- As with any prokaryote other than archaea’s cell wall, it surrounds the cell and mediates the interaction of the cell with its environment.
- It can also be used to maintain cell shape, protect against viruses, heat, acidity, or alkalinity.
- It can appear like a microsieve during the formation of pores-like structures and thus enable or disable transport processes.
- Sometimes, cells wall components may make up over 10% of total cellular protein.
- A wide range of cell envelope structures and compounds have been described and documented so far.
- The S-layer is the archaeal cell wall with the highest frequency.
- Other archaeal cell wall structures are pseudomurein, methanochondroitin, glutaminylglycan, sulfated heteropolysaccharides and protein sheaths and they are sometimes associated with additional proteins and protein complexes like the STABLE protease or the bindosome.
- Recent advancements in electron microscopy have also shown the existence of an outer (most) cellular membrane within several archaeal group, similar to the Gram-negative cell walls within bacteria.
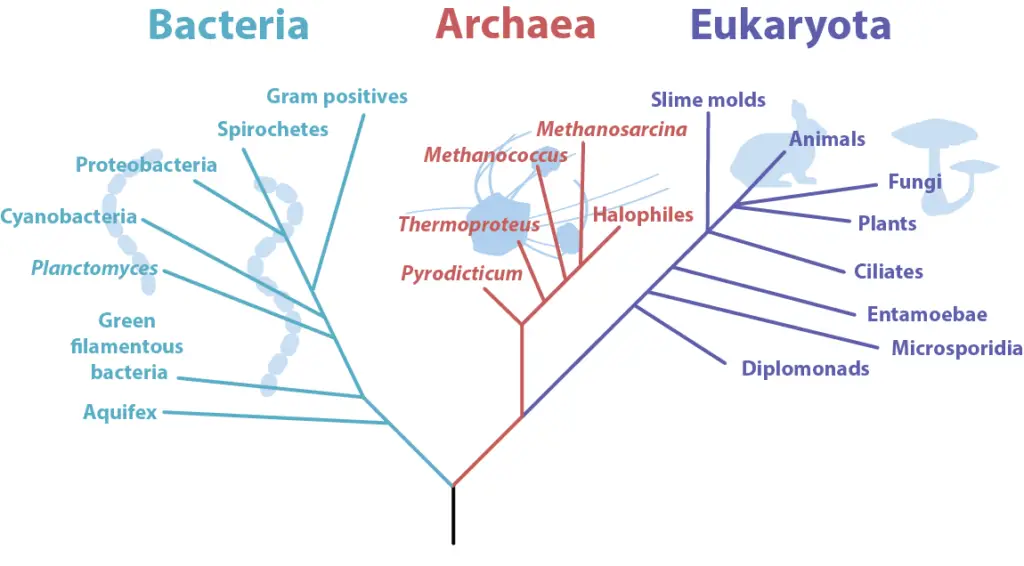
Archaeal Cell Wall Introduction
- Archaea are the third major lineage, or “domain”, after bacteria and eukaryotes.
- Since the discovery of archaeal cells walls and their first descriptions, the S-layer has been suggested as the most important and ancient archaeal envelope structure.
- The S-layer is a paracrystalline 2D crystallinity that forms paracrystalline crystal lattices with the p1- to p4-, and p6-symmetry. It is a particularly important component of organisms known as extremophiles.
- The Sulfolobales, for example, thrive at 65 to 75 degrees Celsius and a pH of around 2. They can grow in hot sulfuric acids, and the only barrier between the outside world and the cytoplasm is the S layer with a distinctive p3-symmetry.
- Pyrolobus fumarii is also the current record holder of the highest temperature for growth at 113 degrees Celsius. He is enclosed by an S-layer that has, in this instance, p4symmetry.
- It is currently accepted that the S layer was the first archaeal cell-wall to develop.
- In recent years, however, a growing number of archaeal cells envelopes have been described in a different way from Slayers and pseudomurein to methanochondroitin and protein sheaths.
- These outermost layers are made up of single and double membranes, mucous layers, and polysaccharides.
- Two microorganisms that do not have an S-layer or other solid cell walls rank among the extremophiles. They seem to have adapted to extreme environments without the protection of a S-layer: Ferroplasma Acidiphilum, and Thermoplasma species.
One Membrane Archaea
- Even though they live in extreme conditions (e.g. Ferroplasma acidiphilum, Thermoplasma species can survive in low pH levels of 1-2 and 60 degrees Celsius. They do not have any cell walls.
- These organisms are bounded only by one membrane and exhibit a pleomorphic shape.
- Thermoplasma acidophilum cells were shown to retain their integrity via mannose-rich glycoproteins, lipoglycans, and plasma membrane anchors. These forms a protective glycocalix at cell surface.
- Ferroplasma acidamanus membranes were found to contain tetraetherlipids that form monolayer membranes rather than bilayer membranes. This allows the cells to survive in acidic environments.
- These monolayer membranes are stable due to their greater resistance to hydrolysis.
- In this regard, it is possible that effective proton pumps or acid stable membrane proteins could also play a significant role.
Double Membranes of Archaea
- Different phyla of archaea can contain microorganisms with double membranes as their outermost structures.
- It is interesting that all the double-membraned Archaea mentioned so far in literature have in common the close interaction of other microorganisms.
- The architecture of double-membraned arhea is similar to the cell envelope of Gram negative bacteria.
- Three layers make up the Gram-negative cell envelope: the outer membrane, peptidoglycan and inner membrane.
- The outer membrane is composed of a bilayer that consists of an inner leaflet with phospholipids, and an outer leaflet with glycolipids (e.g. lipopolysaccharides).
- The outer membrane also contains two major classes of proteins: mainly lipoproteins and b-barrel protein.
- The inner membrane, however, forms a phospholipid bilayer.
- The periplasm, an aqueous chamber, is enclosed by both the inner-and outer membranes.
- The Gram-negative bacteria periplasmic space is approximately 10% of total cell volume. It contains a thin layer of peptidoglycan and soluble proteins, such as hydrolytic enzymes.
- These proteins are essential for substrate initial degradation, binding proteins involved in transport of substances and receptors for Chemotaxis.
- While they look very similar, the archaeal double-membrane is different from the Gram negative cell envelope in terms of biochemical composition and the function of the various compartments.
- The space between the inner and outer layers of archaea is called pseudoperiplasmic space. It is filled with pseudo-periplasm.
- The following Archaea contain Double Membranes; Crenarchaeon Ignicoccus hospitalis, ARMAN archaea (archaeal Richmond Mine acidophilic nanoorganisms), Methanomassiliicoccus luminyensis.
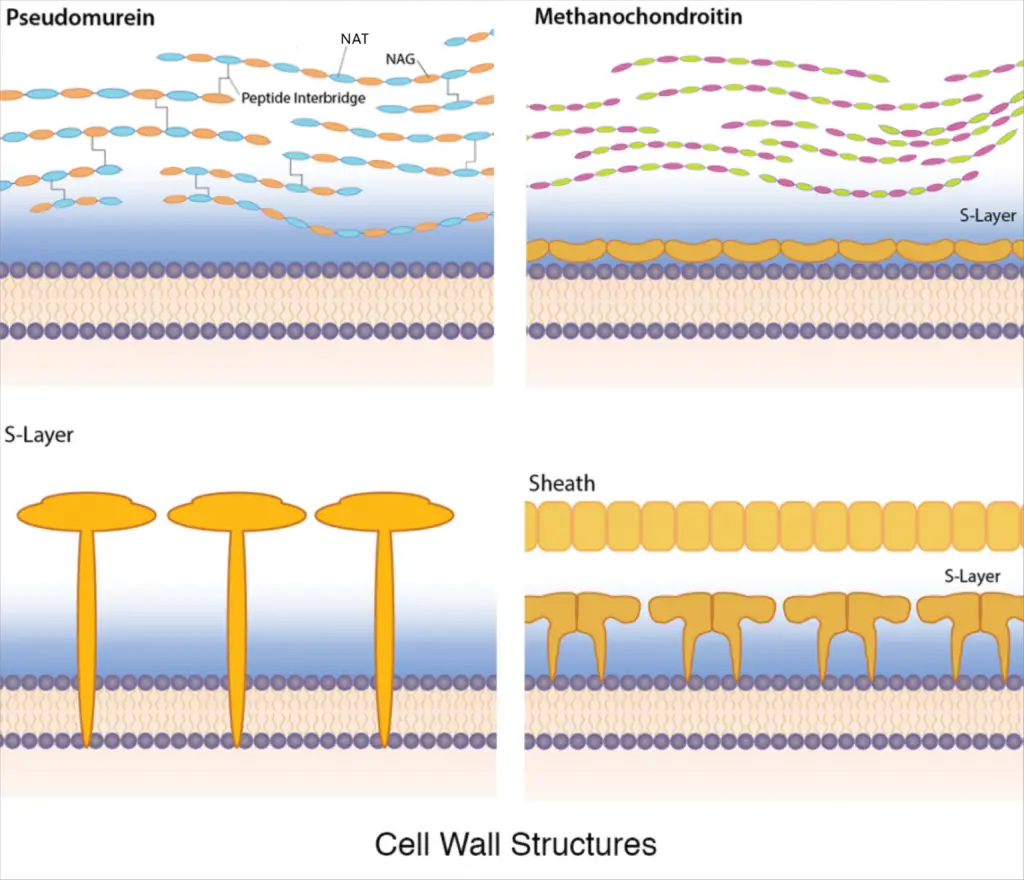
What is S-Layer?
The S-layer, or surface layer, is a fundamental component of the cell envelope in many archaea and some bacteria. It represents one of the most common cell wall variations, particularly among Crenarchaea. This layer consists primarily of proteins that self-assemble into a regular, two-dimensional array on the cell surface.
Structure and Composition
- Protein Composition: The S-layer proteins are often highly glycosylated, meaning they contain significant amounts of sugar moieties. This glycosylation is crucial, as it enhances the protein’s stability and protects it from degradation, especially in extreme environments with high temperatures and acidity.
- Assembly: S-layer proteins arrange themselves into a pseudocrystalline lattice. This lattice is typically a 2D structure, consistently patterned across the cell surface. The protein mass in the S-layer can range from 40 to 325 kDa, and these proteins form distinct symmetrical arrays.
- Anchoring: The S-layer proteins may include a large subunit that creates the primary array and a smaller subunit that functions as an anchor. This anchoring can be via a stalk-like structure, known as tetrabrachion in some species. This stalk secures the S-layer to the cytoplasmic membrane or to a pseudoperiplasmic layer of murein.
Functional Roles
- Protection: The S-layer provides robust protection against environmental stressors. This includes shielding the cell from viruses and other potentially harmful particles, thereby contributing to the cell’s defense mechanisms.
- Stability: The high glycosylation level and the 2D crystalline nature of the S-layer confer significant stability to the protein structure. This stability is crucial for withstanding extreme conditions, such as high acidity and temperature, encountered by certain archaea like those in the Sulfolobales.
- Cell Integrity: The S-layer helps maintain cell shape and integrity, particularly under fluctuating osmotic conditions. It also acts as a molecular sieve, allowing selective permeability and potentially contributing to nutrient uptake and waste removal.
- Quasiperiplasm: The space between the cytoplasmic membrane and the S-layer, termed the quasiperiplasm, is a unique feature. This area can be quite wide, up to 70 nm in some cases, such as with Staphylothermus marineus.
Structural Variability
- Symmetry and Lattice Constants: The S-layer lattice can exhibit various symmetries, such as p2 or p4, depending on the number of central crystal units. The lattice constants typically range from 11 to 30 nanometers. For instance, Sulfolobales possess glycosylated S-layers with p3-symmetry and lattice constants around 21 nm.
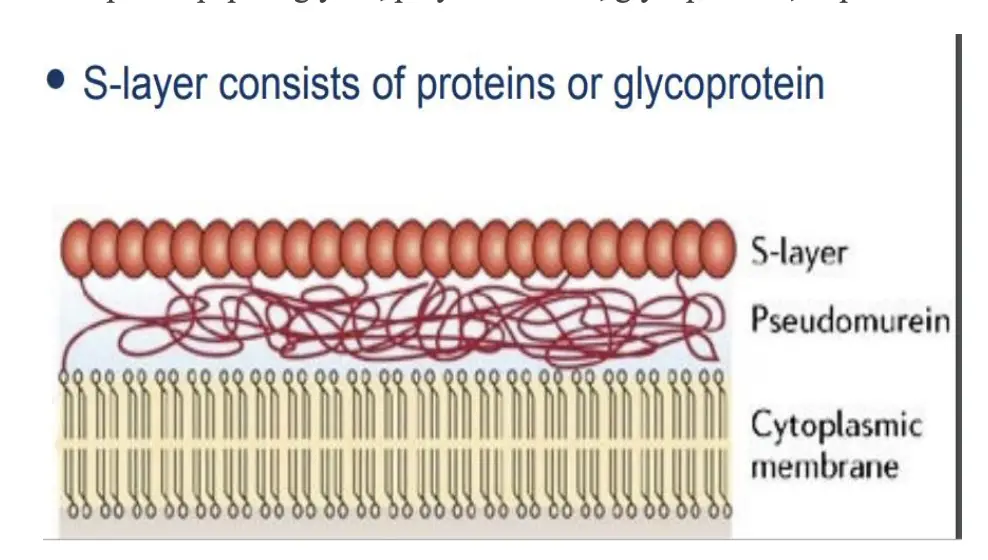
Cell Wall Structures of Methanogenic Archaea
Methanogenic archaea, a significant group within the euryarchaeal Kingdom, exhibit a remarkable diversity in their cell wall structures. This diversity reflects their varied phylogeny, physiology, and morphology. Methanogens are classified into several orders, including Methanobacteriales, Methanomicrobiales, and Methanopyrales. Their cell walls can feature pseudomurein, methanochondroitin, and S-layers, each contributing to their unique structural and functional properties.
Pseudomurein
Pseudomurein is a key component of the cell walls in Methanobacteriales and Methanopyrales. It is analogous to the peptidoglycan found in bacteria but has distinct structural differences. The core of pseudomurein consists of disaccharide chains linked by peptide subunits.
- Structure: Pseudomurein chains are built from alternating units of β(1-3)-linked N-acetyl-d-glucosamine and N-acetyl-l-talosaminuronic acid. These chains are cross-linked by peptide subunits predominantly composed of L-amino acids like glutamic acid, alanine, and lysine.
- Differences from Bacterial Peptidoglycan: Key differences include the use of β(1-3) linkages instead of β(1-4), the substitution of N-acetyl-muramic acid with N-acetyl-l-talosaminuronic acid, and the predominance of a- and e-peptide bonds. Notably, pseudomurein lacks d-amino acids found in bacterial peptidoglycan.
These structural variations contribute to the rigidity and stability of the methanogenic cell wall, distinguishing it from bacterial counterparts.
Methanochondroitin
Methanochondroitin is found exclusively in certain Methanosarcina species. It serves as a structural component, contributing to the cell’s shape and stability.
- Structure: The core structure of methanochondroitin is a trimer consisting of [-)β-d-GlcA-(1-3)-β-d-GalNAc-(1-4)-β-d-GalNAc-(1-]n. This arrangement forms a fibrillar polymer that can create either a compact or loose matrix depending on environmental conditions.
- Degradation: The integrity of methanochondroitin layers can be compromised under high osmolality, high concentrations of divalent cations such as Ca²⁺ and Mg²⁺, or nutrient deprivation. For instance, Methanosarcina barkeri shows a significant reduction in glucuronic acid under such conditions, affecting the cell wall’s structural integrity.
- Comparison to Eukaryotic Chondroitin: Methanochondroitin resembles eukaryotic chondroitin sulfate but differs primarily in the absence of sulfate residues and the molar ratios of GalNAc to GlcA.
S-Layers
In some Methanosarcina species, an additional S-layer is present. This layer is located between the cytoplasmic membrane and the methanochondroitin layer.
- Function: The S-layer contributes to cell wall protection and structural integrity. It provides a crystalline array of proteins that enhances the cell’s resistance to environmental stresses.
Types of Archaebacteria
Archaebacteria, now classified into four phyla based on ribosomal RNA sequence analyses, are distinct from other domains of life. These phyla include Euryarchaeota, Crenarchaeota, Nanoarchaeota, and Korarchaeota. Each phylum represents unique organisms adapted to various environments and conditions.
Phylum | Representative Organisms | Characteristics | Representative Micrograph |
---|---|---|---|
Euryarchaeota | – Methanogens<br>- Halobacteria | – Methanogens produce methane, a metabolic waste. <br>- Halobacteria thrive in extreme saline environments.<br>- Halobacteria have reddish blooms due to bacteriorhodopsin. | – Methanogens: Cause flatulence in humans.<br>- Halobacterium strain NRC-1: Shows reddish coloration from bacteriorhodopsin. |
Crenarchaeota | – Sulfolobus | – Carbon fixation.<br>- Many are sulfur-dependent extremophiles.<br>- Some are thermophilic or hyperthermophilic.<br>- Thrive in volcanic springs with high temperatures and low pH. | – Sulfolobus: Grows in volcanic springs at 75º-80º C and pH 2-3. |
Nanoarchaeota | – Nanoarchaeum equitans | – Contains only one known species.<br>- Obligate symbiont with Ignicoccus.<br>- Found in extreme environments like hydrothermal vents. | – Nanoarchaeum equitans: Small spheres in contact with Ignicoccus. |
Korarchaeota | – Uncultivated species | – Considered one of the most primitive forms of life.<br>- Found only in specific hot springs, like the Obsidian Pool in Yellowstone. | – Korarchaeota: Seen in the Obsidian Pool at Yellowstone National Park. |
Euryarchaeota
- Methanogens: These organisms generate methane as a byproduct of their metabolic processes. They are critical in various ecological systems, including the human gut.
- Halobacteria: Known for their ability to survive in highly saline environments. They exhibit a reddish color due to the presence of bacteriorhodopsin, a pigment related to retinal.
Crenarchaeota
- Sulfolobus: This genus is notable for its role in carbon fixation and its ability to endure extreme conditions such as high temperatures and acidic environments. It is commonly found in geothermal areas.
Nanoarchaeota
- Nanoarchaeum equitans: This species is an obligate symbiont of Ignicoccus. It was discovered in extreme environments, such as hydrothermal vents, where it forms a close symbiotic relationship with its host.
Korarchaeota
- Uncultivated Species: Korarchaeota are among the most ancient forms of life, found only in very specific environments like the Obsidian Pool. Their cultivation in laboratory settings has yet to be achieved, making them a subject of interest in studies of early life forms.
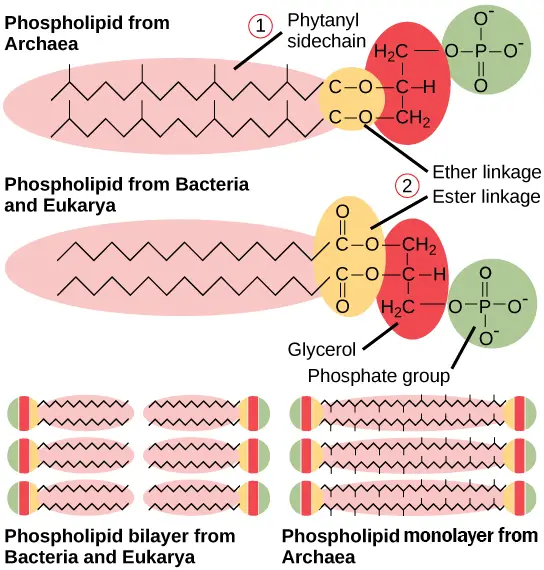
Key Differences Between Archaea and Bacteria
1. Plasma Membrane
The plasma membrane of Archaea and Bacteria displays significant differences in structure and composition.
- Lipid Composition: Archaea possess unique lipid membranes compared to Bacteria. Archaea use ether linkages between glycerol and isoprenoid chains, whereas Bacteria have ester linkages in their phospholipids. This ether linkage in Archaea provides enhanced chemical stability.
- Glycerol Chirality: In Archaea, the glycerol backbone is in the L-isomeric form. In contrast, Bacteria and Eukaryotes feature the D-isomeric form. This chirality affects membrane fluidity and stability.
- Monolayer vs. Bilayer: Archaea can have a monolayer plasma membrane, where isoprenoid chains from one phospholipid interconnect with those from the opposite side. Bacteria and Eukaryotes typically have a bilayer structure, with two separate layers of lipids.
2. Cell Wall
The cell walls of Archaea and Bacteria differ notably in composition and structure.
- Peptidoglycan Absence: Archaea lack peptidoglycan, a key component of Bacterial cell walls. Instead, Archaea may have various cell wall types, including proteinaceous S-layers. Some Archaea might lack a cell wall entirely.
- S-Layer: Many Archaea feature a protein or glycoprotein S-layer that forms a crystalline array. In Bacteria, an S-layer is an additional structure, not a primary component of the cell wall.
- Pseudomurein and Methanochondroitin: Some Archaea contain pseudomurein, similar to peptidoglycan but with different chemical links. Methanochondroitin, a polymer similar to vertebrate connective tissue, is present in some archaea, providing structural support.
3. Ribosomes
- Size and Shape: Both Archaea and Bacteria have 70S ribosomes. However, archaeal ribosomes differ in rRNA nucleotide sequences and protein composition. This distinction contributes to their resistance to certain antibiotics that target bacterial ribosomal functions.
4. Unique Structures
- Cannulae: Exclusive to some Archaea, these hollow tube-like structures connect cells after division, potentially anchoring them to surfaces and forming networks.
- Hami: These long, helical tubes with hooks assist in cell attachment and community formation, unique to Archaea.
- Pili: Present in both Archaea and Bacteria, archaeal pili are protein structures adapted from bacterial pili, facilitating surface attachment.
- Flagella: Archaeal flagella differ markedly from bacterial flagella. Archaeal flagella use ATP for rotation, unlike the proton motive force in bacteria. The filament is composed of various types of flagellin proteins and grows by adding proteins to the base rather than the tip. Unlike bacterial flagella, archaeal flagella do not exhibit run-and-tumble motility but instead rotate to propel the cell.
5. Classification
Currently, Archaea are classified into two recognized phyla: Euryarchaeota and Proteoarchaeota. Additional phyla such as Nanoarchaeota and Korarchaeota are proposed but not officially recognized, primarily due to limited environmental sequence data.
Understanding these distinctions helps clarify why Archaea and Bacteria, though both prokaryotic, are fundamentally different in their cellular structures and functions.
Feature | Archaea | Bacteria |
---|---|---|
Plasma Membrane | – Ether linkages between glycerol and isoprenoid chains<br>- L-isomeric form of glycerol<br>- Can be a monolayer | – Ester linkages between glycerol and fatty acids<br>- D-isomeric form of glycerol<br>- Always a bilayer |
Cell Wall | – Lacks peptidoglycan<br>- May have proteinaceous S-layer or no cell wall<br>- Can contain pseudomurein or methanochondroitin | – Contains peptidoglycan<br>- S-layer is an additional structure, not a primary component |
Ribosomes | – 70S size<br>- Unique rRNA nucleotide sequences and proteins<br>- Antibiotic resistance due to ribosomal differences | – 70S size<br>- Common rRNA nucleotide sequences and proteins |
Unique Structures | – Cannulae: Hollow tubes connecting cells<br>- Hami: Helical tubes with hooks for attachment<br>- Pili: Protein structures for attachment<br>- Flagella: ATP-powered, varied flagellin proteins | – Similar structures in some cases but evolved independently from those in Archaea |
Classification | – Two recognized phyla: Euryarchaeota, Proteoarchaeota<br>- Additional proposed phyla: Nanoarchaeota, Korarchaeota, Aigarchaeota, Lokiarchaeota | – Various phyla, including Proteobacteria, Firmicutes, Actinobacteria, etc. |
Structural Differences and Similarities between Bacteria and Archaea
Structural Character | Archaea | Bacteria |
---|---|---|
Cell Type | Prokaryotic | Prokaryotic |
Cell Morphology | Variable | Variable |
Cell Wall | Does not contain peptidoglycan | Contains peptidoglycan |
Cell Membrane Type | Lipid bilayer or lipid monolayer | Lipid bilayer |
Plasma Membrane Lipids | Phytanyl groups, ether-linked phospholipids | Fatty acids, ester-linked phospholipids |
References
- Albers, SV., Meyer, B. The archaeal cell envelope. Nat Rev Microbiol 9, 414–426 (2011). https://doi.org/10.1038/nrmicro2576
- Meyer, B.H. and Albers, S.-V. (2022). Archaeal Cell Walls. In eLS, John Wiley & Sons, Ltd (Ed.). https://doi.org/10.1002/9780470015902.a0000384.pub3
- Klingl A, Pickl C, Flechsler J. Archaeal Cell Walls. Subcell Biochem. 2019;92:471-493. doi: 10.1007/978-3-030-18768-2_14. PMID: 31214995.
- https://en.wikipedia.org/wiki/Archaea#Cell_wall_and_archaella
- https://courses.lumenlearning.com/wm-biology2/chapter/archaea-vs-bacteria/