carriers of genetic material and that they segregate independently during meiosis, which accounts for the patterns of inheritance observed by Mendel.
- This theory linked the physical behavior of chromosomes with Mendel’s laws of inheritance, providing a comprehensive explanation for how traits are passed down through generations.
How Chromosomal Theory of Inheritance supports Mendel’s law?
The Chromosomal Theory of Inheritance directly supports Mendel’s laws of inheritance by explaining the physical processes underlying the transmission of genetic traits. Several aspects of this theory align with Mendel’s principles, providing a clear connection between the behavior of chromosomes and the inheritance patterns described by Mendel. Key points of support include:
- Chromosomes Occur in Pairs
In somatic (body) cells, chromosomes exist in pairs—one inherited from the father and the other from the mother. This pairing forms homologous chromosomes, similar to Mendel’s concept of paired alleles for each trait. - Segregation of Homologous Chromosomes During Meiosis
During gametogenesis, homologous chromosome pairs segregate independently, ensuring that each gamete receives only one chromosome from each pair. This supports Mendel’s law of segregation, which states that allele pairs separate during the formation of gametes. - Random Sorting and Independent Assortment
The sorting of chromosomes into gametes is random, mirroring Mendel’s law of independent assortment. This means that the inheritance of one trait is not influenced by the inheritance of another, as chromosomes (and the genes they carry) assort independently. - Equal Contribution of Chromosomes from Both Parents
Both male and female gametes, despite differing in size and morphology, carry the same number of chromosomes. This observation aligns with Mendel’s conclusion that both parents contribute equally to the genetic makeup of their offspring. - Fixed Chromosome Number Across Generations
After fertilization, the gametes combine to restore the diploid chromosome number in the offspring. This ensures the continuity of genetic information and maintains a stable chromosome number, which is crucial for the inheritance of traits.
Morgan’s experiments on Dorsophila melanogaster
Thomas Hunt Morgan’s experiments on Drosophila melanogaster provided groundbreaking insights into the Chromosomal Theory of Inheritance and uncovered the concept of sex-linked inheritance. Conducted in 1910, Morgan’s work on the common fruit fly (Drosophila melanogaster) demonstrated how specific traits, like eye color, are linked to the X chromosome, thereby expanding Mendel’s principles and explaining inheritance patterns that Mendel’s laws alone could not account for. Key aspects of Morgan’s experiments include:
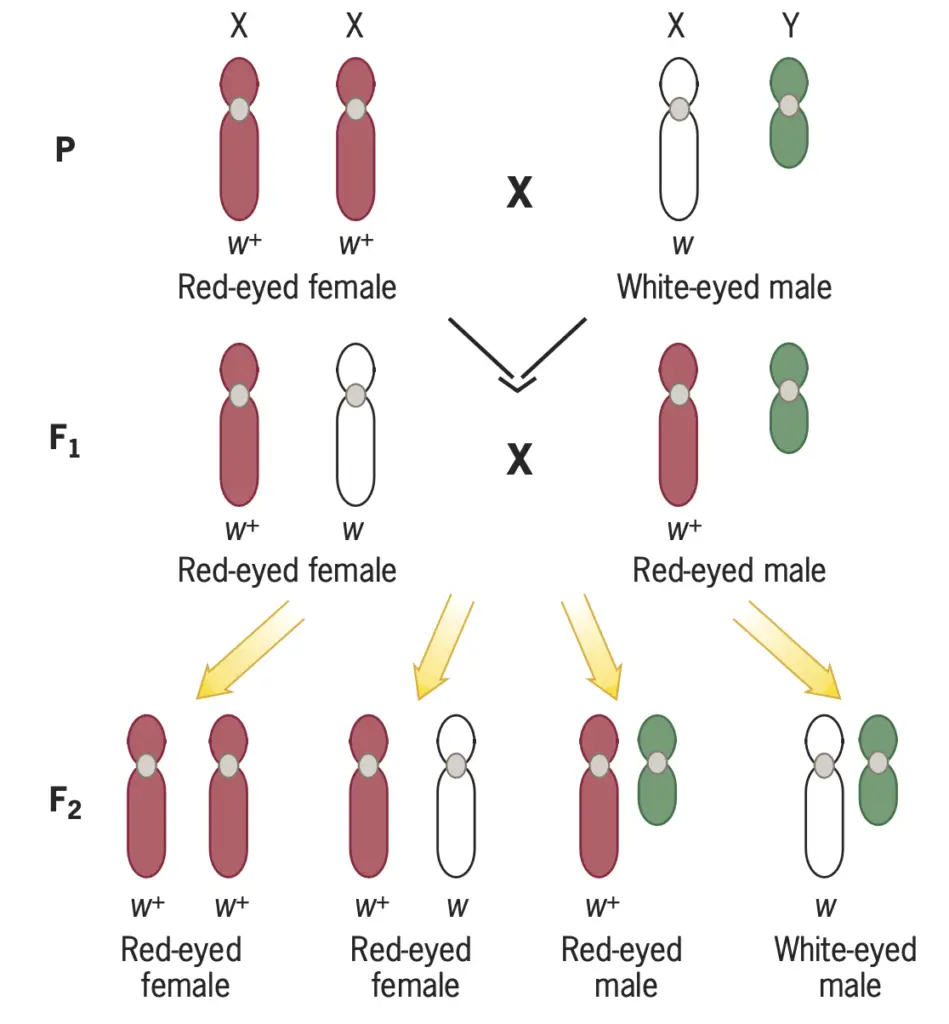
- Use of Drosophila melanogaster
Drosophila melanogaster, or the fruit fly, became an ideal model for genetic studies due to its simple chromosome structure. It has four pairs of chromosomes: three pairs of autosomes and one pair of sex chromosomes (X and Y). At the time of Morgan’s experiments, it was known that X and Y chromosomes were related to determining the sex of an organism. - Initial Cross: Red-Eyed Female × White-Eyed Male
In Drosophila, normal flies have red eyes, while mutated flies have white eyes. Morgan crossed a red-eyed female (Xw+ Xw+) with a white-eyed male (Xw Y). In the F1 generation, all offspring had red eyes. This outcome was consistent with the dominant-recessive inheritance pattern, as red eyes are dominant (Xw+) over white eyes (Xw), following Mendel’s principles of dominance. - Reciprocal Cross: Red-Eyed Male × White-Eyed Female
In a reciprocal cross, Morgan mated a red-eyed male (Xw+ Y) with a white-eyed female (Xw Xw). This time, the F1 generation produced surprising results: all female progeny had red eyes, while all male progeny had white eyes. This outcome deviated from Mendel’s law of independent assortment, suggesting a link between eye color and the sex of the offspring. - Explanation of Sex-Linked Inheritance
Morgan explained these results by suggesting that the gene responsible for eye color was located on the X chromosome, and that eye color was a sex-linked trait. Since males have only one X chromosome, they are hemizygous for X-linked traits, meaning they only possess one allele for any gene on the X chromosome. Therefore, if a male inherits a recessive allele (Xw) for white eyes, he will exhibit the trait, as there is no dominant allele on a second X chromosome to mask it. - Support for the Chromosomal Theory of Inheritance
Morgan’s experiments provided direct evidence supporting the Chromosomal Theory of Inheritance. His findings demonstrated that genes are located on chromosomes, and that the behavior of chromosomes during meiosis can explain how traits are inherited. The linkage between eye color and the X chromosome also revealed the concept of gene linkage, further explaining deviations from Mendel’s law of independent assortment. - X-Linked Trait Identification
Morgan’s discovery that the gene for eye color in Drosophila was located on the X chromosome marked the first identification of an X-linked trait. This experiment established the foundational principles of X-linked inheritance and helped explain why certain traits, like eye color in Drosophila, did not follow Mendelian inheritance patterns in every case.
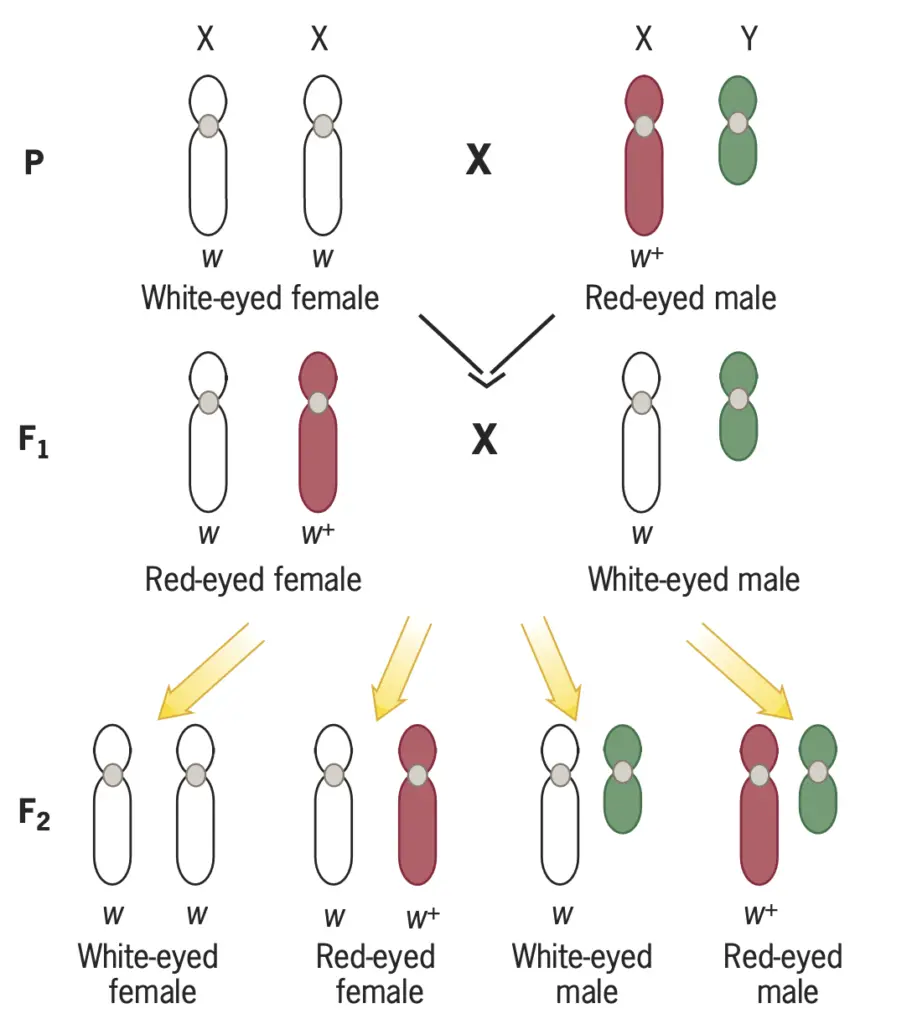
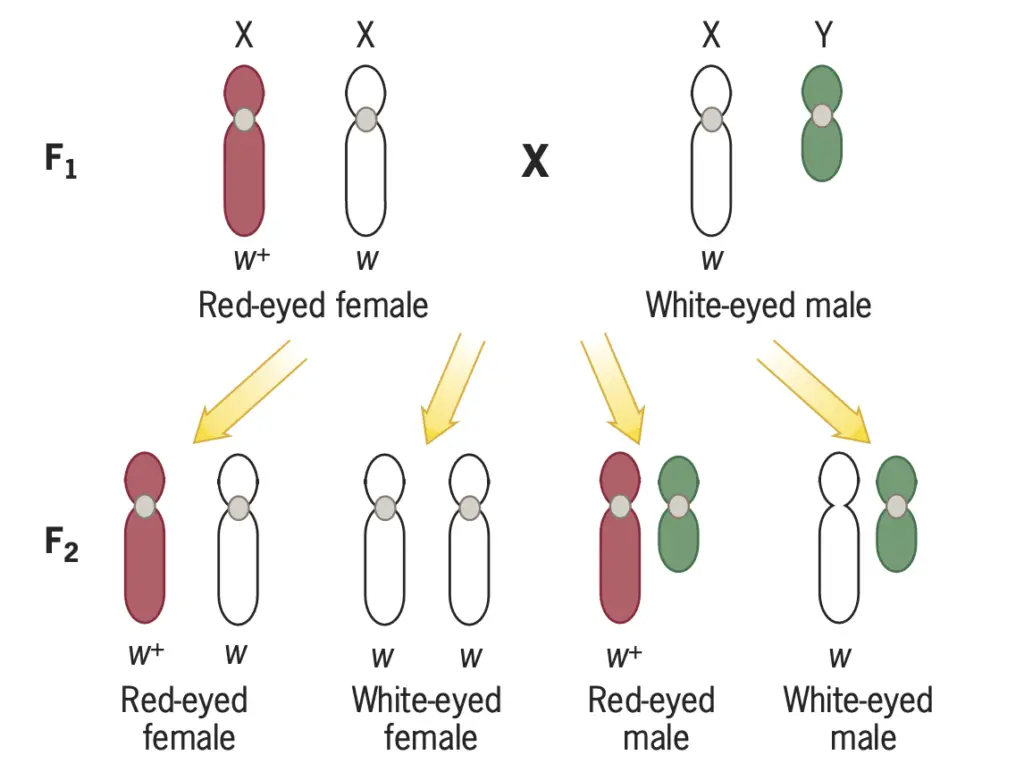
Through these experiments, Morgan not only confirmed that genes are carried on chromosomes, but also introduced the concept of sex-linked traits, explaining variations in inheritance that could not be accounted for by Mendel’s laws alone. His work was pivotal in advancing the understanding of genetic inheritance and solidifying the Chromosomal Theory of Inheritance.
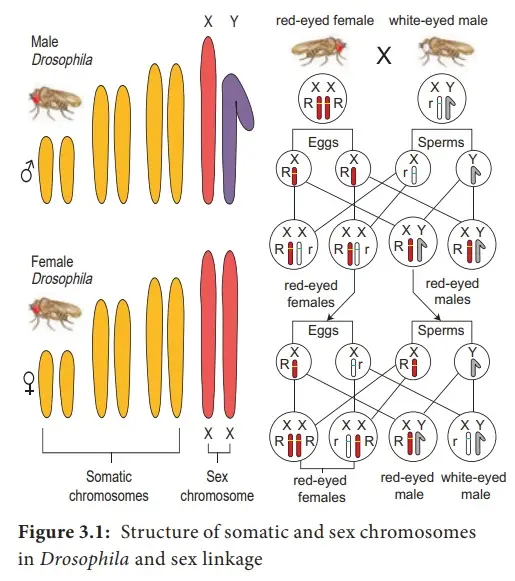
NON disjunction as proof of chromosomal theory of Inheritance
The concept of nondisjunction is fundamental to understanding the chromosomal theory of inheritance, which was supported through various genetic experiments. One of the most prominent contributions in this regard comes from Calvin Bridges, a student of Thomas Hunt Morgan, who provided concrete evidence for the theory by demonstrating how chromosome behavior can explain exceptions in inheritance patterns.
Key Experiment by Calvin Bridges
Bridges extended one of Morgan’s classic experiments involving Drosophila melanogaster (fruit flies), focusing on the inheritance of eye color. The gene for eye color was known to be located on the X chromosome, a critical piece of evidence linking specific traits to specific chromosomes. Bridges carried out a large-scale version of the experiment to observe genetic inheritance and any deviations from the expected patterns.
- Initial Cross: White-Eyed Female × Red-Eyed Male
- In this cross, Bridges started with white-eyed females (Xw Xw) and red-eyed males (Xw+ Y).
- According to normal inheritance patterns, the F1 generation should primarily consist of red-eyed females and white-eyed males.
- F1 Generation Results
- Most of the F1 progeny followed this expected pattern, with red-eyed females (Xw+ Xw) and white-eyed males (Xw Y).
- However, a small number of exceptional progeny were observed, including white-eyed females and red-eyed males. These exceptions did not align with traditional Mendelian inheritance.
- Further Crosses: Red-Eyed Males × White-Eyed Females
- Bridges continued his investigation by crossing the exceptional F1 red-eyed males with normal white-eyed females.
- Interestingly, the red-eyed males were sterile, failing to produce offspring.
- In contrast, the white-eyed females were fertile. When crossed with normal red-eyed males, these white-eyed females produced a substantial number of F2 progeny, including white-eyed females and red-eyed males.
- Nondisjunction as the Explanation
- The key to understanding the exceptional offspring lies in the phenomenon of nondisjunction—an error in cell division where homologous chromosomes fail to separate during meiosis.
- In this case, nondisjunction of the X chromosomes during the formation of gametes resulted in some offspring receiving two X chromosomes or none at all. This explained why certain progeny exhibited unexpected phenotypes, such as the white-eyed females and red-eyed males in the F1 and F2 generations.
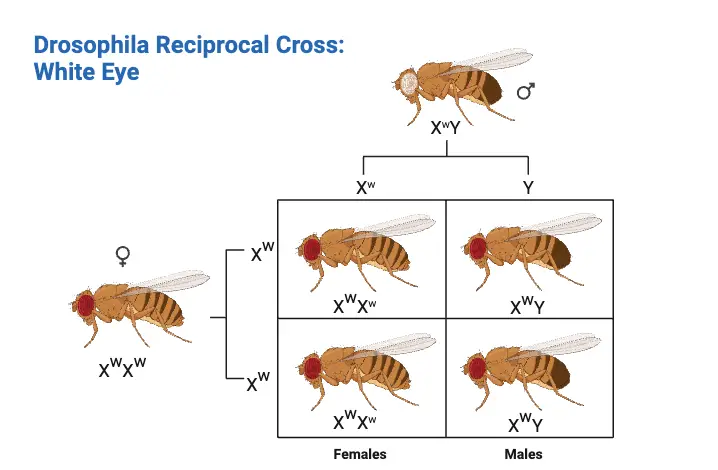
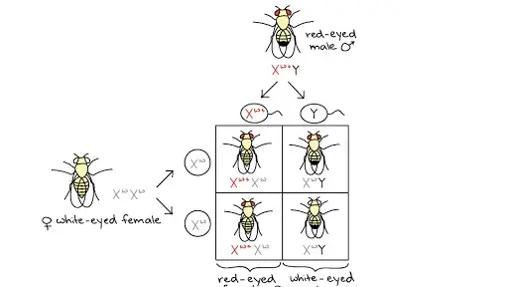
Explanation of Results in Bridges’ Experiment on Nondisjunction
Calvin Bridges’ experiment provided crucial insights into chromosomal inheritance, particularly through the phenomenon of nondisjunction. This term refers to the failure of homologous chromosomes to separate properly during meiosis, leading to gametes with abnormal chromosome numbers. His explanation of the results in Drosophila melanogaster highlighted how this error could lead to unexpected phenotypes, supporting the chromosomal theory of inheritance.
Abnormal X Chromosome Behavior
Bridges observed that the exceptional F1 progeny, including white-eyed females and red-eyed males, were a result of abnormal X chromosome segregation during meiosis in the parental (P) generation. Normally, during gametogenesis, the X chromosomes in females segregate evenly, but in some cases, they fail to disjoin, resulting in two possible errors:
- Diplo-X Gametes: Eggs with two X chromosomes.
- Nullo-X Gametes: Eggs with no X chromosomes.
Fertilization of Abnormal Gametes
When these abnormal gametes are fertilized by normal sperm, zygotes with irregular numbers of sex chromosomes are formed. The combination of these abnormal eggs with the sperm determines the phenotype of the resulting offspring.
- XwXwY (White-Eyed Females):
If an egg containing two X chromosomes (XwXw) is fertilized by a normal sperm carrying the Y chromosome, the zygote will have an XXY configuration (XwXwY). Since both X chromosomes carry the recessive w (white-eye) allele, the resulting fly will be female with white eyes. - Xw+O (Red-Eyed Males):
If an egg without an X chromosome (denoted as O) is fertilized by a sperm carrying the normal red-eye allele (Xw+), the zygote will have the XO configuration (Xw+O). Despite having only one X chromosome, this zygote will develop into a male with red eyes, since the Xw+ allele is dominant over the mutant w allele.
Sex Determination in Drosophila
Bridges’ findings also contributed to the understanding of sex determination in Drosophila. Through cytological examination, he confirmed that:
- XXY Flies are female.
- XO Flies are male.
This led to the conclusion that the Y chromosome in Drosophila is not involved in determining the organism’s sexual phenotype. Instead, the presence of two X chromosomes results in a female, while one X chromosome (even without a Y) results in a male. However, the Y chromosome was found to be essential for male fertility, as XO males were sterile, suggesting its role in male sexual function, though not in sex determination.
Confirmation of Chromosomal Constitutions
Bridges was able to confirm these observations through direct cytological analysis, observing the chromosomal makeup of the exceptional flies under a microscope. This confirmed that the white-eyed females were indeed XwXwY, while the red-eyed males were Xw+O.
Key Causes of Nondisjunction
- Faulty Chromosome Movement
One primary cause of nondisjunction is the improper movement of chromosomes during meiosis. Chromosomes must align correctly at the metaphase plate and move toward opposite poles during anaphase. Any disruption in this process can result in one cell receiving too many chromosomes while the other receives too few. In Bridges’ experiment with Drosophila, this abnormal movement often involved the X chromosome, leading to unexpected phenotypes such as XXY females and XO males. - Imprecise or Incomplete Homologous Pairing
For chromosomes to segregate correctly, homologous chromosomes need to pair precisely during prophase I of meiosis. If homologous chromosomes do not align properly, this imprecise pairing can lead to errors in segregation. As observed in Bridges’ study, the nondisjunction of sex chromosomes in females resulted in the formation of abnormal eggs with two X chromosomes (diplo-X) or none at all (nullo-X). - Centromere Malfunction
The centromere plays a critical role in ensuring proper chromosome segregation by attaching to spindle fibers during meiosis. A malfunctioning centromere can result in one chromosome not attaching properly, leading to nondisjunction. In the case of Bridges’ exceptional progeny, the improper attachment of sex chromosomes during meiosis contributed to the abnormal outcomes seen in the F2 generation.
Mechanisms of Nondisjunction in XXY Flies
From Bridges’ experiments, the F2 generation of XXY females produced a high frequency of exceptional offspring. This pattern suggests that nondisjunction was persistent, affecting successive generations. Nondisjunction of sex chromosomes in XXY females can occur in two main ways:
- Disjunction of X Chromosomes from Each Other:
In this scenario, the two X chromosomes may segregate, forming one gamete with a single X chromosome and another gamete with both the X and Y chromosomes (X and XY). - Disjunction of X from Y Chromosome:
Alternatively, the X chromosome may disjoin from the Y chromosome to form either XX and Y. This leads to the production of abnormal eggs, including diplo-X or nullo-X eggs. In the absence of proper disjunction, the X chromosome not segregating with the Y chromosome can migrate to either pole during the first meiotic division, producing exceptional zygotes when fertilized by normal sperm.
How Nondisjunction Supports the Chromosomal Theory of Inheritance
Nondisjunction served as a critical piece of evidence in favor of the chromosomal theory of inheritance. The theory posits that genes are located on chromosomes, and the inheritance of traits follows the behavior of these chromosomes during meiosis and fertilization. Bridges’ observations demonstrated that exceptions to normal inheritance patterns could be directly linked to chromosomal behavior, thus providing proof that chromosomes are indeed the carriers of genetic information.
- Chromosomes as Gene Carriers: The results of Bridges’ experiment showed that the location of the eye color gene on the X chromosome determined the inheritance pattern. The presence of exceptional progeny due to nondisjunction illustrated that specific traits are tied to the behavior of specific chromosomes.
- Exceptions in Inheritance: Nondisjunction explained the presence of offspring that deviated from the standard Mendelian ratios. Such exceptions would not be possible if traits were inherited solely according to the principles of dominant and recessive alleles without the influence of chromosome behavior.
- Further Validation: The fact that exceptional progeny continued to produce exceptional offspring in successive generations reinforced the idea that chromosome behavior is central to inheritance. The consistent patterns observed through multiple generations further solidified the connection between chromosomes and genetic traits.
Salient features of the Chromosomal theory of inheritance
Below are the salient features of this theory:
- Somatic Cell Composition
- The somatic cells of organisms, which make up most of the body’s tissues, are derived from the zygote through repeated cell divisions known as mitosis.
- Each somatic cell contains two identical sets of chromosomes: one inherited from the female parent (maternal chromosomes) and the other from the male parent (paternal chromosomes). These sets of chromosomes form homologous pairs.
- Chromosomal Structure and Uniqueness
- Chromosomes retain their structural uniqueness and individuality throughout the organism’s life cycle.
- This structural integrity ensures that each chromosome carries specific hereditary information consistently over generations.
- Genes as Determiners of Traits
- Each chromosome carries specific determiners, originally termed “Mendelian factors,” which are now referred to as genes.
- These genes are responsible for the transmission of traits and the regulation of various biological processes. They occupy specific locations (loci) on the chromosomes.
- Chromosome Behavior in Gamete Formation
- During the formation of gametes (eggs and sperm) through meiosis, chromosomes exhibit specific patterns of behavior, including segregation and independent assortment.
- This process provides clear evidence that genes are located on chromosomes, and it explains how hereditary factors are passed from parents to offspring during sexual reproduction.
- Homologous Chromosomes
- The chromosomes in a homologous pair (one maternal and one paternal) contain corresponding genes, although these genes may have different forms (alleles).
- The pairing and subsequent separation of homologous chromosomes during meiosis allow for genetic variation among offspring, contributing to Mendelian inheritance patterns.
- Evidence from Chromosome Segregation
- The segregation of chromosomes during meiosis parallels Mendel’s law of segregation, where paired alleles are separated into different gametes.
- This direct correlation between chromosome behavior and Mendel’s laws further solidifies the role of chromosomes in inheritance.
- https://www.brainkart.com/article/Chromosomal-Theory-of-Inheritance_38220/
- https://pressbooks-dev.oer.hawaii.edu/biology/chapter/chromosomal-theory-and-genetic-linkage/#:~:text=Section%20Summary,assortment%2C%20and%20occasionally%2C%20linkage.
- https://bioprinciples.biosci.gatech.edu/module-4-genes-and-genomes/4-4-linkage-sex-linkage-and-pedigree-analysis/
- https://www.pw.live/chapter-chromosomal-basis-of%20-inheritance-class-12/chromosomal-theory-of%20-inheritance
- https://bioprinciples.biosci.gatech.edu/module-4-genes-and-genomes/4-4-linkage-sex-linkage-and-pedigree-analysis/
- https://www.bankofbiology.com/2020/07/principles-of-inheritance-and-variation_89.html
- https://collegedunia.com/exams/chromosomal-theory-of-inheritance-biology-articleid-224
- https://plantlet.org/linkage-and-recombination-part-1-chromosomal-theory-linkage-crossing-over/