What is the central dogma of molecular biology? – What is Central Dogma?
The Central Dogma of molecular biology, a pivotal concept in modern biology, delineates the flow of genetic information within a biological system. This concept, first elucidated by Francis Crick in 1957, posits a unidirectional information flow from DNA to RNA, culminating in the synthesis of proteins. It has profoundly influenced our understanding of genetic information storage, replication, and expression in living organisms.
Let us dissect the components and processes of the Central Dogma:
- DNA Replication: This initial step involves the precise duplication of DNA molecules. Occurring during cell division, it ensures the transfer of identical genetic information to daughter cells. DNA replication is fundamental in maintaining the integrity of genetic information across generations.
- Transcription: In this stage, a specific DNA segment serves as a template for synthesizing a complementary RNA molecule, particularly messenger RNA (mRNA). This process translates the genetic code from DNA to RNA, facilitating its transport to the cell’s cytoplasm.
- Translation: The final stage of the Central Dogma, translation, involves the synthesis of proteins. Here, the genetic code borne by mRNA directs the assembly of amino acids into proteins. This process occurs in ribosomes, where transfer RNA (tRNA) molecules bring amino acids in the sequence specified by the mRNA.
Therefore, the Central Dogma encapsulates a sequential and systematic flow of genetic information, starting from DNA replication, through transcription to mRNA, and culminating in protein synthesis during translation. It’s crucial to recognize that this model, while generally applicable, has its exceptions and complexities, such as post-transcriptional modifications and the roles of non-coding RNAs in gene regulation.
The Central Dogma is not just a theoretical construct but a practical guide to understanding gene expression. Gene expression, a two-stage process involving transcription and translation, begins with the conversion of DNA information into portable RNA messages. These messages then travel to the ribosomes, where they are decoded to synthesize specific proteins. This process exemplifies the intricate and precise nature of genetic information transfer.
Furthermore, the Central Dogma classifies the interactions between biopolymers, namely DNA, RNA, and proteins, into general, special, and unknown transfers. General transfers, occurring in almost all cells, include the regular flow of information through transcription and translation. Special transfers, observed under specific conditions or in certain viruses, involve processes like reverse transcription, where RNA information is converted back into DNA. Unknown transfers, believed never to occur naturally, include direct protein synthesis from DNA without mRNA involvement.
In summary, the Central Dogma of molecular biology provides a comprehensive framework for understanding the flow of genetic information in cells. It underscores the processes of DNA replication, RNA transcription, and protein translation, offering a detailed insight into the molecular basis of life. This concept remains a cornerstone in the field of molecular biology, guiding research and advancements in genetic understanding.
The flow of information can be unidirectional, and indefinitely.
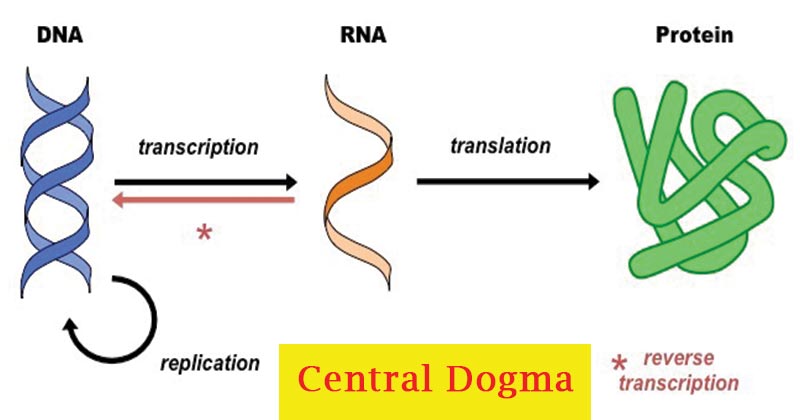
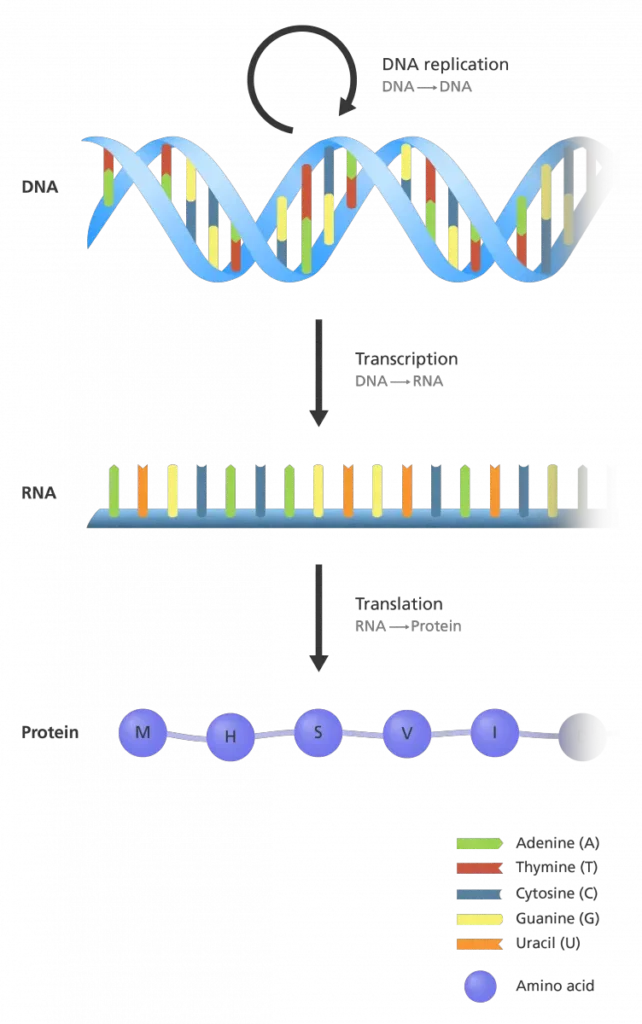
Central Dogma Steps
In the realm of molecular biology, the Central Dogma delineates the fundamental steps through which genetic information is transferred within a biological cell. This process comprises two critical stages: transcription and translation. Each step is a marvel of biological precision and complexity, involving a series of intricate molecular interactions.
- Transcription: The first phase in the Central Dogma, transcription, involves the transference of genetic information from DNA to RNA. This process is mediated by the enzyme RNA Polymerase. The DNA strand, which serves as a blueprint for this process, can be dissected into three distinct regions: the promoter, the structural gene, and the terminator.
- The strand of DNA that undergoes transcription is termed the ‘template strand’, while its counterpart is known as the ‘coding strand’.
- The initiation of transcription begins when DNA-dependent RNA Polymerase binds to the promoter region. This enzyme then facilitates the polymerization of nucleotides, proceeding in the 3′ to 5′ direction along the DNA template.
- Upon reaching the terminator sequence, transcription is concluded, culminating in the release of the nascent RNA strand. This RNA molecule then undergoes various post-transcriptional modifications to become fully functional.
- Translation: Following transcription, the process of translation marks the conversion of the RNA-encoded genetic information into specific proteins. This step is not only intricate but also energy-intensive.
- The site of translation is the ribosome, a complex molecular machine composed of a larger and a smaller subunit. The larger subunit is designed to accommodate two tRNA molecules in close proximity, facilitating the formation of peptide bonds.
- Translation commences when the mRNA strand associates with the smaller subunit of the ribosome. The tRNA molecules, each carrying a specific amino acid, bind to complementary codons on the mRNA. These tRNA molecules are situated in the larger ribosomal subunit.
- As the ribosome traverses the mRNA strand, tRNA molecules successively bind to their respective codons. Peptide bonds are formed between the amino acids they carry, thus elongating the polypeptide chain. This process repeats, sequentially adding amino acids and synthesizing long chains that fold into functional proteins.
What is Genetic Code?
- The genetic code is a fundamental aspect of molecular biology, encapsulating a set of rules that dictate how the information encoded in DNA and RNA is translated into the sequence of amino acids, the building blocks of proteins. It is essentially the language used by cells to interpret the instructions contained in genetic material, enabling the synthesis of proteins that perform a myriad of functions in the body. This code is remarkably universal and standard across a vast array of living organisms, from the simplest bacteria to complex organisms like humans.
- Understanding the genetic code is crucial for decoding the genetic instructions and predicting the amino acid sequence of proteins based on genetic information. This understanding has significantly propelled advancements in genetics and molecular biology, with wide-ranging applications in genetic engineering and biotechnology.
- The genetic code is based on nucleotides, the basic units of DNA and RNA. These nucleotides are adenine (A), cytosine (C), guanine (G), and thymine (T) in DNA, with thymine being replaced by uracil (U) in RNA. Combinations of these four nucleotides in RNA (A, U, C, G) form codons, each comprising three nucleotides.
- There are 20 standard amino acids, and the four types of nucleotides can be arranged in groups of three to form 64 different codons (4^3 combinations). Out of these 64 codons, three function as stop codons (UAA, UAG, and UGA), signaling the termination of protein synthesis. The remaining 61 codons, known as sense codons, are responsible for coding the 20 amino acids. The codon AUG holds a dual role, coding for the amino acid methionine (Met) and also serving as the start codon, initiating protein synthesis.
- The genetic code is notable for its degeneracy, meaning that multiple codons can code for the same amino acid. This feature is essential for the robustness of the genetic code, as it allows for certain mutations to occur without altering the amino acid sequence of proteins. Each codon is specific for only one amino acid, and this specificity is maintained universally across different organisms.
- In summary, the genetic code is a sophisticated system that translates genetic information into the language of proteins. Its universality, degeneracy, and specificity are key characteristics that facilitate the accurate and efficient translation of genetic information into functional proteins, essential for life. Understanding the genetic code is not only fundamental to molecular biology but also pivotal in various scientific and medical fields, offering insights into genetic diseases, guiding the development of new therapies, and advancing biotechnological innovations.
Key features of the Genetic Code
The genetic code is a quintessential component of molecular biology, embodying a set of rules by which information encoded in genetic material is translated into proteins. This code is deciphered through various key features, each playing a crucial role in the synthesis and regulation of proteins. Understanding these features is essential for comprehending how genetic information is translated into functional proteins.
- Codons: At the core of the genetic code are codons, which are groups of three nucleotides on the mRNA molecule. With four nucleotide options available (adenine, uracil, cytosine, and guanine in RNA), there are 64 possible codons (4^3 combinations). Each codon corresponds to a specific amino acid or a signal that influences the start or stop of protein synthesis.
- Start Codon: The AUG codon holds a pivotal role as the start codon, marking the commencement of protein synthesis. Intriguingly, this codon also encodes for the amino acid methionine, which means that methionine is the first amino acid in every newly synthesized protein.
- Stop Codons: Equally vital are the stop codons – UAA, UAG, and UGA. These codons do not correspond to any amino acids; instead, they signal the termination of protein synthesis. When the ribosome encounters a stop codon, it halts protein synthesis and releases the newly formed polypeptide chain.
- Amino Acid Assignments: In the genetic code, each codon is associated with a specific amino acid or a signal for the initiation or termination of translation. For instance, the codon UUU is coded for the amino acid phenylalanine, while UGA functions as a stop codon.
- Redundancy: A notable feature of the genetic code is its degeneracy or redundancy. This means that most amino acids are specified by more than one codon. Such redundancy imparts a degree of error tolerance to the genetic code, allowing certain mutations in the DNA sequence to occur without necessarily altering the amino acid sequence of the resulting protein.
- Universality: The genetic code is nearly universal across all known life forms, with only minor variations. This universality is a compelling indication of a common evolutionary origin for all life on Earth.
- Non-Coding Codons: Besides coding for amino acids, some codons serve regulatory functions within the mRNA. These non-coding codons are involved in controlling various aspects of the translation process, adding another layer of regulation to protein synthesis.
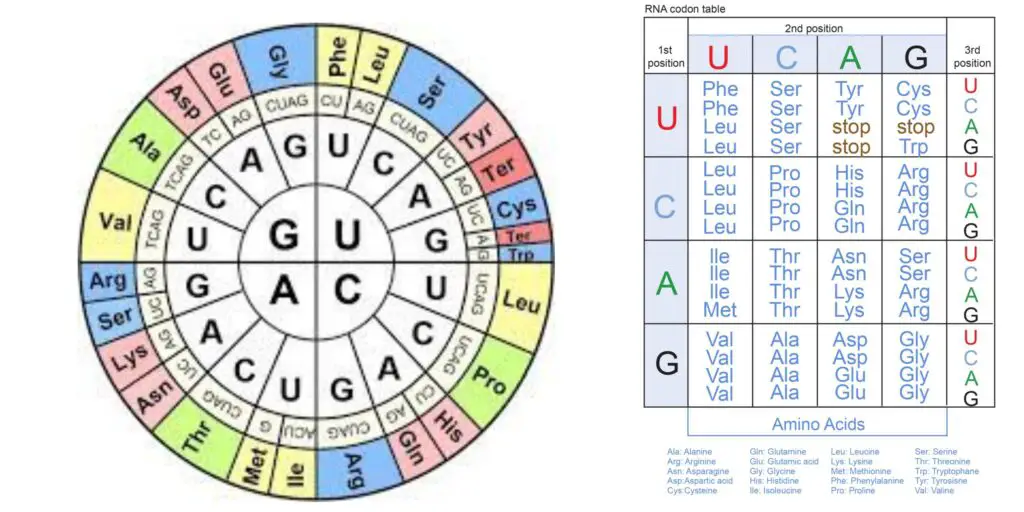
Relation between Central Dogma and Genetic Code
The Central Dogma of molecular biology and the genetic code are intrinsically linked concepts in molecular biology, collectively elucidating the flow and translation of genetic information within living organisms. Their relationship is pivotal for understanding how genetic information is stored, processed, and expressed in cells. The connection between these two fundamental concepts can be explained through the processes of transcription and translation, which are key components of the Central Dogma.
- Genetic Code and Transcription:
- Transcription, an essential process in the Central Dogma, involves the conversion of genetic information from DNA to RNA. In this process, the role of the genetic code is crucial.
- A specific segment of DNA acts as a template for the synthesis of a complementary RNA molecule, notably messenger RNA (mRNA). The genetic code is instrumental in determining how the nucleotide sequence in DNA is transcribed into the corresponding sequence in mRNA.
- This transcription process adheres to the rules of the genetic code, ensuring that the genetic information is accurately and efficiently transcribed.
- Genetic Code and Translation:
- Translation, another critical process of the Central Dogma, involves synthesizing proteins using the information encoded in mRNA. Here again, the genetic code plays a vital role.
- The genetic code defines the relationship between the codons in mRNA and the amino acids they encode. Each codon, a sequence of three nucleotides, corresponds to a specific amino acid.
- Transfer RNA (tRNA) molecules, each with a specific anticodon, recognize and pair with the appropriate codons in the mRNA, in accordance with the genetic code. The tRNA molecules transport the corresponding amino acids, allowing the ribosome to correctly assemble these amino acids into a protein.
- This translation process is governed by the genetic code, which ensures that the genetic information in mRNA is accurately translated into the amino acid sequence of proteins.
Exceptions to the Central Dogma
While the central dogma of molecular biology provides a general framework for the flow of genetic information, there are exceptions to this principle. These exceptions challenge the straightforward sequence of DNA to RNA to protein. Here are some notable exceptions to the central dogma:
- Retroviruses: Retroviruses, such as HIV, have an RNA genome and replicate by transcribing their RNA into DNA using a unique enzyme called reverse transcriptase. This reverse transcription step allows the retroviral RNA to be integrated into the host cell’s DNA. The process involves the conversion of RNA to DNA, which deviates from the central dogma. The information flow in retroviruses is RNA → DNA → RNA → protein.
- RNA Viruses: Some viruses have RNA genomes and can directly utilize their RNA to produce proteins without the need for DNA intermediates. RNA viruses, like influenza virus or SARS-CoV-2, bypass the DNA replication step and synthesize proteins directly from their RNA genome. In these cases, the information flow is RNA → protein, which is contrary to the central dogma.
- Prions: Prions are unique infectious agents composed solely of proteins. They can cause diseases like Creutzfeldt-Jakob disease and mad cow disease. Prions do not involve the transfer of genetic information from DNA or RNA; instead, they induce a conformational change in normal proteins, causing them to adopt an abnormal shape. This altered protein structure can lead to the misfolding of other normal proteins, resulting in disease progression. Prions follow a Protein → Protein information flow, which is distinct from the central dogma.
General transfers of biological sequential information
In the study of molecular biology, the Central Dogma provides a framework for understanding the general transfers of biological sequential information. These transfers, fundamental to cellular function and heredity, include the replication of DNA, transcription of DNA to RNA, and translation of RNA into protein. Each process plays a pivotal role in the maintenance and expression of genetic information.
- DNA Replication: At the heart of genetic continuity lies DNA replication, a process essential for the provision of genetic material to progeny cells, be they somatic or reproductive. The key to this process is the replication of DNA to DNA, representing a critical step in information transfer.
- The replication mechanism involves a complex group of proteins known as the replisome. This ensemble performs the intricate task of copying information from the parent DNA strand to the complementary daughter strand.
- The components of the replisome include:
- A helicase, which unwinds the superhelical and double-stranded DNA, creating a replication fork.
- SSB protein that stabilizes the unwound DNA.
- Primase, which introduces a complementary RNA primer to each template strand, serving as a starting point for replication.
- DNA polymerase III, reading the template strand from 3′ to 5′ and synthesizing the new strand from 5′ to 3′.
- DNA polymerase I, which replaces RNA primers with DNA.
- DNA ligase, linking Okazaki fragments with phosphodiester bonds to form a continuous DNA strand.
- This crucial process predominantly occurs during the S phase of the cell cycle.
- Transcription: Transcription is the next critical phase, where the information in DNA is transcribed into messenger RNA (mRNA). This process is catalyzed by enzymes such as RNA polymerase and various transcription factors.
- In eukaryotes, the initial RNA transcript, known as pre-mRNA, undergoes several processing steps. These include the addition of a 5′ cap and a poly-A tail, followed by splicing. Alternative splicing enhances the diversity of proteins that can be produced from a single mRNA.
- The end product of transcription is a mature mRNA strand, ready for translation.
- Translation: The final step in the Central Dogma is translation, where the mature mRNA is decoded to synthesize proteins.
- In prokaryotic cells, transcription and translation can occur simultaneously, while in eukaryotes, these processes are spatially separated, necessitating the transport of mRNA from the nucleus to the cytoplasm.
- Ribosomes read the mRNA triplet codons, starting typically with an AUG codon. Initiation and elongation factors facilitate the entry of aminoacylated tRNAs into the ribosome-mRNA complex, ensuring the correct match between the mRNA codon and the tRNA anti-codon.
- As amino acids are linked into a growing peptide chain, it begins to fold into its functional conformation. Translation terminates at a stop codon (UAA, UGA, or UAG).
- Post-translational modifications are often required for the protein to become fully functional. These may include proper folding aided by chaperone proteins, excision of segments (inteins), cross-linking, or attachment of cofactors.
Special transfers of biological sequential information
Special transfers of biological sequential information, as described by the Dogmas, involve unique processes that deviate from the typical flow of information in the central dogma. These special transfers include reverse transcription, RNA replication, and direct translation from DNA to protein.
- Reverse Transcription: Reverse transcription is the process in which genetic information is transferred from RNA to DNA, contrary to the usual direction of transcription. This transfer occurs in certain organisms, such as retroviruses like HIV, as well as in eukaryotes during retrotransposon activity and telomere synthesis. Reverse transcription is facilitated by enzymes called reverse transcriptases, which catalyze the synthesis of DNA from an RNA template.
- RNA Replication: RNA replication involves the copying of one RNA molecule to produce another RNA molecule. This process is observed in many viruses, where RNA-dependent RNA polymerases are responsible for copying RNA to generate new RNA strands. Interestingly, RNA-dependent RNA polymerases are also found in various eukaryotes, where they play a role in RNA silencing.
- RNA Editing: RNA editing refers to the alteration of an RNA sequence through the action of protein complexes and guide RNA molecules. It can be seen as a transfer of information from one RNA molecule to another, leading to changes in the RNA sequence. RNA editing is a significant process that contributes to the diversity of gene expression and protein variants.
- Direct Translation from DNA to Protein: In certain experimental conditions, direct translation from DNA to protein has been demonstrated in a cell-free system. Extracts from E. coli containing ribosomes, but without intact cells, were capable of synthesizing proteins from single-stranded DNA templates isolated from other organisms. This process occurred with the assistance of ribosomes and was enhanced by the presence of neomycin. However, it was unclear whether this mechanism of translation corresponded specifically to the genetic code, as further studies were required to determine the fidelity and specificity of this translation process.
These special transfers of biological sequential information showcase exceptional cases in molecular biology where the transfer of information occurs in non-traditional directions or in unique circumstances. They provide valuable insights into the versatility and adaptability of biological systems, expanding our understanding of the complexity of genetic information transfer.
Is the Central Dogma of Biology Unidirectional?
The Central Dogma of Biology is often described as unidirectional, emphasizing the flow of biological information from DNA to RNA and then to protein. However, this interpretation overlooks certain information transfers, such as DNA replication. To provide a more accurate understanding, it is important to refer back to Francis Crick’s original articulation of the Central Dogma in 1957.
According to Crick, the Central Dogma states that information transfers occur exclusively from nucleic acid (DNA and RNA) to either nucleic acid or protein. In other words, information flows from DNA to RNA through transcription and from RNA to protein through translation. This unidirectional flow covers the fundamental transfers of genetic information within living systems.
Crick’s version of the Central Dogma includes the conventional transfers that are widely accepted in molecular biology, such as transcription, translation, and DNA replication. These processes represent the primary routes of information flow in biological systems.
Additionally, Crick’s Central Dogma acknowledges the existence of a few “special transfers” that deviate from the unidirectional flow. These special transfers include reverse transcription, where genetic information is transferred from RNA to DNA, and RNA replication, which involves the copying of one RNA molecule to produce another. These special transfers represent exceptions to the typical flow described by the Central Dogma.
By recognizing Crick’s original formulation, we gain a more comprehensive understanding of the Central Dogma of Biology. It encompasses the essential transfers of genetic information while acknowledging the existence of certain special transfers that challenge the unidirectional nature of the flow. This nuanced perspective allows for a more accurate representation of the diverse processes involved in molecular biology.
Significance of the Central Dogma of Molecular Biology
- Framework for Genetic Information Flow: The Central Dogma elucidates the directional flow of genetic information, from DNA to RNA to proteins. This fundamental principle aids in comprehending how genetic information is stored, transmitted, and expressed within cells, forming the basis for various biological processes, including cell growth, differentiation, and response to environmental stimuli.
- Understanding Genetic Replication and Expression: By outlining the processes of DNA replication, transcription, and translation, the Central Dogma provides insight into how genetic information is replicated and how genes are expressed. This understanding is crucial for research in genetics, developmental biology, and evolutionary biology.
- Basis for Genetic Engineering and Biotechnology: The principles embodied in the Central Dogma are fundamental to the fields of genetic engineering and biotechnology. They provide the scientific foundation for techniques such as cloning, gene therapy, and the production of recombinant proteins, which have significant applications in medicine, agriculture, and industry.
- Medical Applications: Knowledge of the Central Dogma is pivotal in medical science, particularly in understanding genetic disorders, cancer biology, and the development of new therapeutic strategies. It enables researchers to comprehend how mutations in DNA can lead to various diseases and aids in the development of targeted treatments, including personalized medicine.
- Molecular Evolution and Phylogenetics: The Central Dogma contributes to our understanding of molecular evolution. By studying the changes in DNA and protein sequences over time, scientists can trace the evolutionary history of organisms and understand the molecular basis of evolution.
- Tool for Diagnostic and Therapeutic Techniques: Understanding the mechanisms outlined in the Central Dogma has led to the development of diagnostic techniques, such as PCR (Polymerase Chain Reaction) and gene sequencing, which are essential in identifying genetic disorders and infectious diseases. Similarly, RNA interference (RNAi) and CRISPR-Cas9 gene editing technologies, rooted in the principles of the Central Dogma, offer revolutionary therapeutic potentials.
- Understanding Limitations and Exceptions: While the Central Dogma provides a general guideline for genetic information flow, it also highlights the exceptions and complexities in biological systems, such as the existence of reverse transcription in retroviruses and the role of non-coding RNAs in gene regulation. This understanding pushes the boundaries of current scientific knowledge and encourages further research and discovery.
Central Dogma of Molecular Biology Cheat Sheet
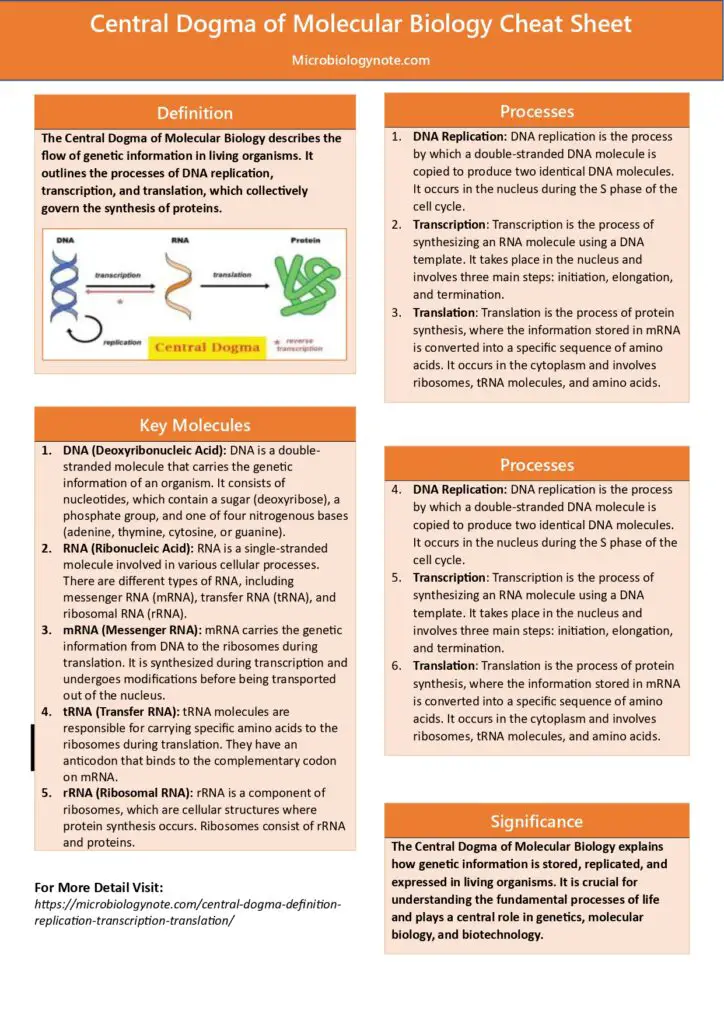
FAQ
What is the Central Dogma of molecular biology?
The Central Dogma is a concept that describes the flow of genetic information within a biological system, specifically the transfer of information from DNA to RNA to protein.
What are the three general transfers in the Central Dogma?
The three general transfers in the Central Dogma are DNA replication (DNA to DNA), transcription (DNA to RNA), and translation (RNA to protein).
What are the three special transfers in the Central Dogma?
The three special transfers in the Central Dogma are reverse transcription (RNA to DNA), RNA replication (RNA to RNA), and direct translation from DNA to protein.
Do the special transfers occur naturally in all cells?
No, the special transfers described in the Central Dogma, such as reverse transcription and RNA replication, do not occur naturally in all cells. They are observed in specific cases, such as certain viruses or under experimental conditions.
What is reverse transcription?
Reverse transcription is the process of transferring genetic information from RNA to DNA, which is the reverse of the normal transcription process. It is known to occur in retroviruses like HIV and certain eukaryotes during retrotransposon activity.
What is RNA replication?
RNA replication is the process of copying one RNA molecule to produce another RNA molecule. It is observed in many viruses, and enzymes called RNA-dependent RNA polymerases are involved in this replication process.
Can genetic information be transferred directly from DNA to protein?
In certain experimental conditions, it has been demonstrated that genetic information can be directly translated from DNA to protein in a cell-free system. However, the fidelity and specificity of this translation process compared to the natural genetic code are still under investigation.
What is the significance of the Central Dogma in molecular biology?
The Central Dogma provides a framework for understanding how genetic information is transferred and utilized within cells. It forms the basis for studying gene expression, protein synthesis, and the relationship between genotype and phenotype.
Are there any unknown transfers in the Central Dogma?
Yes, the Central Dogma describes three unknown transfers, including the synthesis of protein from protein, synthesis of RNA using the primary structure of a protein as a template, and DNA synthesis using the primary structure of a protein as a template. These transfers are not thought to naturally occur.
How does the Central Dogma contribute to our understanding of genetics and evolution?
The Central Dogma helps us understand the fundamental processes of DNA replication, transcription, and translation, which are essential for the inheritance of genetic traits and the production of proteins. By studying these processes, we can gain insights into genetic disorders, evolutionary relationships, and the functioning of living organisms at the molecular level.
References
- Central Dogma. Harvard University (2018) [Online] Available at: https://sitn.hms.harvard.edu/art/2018/central-dogma/
- The Central Dogma of Biology. London Health Science Center. (2021) [Online] Available at: https://www.lhsc.on.ca/medical-genetics-program-of-southwestern-ontario/the-central-dogma-of-biology
- Perkins, D. et al. (2005) Expanding the ‘central dogma’: the regulatory role of nonprotein coding genes and implications for the genetic liability to schizophrenia. Molecular Psychiatry. 10, pp. 69–78 https://doi.org/10.1038/sj.mp.4001577
- Cobb, M. (2017) 60 years ago, Francis Crick changed the logic of biology. PLoS Biology. 15(9): e2003243. https://doi.org/10.1371/journal.pbio.2003243
- https://www.yourgenome.org/facts/what-is-the-central-dogma/
- https://www.khanacademy.org/science/ap-biology/gene-expression-and-regulation/translation/a/intro-to-gene-expression-central-dogma
- https://learn.genetics.utah.edu/content/basics/beyond
- http://thebiologyprimer.com/the-central-dogma-echapter
- https://www.biointeractive.org/classroom-resources/central-dogma-and-genetic-medicine
- https://chemistrytalk.org/central-dogma-of-biology/
- https://www.azolifesciences.com/article/From-DNA-to-Protein3b-The-Central-Dogma-of-Molecular-Biology.aspx
سنٹرل ڈاگما کر ترجمہ مرکزی عقیدہ کرنا لفظی طور پر شاید درست ہو لیکن عملی طور پر نامناسب ہے- بیالوجی (یا عمومی طور پر سائنس) میں کوئی عقائد نہیں ہوتے، نظریات ہوتے ہیں جو شواہد کی بنا پر قائم کیے جاتے ہیں- عقائد کا بنیادی نکتہ ہی یہ ہے کہ عقائد بغیر کسی ثبوت یا مشاہدے کی قبول کیے جاتے ہیں- سنٹرل ڈاگما کی ترکیب فرانسس کرک نے 1958 میں تجویز کیا تھا جب ہمارا مائیکروبیالوجی اور جینیات کا علم نہ ہونے کے برابر تھا- اور یوں بھی فرانسس کرک نے یہ ترکیب صرف اپنی سہولت کے لیے استعمال کی تھی کیونکہ وہ ہائیپوتھیسس کی ترکیب ایک دوسرے تناظر میں پہلے ہی استعمال کر چکے تھے- اب اسے مرکزی نظریہ کہنا زیادہ مناسب ہے