What is a Checkpoint?
- Checkpoints are critical regulatory mechanisms within the eukaryotic cell cycle that ensure the accurate and timely progression of cellular events. The concept of checkpoints was introduced by researchers Hartwell and Weinert in 1989, who identified specific pathways that halt the cell cycle in response to incomplete processes or detrimental conditions. This groundbreaking work was primarily conducted through studies on radiation-sensitive mutants of Saccharomyces cerevisiae, or budding yeast.
- The fundamental role of checkpoints is to monitor and assess the completion of various cell cycle events, sending inhibitory signals when conditions are unfavorable. For instance, when wild-type yeast cells are exposed to DNA-damaging radiation, they pause their division to allow for DNA repair. Conversely, mutations in the RAD9 gene compromise this checkpoint function, enabling damaged cells to continue dividing, often leading to cell death. This highlights that while Rad9 is not crucial for the actual repair of DNA, it is essential for the checkpoint mechanism that prevents division until the damage is rectified.
- In the context of the eukaryotic cell cycle, three main checkpoints have been identified: the G1/S checkpoint, the G2/M checkpoint, and the metaphase/anaphase checkpoint. Each of these checkpoints serves as a critical juncture where the cell assesses its readiness to proceed to the next stage of division. If the conditions for successful division—such as adequate cell size, proper nutrient availability, and genomic integrity—are not met, the cell cycle can be temporarily halted.
- To comprehend how these checkpoints function, it is important to identify the components that act as ‘accelerators’ and ‘brakes’ within the cell cycle machinery. The cell cycle is driven by a series of signals that promote progression, while checkpoint pathways function as surveillance mechanisms that detect and respond to unfavorable conditions. When an issue is identified, these checkpoints initiate a signaling cascade that effectively ‘brakes’ the cell cycle engine, allowing time for the necessary repairs or adjustments.
- Traditionally, the term ‘checkpoint’ encompasses both the paused state of the cell cycle and the signaling pathways that induce this arrest. The significance of checkpoint pathways cannot be overstated; they are vital for maintaining cellular integrity, especially under stress or adverse conditions where the ability to proliferate is compromised. Despite species-specific variations, the molecular mechanisms governing cell cycle control are highly conserved across eukaryotes, underscoring the evolutionary importance of these processes.
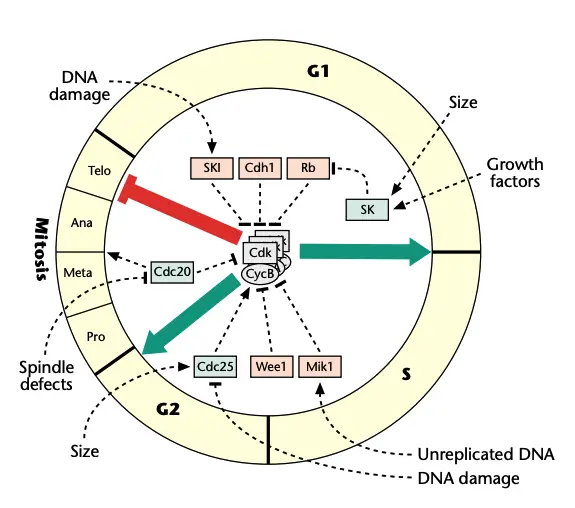
The Cell Cycle Engine
The cell cycle engine is a complex regulatory system that orchestrates the processes of cell growth and division in eukaryotic cells. This engine primarily consists of cyclin-dependent protein kinases (Cdks) and their regulatory subunits known as cyclins. These components play a pivotal role in controlling the progression through the various phases of the cell cycle, ensuring proper cellular function and genomic integrity.
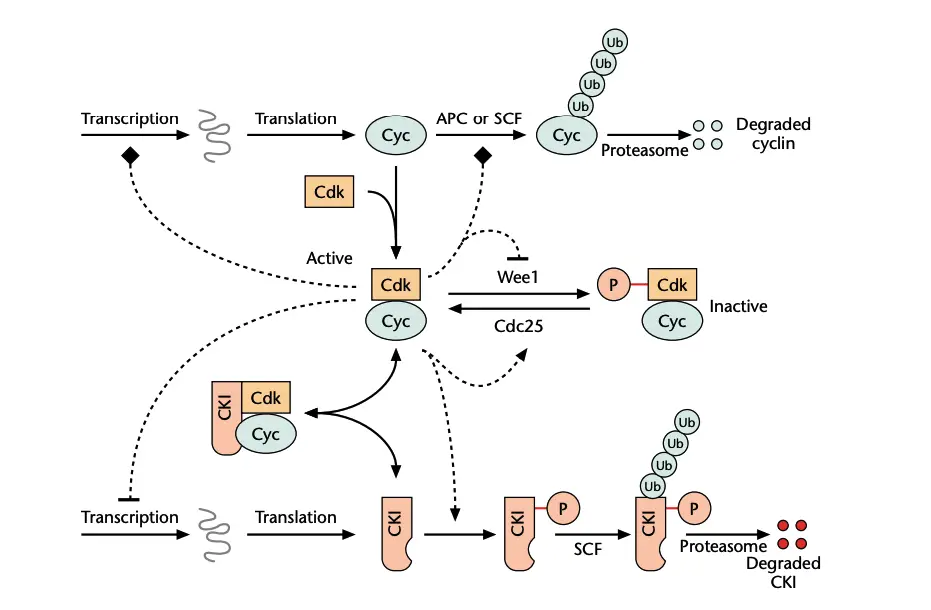
- Core Components: The interaction between Cdks and cyclins is highly conserved across species, although higher eukaryotes exhibit a greater diversity of Cdk forms compared to lower eukaryotes. For instance, budding yeast (Saccharomyces cerevisiae) possesses a single essential Cdk (Cdc28), while mammals have multiple forms (Cdk1–Cdk6). Similarly, cyclins differ in types, with yeasts using Clb-type and Cln-type cyclins, whereas metazoans utilize A, B, C, D, and E types.
- Cyclin–Cdk Complex Classes: Cyclin–Cdk complexes can be categorized into three classes based on their specific functions in the cell cycle:
- G1 Cyclin–Cdk Complexes: Crucial for advancing through the G1 phase and committing the cell to enter the S phase.
- S Cyclin–Cdk Complexes: Responsible for initiating and completing DNA replication, ensuring that genetic material is accurately copied.
- M Cyclin–Cdk Complexes: Drive the cell into mitosis while preventing premature re-entry into the G1 phase.
- Regulation of Cdk Activity: The activity of Cdks is regulated through several mechanisms rather than fluctuations in their expression levels. The key regulatory processes include:
- Cyclin Availability: The presence of cyclins is essential for Cdk activation. Cyclin levels are modulated through transcriptional regulation and specific proteolysis, with cyclins being marked for degradation by ubiquitin through the SCF and APC pathways. The SCF complex recognizes phosphorylated cyclins, while the APC ubiquitinates them when specificity factors become active.
- Stoichiometric Inhibition: Cyclin–Cdk complexes can be inactivated by cyclin kinase inhibitors (CKIs), which bind to the complexes to form inactive trimers. Lower eukaryotes have a single inhibitor for CycB–Cdk1, whereas higher eukaryotes employ multiple inhibitors from the INK4a and Cip1 families.
- Inhibitory Phosphorylation: Additional inactivation occurs via phosphorylation of specific residues near the Cdk active site, mediated by Wee1 kinases. Cdc25 phosphatases remove these inhibitory phosphate groups, thereby restoring Cdk activity.
- Feedback Mechanisms: The regulatory processes governing the cyclin–Cdk interactions create feedback loops that enhance the overall efficiency of the cell cycle engine. Active Cdks phosphorylate transcription factors that regulate cyclin and CKI genes, as well as components of the APC. A positive feedback loop is evident when Cdks activate Cdc25 and inhibit Wee1, thus promoting their own activity. Conversely, a negative feedback loop occurs when CycB–Cdk1 activates APC complexes that degrade the CycB subunit.
- Stable States and Transitions: These feedback loops facilitate the establishment of three distinct stable states that correspond to the major phases of the cell cycle: G1, S-G2, and M. The transitions between these states (G1 to S-G2 to M and back to G1) are irreversible, ensuring that the cell cycle proceeds in a regulated manner.
- Role of Checkpoints: Checkpoints serve as critical surveillance mechanisms that relay intracellular and extracellular signals to the cell cycle engine. They stabilize a particular phase of the cell cycle and prevent or delay transitions to subsequent phases when conditions are not favorable. This function is vital for maintaining cellular health and genomic integrity, particularly under stress or damage.
G1 Checkpoints
G1 checkpoints are critical regulatory mechanisms that ensure cells assess their environment and internal state before committing to division. This phase of the cell cycle includes crucial assessments of nutrient availability, growth factor presence, and genomic integrity. In eukaryotic organisms, the control points during G1 are designated as “Start” in yeast and the “restriction point” in mammals.
- Start in Budding Yeast:
- Defined by Hartwell et al. in 1974, Start represents the earliest genetically controlled step in the cell cycle. Cells in nutrient-poor conditions or exposed to mating pheromones will arrest at this checkpoint, preventing them from entering the next cell cycle until conditions improve.
- The CDC28 gene product, identified as the essential cyclin-dependent kinase (Cdk) Cdc28, is vital for the progression past Start. Budding yeast has three unstable G1 cyclins (Cln1, Cln2, Cln3) that form complexes with Cdc28, thereby regulating the Start checkpoint.
- The sequence of activation begins with Cln3–Cdc28, which triggers the synthesis of Cln1 and Cln2. Subsequently, Cln1–Cdc28 and Cln2–Cdc28 complexes promote the appearance of B-type cyclins (Clb1–6), ultimately driving DNA synthesis and mitosis.
- In conditions of nutrient starvation or pheromone exposure, Cln3–Cdc28 activity diminishes, inhibiting Cln1 and Cln2 transcription. Starvation reduces Cln3 protein synthesis, while pheromones activate the expression of a cyclin kinase inhibitor (CKI), Far1, which inhibits Cln1–3–Cdc28 complexes. Thus, checkpoint signals at Start function primarily by blocking G1 cyclin–Cdk complex activation.
- Restriction Point in Mammals:
- The restriction point marks a critical juncture in G1, after which mammalian cells can proceed with the cell division cycle even in the absence of growth factors. This point typically occurs 3-4 hours after mitosis.
- Research by Zetterberg and Larsson in 1985 revealed that if cells are deprived of growth factors or exposed to substances like cycloheximide (which reduces protein synthesis), they can experience delays in division. Specifically, this delay is about eight hours after reintroducing growth factors.
- The decline in cyclin D, a G1 cyclin, due to its instability and lack of synthesis during growth factor deprivation, is central to this delay. Cyclin D plays a pivotal role in interacting with the retinoblastoma protein (Rb), which inhibits cell proliferation by inactivating E2F transcription factors necessary for cyclins A and E expression.
- Cyclin D-dependent kinases phosphorylate Rb, thereby removing the inhibition on E2F and promoting cell growth and division.
- Irreversible Transitions:
- Both Start in budding yeast and the restriction point in mammals represent irreversible transitions in the cell cycle, facilitated by positive feedback loops in the Cdk control system. In mammals, G1 phase stability arises from three mechanisms that keep S and M cyclin–Cdks inactive: transcription of cyclin genes is repressed, rapid degradation of cyclins occurs, and high levels of stoichiometric inhibitors (e.g., p27Kip1) are present.
- As long as these antagonists are active, S and M cyclin–Cdk activities remain low. However, G1 cyclin–Cdk complexes, particularly CycD–Cdk4,6, initiate the shift towards S/G2/M states by phosphorylating Rb and sequestering p27Kip1, thus leading to an irreversible commitment to DNA replication and cell division.
- Response to DNA Damage:
- The G1 checkpoint also serves to monitor for DNA damage. When DNA integrity is compromised, a signaling network halts the cell cycle by inactivating Cdks. In budding yeast, DNA damage activates Rad53, which inhibits the transcription factor Swi4–Swi6, preventing progression past Start.
- In mammalian cells, ionizing radiation induces double-stranded breaks that activate ATM (ataxia-telangiectasia mutated) kinase. ATM phosphorylates various substrates involved in DNA repair, apoptosis, and cell cycle arrest.
- Specifically, ATM phosphorylates MDM2, leading to the accumulation of the p53 protein, which promotes transcription of p21Cip1, a CKI that inhibits cyclin–Cdk complexes. Additionally, p53 stimulates genes that facilitate DNA repair and can trigger apoptosis if damage is irreparable.
- ATM also activates the Chk1 and Chk2 kinases, which further stabilize p53 and phosphorylate Cdc25, a phosphatase that activates cyclin–Cdk complexes. The degradation of Cdc25A enforces G1 arrest, thereby allowing time for DNA repair processes to occur.
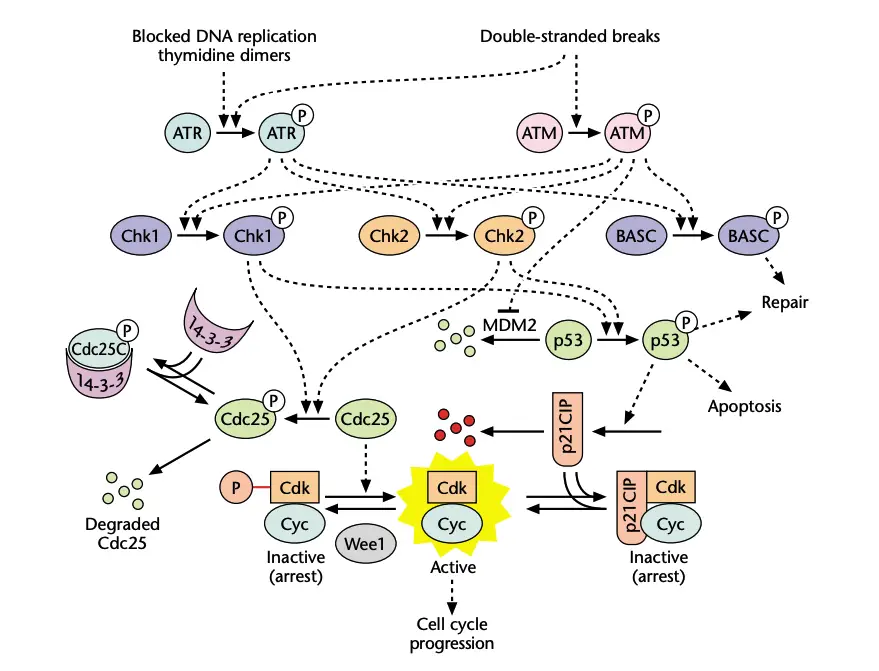
G2 Checkpoints
G2 checkpoints serve as critical regulatory mechanisms that ensure cells do not enter mitosis until they have successfully completed DNA replication and resolved any potential damage. These checkpoints are vital for maintaining genomic integrity and preventing the propagation of errors during cell division. In both fission yeast and mammalian cells, the regulation of the G2 to M phase transition is primarily controlled by cyclin B-dependent kinase activity, also referred to as M phase-promoting factor (MPF).
- M Phase-Promoting Factor (MPF):
- MPF is composed of cyclin B and its associated kinase, Cdk1. Before cells proceed to mitosis, they must verify that their DNA is fully replicated and free from damage.
- Cells that detect incomplete DNA replication or damage are delayed in the S–G2 phase due to the action of the kinase Wee1, which phosphorylates tyrosine and threonine residues on the catalytic subunit of MPF, rendering it inactive as a dimer known as preMPF.
- The transition from G2 to M phase occurs rapidly through the conversion of preMPF into active MPF, a process catalyzed by the phosphatase Cdc25C. This transition is influenced by a series of positive feedback loops that enhance cyclin B–Cdk1 activity.
- DNA Damage Controls:
- In response to DNA damage, the G2 to M transition is inhibited by blocking Cdc25C’s activity. When Cdc25C is phosphorylated by checkpoint kinases Chk1 and Chk2, it binds to the 14-3-3 protein, which sequesters it in the cytoplasm, preventing it from acting on preMPF, which resides in the nucleus.
- Phosphorylation by Chk2 can also reduce the catalytic activity of Cdc25C directly. Therefore, Chk1 and Chk2 play a crucial role in mediating cell cycle arrest by promoting the formation of inactive, phosphorylated cyclin–Cdk dimers, thereby preventing progression to mitosis.
- Unreplicated DNA Controls:
- In mammals, the ataxia telangiectasia and Rad3-related protein (ATR) is a key transducer that responds to unreplicated DNA and certain types of damage, such as thymidine dimers. ATR phosphorylates and activates Chk1, leading to a series of cellular responses.
- The active form of Chk1 promotes the sequestration of Cdc25 and stabilizes the tumor suppressor protein p53. This stabilization results in a halt at the S–G2 checkpoint, induction of DNA repair mechanisms, and, under severe conditions, can initiate apoptosis.
- Studies indicate that ATR and Chk1 are encoded by essential genes, as evidenced by the embryonic lethality and increased DNA damage observed in mice lacking either of these proteins. This highlights their critical role in coordinating cell cycle events, independent of external threats to genomic integrity.
- Mechanisms in Fission Yeast:
- A similar signaling pathway exists in fission yeast, where the Rad3 protein activates Chk1 and Chk2, leading to the phosphorylation and subsequent sequestration of Cdc25. Chk2 also stabilizes Mik1, a kinase that operates alongside Wee1, reinforcing the cell’s ability to prevent entry into mitosis when DNA remains unreplicated.
- These interactions demonstrate the intricate balance of signaling pathways that collectively stabilize preMPF and enforce cell cycle checkpoints.
- Size Control Mechanisms:
- In fission yeast, the regulation of cell size occurs during the G2 phase rather than G1. The kinase Wee1 is pivotal in controlling the threshold size for mitosis, as shown by experiments in which mutations in the wee1 gene led to cells dividing at substantially smaller sizes compared to wild-type cells.
- Conversely, increasing the dosage of the wee1 gene resulted in larger cells at mitosis. Cdc25 also significantly impacts cell size; for instance, overexpression of cdc25 can induce a smaller cell phenotype known as the “wee” phenotype.
- The interplay among Wee1, Cdc25, and Cdc13–Cdc2, along with upstream protein kinases like Nim1, illustrates how the nutritional status and cellular size influence the G2 to M transition, ensuring that only adequately sized and healthy cells proceed to division.
Metaphase (Spindle) Checkpoint
The metaphase (spindle) checkpoint is a crucial regulatory mechanism that ensures the proper alignment and attachment of chromosomes to the mitotic spindle before anaphase begins. This checkpoint prevents premature separation of sister chromatids, thus safeguarding genomic integrity during cell division. The function of the spindle checkpoint is vital, as it not only ensures that all chromosomes are correctly attached but also prevents the progression of the cell cycle in the presence of errors or incomplete preparations.
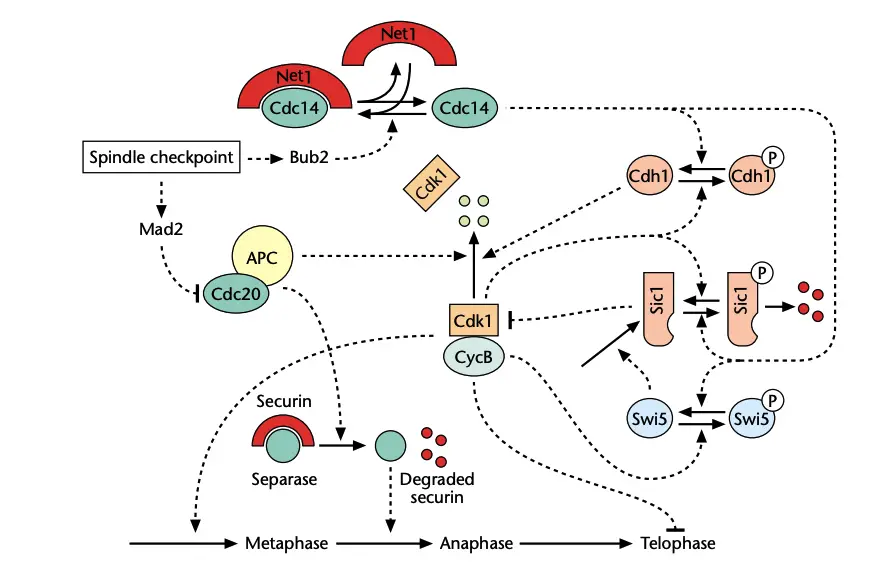
- Role of the Spindle Checkpoint:
- The spindle checkpoint guarantees that sister chromatids are separated only when all chromosomes are securely attached to the bipolar mitotic spindle via their kinetochores. This mechanism is essential for preventing aneuploidy, a condition characterized by an abnormal number of chromosomes.
- If kinetochores remain unattached, such as when cells are treated with microtubule-depolymerizing drugs, the spindle checkpoint is activated. Under these conditions, cells will not transition from metaphase to anaphase, indicating that the checkpoint effectively blocks both the metaphase-to-anaphase and anaphase-to-telophase transitions.
- Key Proteins Involved:
- The spindle checkpoint was initially identified in budding yeast, where several key proteins were discovered. Screens for mutants that failed to arrest in mitosis after drug treatment led to the identification of six crucial proteins: Bub1, Bub2, Bub3 (collectively referred to as “budding uninhibited by benzimidazole” proteins) and Mad1, Mad2, and Mad3 (mitosis arrest deficient proteins).
- These proteins are localized at kinetochores and spindle poles, where they generate signals necessary for the spindle checkpoint to function effectively.
- Transition from Metaphase to Anaphase:
- The transition from metaphase to anaphase is regulated by a negative feedback loop involving MPF (CycB–Cdk1). MPF promotes early mitotic events, including nuclear envelope breakdown and spindle assembly, while inhibiting later processes.
- Once activated, Cdc20-APC (anaphase-promoting complex) initiates anaphase by targeting securin for degradation. Securin serves to inhibit separase, a protease necessary for cleaving the cohesin proteins that hold sister chromatids together. The degradation of securin allows separase to become active, thus initiating sister chromatid separation.
- Additionally, Cdc20-APC targets B-type cyclins for degradation, effectively reducing MPF activity and facilitating the exit from mitosis.
- Mechanism of Action:
- Unattached kinetochores activate the protein Mad2, which then binds to Cdc20. This interaction inhibits Cdc20’s function, preventing the degradation of securin and mitotic cyclins, and thereby halting the cell cycle in metaphase until all chromosomes are properly aligned.
- This inhibition is critical, as it allows time for any existing issues to be resolved before the cell commits to anaphase.
- Mitotic Exit Network (MEN):
- Exit from mitosis is regulated through positive feedback loops that interact with the spindle checkpoint. In budding yeast, mitotic cyclins Clb1 and Clb2 engage in antagonistic relationships with their negative regulators, Sic1 and Cdh1.
- To exit mitosis, the upregulation of Sic1 and activation of Cdh1 are necessary, as these molecules facilitate the degradation of the mitotic cyclins. The phosphatase Cdc14 plays a central role in this process, as it must be released from the nucleolus to activate Cdh1 and stabilize Sic1.
- The release of Cdc14 from the nucleolus is contingent upon the spindle checkpoint; if a spindle is absent, Bub2 inhibits the activation of MEN, thus preventing the release of Cdc14.
- DNA Damage Response:
- In budding yeast, the metaphase checkpoint also plays a role in the response to DNA damage, as the S and M phases overlap, eliminating a distinct G2 phase.
- DNA damage activates Mec1 (a homologue of ATM/ATR), which subsequently phosphorylates and activates Chk1 and Rad53 (a Chk2 homologue). This cascade of activation leads to the inhibition of Cdc5, an APC activator, thereby maintaining high levels of Clb–Cdc28 activity and blocking anaphase.
- Moreover, Chk1 phosphorylates and stabilizes Pds1, which further promotes the attachment of sister chromatids, arresting cells at the transition from metaphase to anaphase.