What is Cell Division?
- Cell division is a fundamental process through which organisms generate new cells. This process is crucial for growth, development, repair, and reproduction. Different types of cell division occur depending on the organism involved. Prokaryotes, such as bacteria, divide through a relatively simple process called binary fission, while eukaryotic organisms, which include plants, animals, and fungi, typically use more complex mechanisms like mitosis and meiosis.
- In eukaryotes, mitosis is the process by which a cell divides to produce two genetically identical daughter cells. This type of division is essential for growth and repair in multicellular organisms. During mitosis, the cell’s genetic material, contained in the form of chromosomes, is duplicated and equally distributed between the two daughter cells, ensuring each receives a full set of genes. Mitosis is responsible for a wide range of cellular activities, from replacing dead or damaged cells, to the development of tissues and organs.
- Meiosis, on the other hand, is a form of cell division that is specifically associated with sexual reproduction. Unlike mitosis, meiosis results in four non-identical daughter cells, each with half the number of chromosomes as the parent cell. This reduction in chromosome number is crucial because it ensures that when gametes (sperm and egg cells) fuse during fertilization, the resulting offspring have the correct number of chromosomes. Additionally, meiosis promotes genetic variation through processes such as recombination and independent assortment, which shuffle the genetic material and create unique combinations of genes.
- Cell division is not merely a passive event but is driven by various biological factors. For instance, in multicellular organisms, cells divide to replace worn-out, damaged, or dead cells. This is particularly evident when tissues are injured; for example, when a skin wound occurs, cells divide to fill the gap and restore the integrity of the tissue. Furthermore, cell division is necessary for growth in living organisms. While it may seem like an organism’s cells are simply growing as it expands, growth is primarily due to the division of cells into new, smaller units that collectively support the organism’s increasing size.
- In humans, for instance, approximately two trillion cells divide every day. This staggering number highlights the importance of efficient and accurate cell division processes in maintaining the overall health and function of the body. From the development of embryos to tissue repair and immune response, cell division plays a vital role in maintaining the balance of life.
Definition of Cell Division
Cell division is the process by which a single cell divides to produce two or more new cells, essential for growth, repair, and reproduction in living organisms.
Discovery of Cell Division
The discovery of cell division is a fundamental aspect of cellular biology, providing insights into the mechanisms that govern growth, reproduction, and development in organisms. The concept of cell division, particularly mitosis, was gradually uncovered through the work of several pioneering scientists. Each discovery built upon the observations of previous researchers, contributing to a comprehensive understanding of how cells divide and replicate.
- 1879: Dr. Schleicher
Dr. Schleicher is credited with coining the term ‘karyokinesis,’ derived from Greek words: “karyo” meaning nucleus, and “kinesis” meaning division. This term described the process of the nucleus dividing during cell division. This marked a significant step in the scientific understanding of how cells reproduce and grow. - 1882: Walter Flemming
The German biologist Walter Flemming, one of the founding figures of cytogenetics, made important contributions to the study of cell division. Flemming observed that, in addition to the division of the nucleus, thread-like structures were also equally divided. He named this process mitosis (from Greek “mito” meaning thread, and “sis” meaning division). This was the first comprehensive description of the process by which chromosomes divide and ensure that genetic material is passed on to daughter cells. - 1888: Waldeyer
Building on Flemming’s work, the scientist Waldeyer made an important observation that the thread-like structures, which Flemming had previously noted, could be stained. He named these structures chromosomes (from Greek “chroma” meaning color, and “soma” meaning body) because they absorbed color during staining. This naming helped identify chromosomes as essential components of the cell that were integral to the process of mitosis. - T. O. Caspersson
Later, T. O. Caspersson contributed to the understanding of the molecular events during mitosis by observing that DNA replication occurred during prophase. Prophase, being one of the early stages of mitosis, is where the chromosomes become visible, and the process of DNA replication prepares the cell for subsequent division. - 1925: Polyster et al.
In 1925, Polyster and colleagues used a quantitative method known as Feulgen micro-spectrophotometry to study DNA content during the different phases of the cell cycle. By passing light through cells at various stages, they were able to measure the DNA content in different phases of mitosis. Their findings revealed distinct patterns in DNA content during each stage of the cell cycle:- Interphase: 4C (indicating the cell has replicated its DNA but is not yet dividing)
- Prophase: 4C (the chromosomes are condensed, but the nuclear division has not occurred yet)
- Metaphase: 4C (chromosomes are aligned in the middle of the cell, ready for separation)
- Anaphase: 2C (the sister chromatids are separated and moving toward opposite poles)
- Telophase: 2C (the chromatids are now at opposite poles, and the cell is nearing the end of mitosis)
- Cell: 2C (final DNA content after division, where each daughter cell contains half the DNA content of the original cell).
Types of Cell Division
The primary types of cell division include binary fission, meiosis, and mitosis, each with unique characteristics and functions.
- Binary Fission:
- This is the simplest form of cell division, primarily occurring in single-celled organisms, such as bacteria.
- During binary fission, the parent cell duplicates its genetic material and divides into two identical daughter cells.
- The process begins with the replication of the cell’s DNA, followed by the elongation of the cell. Then, a septum forms, eventually splitting the cell into two separate entities.
- Therefore, binary fission enables rapid reproduction, allowing bacterial populations to grow exponentially under favorable conditions.
- Mitosis:
- Mitosis is the process through which somatic (body) cells divide to create identical copies of themselves.
- This type of cell division is essential for growth, tissue repair, and asexual reproduction in multicellular organisms.
- Mitosis consists of several stages: prophase, metaphase, anaphase, and telophase, followed by cytokinesis.
- Prophase: Chromatin condenses into visible chromosomes, and the nuclear membrane begins to break down.
- Metaphase: Chromosomes align at the cell’s equatorial plane, preparing for separation.
- Anaphase: Sister chromatids are pulled apart toward opposite poles of the cell.
- Telophase: Chromatids reach the poles, and the nuclear membrane re-forms around each set of chromosomes.
- Cytokinesis: The cytoplasm divides, resulting in two genetically identical daughter cells.
- Almost all body cells undergo mitosis, including those in the eyes, skin, hair, and muscles, highlighting its role in maintaining tissue homeostasis and responding to injury.
- Meiosis:
- Meiosis is a specialized form of cell division that occurs in sexually reproducing organisms, leading to the formation of gametes (sperm and egg cells).
- Unlike mitosis, meiosis results in four non-identical daughter cells, each containing half the chromosome number of the original cell.
- This reduction is crucial for maintaining the species-specific chromosome number during fertilization.
- Meiosis consists of two consecutive divisions: meiosis I and meiosis II, each with its own stages.
- Meiosis I: Homologous chromosomes pair and exchange genetic material through a process called crossing over, then separate into two daughter cells.
- Meiosis II: Similar to mitosis, the sister chromatids of each chromosome separate, resulting in four haploid cells.
- Therefore, meiosis not only produces gametes but also contributes to genetic diversity within a population through recombination and independent assortment.
Eukaryotic Cell Division
Eukaryotic cell division occurs primarily through two distinct processes: mitosis and meiosis. Each process plays a vital role in maintaining the organism’s growth, reproduction, and genetic diversity.
Mitosis
Mitosis is the more common form of cell division in eukaryotic organisms and results in two genetically identical daughter cells. This process is essential for growth, development, and tissue repair. The following stages outline the progression of mitosis:
- Prophase:
- During prophase, the nuclear envelope begins to break down, and chromosomes start to condense, making them visible under a microscope.
- The centrioles (in animal cells) move toward opposite poles of the cell, initiating the formation of the mitotic spindle, a structure made of microtubules that will help separate the chromosomes.
- Organelles like the nuclear envelope, endoplasmic reticulum, and Golgi complex are disassembled or no longer visible.
- Chromosomal material condenses, ensuring the chromosomes are compact and ready for alignment.
- Metaphase:
- By this stage, the nuclear envelope has fully disintegrated, and the chromosomes are fully condensed.
- The chromosomes align along the metaphase plate, an imaginary line at the center of the cell.
- Spindle fibers connect to the chromosomes via their kinetochores (protein structures at the centromere) and centrosomes.
- The centromere holds the two sister chromatids together at this stage, making it the most appropriate time to study chromosomal morphology under a microscope.
- Anaphase:
- Anaphase begins when the sister chromatids (or homologous chromosomes in meiosis) are pulled toward opposite poles of the mother cell by the spindle fibers.
- Each chromosome advances toward the cell pole, with the centromere leading and the arms trailing behind.
- This separation ensures that each daughter cell will receive an identical set of chromosomes.
- Telophase:
- During telophase, the chromatids, now considered individual chromosomes, reach the opposite poles of the cell.
- The DNA starts to de-condense and revert to its chromatin form, and a new nuclear envelope forms around each set of chromosomes, re-establishing the nuclei in the daughter cells.
- The cell is now nearing the end of mitosis, with the nuclear material enclosed in two distinct nuclei.
Meiosis
Meiosis, on the other hand, is crucial for the production of gametes (sperm and egg cells) and is responsible for generating genetic diversity. Meiosis occurs in two main stages, each consisting of several phases similar to those in mitosis, but with key differences. Meiosis I reduces the chromosome number by half, while meiosis II resembles mitosis.
Meiosis I
- Prophase I:
- Prophase I is significantly longer and more complex than prophase in mitosis.
- Chromosomes undergo homologous recombination, and crossing-over occurs, where segments of chromatids are exchanged between homologous chromosomes, leading to genetic variation.
- Prophase I is further divided into five sub-stages (leptotene, zygotene, pachytene, diplotene, and diakinesis), each with unique chromosomal behaviors.
- Metaphase I:
- Homologous chromosomes align along the metaphase plate.
- Microtubules from opposite spindle poles attach to the kinetochores of each homologous chromosome.
- Anaphase I:
- During anaphase I, homologous chromosomes are separated to opposite poles, but the sister chromatids remain attached at this stage.
- Telophase I:
- The nuclear membrane reforms around the separated chromosomes, and cytokinesis follows, dividing the cytoplasm and resulting in two daughter cells.
- Chromosomes do not de-condense into their interphase form but remain somewhat dispersed.
- After telophase I, a brief resting phase called interkinesis occurs, preparing the cells for the second meiotic division.
Meiosis II
- Prophase II:
- Prophase II begins after cytokinesis without DNA replication. The nuclear membrane disappears, and the chromosomes condense again.
- Metaphase II:
- Chromosomes align along the metaphase plate of the two daughter cells.
- Microtubules link the sister chromatids to opposing spindle poles.
- Anaphase II:
- In anaphase II, the centromere of each chromosome splits, allowing the sister chromatids to move toward opposite poles of the cell.
- Telophase II:
- The separated chromatids reach the cell poles, and the nuclear envelope reforms around each set of chromosomes.
- Cytokinesis occurs, leading to the formation of four genetically distinct, haploid daughter cells.
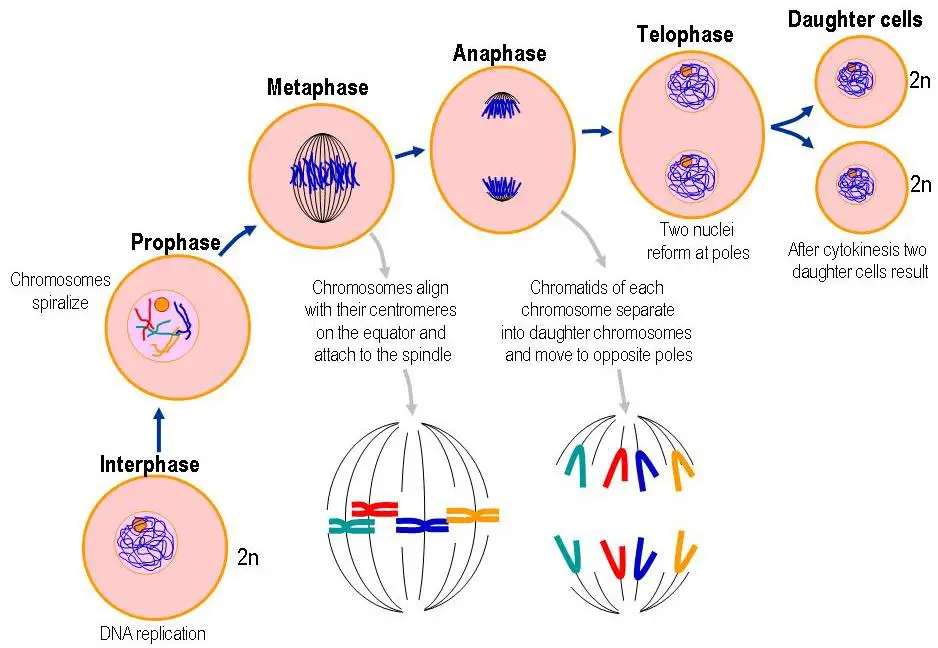
Prokaryotic Cell Division
Prokaryotic cell division is a straightforward yet essential process for the reproduction and survival of prokaryotic organisms, such as bacteria. Unlike eukaryotic cells, prokaryotes do not possess complex internal structures like organelles, and their cell division is relatively simple. The primary method of prokaryotic cell division is binary fission, a form of asexual reproduction, though budding can also occur in some cases.
- Simple Process:
- Prokaryotic cell division is less complex compared to eukaryotic cell division. This simplicity arises because prokaryotes lack many of the specialized organelles found in eukaryotic cells, such as a nucleus or membrane-bound organelles.
- As a result, the cell division process for prokaryotes is essentially a way of reproducing, ensuring the continuation of the organism.
- DNA Replication and Division:
- The first critical step in prokaryotic cell division is the replication of the DNA. Prokaryotes typically have a single, circular DNA molecule, which replicates to form two identical copies.
- After replication, these two copies are segregated to opposite ends of the cell, allowing for the division of genetic material between the two future daughter cells.
- Therefore, the process starts with DNA replication followed by the splitting of the two identical genetic copies.
- Cell Elongation:
- Following DNA replication, the cell elongates to accommodate the duplicated genetic material. This elongation allows the cell to prepare for the final step of division, ensuring that the genetic material is appropriately separated and that the cellular contents are evenly distributed between the two daughter cells.
- Septum Formation:
- One of the key steps in prokaryotic cell division is the formation of the septum, a cell wall structure that begins to form at the cell’s midsection during the final stages of division.
- The septum is composed of peptidoglycan, a complex polymer that provides structural support to the cell. As the septum forms, it grows inward, eventually leading to the complete separation of the two daughter cells.
- This septum formation is crucial for ensuring the physical division of the parent cell into two distinct, independent daughter cells.
- Daughter Cells:
- Once the division is complete, the resulting daughter cells are fully separated and capable of independent function. Each daughter cell is equipped with all the necessary components to carry out vital cellular processes such as metabolism, growth, and replication.
- Therefore, the daughter cells are genetically identical and functionally autonomous.
- Rapid Cell Division:
- Prokaryotic cell division is remarkably fast under optimal conditions. In environments where nutrients are abundant, prokaryotic cells can divide rapidly, sometimes generating multiple generations within a short span of time.
- This rapid division enables prokaryotic populations to grow quickly and adapt to changing environmental conditions.
- Genetic Stability:
- Despite the simplicity of binary fission, prokaryotic cell division ensures substantial genetic stability. The replicated DNA is faithfully divided and distributed between the daughter cells, maintaining consistency in genetic material across generations.
- Adaptability and Evolution:
- Prokaryotic cell division also contributes to the organism’s adaptability and evolutionary processes. Through binary fission, beneficial mutations can accumulate over time, allowing prokaryotes to evolve and adapt to new environments.
- The process of genetic variation is facilitated by factors like plasmid transfer, which may occur during division or through other mechanisms, contributing to genetic diversity within a population.
- Plasmids and Divisome:
- In addition to the main chromosomal DNA, prokaryotes may also carry plasmids, small, circular DNA molecules that replicate independently of the chromosomal DNA. During binary fission, plasmids are also replicated and distributed to the daughter cells.
- The divisome is a protein complex that plays a central role in prokaryotic cell division. It ensures the correct constriction of both the inner and outer membranes during division.
- Additionally, the divisome facilitates proper remodeling of the peptidoglycan cell wall at the division site, ensuring that the septum forms correctly.
- An important component of the divisome is FtsZ, a tubulin-like protein that forms a contractile ring around the middle of the cell, helping to pinch the cell membrane and separate the daughter cells during the final stages of division.
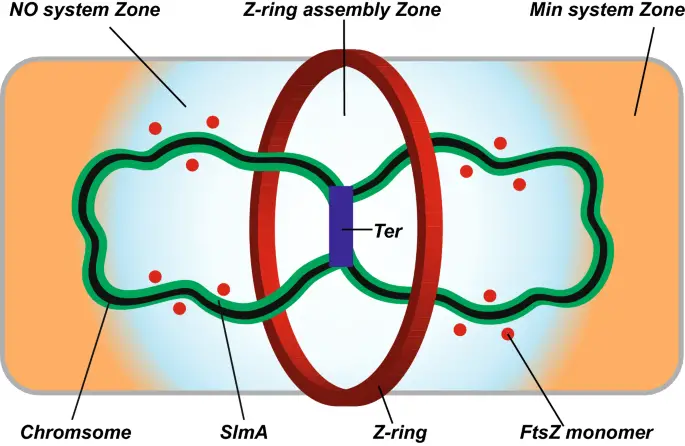
Phases of the Cell Cycle
The cell cycle is a fundamental process that governs the growth, replication, and division of cells. It consists of two primary phases: Interphase and the Mitosis Phase (M-phase). During interphase, the cell prepares for division by growing and replicating its genetic material, while the M-phase is where the actual division of the cell takes place. Understanding each phase of the cell cycle is essential for comprehending how cells maintain their integrity and function through successive generations.
- Interphase:
Interphase is often referred to as the “resting phase” of the cell cycle. However, it is far from inactive, as many critical processes occur during this phase. The cell undergoes significant growth, and its genetic material is replicated in preparation for division. Interphase is subdivided into three distinct stages:- G1 Phase (Gap 1):
During this phase, the cell undergoes significant growth but does not replicate its DNA. It continues to perform its normal cellular functions, which vary depending on the type of cell. For instance, a nerve cell may perform its role in transmitting signals, while other cells may be involved in producing proteins or metabolizing nutrients. - S Phase (Synthesis):
The primary event in the S-phase is DNA replication. The cell’s entire genetic material is duplicated, ensuring that each daughter cell will receive a complete set of chromosomes during mitosis. This replication process is essential for maintaining the genetic integrity of the organism. - G2 Phase (Gap 2):
After DNA replication is complete, the cell enters the G2 phase, during which it continues to grow. Additionally, the cell synthesizes proteins and other molecules required for the upcoming mitotic division. The G2 phase serves as a final preparation period before the cell enters the M-phase.
- Quiescent Stage (G0):
Some cells, like nerve cells, may exit the G1 phase and enter a state known as G0. In this stage, cells are no longer actively preparing to divide and remain in a quiescent state. Cells in G0 are essentially inactive regarding replication and division but continue to perform essential functions within the organism.
- G1 Phase (Gap 1):
- M Phase (Mitosis Phase):
The M-phase represents the actual division of the cell and consists of two processes: karyokinesis and cytokinesis. Karyokinesis is the division of the nucleus, while cytokinesis is the division of the cytoplasm. Mitosis is subdivided into four distinct stages:- Prophase:
Prophase is divided into two sub-stages: early prophase and late prophase. During early prophase, the chromosomes condense, becoming visible under a microscope. The nuclear envelope begins to break down, and spindle fibers start to form. In late prophase, the nuclear envelope fully disintegrates, and the spindle fibers extend across the cell, attaching to the chromosomes at specialized regions called kinetochores. - Metaphase:
In this stage, the chromosomes align along the metaphase plate, a region equidistant from the two poles of the cell. The chromosomes consist of two sister chromatids connected by a centromere. Microtubules from the mitotic spindle attach to the kinetochores of each chromosome, ensuring that the chromatids are properly positioned for separation. - Anaphase:
Anaphase marks the separation of the sister chromatids. The centromeres split, and the sister chromatids are pulled toward opposite poles of the cell by the spindle fibers. This ensures that each daughter cell will receive a complete set of chromosomes. - Telophase:
During telophase, the cell nears completion of its division. The separated chromatids reach the opposite poles of the cell and begin to de-condense, returning to their chromatin form. New nuclear envelopes form around each set of chromosomes, creating two distinct nuclei. The mitotic spindle disassembles as the cell prepares for cytokinesis.
- Cytokinesis:
Cytokinesis is the final step of the M-phase. It is the process by which the cytoplasm and cellular components are divided into two daughter cells. In animal cells, a cleavage furrow forms, pinching the cell membrane inward until the two daughter cells are separated. In plant cells, a cell plate forms, eventually leading to the formation of a new cell wall between the two daughter cells. Cytokinesis ensures that each daughter cell receives a complete set of organelles and other cellular materials.
- Prophase:
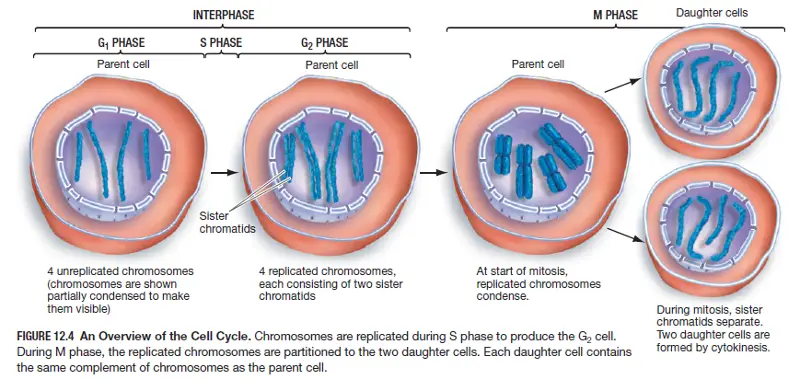
Functions of Cell Division
Below are the primary functions that cell division facilitates, each of which plays a critical role in an organism’s life cycle:
- Reproduction and the Generation of New Offspring:
- Cell division is a fundamental process for reproduction in both unicellular and multicellular organisms.
- In single-celled organisms, such as bacteria, cell division (typically through binary fission) is the primary method of reproduction. A single parent cell divides to produce two genetically identical offspring.
- In multicellular organisms, cell division plays a vital role in sexual reproduction. Through meiosis, gametes (sperm and egg cells) are produced, each carrying half of the organism’s genetic material. When these gametes fuse during fertilization, they create a new organism with a complete set of chromosomes.
- Growth and Proliferation of the Organism:
- Cell division is essential for the growth of organisms, both in size and complexity.
- During development, a fertilized egg undergoes numerous rounds of mitosis, leading to the formation of tissues and organs as the organism grows. This process ensures that the organism’s cells increase in number, allowing it to achieve the size and complexity necessary for survival.
- Besides growth, cell division also facilitates the proliferation of cells, which is essential for maintaining the organism’s health and function over time.
- Regeneration and Repair of Damaged Tissues:
- Cell division plays a crucial role in repairing and regenerating tissues that have been injured or damaged.
- For instance, when the skin is cut, the body relies on cell division to replace the damaged cells and restore the tissue’s integrity. Similarly, cells divide to repair organs that have been injured due to trauma or disease. This process ensures the organism’s ability to recover from physical damage and maintain its health.
- Therefore, cell division not only supports growth but also plays a vital role in maintaining and repairing the body’s various structures.
- Formation of Gametes for Genetic Continuity:
- Meiosis, a form of cell division, is critical for the formation of gametes—sperm and egg cells—which are necessary for sexual reproduction.
- Gametes contain half the number of chromosomes of a regular somatic cell, ensuring that when they combine during fertilization, the resulting offspring will inherit the correct number of chromosomes. This process is essential for the continuity of species, as it allows genetic material to be passed from one generation to the next.
Significance of Cell Division
Cell division is a fundamental biological process that plays a crucial role in the growth, repair, and reproduction of organisms. There are two primary forms of cell division—mitosis and meiosis—each with distinct significance for different aspects of an organism’s lifecycle. Below is an explanation of the significance of these two processes.
Significance of Mitosis
Mitosis is vital for maintaining cellular functions and promoting growth and repair in multicellular organisms. It ensures that cells reproduce efficiently, producing two genetically identical daughter cells. The following points highlight the key significance of mitosis:
- Genetic Consistency: Mitosis typically produces diploid daughter cells that contain the same genetic information as the parent cell, ensuring that the genetic makeup is preserved from one generation of cells to the next.
- Growth and Development: Mitosis is the driving force behind the growth of multicellular organisms. It allows organisms to increase in size by increasing the number of cells, which is necessary for their overall development. This process is essential during embryonic development, as well as during the growth of tissues in adults.
- Nucleus-Cytoplasm Ratio: As cells grow, their volume increases, impacting the nucleus-to-cytoplasm ratio. To restore this ratio, cells undergo mitosis, ensuring that the newly formed cells maintain a functional balance between the nucleus and the surrounding cytoplasm.
- Cell Healing and Repair: Mitosis is critical for the repair of damaged tissues. Certain cells in the body, such as blood cells, the lining of the stomach, and the skin’s epidermal cells, are regularly replaced through mitosis. This helps maintain the integrity and functionality of these tissues.
- Continual Growth in Plants: In plants, mitosis facilitates growth throughout their entire lifespan. In particular, meristematic tissues, located in regions like the tips of roots and shoots, continuously divide and contribute to apical (vertical) and lateral growth, helping plants to develop throughout their lives.
Significance of Meiosis
Meiosis, by contrast, is specialized for the formation of gametes and plays a critical role in sexual reproduction and genetic diversity. It introduces variation into populations, which is crucial for evolution. The following points outline the significance of meiosis:
- Preservation of Chromosome Number: Meiosis ensures that sexually reproducing organisms maintain a stable chromosome number across generations. By halving the chromosome number during the formation of gametes (sperm and egg cells), meiosis prevents the doubling of chromosomes that would otherwise occur if gametes were diploid.
- Genetic Variation: A primary function of meiosis is to increase genetic variation within populations. Through processes such as crossing-over and the independent assortment of chromosomes, meiosis produces gametes with unique combinations of genes. This variability is essential for the adaptability and survival of species.
- Evolutionary Impact: The genetic variations produced by meiosis are the raw material for evolutionary processes. As organisms with beneficial genetic traits are more likely to survive and reproduce, meiosis contributes to natural selection and evolution.
- https://www.pw.live/exams/school/cell-division/
- https://www.biologyonline.com/dictionary/cell-division
- https://www.geeksforgeeks.org/cell-division/
- https://plantlet.org/cell-division-types-stages-processes/
- https://www.worksheetsplanet.com/what-is-cell-division/